Abstract
A calibrated Rhizocarpon spp. lichen growth curve spanning the last 680 yr was developed for the Bella Coola and Mount Waddington areas, southern Coast Mountains of British Columbia (Canada). It is based on 18 control surfaces whose ages were determined using radiocarbon dates, tree-ring dated moraines, and ice front positions derived from historical air photographs. Population distribution statistics were used to assess the validity of each control lichen measurement. The relationship is characterized by logarithmic growth during the first 100 yr following surface stabilization, and is described as linear for the successive 600 yr. Application of the curve at Tiedemann Glacier revealed evidence for six periods of late-Neoglacial moraine formation in ca. AD 620, 925, 1118, 1392, 1575, and 1621. The study confirms the importance of incorporating a multisite approach and a lichen population dynamic assessment. Large samples sizes and replication are also noted as necessary in order to adequately assess any dating errors.
Introduction
Lichenometric dating is based on the assumption that the largest lichen growing on a suitable substrate provides an approximation of the minimum age of exposure of this surface (CitationAndrews and Webber, 1969; CitationGellatly, 1982; CitationInnes, 1985; CitationMcCarthy, 1997). Lichenometry is commonly used in arctic and alpine environments to provide relative ages for glacially deposited surfaces, where trees are unavailable for dendrochronological dating or where radiocarbon dating is impractical (e.g., CitationSmith et al., 1995). Beschel was the first to use this technique in the European Alps, and since then it has been widely applied (CitationBeschel, 1961, Citation1973; CitationPorter, 1981; CitationSmith et al., 1995; CitationHarrison and Winchester, 2000; CitationSmith and Desloges, 2000). The most widely used lichen growth curves have been developed for Rhizocarpon spp. (CitationGellatly, 1982; CitationInnes, 1985; CitationAndré, 1986; CitationBroadbent and Bergqvist, 1986; CitationRodbell, 1992; CitationSolomina and Calkin, 2003).
The application of lichenometric techniques necessitates the construction of local or regional lichen growth curves (i.e., a plot of diameter vs. age). Growth curves are normally sigmoidal in shape, as they encompass growth patterns that include a period of great-growth, uniform-growth, and senescence (CitationInnes, 1985). Two approaches have been developed in order to describe lichen growth rates in a given area: one “traditional” and another “statistical”. The statistical approach is normally used to build growth curves based on large sample sizes that are restricted to the single largest lichen found on individual boulders. Statistical models are used as means of interpreting thallus-size distributions (CitationMcCarroll, 1994; CitationBull et al., 1995; CitationBull and Brandon, 1998; CitationMcCarthy, 1999; CitationReynolds, 2001). This approach seems particularly useful for dating multiple events on diachronic surfaces, such as snow avalanche boulder (CitationMcCarroll, 1994) and rockfall deposits generated by earthquakes (CitationBull and Brandon, 1998).
The basic assumption of the traditional method is that the largest lichen growing on a rock surface or other suitable substrate is the oldest one, and thus provides a minimum date for the deposition or exposure of this surface (CitationAndrews and Webber, 1969; CitationGellatly, 1982; CitationMcCarthy, 1997). Although some researchers use only the largest lichen found at a site to provide a relative surface age, others establish the mean diameter of the 5, 10, 20, 25, or 50 largest lichens to reduce the impact of anomalously large lichens (CitationBradley, 1985; CitationInnes, 1985; CitationMcCarroll, 1994; CitationBull et al., 1995). Traditional growth curves are constructed by establishing the size of lichens found growing on surfaces of known age (CitationGellatly, 1982; CitationInnes, 1985). The most commonly used surfaces include gravestones and glacial moraines that have been independently dated using historical records, radiocarbon dating or dendrochronology (e.g., CitationLewis, 2001; CitationWiles et al., 2002; CitationSolomina and Calkin, 2003).
Both the traditional and the statistical approaches to lichenometry introduce systematic errors that are related to imprecise dating of the age-calibrated surface involved (CitationGellatly, 1982; CitationBradley, 1985; CitationRodbell, 1992; CitationBull and Brandon, 1998; CitationSolomina and Calkin, 2003). If the sites to be dated are quite distant from the location where the growth curve is constructed, a calibration may be necessary in order to correct for any spatial variability in the growth rate. Other notable issues inherent to lichenometry include the application of curves that include several species of Rhizocarpon (CitationLuckman, 1977; CitationWiles et al., 2002); and that do not reflect growth trends associated with different geologic substrates (e.g., CitationPorter, 1981) or regional climate variability (e.g., CitationOsborn and Taylor, 1975).
In the southern Coast Mountains of British Columbia, an aggregate Rhizocarpon spp. curve was developed by CitationSmith and Desloges (2000) for the Bella Coola and Monarch Icefield areas. Twelve control points were included in the analysis, with surface age being derived from low elevation gravestones (55 m a.s.l.) and high-elevation (1300–1450 m a.s.l.) tree-ring dated moraines (including correcting factors for ecesis, missing pith, and height of coring). The relationship between thallus diameter and surface age covers a period of 20 to 165 yr.
The purpose of this paper is to verify and extend the applicability of the Bella Coola Rhizocarpon spp. lichen growth curve developed by CitationSmith and Desloges (2000) to the Mount Waddington region (map sheet 92N, 1:50,000) in the British Columbia Coast Mountains (). The Bella Coola (BC) curve was suggested as representative of the regional growth of Rhizocarpon spp., as it is well constrained by both high- and low-elevation control points that display consistent lichen growth trends (CitationSmith and Desloges, 2000). Additional control points developed during the course of our study of late-Neoglacial glacial activity in the Mount Waddington area provided the opportunity to extend the curve back to ca. 680 cal. yr BP and to increase its spatial applicability (CitationLarocque and Smith, 2003). In this paper, we provide previously unpublished data on our control points and calibrate a new lichen curve for the Mount Waddington area.
Study Area
The study area is located on the lee side of the Coast Mountains of British Columbia (Canada), between latitudes 51°14′N and 51°36′N (). It is located at the northern limit of the Cascade magmatic arc that formed in response to the subduction of the Juan de Fuca Plate under the North American Plate (CitationHickson, 1994). The Coast Mountains are largely composed of intrusive granodiorites and granites, volcanic andesites and rhyolites, and metamorphosed sedimentary and volcanic rocks (CitationHickson, 1994; CitationMonger and Journeay, 1994). Some of the highest peaks in British Columbia are found in this area, with Mount Waddington reaching an elevation of 4019 m a.s.l.
Although the climate of high-elevation sites within the Mount Waddington region is unknown, precipitation totals decrease substantially from west to east within this portion of the Coast Mountains. While maritime climate conditions at Bella Coola (52°22′N, 126°41′W, 18 m a.s.l.; CitationMeteorological Service of Canada, 2002) result in annual precipitation totals of 1677 mm yr−1, rain shadow influences on the east side of the Coast Mountains result in precipitation totals averaging only 426 mm yr−1 at Williams Lake (52°11′N, 122°03′W, 940 m a.s.l.) in the Interior Plateau. Within the Mount Waddington area, precipitation totals at the most representative climate stations at Big Creek (51°43′N, 123°02′W, 1128 m a.s.l.) and Tatlayoko Lake (51°40′N, 124°24′W, 853 m a.s.l.) average 334 mm to 429 mm yr−1, of which 30 to 40% falls as snow. Mean annual air temperatures at the two stations average between 2.2 to 4.2°C, with winter minimum and summer maximums averaging −9 to −17.5°C and 18.6 to 22.7°C, respectively (CitationMeteorological Service of Canada, 2002).
Five sites were selected for detailed lichenometric study and analysis: Tiedemann, Oval, Whitesaddle, Razor Creek, and Cathedral glaciers. The study sites range in elevation from 500 m a.s.l. at Tiedemann Glacier to 1675 m a.s.l. at Whitesaddle Glacier. All the surveys reported were undertaken below the local treeline and within glacial forefields established following maximum late-Neoglacial advances. Variations in temperature and precipitation are expected between the sites, as aspect, elevation, and local factors are not homogenous.
Methodology
The largest lichen thallus is considered the most appropriate parameter for providing the best estimate of surface age in lichenometric studies because its size is proportional to the length of time that the surface has been exposed or stabilized, and it represents the highest potential growth (CitationInnes, 1985). In this study, the largest diameter of near circular thalli from the genus Rhizocarpon was measured using digital calipers (precision of ±0.1 mm) and applied to calibrate the BC curve to conform to the methodology used by CitationSmith and Desloges (2000; pers. comm.). No distinction between rock types was made as their influence is considered minimal (CitationO'Neal and Schoenenberger, 2003), but lichens were mostly associated with andesitic, granodioritic, and granitic rocks. Anomalous lichens (noncircular, damaged, competing, merging, wrapped around corners) or those found below snow avalanche zones were systematically rejected.
As species of Rhizocarpon cannot be differentiated by visual inspection, 10 random lichen samples were identified (K. A. Glew, Department of Biology, University of Puget Sound) using chemical spot tests and microscopic descriptions of apothecia (reproductive structures) using the identification keys produced by CitationRunemark (1956), CitationThomson (1967, Citation1997), CitationPoelt (1988), and CitationPurvis et al. (1992). This subset of lichens was from different locations and was found growing on a variety of rock lithologies.
Wherever possible, a minimum sample of 30 largest thalli was measured at each sampling point over a search area of ca. 100 m2. The search areas were kept deliberately small in order to exclude any possible misinterpretation due to moraine morphology. However, because of the nature of some control points (one boulder or a small group of boulders; see control sites description), smaller search areas and fewer thalli (n = 7) were also included. In total, 11 control points were established by using historical air photographs to position glacier fronts, by dating historical structures, by applying radiocarbon dating, and by establishing the ages of living trees found growing on lateral moraines. A statistical procedure is proposed to determine the validity of the selected control sites (c.f. CitationBradwell, 2001). Frequency histograms of sampled lichen populations on each site were produced to assess the normality of the distribution and the presence of outliers. Sampling of largest lichens in other studies has shown that populations follow in most cases a Gaussian distribution (CitationSmirnova and Nikonov, 1990; CitationBull et al., 1994; CitationMcCarroll, 1994; CitationBull and Brandon, 1998; CitationMcCarthy, 1999). Moreover, analysis of thallus measurements (on largest lichens, n ≥ 30) used to date end and lateral moraines in the Mount Waddington area (CitationLarocque and Smith, 2003), showed that 91.5% of all samples (n = 142 moraines) were normally distributed. Where the sample size was greater than 30 and there was a possibility that older lichen might be included in the sample (e.g., colonization of supraglacial debris), a Shapiro-Wilk normality test was employed to identify non-normally distributed lichen samples (CitationPearson et al., 1977). When a non-normal distribution was detected, outliers were rejected. Where the sample size was smaller than 30, the largest thalli were not eliminated, as the risks of underestimating surface ages are greater with fewer individuals and the statistical significance is greatly reduced.
The selected control measurements were added to the BC curve (CitationSmith and Desloges, 2000) to evaluate the degree of correspondence between Bella Coola–Monarch Icefield and Mount Waddington regions. Selected points were translated into line equations defining the best fit of a particular statistical lichen growth relationship for the Bella Coola/Waddington (BCW) area. Following the methods used by CitationRodbell (1992), CitationReynolds (2001), and CitationO'Neal and Schoenenberger (2003), 95% confidence intervals were established in order to assign age-specific error estimates. Detailed lichenometric sampling of morphologically prominent lateral and end moraine sequences at Tiedemann Glacier were undertaken along survey transects to evaluate the sensitivity of our calibrated curve.
Results
LICHEN IDENTIFICATION
The majority of lichens submitted were identified as Rhizocarpon geographicum (L.) DC. with two thalli identified as Rhizocarpon macrosporum Räsänen (). While the Rhizocarpon geographicum (L.) DC. were found on a variety of substrates, the Rhizocarpon macrosporum Räsänen thalli were both found growing on fine-grained andesitic substrates. Although this species has been reported to grow at a faster rate than R. geographicum (CitationLuckman, 1977), its limited occurrence in the Mount Waddington area suggests the majority of lichen measured were likely Rhizocarpon geographicum (L.) DC.
LICHEN GROWTH CURVE
The lichen growth curve presented (BCW curve) is derived from control points established in the Bella Coola–Monarch Icefield (CitationSmith and Desloges, 2000) and from new sites in the Mount Waddington area (; see CitationSmith and Desloges [2000] for details of control points used to construct the original BC curve). Although these two areas are ca. 100 km apart, both include independently dated control sites located above 1300 m a.s.l. on the lee side of the Coast Mountains where the environmental conditions are relatively homogenous.
In the Mount Waddington area, 11 potential control points were examined to further calibrate the BCW ():
At Tiedemann Glacier (51°19′N; 124°54′W), aerial photographs dating from 1954 to 1994 (BC1849-76; BC5148-69; BC7859-203; BCC286-95; BCC94039-178) were used to map the position of the receding ice front. Lichens were measured at 50-m intervals along a valley-bottom transect that extended downvalley from the 2000 ice-front position. Photograph overexposure in 1954 and intense glaciofluvial activity in the ice proximal area in 1965 greatly reduces the validity of the lichen measurements taken at sites 19 and 20. Lichen samples from sites 8 and 19 include abnormally large lichens that failed the Shapiro-Wilk normality test (, ), which resulted in the deletion of three measurements at site 8 (sizes: 27.5, 22.1, and 21 mm) and four measurements at site 19 (sizes: 40, 34.9, 32.7, and 30.4 mm). Lichen measurements from sites 19 and 20 were not included in the analysis leading to the BCW growth curve presented in . The remaining three control sites are considered reliable in local and regional dating application.
At Oval Glacier (51°29′N; 125°15′W), control point 16 was derived from a subfossil log found partially buried by a large boulder on the distal slope of a prominent moraine. The maximum lichen diameter (34.4 mm) measured on the boulder provides an additional dated control point. Annual growth rings in the subfossil log were crossdated to a local living subalpine fir tree-ring chronology using standardized dendrochronological techniques (CitationStokes and Smiley, 1968), giving a kill date of 1864 that provides a minimum age for moraine formation.
Radiocarbon dated subfossil wood found at Whitesaddle Glacier (51°36′N; 124°50′W) extends the BCW curve from 165 yr to 680 yr. Calibrated radiocarbon dates of approximately 30 outer rings (#18: 760 ± 50 14C yr BP, Beta-166885; #23: 540 ± 60 14C yr BP, Beta-165115) were obtained from two in situ stumps killed by distal spillage from the crest of the outermost lateral moraine. Lichens found growing on boulders included in a debris tongue directly related to the killing of the stump at site 18 were used to establish a control point dated to 680 cal. yr BP (max. diameter: 85 mm; ). A lack of lichen on boulders overlying the second in situ stump (#23) and the lack of geomorphological evidence for a distal collapse of the moraine suggest the date is not as reliable. As a result, only the control point established at site 18 was considered reliable.
Lichens found growing on a rock cairn constructed during a geological survey in 1958 on a terminal moraine at Razor Creek Glacier (site 21; 51°34′N; 124°46′W) were measured and initially included in the BCW curve. As shown on , however, the largest lichen (9.4 mm) found on the cairn is well beyond the 95% confidence interval envelope and leads to an overestimation of surface age if integrated in the growth curve. It is likely that growth conditions (e.g., wind desiccation) on the raised structure may lead to slower annual increment rates.
At Cathedral Glacier (site 22; 51°14′N; 124°52′W), located on the west side of the Homathko Icefield, maximum lichen diameters (94.9 mm) on the crest of the outermost lateral moraine do not correspond to the cross-dated age of a partially buried bole (). Given that the bole was found in a cavity of the distal moraine slope, proximal to a snow avalanche path, it was likely killed by a snow avalanche in ca. 1691. The multimodal distribution of the largest lichens sampled supports this hypothesis (). For this reason, this point was not integrated in the BCW curve.
Comparison of radial growth trends of lichens found growing in the Bella Coola and Mount Waddington areas indicates they share similar age-size relationships. For instance, the radial measurements from the low elevation Hagensborg graveyard site show a close association for younger ages to those measured at Tiedemann Glacier (sites 3, 5, 7, and 8; ). The new BCW Rhizocarpon spp. lichen growth curve includes the 12 control points included in the original BC curve and six new points from the Mount Waddington area (; ).
The first part of the BCW Rhizocarpon spp. curve is best described as a logarithmic relationship:
with an r
2 of 0.981 (P < 0.000; ). Using this relationship, the lichen ecesis interval is estimated at 9 yr (± 3 yr), which is similar to the 7- to 8-yr delay in lichen establishment found by CitationO'Neal and Schoenenberger (2003) in the northern Cascades. The great growth interval extends from 9 to 60 yr (0 to 25.4 mm thallus diameter). The average growth rate during this period of time is about 0.52 mm yr−1. Between 60 and 165 yr, the growth rate decreases to 0.28 mm yr−1. Uniform growth, characterized by a linear function, starts at 100 yr and was estimated from the junction of the logarithmic and the straight-line functions. After 165 yr, only one radiocarbon dated calibration point is included in the curve. The line equation between 100 and 680 yr (32 to 85 mm thallus diameter) is:
While this portion of the curve from 165 to 680 yr could not be described by regression analysis, the average rate of radial growth (0.13 mm yr−1) suggest that as lichens age, they experience a linear growth.
An error envelope was defined based on 95% confidence intervals of the regression analysis and the calibrated radiocarbon date of site 18. This error is minimal between 20 and 28 yr, where the measurements are numerous and form a compact group. For a 25-yr-old surface (13.6 mm lichen), the error is estimated to be +6 and −5 yr. In contrast, the inherent dating error increases to +45 and −30 yr for a 150-yr-old surface, as the number of points decreases. A large difference exists with the confidence intervals of the regression equation including the deleted points (#19–21). Using wider confidence intervals, a 150-yr-old surface is bracketed by +159 and −84 yr, which leads to meaningless age estimates. An error of +42 and −44 is found on a 300-yr-old surface, with decreasing negative errors to −31 yr on a surface dated at 650 yr and an increasing positive values almost doubling (+77) for the same surface age.
Application of the BCW Lichen Growth Curve to Tiedemann Glacier
The reliability of the BCW lichen growth-rate curve for surface-age dating was assessed at Tiedemann Glacier, where two nested sets of lateral moraines have been described by previous researchers (CitationRyder and Thompson, 1986). While the outer set of well-weathered and forest-covered moraines is ascribed to the broadly recognized Tiedemann glacial advance (early-Neoglacial) at ca. 3300 14C yr BP, the inner sequence of largely unvegetated moraine ridges is associated with distinct late-Neoglacial advance-retreat episodes that were initiated prior to 900 14C yr BP (CitationFulton, 1971; CitationRyder and Thompson, 1986).
A lichenometric survey in July 2000, focused on samples collected along eight transects which crossed the best preserved of the late-Neoglacial moraine crests on the northern perimeter of the Tiedemann Glacier forefield (). Moraine crests were numbered according to their position on the transects (moraine 1 being the most distal), with moraine numbers from different transects at a site not necessarily being synchronous. The steep proximal slopes of the lateral moraine show evidence of massive slope failures and it is difficult to correlate the individual moraine crests (transects d to h) solely on the basis of their morphological characteristics. Transects positioned within distal sections of the forefield (a to c) revealed the presence of four nonfragmented and relatively well-preserved terminal moraines (). The most distal late-Neoglacial moraines on transects d to h exhibit a high degree of surface weathering. In general, the limited vegetation cover present on these late-Neoglacial moraines and their low elevation (ca. 700–900 m) suggests that lichen colonization patterns have probably not been influenced by either lingering snowpacks or competition. Independent dating control for moraine ages was obtained from radiocarbon and tree-ring dates, and existent North American Pacific Coast Neoglacial histories.
Lichenometric surveys were completed at 24 measurement sites (), which enabled a good replication of moraine dates. Data from three did not pass the Shapiro-Wilk normality test and these dates were therefore carefully analyzed. For the data analysis a conservative approach was adopted, so as to not discard abnormally large lichen where no sign of disturbance was noted; especially if smaller lichen populations were sampled. As a result, three outliers were removed from the distribution at sites A2 (91.2 mm), B2 (88.8 mm), and F1 (183 mm).
Using the BCW lichen growth curve equations, dates of substrate stabilization associated with an ± error envelope were calculated (), and transposed onto a frequency histogram (). Two dates do not show consistency with the transect moraine sequences: moraine D1 (AD 1499) is younger than D2 (AD 947), and G2 (AD 1600) is younger than G3 (AD 1472). At these sites, evidence of lichen disturbance by vegetation was encountered.
Six periods of late-Neoglacial moraine formation were recorded at Tiedemann Glacier, with minimum dates of AD 620, 925, 1118, 1392, 1575, and 1621. The lichenometric interpretations of multiple late-Neoglacial glacial events are supported by the findings of previous research efforts in the Pacific Northwest (e.g., CitationDesloges and Ryder, 1990). A 7th-century glacial advance (620–684) was recorded at moraines E1 and F1, which shared similar morphological characteristics (accentuated weathering and similar emplacement). Supporting evidence of a pre-Little Ice Age advance at Tiedemann Glacier was reported by CitationFulton (1971) from an 8th-century basal peat deposit (1270 ± 140 14C yr BP: AD 719, 746, 769) and by CitationRyder and Thomson (1986) from a glacially transported log (1330 ± 65 14C yr BP: AD 674). Other supporting evidence for a widespread glacial advance in the Coast Mountains at this time comes from Bridge Glacier, where an in situ glacially-sheared stump recovered in 2002 provided an age of 1500 ± 80 14C yr BP (AD 430–650), and from Alaska where investigations record the onset of a glacial advance in the Kenai Fjords (AD 596 to 653; CitationWiles and Calkin, 1991, Citation1993, Citation1994; CitationWiles et al., 1995), at Tebenkof Glacier (AD 590 to 695; CitationWiles et al., 1999), at Sheridan Glacier, and at Icy Bay (AD 560 to 601; CitationCalkin et al., 2001).
Lichen measurements at D2, G1, and at location H1 indicate that a succeeding late-Neoglacial moraine-building episode occurred between AD 925 and 947. Despite the fact that the moraines are difficult to correlate on the basis of morphological characteristics, we believe the multiple and well-replicated lichen dates adequately support a 10th-century glacial event at Tiedemann Glacier. Although regional evidence for glacial activity during this period is sparse, advances in the St. Elias and Wrangell Mountains (AD 700 to 900; CitationDenton and Karlén, 1973) and Kenai Fjords (AD 897 to 968; CitationWiles and Calkin, 1993) may correspond to this event.
Lichen at A1, B1, C1, and possibly F2, suggest that a third glacial advance in the 12th century (1118–1310) led to the construction of moraines. This moraine-building event is presumably correlative to synchronous advances recorded at Klinaklini Glacier (900 ± 40 14C yr BP: AD 1160) in the Coast Mountains (CitationRyder and Thomson, 1986) and in the Monashee Mountains (910 ± 130 14C yr BP: AD 1071, 1079, 1128; CitationLowdon and Blake, 1975; referenced by CitationRyder and Thomson, 1986).
Measurements at A2, B2, and possibly G3, record a period of moraine building lichenometrically-dated to between AD 1392 and 1472. Prominent proximal slope failures at C2 have removed any corresponding evidence of this event. In the southern British Columbia Coast Mountains, there is evidence that Colonel Foster Glacier (AD 1397; CitationLewis, 2001), Purgatory Glacier (460 ± 50 14C yr BP: AD 1439; 480 ± 50 14C yr BP: AD 1434; CitationDesloges and Ryder, 1990), Jacobsen Glacier (400 ± 60 14C yr BP: AD 1468; CitationDesloges and Ryder, 1990), and other glaciers described by CitationRyder and Thomson (1986) were advancing and building moraines at this time. Synchronous advances in the Kenai Mountains (Bering: AD 1420, Gravingk: AD 1442, Tustemana: AD 1460, Bear, Exit, and Yalik glaciers: AD 1460) highlight the widespread nature of this activity.
Lichen dating to the late-16th century (1575–1641) corresponds to moraine-building events at A3, B3, C3, D3, and E2. The morphological continuity of the sampling locations supports this assumption. The moraine-building event may correlate to a high-water stage of glacially dammed Lake Alsek in 1582 (CitationClague and Rampton, 1982). Evidence for advanced terminal positions at Mount Baker (early-1500s to AD 1559; CitationHeikkinen, 1984) and Mount Rainier (AD 1519, 1528, 1552; CitationSigafoos and Hendricks, 1972) in Washington state, at Hubbard Glacier in the Kenai Mountains (AD 1587 to 1650; CitationWiles and Calkin, 1994; 2001; radiocarbon date intercepting the calibration curve at AD 1525, 1558, 1631; CitationBarclay et al., 2001), point to the probability that this was a regional event.
The most recent moraine-building episode recorded at Tiedemann Glacier dates to between AD 1621–1780. This interpretation is supported by a glacially transported log (300 ± 60 14C yr BP: AD 1637) located by CitationRyder and Thomson (1986) at a site farther up-valley and a tree-ring date of 1624 obtained on moraine C4 (CitationLarocque and Smith, 2003). This early-17th century advance closely matches time-synchronous moraines located at sites throughout the region (CitationClague and Mathewes, 1996; CitationSmith and Laroque, 1996; CitationLewis, 2001).
Discussion
In the Pacific Northwest of North America and Alaska, nine lichen growth curves exist that are relevant to this paper (, ). CitationSolomina and Calkin (2003) reviewed five lichen growth curves previously developed in Alaska and proposed new relationships. Four of these are integrated in the following comparison, the exception being the curve that CitationDenton and Karlén (1973) produced for the White River Valley and Skolai Pass in southeastern Alaska. Their lichenometric curve was based on the maximum diameter of the largest lichen measured at 13 dated control surfaces dominated by andesitic substrates, located between 1100 and 1700 m a.s.l. Their lichen growth curve shows an initial period of rapid growth, followed by a near linear period of growth that lasted for at least 2780 14C yr (wood within organic layer). In the same general area, CitationWiles et al. (2002) developed a Rhizocarpon growth curve for the Wrangell Mountains that was based on maximum lichen diameters from nine dated control sites between 61 and 202 yr in age. The difference noted after 100 yr between the two curves may be related to more maritime conditions encountered at the control sites incorporated by CitationWiles et al. (2002).
In northern Alaska, 14 control sites were used from CitationCalkin and Ellis (1980, Citation1984) to develop a 1264-yr-old lichen growth curve along a 120-km transect located in the Central Brooks Range up to 1700 m altitude (CitationSolomina and Calkin, 2003). Farther south at Seward Peninsula, on the western maritime portion of Alaska, seven control sites originally provided by CitationCalkin et al. (1998) were reinterpreted into a 217-yr-long lichen-surface age relationship integrating low elevation growth (<600 m a.s.l.). In the southern Alaska Range, CitationSolomina and Calkin (2003) used the lichen control measurements provided by CitationBijkerk (1980), CitationPéwé and Reger (1983), and CitationBegét (1994) to determine lichen growth on up to 3708-yr-old surfaces. The last relevant curve from Alaska is located on the maritime Kenai Peninsula, in the Gulf of Alaska, and was developed using Rhizocarpon section Alpicola (CitationWiles, 1992; CitationWiles and Calkin, 1994, CitationSolomina and Calkin, 2003). The relationship between lichen growth and surface age is limited to 264 yr.
CitationLewis (2001) and CitationLewis and Smith (2004) constructed a Rhizocarpon growth curve for Vancouver Island, British Columbia, that was based on the maximum diameter of the largest lichen found at 26 control sites from a low elevation graveyard (160 m a.s.l.) and four tree-ring dated moraines found at 1300 m a.s.l. in Strathcona Provincial Park. This Rhizocarpon curve extends to 290-yr-old surfaces and suggests that lichen growth rates are dependent upon water supply and substrate (bedrock vs. boulder).
In Washington State, CitationPorter (1981) undertook a detailed lichenometric analysis at Mount Rainier (1000–2000 m a.s.l.). Control measurements were obtained from the maximum diameter of the largest lichen discovered on historical rock structures as old as 120 yr. His investigations suggested there was a general reduction of lichen growth rate with time, notably following a 20-yr period of great growth after surface stabilization. Moreover, he provided evidence suggesting reduced growth rates at high-elevation sites were dependent upon whether the substrate was andesitic or granodioritic. The control measurements from this study and the ones provided for Mount Hood (CitationLillquist, 1988) and Mount Baker (CitationO'Neal and Schoenenberger, 2003) were combined into a Rhizocarpon curve applicable to the Cascade Mountains of Washington and northern Oregon (CitationO'Neal and Schoenenberger, 2003). The lichen growth-surface exposure relationship was developed for elevations between 1195 to 1825 m on up to 145-yr-old surfaces. Contrary to CitationPorter (1981), this research suggests that substrate influences lichen growth insignificantly.
In general, most of the published lichen growth curves for the Pacific Northwest span a relatively short period of time, resulting in thallus growth rates that suggest a logarithmic or exponential relationship associated to the great growth period for the first 75 to 100 yr. Although an extrapolated linear relationship based on great growth rates is characteristically used to date older surfaces, CitationInnes (1985) and CitationBradwell (2001) noted that the inherent assumptions possibly lead to dating errors. CitationInnes (1985) suggests that a linear relationship may be safely applicable up to ca. 150 mm thallus diameter. The oldest lichen growth curves published in Pacific North America remain those by CitationDenton and Karlén (1973) and by CitationSolomina and Calkin (2003), which show linear rates of growth persisting for the past 3000 to 4000 yr. After the linear growth period, lichens tend to experience senescence, mortality or reduced rates of radial expansion. This behavior results in thallus growth rates that suggest a logarithmic, exponential, or composite relationship (CitationInnes, 1985; CitationMcCarthy and Smith, 1995; CitationBradwell, 2001; CitationSolomina and Calkin, 2003).
Lichen growth in the Bella Coola–Mount Waddington area is intermediate between growth rates observed in southern (Porter, O'Neal/Schoenenberger, and Lewis) and northern (Brooks Range) curves. The BCW growth curve appears to be similar to the first 60 yr of the CitationPorter (1981), CitationO'Neal and Schoenenberger (2003), and CitationLewis (2001) curves, but after that point, declining growth rates suggest a closer relationship to the growth curves developed by CitationSolomina and Calkin (2003), CitationDenton and Karlén (1973), and CitationWiles et al. (2002) in southern Alaska and Seward Peninsula (). The ecological reasons for these differential age-size relationships are difficult to assess, as there are few details available on specific environments at the various sites (). Although the role of lithology on lichen growth was documented by CitationPorter (1981), it does not offer a complete explanation for the apparent spatial variation in R. geographicum growth trends emerging within the Pacific Northwest and Alaska, a conclusion supported by CitationO'Neal and Schoenenberger (2003) in the northern Cascades. Faster rates of radial expansion at the more southerly sites suggest that environmental conditions in these regions favor lichen growth, whereas more extreme conditions in Alaska and in the Mount Waddington area, induced by latitude and possibly elevation, result in declining rates of growth with age.
Many studies have demonstrated the impact of air temperature, moisture availability, altitude, and substrate on lichen growth (e.g., CitationInnes, 1985; CitationBenedict, 1990). Nevertheless, it is not unusual to encounter lichen growth curves applied to areas covering 100s of km2, thereby implying that relatively homogenous growing conditions exist throughout a region (CitationRodbell, 1992; CitationMcCarthy and Smith, 1995; CitationCalkin et al., 1998; CitationBradwell, 2001; CitationO'Neal and Schoenenberger, 2003; CitationSolomina and Calkin, 2003). The BCW curve does suggest that it is possible to extend to at least 100 km the applicability of a lichenometric dating curve for surfaces younger than 165 yr, without introducing major dating constraints. In the first 100 yr of calibrated lichen growth, uniform growth rates are apparent despite variations in elevation and environmental conditions (CitationSmith and Desloges, 2000; CitationLewis, 2001, Citation2004; CitationPorter, 1981) (, and ).
Investigations at Tiedemann Glacier and other glaciers in the Mount Waddington area (CitationLarocque and Smith, 2003) suggest that the BCW lichen growth curve provides comparative relative ages for surfaces independently dated using 14C and tree-rings in this region. Nevertheless, its site-specific applicability is limited in some settings. For instance, at Tiedemann Glacier the assigned age on the same continuous moraine crest varied by 200 yr or more (, ). While these findings are most likely due to factors such as variable rates of substrate stabilization (e.g., CitationGellatly, 1982; CitationInnes, 1985; CitationRodbell, 1992) and lichen population dynamics (CitationInnes, 1985; CitationMcCarthy, 1997, Citation1999), they do emphasize the importance of replication in lichen measurements. Further difficulties arise in assigning appropriate errors to lichen growth curves. While appropriate control points can be identified to permit the calculation of the 95% confidence intervals, this is rarely possible in remote settings where there is limited dating control. Although errors associated with confidence intervals do not include the entire range of dating errors encountered in lichenometric studies, it provides a useful statistical estimate. Reducing the confidence intervals by, for example, increasing the number of control measurement sites or the accuracy of independently dated surfaces is an important concern because it gives the opportunity to associate an acceptable error with the lichen date.
Conclusion
This paper confirmed and strengthened the dating applicability of the Bella Coola–Monarch Icefield lichen growth curve developed by CitationSmith and Desloges (2000) to lichenometric studies in the Mount Waddington area. A significant contribution of the BCW curve is the extension back to 680-yr-old surfaces, which will permit the relative age dating of pre-Little Ice Age landforms. The study also stresses the importance of incorporating a statistically adequate number of radial measurements and statistical normality testing to eliminate outliers that are inherent to lichen populations. High replication is necessary as substrate stabilization, lichen population distribution, and local disturbance greatly affect lichen growth, leading to dating errors of several decades. These conditions satisfied, lichen dating likely leads to better estimate of glacial activity then tree-ring analysis, as lichens are abundant and establish shortly after surface exposure.
FIGURE 1. Location of control sites in the Mount Waddington area. In total, 11 control lichen measurement sites were sampled from five different glaciers
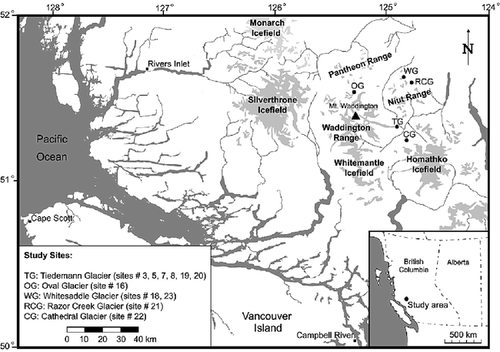
FIGURE 2. Distribution of lichen thalli on control sites. Most of the populations are normally distributed (Shapiro-Wilk normality test), except sites 8 and 19. At these sites, outliers (indicated by an asterisk) were removed from the distribution
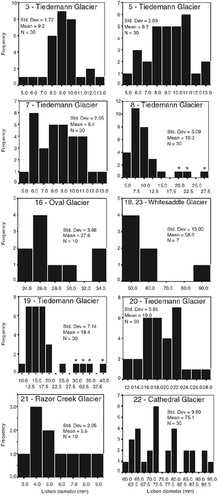
FIGURE 3. Lichen growth curve developed for the Mount Waddington area. A logarithmic relationship explains best the first 100 yr of growth. Between 100 and 680 yr, a linear function describes the growth. The error associated with the curve corresponds to the 95% confidence interval of the logarithmic regression and the 14C calibrated 95% probability
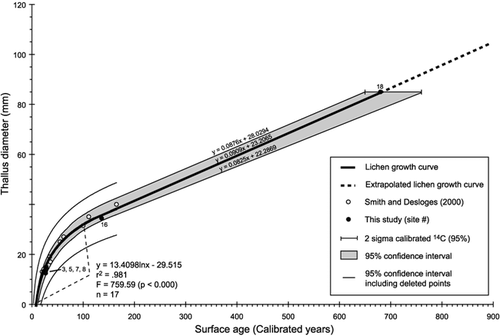
FIGURE 4. Oblique aerial photograph of late-Neoglacial forefield of the Tiedemann Glacier, July 2001
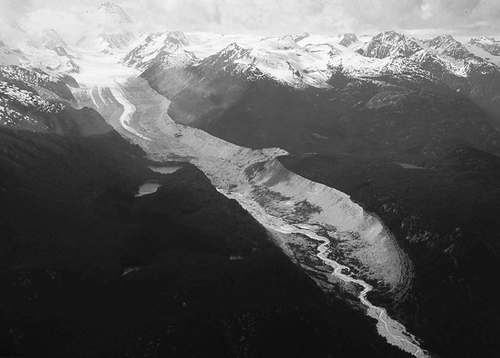
FIGURE 5. Location of lichen measurement transects at Tiedemann Glacier. Lichens were measured along eight transects, for a total of 24 sampling sites (see ). One radiocarbon date of 1270 ± 140 yr BP was obtained from basal peat outside the outermost Little Ice Age moraine (CitationFulton, 1971)
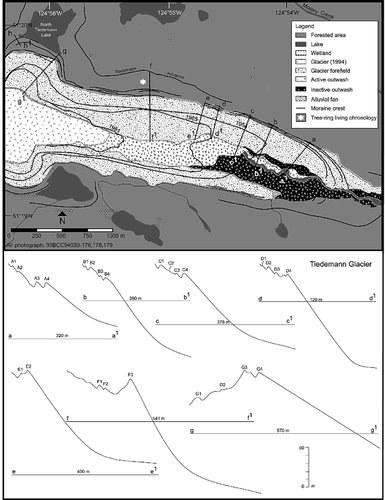
FIGURE 6. Typical lateral moraines at Tiedemann Glacier, showing sparsely distributed trees (whitebark pine, subalpine fir, and Douglas-fir) on four successive bouldery ridges
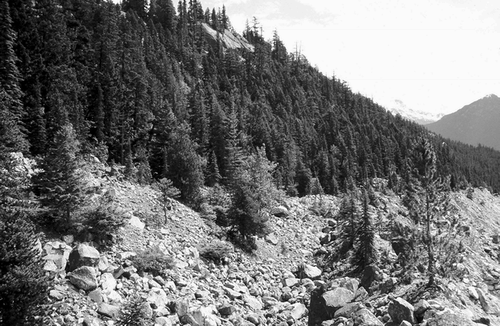
FIGURE 7. Frequency distribution of lichen dates obtained at Tiedemann Glacier. Moraine crests were numbered (capital letters) according to their position on the transects (moraine 1 being the most distal), with moraine numbers from different transects (lower letters) at a site not necessarily being synchronous. Stabilization of lateral moraine, leading to lichen colonization, occurred in six distinct periods: with minimum dates of AD 620 (1), 925 (2), 1118 (3), 1392 (4), 1575 (5), and 1621 (6)
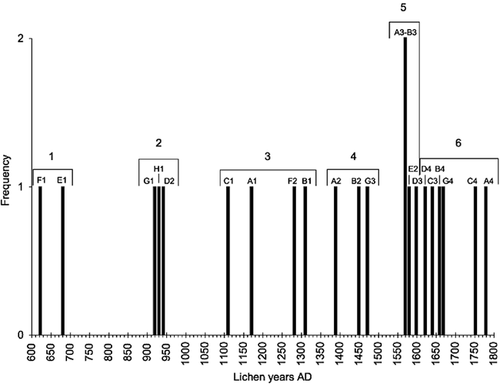
FIGURE 8. Comparative graph of lichen growth curves developed along the Pacific Coast of North America: CitationDenton and Karlén (1973), and CitationWiles et al. (2002) in the Wrangell and St. Elias Mountains of Alaska; CitationSolomina and Calkin (2003) in the Brooks Range, Seward Peninsula, Alaska Range, and Kenai Mountains; CitationLewis (2001) and Larocque and Smith (this study) in the southern B.C. Coast Mountains; CitationPorter (1981) and CitationO'Neal and Schoenenberger (2003) in the Cascade Range of Washington and Oregon
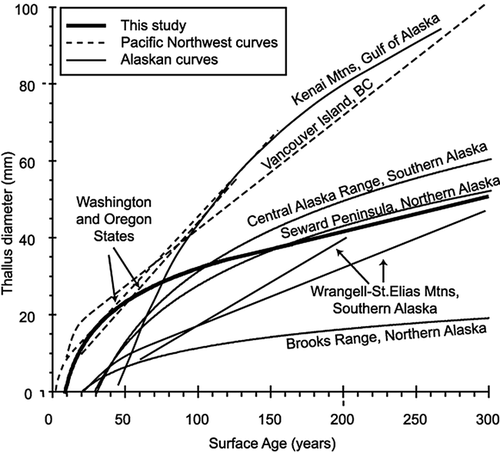
TABLE 1 Lichen species identification (n = 10) (K. A. Glew, 24 February 2002). Eight lichens were identified as Rhizocarpon geographicum (L.) DC. from a variety of lithologies (gneiss, andesite, granite, granodiorite). R. macrosporum Räsänen was found in 2 samples of andesite
TABLE 2 Control points used to develop the Bella Coola/Mount Waddington (BCW) lichen growth curve. Asterisks represent sites not included in the curve
TABLE 3 Statistics of lichen measurements on Mount Waddington control sites. Shapiro-Wilk test was applied on samples n = 30 to assess the normality of the distributions (p ≤ 0.01). Fail tests represent non-normally distributed samples and include outliers that have been removed from the samples to construct the lichen growth curve
TABLE 4 Statistics of lichen measurements at Tiedemann Glacier, and dates derived from the Bella Coola/Mount Waddington (BCW) lichen growth curve. Asterisks indicate samples where an outlier was removed from the distribution
TABLE 5 Rhizocarpon spp. Growth curves developed along the Pacific Coast of North America
Acknowledgments
We greatly appreciate the field assistance provided by Laurel George and Alexis Johnson. We would like to acknowledge the participation of Katherine A. Glew, from the University of Puget Sound, who undertook lichen identifications. We appreciate the comments made by J. B. Benedict and an anonymous reviewer to an earlier version of the manuscript. This research was funded by Natural Sciences and Engineering Research Council of Canada, Canadian Foundation for Climate and Atmospheric Research and Inter-American Institute for Global Change grants to D. Smith.
References Cited
- André, M-F. 1986. Dating slope deposits and estimating rates of rock wall retreat in northwest Spitsbergen by lichenometry. Geografiska Annaler 68A:65–75.
- Andrews, J. T. and P. J. Webber . 1969. Lichenometry to evaluate changes in glacial mass budgets: as illustrated from north-central Baffin Island, N.W.T. Arctic and Alpine Research 1:181–194.
- Barclay, D. J. , P. E. Calkin , and G. C. Wiles . 2001. Holocene history of Hubbard Glacier in Yakutat Bay and Russell Fiord, southern Alaska. Geological Society of America Bulletin 113:388–402.
- Barendsen, G. W. , E. S J. Deevy , and I. J. Gralenski . 1957. Yale natural radiocarbon measurements III. Science 126:908–919.
- Begét, J. E. 1994. Tephrochronology, lichenometry and radiocarbon dating at Gulkana Glacier, central Alaska Range, USA. The Holocene 4:307–313.
- Benedict, J. B. 1990. Experiments on lichen growth. I. Seasonal patterns and environmental controls. Arctic and Alpine Research 22:244–252.
- Beschel, R. E. 1961. Dating rock surfaces by lichen growth and its application to glaciology and physiography (lichenometry). In Raasch, G. O. (ed.), Geology of the Arctic 2. Toronto: University of Toronto Press, 1044–1062.
- Beschel, R. E. 1973. Lichens as a measure of the age of recent moraines. Arctic and Alpine Research 5:303–309.
- Bijerk, A. 1980. Lichenometric dating of Neoglacial deposits in the McKinley Park area, Alaska. Unpublished report submitted to the National Park Service and National Geographic Society. 55 pp.
- Bradley, R. S. 1985. Quaternary Paleoclimatology. Boston: Allen & Unwin Inc. 472 pp.
- Bradwell, T. 2001. A new lichenometric dating curve for southeast Iceland. Geografiska Annaler 83A:91–101.
- Broadbent, N. D. and K. I. Bergqvist . 1986. Lichenometric chronology and archaeological features on raised beaches: preliminary results from the Swedish north Bothnian coastal region. Arctic and Alpine Research 18:297–306.
- Bull, W. B. and M. T. Brandon . 1998. Lichen dating of earthquake-generated regional rockfall events, southern Alps, New Zealand. Geological Society of America Bulletin 110:60–84.
- Bull, W. B. , J. King , F. Kong , T. Moutoux , and W. M. Phillips . 1994. Lichen dating of coseismic landslide hazard in alpine mountains. Geomorphology 10:253–264.
- Bull, W. B. , P. Schlyter , and S. Brogaard . 1995. Lichenometric analysis of the Kärkerieppe slush-avalanche fan, Kärkevagge, Sweden. Geografiska Annaler 77A:231–240.
- Calkin, P. E. and J. M. Ellis . 1980. A lichenometric dating curve and its application to Holocene glacier studies in the central Brooks Range, Alaska. Arctic and Alpine Research 12:245–264.
- Calkin, P. E. and J. M. Ellis . 1984. Development and application of a lichenometric dating curve, Brooks Range, Alaska. In Mahaney, W. C. (ed.), Quaternary Dating Methods. Amsterdam: Elsevier, 227–246.
- Calkin, P. E. , D. S. Kaufman , B. J. Przybyl , W. B. Whitford , and B. J. Peck . 1998. Glacier regimes, periglacial landforms, and Holocene climate change in the Kigluaik Mountains, Seward Peninsula, Alaska, U.S.A. Arctic and Alpine Research 30:154–165.
- Calkin, P. E. , G. C. Wiles , and D. J. Barclay . 2001. Holocene coastal glaciation of Alaska. Quaternary Science Reviews 20:449–461.
- Clague, J. J. and R. W. Mathewes . 1996. Neoglaciation, glacier-dammed lakes, and vegetation change in northwestern British Columbia. Arctic and Alpine Research 28:10–24.
- Clague, J. J. and V. N. Rampton . 1982. Neoglacial Lake Alsek. Canadian Journal of Earth Sciences 19:94–117.
- Denton, G. H. and W. Karlén . 1973. Lichenometry: its application to Holocene moraine studies in southern Alaska and Swedish Lapland. Arctic and Alpine Research 5:347–372.
- Denton, G. H. and W. Karlén . 1977. Holocene glacial and tree-line variations in the White River Valley and Skolai Pass, Alaska and Yukon Territory. Quaternary Research 7:63–111.
- Desloges, J. R. and J. M. Ryder . 1990. Neoglacial history of the Coast Mountains near Bella Coola, British Columbia. Canadian Journal of Earth Sciences 27:281–290.
- Fulton, R. J. 1971. Radiocarbon Geochronology of Southern British Columbia. Geological Survey of Canada, Paper 71-37.
- Gellatly, A. F. 1982. Lichenometry as a relative-age dating method in Mount Cook National Park, New Zealand. New Zealand Journal of Botany 20:343–353.
- Harrison, S. and V. Winchester . 2000. Nineteenth- and twentieth-century glacier fluctuations and climatic implications in the Arco and Colonia valleys, Hielo Patagonica Norte, Chile. Arctic, Antarctic, and Alpine Research 32:55–63.
- Heikkinen, O. 1984. Dendrochronological evidence of variations of Coleman Glacier, Mount Baker, Washington, U.S.A. Arctic and Alpine Research 16:53–64.
- Heusser, C. J. 1957. Variations of Blue, Hoh, and White Glaciers during recent centuries. Arctic 10:139–150.
- Hickson, C. J. 1994. Character of volcanism, volcanic hazards, and risk, northern end of the Cascade magmatic arc, British Columbia and Washington State. In Monger, J. W. H. (ed.), Geology and Geological Hazards of the Vancouver Region, Southwestern British Columbia. Geological Survey of Canada, 231–250.
- Innes, J. L. 1985. Lichenometry. Progress in Physical Geography 9:187–254.
- Larocque, S. J. and D. J. Smith . 2003. Little Ice Age glacial activity in the Mt. Waddington area, British Columbia Coast Mountains, Canada. Canadian Journal of Earth Sciences 40:1413–1436.
- Lewis, D. H. 2001. Little Ice Age investigations in Strathcona Provincial Park, Vancouver Island, B.C. M.Sc. thesis, University of Victoria, Victoria. 147 pp.
- Lewis, D. H. and D. J. Smith . 2004. Little Ice Age glacial activity in Strathcona Provincial Park, Vancouver Island, British Columbia, Canada. Canadian Journal of Earth Sciences 41:285–297.
- Lillquist, K. D. 1988. Holocene fluctuations of the Coe Glacier, Mount Hood, Oregon. M.Sc. thesis, Portland State University.
- Lowdon, J. A. and W. J. Blake . 1975. Radiocarbon dates XV. Geological Survey of Canada. Paper 75–7: 32 pp.
- Luckman, B. H. 1977. Lichenometric dating of Holocene moraines at Mount Edith Cavell, Jasper, Alberta. Canadian Journal of Earth Sciences 14:1809–1822.
- McCarroll, D. 1994. A new approach to lichenometry: dating single-age and diachronous surfaces. The Holocene 4:383–398.
- McCarthy, D. P. 1997. Habitat selection and ecology of Xanthoria elegans (Link) Th. Fr. in glacier forefields: implications for lichenometry. Journal of Biogeography 24:363–373.
- McCarthy, D. P. 1999. A biological basis for lichenometry?. Journal of Biogeography 26:379–386.
- McCarthy, D. P. and D. J. Smith . 1995. Growth curves for calcium-tolerant lichens in the Canadian Rocky Mountains. Arctic and Alpine Research 27:290–297.
- Meteorological Service of Canada, 2002. Canadian climate normals 1961–1990. URL:http://www.msc.ec.gc.ca/climate/climate_normals_1990/index_e.cfm .
- Monger, J. W H. and J. M. Journeay . 1994. Basement geology and tectonic evolution of the Vancouver region. In Monger, J.W.H. (ed.), Geology and Geological Hazards of the Vancouver Region, Southwestern British Columbia. Geological Survey of Canada, 3–25.
- O'Neal, M. A. and K. R. Schoenenberger . 2003. A Rhizocarpon geographicum growth curve for the Cascade Range of Washington and northern Oregon, USA. Quaternary Research 60:233–241.
- Osborn, G. and J. Taylor . 1975. Lichenometry on calcareous substrate in the Canadian Rockies. Quaternary Research 5:111–120.
- Pearson, E. S. , R. B. D'Agostino , and K. O. Bowman . 1977. Tests for departure from normality: comparison of powers. Biometrika 64:231–246.
- Péwé, T. L. and R. D. Reger . 1983. Delta River area, Alaska Range. In Péwé, T. L. and Reger, R. D. (eds.), Richardson and Glen Highways, Alaska: guidebook to permafrost and Quaternary geology. Fairbanks, 47–136.
- Plafker, G. and D. J. Miller . 1957. Glacial features and surficial deposits of the Malaspina district, Alaska. U.S. Geological Survey open file report. 13 pp.
- Poelt, J. 1988. Rhizocarpon Ram. em. Th. Fr. subgen. Rhizocarpon in Europe. Arctic and Alpine Research 20:292–298.
- Porter, S. C. 1981. Lichenometry studies in the Cascade Range of Washington: establishment of Rhizocarpon geographicum growth curves at Mount Rainier. Arctic and Alpine Research 13:11–23.
- Purvis, O. W. , B. J. Coppins , D. L. Hawksworth , P. W. James , and D. M. Moore . (eds.),. 1992. The Lichen Flora of Great Britain and Ireland. London: The British Lichen Society and The Natural History Museum. 710 pp.
- Reynolds, N. D. 2001. Dating the Bonneville landslide with lichenometry. Washington Geology 29:11–16.
- Rodbell, D. T. 1992. Lichenometric and radiocarbon dating of Holocene glaciation, Cordillera Blanca, Peru. The Holocene 2:19–29.
- Runemark, H. 1956. Studies in the genus Rhizocarpon . I. Taxonomy of the yellow species in Europe. Opera Botanica 2:1–152.
- Ryder, J. M. and B. Thomson . 1986. Neoglaciation in the southern Coast Mountains of British Columbia: chronology prior to the late Neoglacial maximum. Canadian Journal of Earth Sciences 23:273–287.
- Sigafoos, R. S. and E. L. Hendricks . 1972. Recent activity of glaciers of Mount Rainier, Washington. U.S. Geological Survey Professional Paper 387-B: B1–B24.
- Smirnova, T. Y. and A. A. Nikonov . 1990. A revised lichenometric method and its application dating great past earthquakes. Arctic and Alpine Research 22:375–388.
- Smith, D. J. and J. R. Desloges . 2000. Little Ice Age history of Tzeetsaytsul Glacier, Tweedsmuir Provincial Park, British Columbia. Géographie physique et Quaternaire 54:135–141.
- Smith, D. J. and C. P. Laroque . 1996. Dendroglaciological dating of a Little Ice Age glacial advance at Moving Glacier, Vancouver Island, British Columbia. Géographie physique et Quaternaire 50:47–55.
- Smith, D. J. , D. P. McCarthy , and M. E. Colenutt . 1995. Little Ice Age glacial activity in Peter Lougheed and Elk Lakes provincial parks, Canadian Rocky Mountains. Canadian Journal of Earth Sciences 32:579–589.
- Solomina, O. and P. E. Calkin . 2003. Lichenometry as applied to moraines in Alaska, U.S.A., and Kamchatka, Russia. Arctic, Antarctic, and Alpine Research 35:129–143.
- Stokes, M. A. and T. L. Smiley . 1968. An Introduction to Tree-Ring Dating. Chicago: The University of Chicago Press. 73 pp.
- Thomson, J. W. 1967. Notes on Rhizocarpon in the Arctic. Nova Hedwigia 14:421–481.
- Thomson, J. W. 1997. American Arctic lichens. II. The Microlichens. Madison: University of Wisconsin Press.
- Wiles, G. C. 1992. Holocene glacial fluctuations in the southern Kenai Mountains, Alaska. Ph.D. dissertation, State University of New York at Buffalo. 333 pp.
- Wiles, G. C. , D. J. Barclay , and P. E. Calkin . 1999. Tree-ring-dated ‘Little Ice Age’ histories of maritime glaciers from western Prince William Sound, Alaska. The Holocene 9:163–173.
- Wiles, G. C. and P. E. Calkin . 1991. Dendrogeomorphic refinement of a 14C chronology from McCarty fjord, Alaska. GSA Northeastern Section, 26th annual meeting, Southeastern Section, 40th annual meeting. Geological Society of America, 150.
- Wiles, G. C. and P. E. Calkin . 1993. Neoglacial fluctuations and sedimentation of and iceberg-calving glacier resolved with tree rings (Kenai Fjords National Park, Alaska). Quaternary International 18:35–42.
- Wiles, G. C. and P. E. Calkin . 1994. Late Holocene, high-resolution glacial chronologies and climate, Kenai Mountains, Alaska. Geological Society of America Bulletin 106:281–303.
- Wiles, G. C. , P. E. Calkin , and A. Post . 1995. Glacier fluctuations in the Kenai Fjords, Alaska, U.S.A.: an evaluation of controls on iceberg-calving glaciers. Arctic and Alpine Research 27:234–245.
- Wiles, G. C. , G. C. Jacoby , N. K. Davi , and R. P. McAllister . 2002. Late Holocene glacier fluctuations in the Wrangell Mountains, Alaska. Geological Society of America Bulletin 114:896–908.