Abstract
Modern chironomid assemblages consisting of 68 taxa were extracted from the sediments of 39 lakes in southwest Yukon and northern British Columbia, Canada. Important factors accounting for the distribution of the chironomid taxa include sediment organic matter (LOI; weight-loss-on-ignition), total phosphorus, bottom water temperature, and lake alkalinity. The results show the importance of organic matter, which is associated with water depth and lake productivity, in affecting chironomid community composition. Tanytarsus sp. C, Tanytarsus pallidicornis type, Allo/Mesopsectrocladius, Polypedilum, and Procladius were associated with lakes having high LOI values and shallow lake depths, while Paratanytarsus, Sergentia, and Corynocera oliveri type were more prevalent in deeper lakes with more minerogenic sediments. Surprisingly, Chironomus was most abundant in deeper lakes with low LOI values, which might be partially explained by low oxygen levels. Contrary to some findings, Tanytarsus lugens group was common in shallow, productive lakes in this region.
Introduction
The distribution and abundance of aquatic organisms are influenced by numerous environmental factors. Aquatic organisms are influenced by the physical and chemical environment as well as by other organisms, and these in turn are affected by the conditions in the watershed. These factors vary in time and in space (CitationPourriot and Meybeck, 1995). Identifying the relative importance of various abiotic and biotic factors influencing a particular community in a lake system remains a challenge. Biogeographic and paleolimnological analyses contribute to this research effort by documenting population distributions and community patterns and relating these to environmental variability.
Chironomids are major components of the benthic fauna of lakes, especially in northern latitudes (CitationPourriot and Meybeck, 1995; CitationBrinkhurst, 2002). As with any organism, the distribution and abundance of chironomids are influenced by environmental factors. Several aspects of the environment that influence chironomid communities include lake productivity, oxygen content of the hypolimnion and sediments, water properties including salinity, and water temperature (CitationWalker, 1987). Because of the covariance of these parameters, determining the limiting factors is not always straightforward.
Chironomid taxa are used in paleoenvironmental studies because their remains are preserved in lake sediments. Many studies have reported chironomid taxa from lake sediment cores (CitationPorinchu and MacDonald, 2003). Interpreting paleoenvironmental records relies on an understanding of the ecology of the organism in question. In the absence of extensive information about the biology of many chironomid taxa, particularly of North American species (CitationWalker, 1987), geographical studies can provide data about species ranges and the conditions within the lakes that are correlated with community composition. These studies rely on the analysis of chironomid assemblages and water characteristics from a series of lakes and use various statistical methods to determine environmental factors most highly correlated with the abundance of the species in the community.
Several studies have documented the distribution of chironomids in relation to environmental variables in northern regions. CitationWalker and MacDonald (1995) and CitationPorinchu and Cwynar (2000) showed that chironomid communities differed across treeline in Canada and Siberia. CitationWalker et al. (1991) and CitationWalker et al. (2003) reported chironomid assemblages along transects in Labrador and in the Yukon and Northwest Territories, respectively. Lake depth, water chemistry, and surface-water temperature all influenced chironomid community composition. In Labrador lakes, sediment organic matter was not found to be a critical factor in chironomid community composition, although this may have been expected based on previous studies (CitationWalker et al., 1991). July air temperature was also identified as a key factor explaining chironomid distributions in lakes of northern Sweden (CitationLarocque et al., 2001) and western Norway (CitationBrooks and Birks, 2000).
In this study, we analyze the relationship between water chemistry, physical variables, and chironomid assemblages from the surface sediment of a series of lakes in southwest Yukon Territory and northern British Columbia. The purpose of this study is to identify the factors influencing chironomid distributions and abundance in this region. We previously analyzed the diatoms in these lakes (CitationWilson and Gajewski, 2002). Lake size (depth and area), in addition to water chemistry, was an important factor affecting diatom distributions. We here report on another group of benthic organisms to gain a better understanding of the dynamics of these lake systems.
Study Area
Southwest Yukon is in the boreal biome, which is a dominant landscape of the Northern Hemisphere. The majority of lakes analyzed in this study are located along the Alaska Highway between Whitehorse and Beaver Creek, with a few located along the Haines Road in northern British Columbia (). Many sites are concentrated along the Shakwak Trench, which contains Kluane Lake. In addition to some mining, human activity in the region is concentrated in several small towns and along the major roads. There has been little human impact on most of these lakes (personal observation).
The dominant landforms of the southwest Yukon and northern British Columbia are a series of northwest-southeast–trending mountain chains and valleys. The geology is complex and varied but consists largely of metamorphic bedrock and large intrusive bodies composed of granodiorite and quartz diorite (CitationGabrielse et al., 1977; CitationOswald and Senyk, 1977). Much of the area is underlain with extensive glacial and surficial deposits (CitationGabrielse et al., 1977) and is located within the discontinuous scattered permafrost zone. The region lies within the rainshadow of the St. Elias Mountains, resulting in precipitation less than 300 mm per year (CitationWahl et al., 1987). Mean July air temperature in the study region is 12°C and mean January temperature ranges from −21 to −30°C (CitationOswald and Senyk, 1977).
The common tree species in the study area include white spruce (Picea glauca), balsam poplar (Populus balsamifera), and trembling aspen (Populus tremuloides) (CitationJohnson and Raup, 1964). Toward the eastern and southern portion of the study area the forests become more diverse, including paper birch (Betula papyrifera) and pine (Pinus spp.). There is a noticeable absence of black spruce (Picea mariana) in the study area and a lack of alder (Alnus spp.) in the region surrounding Kluane Lake. A significant aspect of the vegetation is the presence of natural grasslands, found in association with open forest stands, mainly on warm and dry, south-facing slopes. Many of the sites clustered at the south end of Kluane Lake, in addition to a few others, had catchments with substantial grassland vegetation. Although most of our sites are within the boreal forest, those in British Columbia are located at treeline, with the southernmost sites located in lichen heath tundra ().
Methods
FIELD METHODS AND WATER CHEMISTRY ANALYSES
Lake water and sediment samples were collected from 34 lakes between 11 and 22 July 2000. An additional 5 lakes were sampled in July or early August in 1996, 1997, or 1998 (Ash, Trout, Patrick, Hungry, and K819). Chironomid analyses were performed on sediment samples that were collected from near the center of the lake using a gravity corer (CitationGlew, 1991), with the exception of the 1998 samples, which were collected with an Ekman Dredge (Hungry and K819) or clear plastic tube fitted with a piston. The upper 1 to 2 cm were extruded at 0.5 cm or 1 cm intervals using a vertical extruder (CitationGlew, 1988).
Conductivity (Cond) and temperature (T) profiles were recorded using a YSI model 33 SCT meter, and oxygen profiles were taken with a YSI model 51B oxygen meter, measured at 0.5 m intervals. Surface-water pH was averaged from 2 or 3 different handheld pH meters (Oakton pHTestr™ 2 and 3) that were calibrated daily to standard buffer solutions. Water transparency (Secchi) was measured using a standard 22-cm-diameter Secchi disc. Water collection, filtering, and sample storage protocols followed CitationEnvironment Canada (1983). Details of the filtering and preparation of water samples for chemistry analyses are given in CitationWilson and Gajewski (2002). Loss-on-ignition (organic matter content) and carbonate content of the sediment were determined by drying 2-cm3 samples overnight at 95°C and then igniting in a muffle furnace at 500°C for 3 h and 925°C for 4 h, respectively (CitationBengtsson and Enell, 1986).
CHIRONOMID ANALYSES
Samples were processed by deflocculating the sediment in hot 10% KOH for 30 min and sieving on a 90 μm mesh. Head capsules were hand sorted with forceps, from a Bogorov counting tray, under a 40× magnification using a stereo microscope, and placed on cover slips to dry. Cover slips were later mounted on slides using Entellan®. Head capsules were extracted from the uppermost 1 cm first, followed by the 1 to 2 cm sample if necessary to obtain a minimum of 50 head capsules (CitationHeiri and Lotter, 2001; CitationQuinlan and Smol, 2001).
Taxonomic identifications were performed at 100× to 400× magnification under bright-field illumination and were based on CitationWiederholm (1983), CitationOliver and Roussel (1983), and CitationWalker (1988, Citation2000). Taxonomy within the subtribe Tanytarsina followed CitationWalker (2000). A number of specimens were encountered that bore a likeness to both the Tanytarsus lugens group and Corynocera oliveri type (both mentum and mandible features) and could not be placed reliably in either category. These were put in a separate grouping designated T. lugens/C. oliveri type. Corynoneura was separated from Corynoneura/Thienemanniella when sculpturing was visible on the head capsule surface (CitationWiederholm 1983), while Cricotopus and Orthocladius were largely indistinguishable and therefore grouped in many cases. The caption also refers to specific figures and their sources for the identification of some taxa (e.g., Parakiefferiella cf. sp. B, Parakiefferiella Fig. 367, and various Psectrocladius taxa).
DATA ANALYSIS
A few of the sites sampled in 1996 to 1998 were without dissolved organic carbon, transparency (Secchi), oxygen, and chlorophyll a (Chla) data. Mean values were substituted in each case (CitationTabachnick and Fidell, 1989) (). Chemical variables that had values below the detection limit in more than half of the study lakes were eliminated from all statistical analyses. This was the case for many of the metals, with the exception of Al, Fe, Mn, and Mo (). In other cases where values were below the limit of detection, the detection limit value was substituted. Environmental variables were tested for non-normal distributions using CALIBRATE version 0.70 (CitationJuggins and ter Braak, 1997). All variables were log-transformed with the exception of surface and bottom-water temperature, pH, conductivity, alkalinity, dissolved organic carbon, and silicon, which were square-root-transformed. Total phosphorus and total filtered phosphorus remained highly skewed after transformation.
Ordinations were performed using CANOCO version 4.0 (Citationter Braak and Šmilauer, 1998). Both canonical correspondence analysis (CCA) and redundancy analysis (RDA) were used to assess the relationship between the chironomid assemblages and the measured physical and environmental variables because the length of the gradient of the first axis in a detrended correspondence analysis (DCA) was relatively small (2.2 standard deviation units) (Citationter Braak and Šmilauer, 1998). Taxon abundances were square-root-transformed for all ordinations, and rare species downweighted in DCA and CCA. RDA was performed with species centering and without standardization. A principal components analysis (PCA) of the environmental data, a DCA of the taxon data, and leverage diagnostics in CANOCO were used to check for outliers.
To reduce the presence of correlated environmental variables, we performed a series of constrained CCAs and RDAs where each environmental variable was selected as the sole variable, and the significance of the first axis tested using a Monte Carlo Permutation test. Only significant (P < 0.05, 500 permutations) variables were retained. Following this, variables were checked for high variance inflation factors (a VIF > 20 indicates that a variable is almost perfectly correlated with another [Citationter Braak and Šmilauer, 1998]) and eliminated sequentially, beginning with the variable having the highest VIF. This proceeded until all VIFs were below five. Forward selection was then used to identify those variables that explained most of the variance in the data. Variance partitioning (CitationBorcard et al., 1992) was used to assess the strength and independence of those variables that explained the majority of the variance in the data, as determined by the series of constrained RDAs, as some of these variables were highly correlated with each other.
Results and Discussion
WATER CHEMISTRY
A summary of lake chemistry and physical properties is given in . More detailed descriptions are provided in CitationWilson and Gajewski (2002). In general the lakes are mostly shallow (mean depth = 5.2 m; maximum depth = 15 m) with nearly half (43%) having a maximum depth of 2 m or less (). Many of the lakes are closed basin, while a few have significant stream inflow (Hungry, Otter Falls, and Little Louise). Surface-water temperatures measured at the time of sampling ranged from 11°C to 20.5°C, although temperatures were rarely above 17°C. The lowest surface-water temperatures were recorded in the northern British Columbia lakes that are located in subalpine tundra (e.g., West Twin, Kusawak, and Three Guardsmen). Temperature profiles were uniform in all of the shallow (<5 m deep) lakes, while lakes of intermediate depth (5–10 m) had either isothermal or weakly stratified water columns by mid-July. Lakes that were >10 m deep had well-developed thermal stratification with epilimnion thickness of 6 to 7 m (not shown).
Alkalinity of the lakes was high compared to many boreal and subarctic regions (mean Alk = 147 mg L−1; median pH = 8.7), and only 2 lakes had a specific conductance less than 100 μS cm−1. This may be due to the presence of abundant glacial till in this part of the Yukon and to the evaporative enrichment associated with closed basin lakes, particularly those near Kluane Lake. Overall, the variability in conductivities among the lakes appears to be related to regional differences in geology, as well as differences in vegetation and the amount of stream inflow to lakes.
Most of the lakes are in the oligotrophic (<10 μg L−1) (52%) and meso-eutrophic (10–30 μg L−1) (40%) ranges, based on epilimnetic total phosphorus (TP) values (). Dissolved organic carbon (DOC) is variable as well (0.8–43 mg L−1) and appears to be related to the relative abundance of grassland or tundra versus forest in the immediate vicinity of the lake, and possibly catchment area as well (CitationWilson and Gajewski, 2002). Sediment organic content (as determined by LOI) ranged from 9% to 80% with a mean of 32% (). LOI was negatively correlated with depth and highly correlated with bottom oxygen (not shown).
CHIRONOMIDS
A total of 5356 head capsules were enumerated from all lakes (mean per sample = 137; minimum = 58; maximum = 344), including those lakes that were later eliminated from the statistical analysis as outliers. Sixty-eight chironomid taxa were identified from the lakes, but only those present at ≥2% abundance in at least 2 lakes were included in the statistical analyses (CitationQuinlan and Smol, 2001), leaving 38 taxa in total. A few lakes had chironomid populations overwhelmingly dominated by a single genus, such as Donjek Kettle (Chironomus > 80%) and Teapot Lake (Glyptotendipes > 70%) (). Psectrocladius D type was abundant only in Kusawak Lake (40% relative abundance), a shallow, low alkalinity, ultra-oligotrophic lake. A number of rheophilic genera including Rheocricotopus, Brillia, Euorthocladius, and Thienemanniella? were present only in Little Louise and Otter Falls lakes, at low relative abundance (each < 1.5%; not shown). These 2 lakes also had the only occurrences of Simuliidae (black fly) larval head capsules in their surface sediments, indicating stream or river influence (CitationCurrie and Walker, 1992).
The dominance of Glyptotendipes in Teapot Lake might be explained by a number of factors. First, Teapot Lake is relatively deep (12 m) with a very small surface area (6500 m2), where metalimnetic oxygen maxima of 150% to 200% and a very shallow (2–3 m), mostly anoxic (O2 < 2 mg L−1) hypolimnion have been observed over the past 2 summers (unpublished data). Total phosphorus is much higher in the metalimnion and hypolimnion (summer averages were approximately 23 and 100 μg L−1, respectively) compared to the epilimnion (9 μg L−1). The small volume of oxygenated hypolimnion that the above conditions allow would explain the near absence of profundal chironomids in the surface sediments compared to lakes of similar depth, and the dominance of littoral taxa. Chironomids living in the littoral zone of the lake could easily be transported down slope (e.g., CitationSchmäh, 1993; CitationWalker et al., 1993), especially given the small diameter and steep sides. Second, Glyptotendipes is known to thrive in mesotrophic-eutrophic and highly conductive environments (CitationSaether, 1979; CitationWalker, 1987; CitationWalker et al., 1995; CitationLotter et al., 1998), which describes well the littoral environment of Teapot Lake (specific conductance = 616 μS cm−1). Furthermore, the abundance of macrophyte remains in the anoxic, deep-water sediments of Teapot Lake suggests that these are plentiful in the littoral zone, providing a substrate for the many species of Glyptotendipes that mine the leaves and stems of aquatic plants (CitationWeiderholm, 1983). Based on CitationWeiderholm (1983), the head capsules found in the surface sediments probably belong to species group A, which includes G. barbipes, G. pallens, and G. paripes. CitationLods-Crozet and Lachavanne (1994) found the G. paripes/G. pallens group exclusively in the submerged macrophyte zone of Lake Geneva, and G. barbipes is characteristic of high conductivity lakes (CitationWiederholm, 1983). Anoxic conditions in much of the water column of Donjek Kettle might explain the dominance of Chironomus in this lake, as members of the genus are known to thrive in low-oxygen environments (e.g., CitationHofmann, 1988; CitationQuinlan et al., 1998).
RELATIONSHIP BETWEEN CHIRONOMIDS AND LIMNOLOGICAL VARIABLES
The Otter Falls sample was eliminated from analysis because of significant river/stream inflow from a much larger, deeper lake; Otter Falls is actually a wide area of a river. Three Guardsmen Lake was eliminated because its sample scores were outside the 95% confidence limits in both the PCA and DCA. The variables Fe and Chla were eliminated from the final ordination analyses because of extreme influence (6.9× and 5.9×, respectively) in some lakes, as revealed with leverage diagnostics (Citationter Braak and Šmilauer, 1998). Because we had relatively few sites, we thought it preferable to eliminate these variables rather than the lakes from the ordination. Secchi depth was also eliminated from the final ordination because these measurements were often constrained by shallow lake depths, yielding inaccurate measures of transparency ().
The series of constrained CCAs and RDAs and forward selection with both ordination methods produced similar results (not shown). However, because the percentage of the total variance in the chironomid data explained by the first 2 axes and the explained variance of individual variables when constrained to axis 1 in RDA was slightly higher than with CCA (21% versus 20% using the 14 variables in ), we present only the results for the RDA.
Of the 14 variables identified as statistically significant in the constrained RDAs, eight remained after elimination of those with the highest variance inflation factors—bottom water temperature (Tb), bottom oxygen (DOb), depth, alkalinity (Alk), dissolved organic carbon (DOC), total phosphorus (TP), Mn, and loss-on-ignition (LOI) (). The forward selection procedure identified LOI, TP, bottom water temperature, and alkalinity as the variables that could explain most of the variation in the chironomid data.
The first 2 axes of the RDA, consisting of the above 8 variables, were identified as statistically significant using Monte Carlo Permutation testing (P < 0.05, 500 permutations). These 2 axes explain 19.5% of the variation in the chironomid data (eigenvalues of 0.12 and 0.08, respectively). Loss-on-ignition, depth, and bottom water temperature were significantly correlated with axis 1, based on the t-values of the canonical coefficients (significant at P < 0.05), while TP and Mn were highly correlated with axis 2 (). LOI explained the majority of the variance in the data (9.8%), followed by bottom water temperature (7.7%), Secchi depth (7.5%), which was later eliminated, and depth (7.4%) ().
The results of variance partitioning between the three most significant environmental variables (as determined by constrained RDAs, but excluding Secchi depth) indicate that LOI and bottom water temperature can be considered statistically independent, as each variable captures a significant amount of variation regardless of the covariables used in the analysis (). By contrast, lake depth covaried with LOI and bottom water temperature when entered together as covariables. However, lake depth did contribute independently to the overall variance when LOI and bottom temperature were entered as single covariables (). LOI proved to be the strongest variable of the four, explaining greater than 7% of the total variance regardless of the covariables used.
These results are comparable to CitationOlander et al. (1999) and CitationLarocque et al. (2001), who also found sediment organic content (as measured by LOI) to be the chief explanatory variable of chironomid assemblages in subarctic lakes of northern Fennoscandia and northern Sweden, respectively. The LOI gradient represented by our Yukon lakes was relatively long (9% to 80%; ) as was that of Olander et al. (17% to 88%) and Larocque et al. (3% to 87%). This may explain why CitationWalker et al. (1991) did not find sediment organic content to be a factor determining the distribution of chironomids across treeline in Labrador, where the LOI gradient was much shorter (6.5% to 40.5%). A number of studies on chironomid ecology have pointed to the importance of substrate in determining species composition and abundance (e.g., CitationMcGarrigle, 1980; CitationPinder, 1986). However, the relative importance of sediment type versus other limnological variables in determining chironomid community composition has been the focus of debate (e.g., CitationWarwick, 1989; CitationWalker and Mathewes, 1989a; CitationWalker et al., 1992). Often, sediment organic content is highly correlated with lake depth and surface water temperature as well, when lakes are sampled along strong latitudinal and altitudinal gradients (CitationWalker et al., 1991; CitationLotter et al., 1997; CitationOlander et al., 1999), making it difficult to separate the effects of these variables on chironomid communities. Surface water temperature was not a factor in determining chironomid assemblages in this study largely because lakes were not sampled along a strong climatic gradient. LOI was negatively correlated with depth (), although the results of the variance partitioning indicate that LOI contributed a large and statistically significant independent contribution to the overall variance when the effects of lake depth and bottom water temperature were partialled out. CitationOlander et al. (1999) and CitationLarocque et al. (2001) also found LOI to vary independently from both surface-water and/or air temperature and lake depth.
Other variables that were important in influencing chironomid composition in these lakes, as determined by forward selection, included total phosphorus, bottom water temperature, and alkalinity. Ion strength and composition are known to affect chironomid distributions (CitationPinder, 1986; CitationWalker et al., 1995). Bottom water temperature is usually a measure of water depth and the degree of thermocline development, with the deepest lakes having the lowest bottom temperatures. Lake depth and bottom temperature were negatively correlated in this study (); however, Tb did have an independent influence on chironomid distributions that was unrelated to lake depth (). Water temperature has been shown to have a strong influence on chironomid communities (CitationWalker et al., 1991; CitationOlander et al., 1999), mostly through its effects on the growth and development of larvae (CitationOliver, 1971; CitationWalker and Mathewes, 1989b). Numerous studies have shown that chironomids respond to changes in the availability of hypolimnetic oxygen caused by changes in the trophic conditions of lakes (e.g., CitationMerilainen et al., 2000; CitationLittle et al., 2000; CitationFrancis, 2001). Few studies have identified TP as a strong variable for identifying these changes (e.g., CitationClerk et al., 2000), although CitationBrodersen and Lindegaard (1997) show TP to be important in determining chironomid communities in shallow lakes where hypolimnetic oxygen is not a factor. Similarly, many of our sites were shallow with uniform oxygen profiles, although the TP values for our study lakes were much smaller than those of CitationBroderson and Lindegaard (1997).
Although LOI seems to be a major determinant of the chironomid assemblages in these lakes, a large number of environmental variables control the distribution of larval chironomids, and some of these factors determine the nature of the sediment organic matter itself. The study of sediment type appears to be an efficient means to study the joint impact of many of the above variables, as the sediments reflect the interactions of nutrient levels, light penetration, aquatic productivity, wind and wave action, distance from shore, and vegetation type (CitationMcGarrigle, 1980).
ECOLOGY OF CHIRONOMID TAXA AND COMPARISON WITH OTHER REGIONS
The RDA biplot placed chironomids indicative of high sediment organic content (LOI) on the far left side of the diagram (e.g., Tanytarsus sp. C, Procladius, Tanytarsus pallidicornis type, Allo/Mesopsectrocladius, Psectrocladius spp., Microtendipes, Dicrotendipes, and Polypedilum), and taxa indicative of more minerogenic sediments on the far right (e.g., Chironomus, Paratanytarsus, Sergentia, Corynocera oliveri type, Cricotopus/Orthocladius [C. tremulus type], and Micropsectra atrofasciata type) (). The first axis also represents a gradient of lake depth, with shallow lakes occurring on the left and deeper lakes on the right. Chironomids characteristic of the deepest lakes with cold hypolimnetic or bottom waters are positioned in the upper right quadrant (e.g., Sergentia, Corynocera oliveri type, Micropsectra atrofasciata type, Heterotrissocladius, Eukiefferiella, Tanytarsus lugens/C. oliveri type) while chironomids typical of the shallow lakes with higher bottom water temperatures are positioned in the lower left quadrant (e.g., Microtendipes, Polypedilum, Cladopelma, Dicrotendipes, Tanytarsus lugens group, Cladotanytarsus mancus group, and Psectrocladius E/F type). This latter group of taxa is also characteristic of high nutrient (TP and DOC) lakes (). Shallow lakes with warm bottom temperatures are typically more productive, having an abundance of algae and aquatic plants that yield higher organic sedimentation—hence, the position of these lakes on the left side of the ordination in the direction of increasing LOI.
The above trends are comparable to other studies of modern chironomid assemblages. Heterotrissocladius, Sergentia, and Micropsectra are common in the profundal zone of deep, thermally stratified lakes in temperate regions (CitationWalker, 1991). Heterotrissocladius and Micropsectra are more typical of oligotrophic lakes (CitationSaether, 1979), although Micropsectra has been known to occur across a trophic gradient (CitationLods-Crozet and Lachavanne, 1994) and was common in mesotrophic lakes in the Swiss Alps (CitationLotter et al., 1998). Sergentia was most abundant in oligotrophic (<10 μg L−1 TP) lakes in our data (, ), although it commonly thrives under mesotrophic conditions (moderate oxygen depletion) (CitationWalker, 1991; CitationQuinlan et al., 1998). However, as very few deep, mesotrophic lakes were sampled in this study, we may simply have missed the preferred niche of this genus. The shallow-water/littoral taxa observed in our lakes are also typical of other regions (e.g., CitationSchmäh, 1993; CitationWalker and MacDonald, 1995; CitationKorhola et al., 2000). One exception may be the Tanytarsus lugens group, which in our data was common in shallow, productive lakes ( and ), but is considered an oligotrophic taxon (e.g., CitationSaether, 1979; Brundin, 1956, in CitationHofmann, 1986), and a cold stenotherm (CitationHofmann, 1988). T. lugens was also present in many oligotrophic lakes of northern Fennoscandia (CitationKorhola et al., 2000). However, CitationLotter et al. (1998) show that T. lugens group is common in mesotrophic lakes from the Alps, and it was a dominant taxon in the mesotrophic profundal zone of Lake Geneva (CitationLods-Crozet and Lachavanne, 1994). CitationAlm and Willassen (1993) and CitationHofmann (1988) also note that it occurs as a profundal species in oligotrophic to weakly eutrophic lakes of middle Europe but can occur in the littoral zone of subarctic lakes where temperatures are lower. These apparent differences in ecology may be related in part to taxonomic uncertainties. Difficulties can arise in distinguishing C. oliveri and T. lugens as noted above (T. lugens group and C. oliveri type grouped in some cases) and by CitationAlm and Willassen (1993). C. oliveri is considered a cold stenotherm (Pinder and Reiss in CitationOlander et al., 1999) and did occur in the deepest lakes with the lowest bottom water temperatures in our sites ( and ). However, given the lack of ecological information on littoral chironomid communities (CitationHofmann, 1986), it is possible that T. lugens has a more widespread trophic and bathymetric distribution than previously documented.
The chironomid distribution with respect to LOI in our data compares well with CitationOlander et al. (1999). Dicrotendipes, Psectrocladius, Allo/Mesopsectrocladius, Microtendipes, and Polypedilum occur in highly organic sediments, and Heterotrissocladius, Micropsectra, and Corynocera oliveri type occur in more minerogenic sediments in both regions. Similarly, CitationLarocque et al. (2001) found Dicrotendipes, Polypedilum, and Microtendipes in more organic sediments, as well as Tanytarsus sp. C and Tanytarsus pallidicornis type, which were prominent in the most organic-rich sediments in our study lakes ( and ). Heterotrissocladius and Micropsectra were also more abundant in Swedish lakes with lower LOI values. One major difference was the dominance of Chironomus in Yukon lakes with the least organic sediment, compared to the Fennoscandian and Swedish lakes, where it was most abundant in highly organic lake bottoms. CitationMcGarrigle (1980) also found species of Chironomus to be more abundant in sediment with high organic content. The three Yukon lakes where Chironomus is most abundant (Donjek, Rat, and Small) have low to moderate oxygen concentrations near the lake bottom (), and in the case of Small Lake, stable oxygen readings were difficult to obtain. It is possible that low oxygen is responsible for their success in these lakes and that the low organic matter content is simply a coincidence. Chironomus was dominant in Donjek Kettle, and this site undoubtedly has a large influence on the relationship of LOI with Chironomus abundance. Donjek Kettle receives large inputs of loess from the Donjek River valley. Perhaps the loess input dilutes the organic matter in the sediment, while the lake otherwise has conditions suitable for Chironomus survival.
This study confirms the importance of sediment organic matter in determining the distribution of chironomid populations in lakes from the southwest Yukon. Sediment organic matter in turn depends on the productivity of the lake in addition to watershed conditions. Future work will investigate the coupling of these ecosystems and the factors causing them to change in time and space.
FIGURE 1. Map of study site in southwest Yukon and northern British Columbia showing location of study lakes
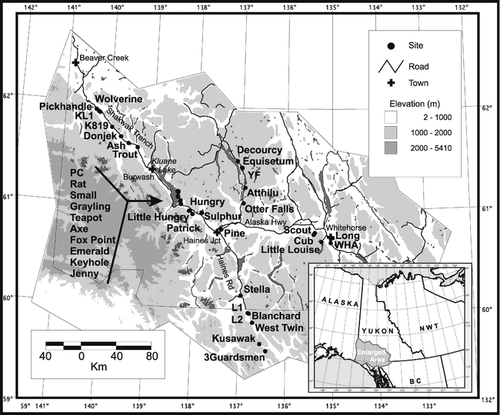
FIGURE 2. Chironomid assemblages from lakes in southwest Yukon and northern British Columbia arranged by weight-loss-on-ignition (%LOI) values. Weight-loss-on-ignition, bottom water temperature, lake depth, and number of head capsules shown for these sites. Three Guardsmen Lake is included to show chironomid fauna even though it was eliminated from the ordinations
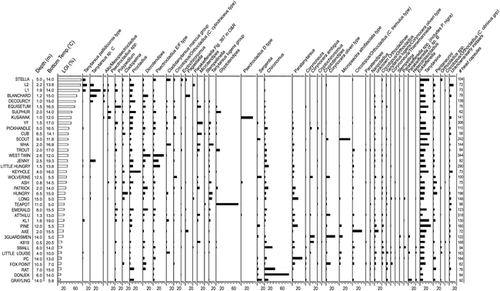
FIGURE 3. Redundancy analysis (RDA) covariance biplots showing the relationship between the 8 measured environmental variables with a statistically significant relationship to chironomid distributions and the 37 lakes (A) and the 38 chironomid taxa (B). Abbreviations for the chironomid taxa are: CHIRO—Chironomus; CLADO—Cladopelma; CLTAN—Cladotanytarsus mancus group; CORAM—Corynocera ambigua; COROL—Corynocera oliveri type, CORYN—Corynoneura; C/T—Corynoneura/Thienemanniella; C/O–CYL—Cricotopus/Orthocladius (C. cylindraceus type); C/O–TRE—Cricotopus/Orthocladius (C. tremulus type); C/O—Cricotopus/Orthocladius; DICRO—Dicrotendipes; ENDO—Endochironomus; EUKF—Eukiefferiella; GLYP—Glyptotendipes; HETER—Heterotrissocladius; MICRPS—Micropsectra atrofasciata type; MICRO—Microtendipes; NANO—Nanocladius; ORTHO—unknown Orthocladiinae; ORTHSPP—Orthocladius spp.; PAGA—Pagastiella; PAKB—Parakiefferiella cf. sp. B (CitationWalker, 1988); PAK367—Parakiefferiella Fig. 367 in Oliver and Roussel; PAKSPP—Parakiefferiella spp. (including P. nigra); PARAT—Paratanytarsus; PENT—Pentaneurini; POLY—Polypedilum; PROC—Procladius; PSECTEF—Psectrocladius E/F type (Fig. 9.61 E/F in Wiederholm); PSECTD—Psectrocladius D type (Fig. 9.61 D in Wiederholm); ALLOMES—Allo/Mesopsectrocladius; PSECT—Psectrocladius spp.; SERG—Sergentia; TANYT—Tanytarsina; TANYC—Tanytarsus sp. C; TANLU—Tanytarsus lugens group; TANLU/COROL—Tanytarsus lugens/Corynocera oliveri type; TANYP—Tanytarsus pallidicornis type
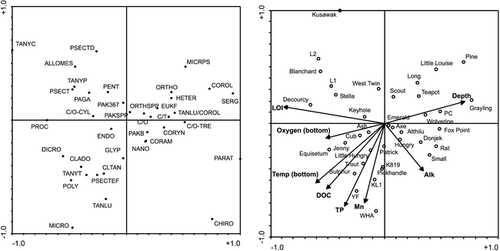
TABLE 1 Water chemistry and physical variables from 39 lakes in southwest Yukon and northern British Columbia. Alkalinity (Alk) was measured as mg/L CaCO3, and conductivity was corrected to 25°C. Lake area was calculated manually from 1:50,000 maps (Energy, Mines and Resources, Canada). “NA” indicates data were not available and, therefore, mean values for that variable were substituted. The variables total phosphours (TP), filtered phosphorus (TP-F), Al, and Mo had detection limit values substituted in some cases (see explanation in text). These were 4 μg/L, 4 μg/L, 0.001 mg/L, and 0.0005 mg/L, respectively. In the case of bottom oxygen for Patrick Lake and K819, these values were assumed to be the same as surface oxygen because both lakes were less than 2 m deep. Elev. = elevation, T = surface water temperature, Tb = bottom water temperature, DOs = surface oxygen, DOb = bottom oxygen, Cond = conductivity, Alk = alkalinity, DOC = dissolved organic carbon, TKN = total Kjeldahl nitrogen, Chla = chlorophyll a, LOI = loss-on-ignition
TABLE 1 (Ext)
TABLE 2 Canonical coefficients, t values, and intra-set correlations for the subset of eight environmental variables for redundancy analysis axes 1 and 2. DOC = dissolved organic carbon, TP = total phosphorus, LOI = loss-on-ignition
TABLE 3 The ratio of the first constrained eigenvalue to the second unconstrained eigenvalue, percentage variance explained by the environmental variables, and results of the Monte Carlo Permutation tests (500 unrestricted permutations) in a constrained redundancy analysis where each variable is used as the sole constraining variable at a time. DOC = dissolved organic carbon, TP = total phosphorus TKN = total Kjeldahl nitrogen, Chla = chlorophyll a, LOI = loss-on-ignition
TABLE 4 Summary of partial redundancy analysis of modern chironomid assemblages in the 37 lakes. P = significance level of Monte Carlo permutation test (500 unrestricted permutations). LOI = loss-on-ignition, Tbot = bottom temperature
Acknowledgments
We thank J. Bunbury for picking chironomids and M. Vetter for help in the field. M. Sawada developed the first version of . Thanks to P. Johnson and students of GEG 4001 for invaluable help in many ways. This work was supported by a Natural Sciences and Engineering Research Council of Canada (NSERC) postdoctoral fellowship to Wilson and a NSERC grant to Gajewski.
References Cited
- Alm, T. and E. Willassen . 1993. Late Weichselian Chironomidae (Diptera) stratigraphy of Lake Nedre Æråsvatn, Andøya, northern Norway. Hydrobiologia 264:21–32.
- Bengtsson, L. and M. Enell . 1986. Chemical analysis. In: Berglund, B. E. (ed.), Handbook of Holocene palaeoecology and palaeohydrology. New York, John Wiley and Sons, 423–454.
- Borcard, D. , P. Legendre , and P. Drapeau . 1992. Partialling out the spatial component of ecological variation. Ecology 73:1045–1055.
- Brinkhurst, R. O. 2002. The benthos of lakes. New Jersey, Blackburn Press, 190 pp.
- Brodersen, K. P. and C. Lindegaard . 1997. Significance of subfossil chironomid remains in classification of shallow lakes. Hydrobiologia 342/343:125–132.
- Brooks, S. J. and H. J B. Birks . 2000. Chironomid-inferred late-glacial and early-Holocene mean July air temperatures for Kråkenes Lake, western Norway. Journal of Paleolimnology 23:77–89.
- Clerk, S. , R. Hall , R. Quinlan , and J. P. Smol . 2000. Quantitative inferences of past hypolimnetic anoxia and nutrient levels from a Canadian Precambrian shield lake. Journal of Paleolimnology 23:319–336.
- Currie, D. C. and I. R. Walker . 1992. Recognition and palaeohydrologic significance of fossil black fly larvae, with a key to the Nearctic genera (Diptera: Simuliidae). Journal of Paleolimnology 7:37–54.
- Environment Canada, 1983. Sampling for Water Quality. Water Quality Branch, Inland Waters Directorate, Ottawa, Ontario, 55 pp.
- Francis, D. 2001. A record of hypolimnetic oxygen conditions in a temperate multi-depression lake from chemical evidence and chironomid remains. Journal of Paleolimnology 25:351–365.
- Gabrielse, H. , D. J. Tempelman-Kluit , S. L. Blusson , and R. B. Campbell . 1977. Geological Survey of Canada Map 1398A, scale = 1:1,000,000.
- Glew, J. R. 1988. A portable extruding device for close interval sectioning of unconsolidated core samples. Journal of Paleolimnology 1:235–239.
- Glew, J. R. 1991. Miniature gravity corer for recovering short sediment cores. Journal of Paleolimnology 5:285–287.
- Heiri, O. and A. Lotter . 2001. Effect of low sum counts on quantitative environmental reconstructions: an example using subfossil chironomids. Journal of Paleolimnology 26:343–350.
- Hofmann, W. 1986. Chironomid analysis. In Berglund, B.E. (ed.), Handbook of Holocene palaeoecology and palaeohydrology. New York, John Wiley and Sons, 715–726.
- Hofmann, W. 1988. The significance of chironomid analysis (Insecta: Diptera) for paleolimnological research. Palaeogeography, Palaeoclimatology, Palaeoecology 62:501–509.
- Johnson, F. and H. M. Raup . 1964. Investigations in southwest Yukon: geobotanical and archaeological reconnaisance. Andover, Massachusetts, Papers of the Robert S. Peabody Foundation for Archaeology, 6, 488 pp.
- Juggins, S. and C. J F. ter Braak . 1997. CALIBRATE version 0.70: a C++ program for analysing and visualising species environment relationships and for predicting environmental values from species assemblages. Steve Juggins, Department of Geography, University of Newcastle, Newcastle upon Tyne, UK NE1 7RU. [email protected] .
- Korhola, A. , H. Olander , and T. Blom . 2000. Cladoceran and chironomid assemblages as quantitative indicators of water depth in subarctic Fennoscandian lakes. Journal of Paleolimnology 24:43–54.
- Larocque, I. , R. I. Hall , and E. Grahn . 2001. Chironomids as indicators of climate change: a 100-lake training set from a subarctic region of northern Sweden (Lapland). Journal of Paleolimnology 26:307–322.
- Little, J. L. , R. I. Hall , R. Quinlan , and J. P. Smol . 2000. Past trophic status and hypolimnetic anoxia during eutrophication and remediation of Gravenhurst Bay, Ontario: comparison of diatoms, chironomids, and historical records. Canadian Journal of Fisheries and Aquatic Sciences 57:333–341.
- Lods-Crozet, B. and J-B. Lachavanne . 1994. Changes in the chironomid communities in Lake Geneva in relation with eutrophication, over a period of 60 years. Archiv für Hydrobiologia 130:453–471.
- Lotter, A. F. , H. J B. Birks , W. Hofmann , and A. Marchetto . 1997. Modern diatom, cladocera, chironomid, and chrysophyte cyst assemblages as quantitative indicators for the reconstruction of past environmental conditions in the Alps. I. Climate. Journal of Paleolimnology 18:395–420.
- Lotter, A. F. , H. J B. Birks , W. Hofmann , and A. Marchetto . 1998. Modern diatom, cladocera, chironomid, and chrysophyte cyst assemblages as quantitative indicators for the reconstruction of past environmental conditions in the Alps. II. Nutrients. Journal of Paleolimnology 19:443–463.
- McGarrigle, M. L. 1980. The distribution of chironomid communities and controlling sediment parameters in L. Derravaragh, Ireland. In Murray, D. A. (ed.), Chironomidae: ecology, systematics, cytology and physiology. Toronto, Pergamon Press, 275–282.
- Merilainen, J. J. , J. Hynynen , A. Teppo , A. Palomaki , K. Granberg , and P. Reinikainen . 2000. Importance of diffuse nutrient loading and lake level changes to the eutrophication of an originally oligotrophic boreal lake: a palaeolimnological diatom and chironomid analysis. Journal of Paleolimnology 24:251–270.
- Olander, H. , H. J B. Birks , A. Korhola , and T. Blom . 1999. An expanded calibration model for inferring lakewater and air temperatures from fossil chironomid assemblages in northern Fennoscandia. The Holocene 9:279–294.
- Oliver, D. R. 1971. Life history of the Chironomidae. Annual Review of Entomology 16:211–230.
- Oliver, D. R. and M. E. Roussel . 1983. The insects and arachnids of Canada. Part 11: the genera of larval midges of Canada, Diptera: Chironomidae. Ottawa, Agriculture Canada Publication No. 1746, 263 p.
- Oswald, E. T. and J. P. Senyk . 1977. Ecoregions of Yukon Territory. Victoria, British Columbia, Canadian Forestry Service, Environment Canada, 115 pp.
- Pinder, L. C V. 1986. Biology of freshwater Chironomidae. Annual Review of Entomology 31:1–23.
- Porinchu, D. and L. Cwynar . 2000. The distribution of freshwater Chironomidae (Insecta: Diptera) across treeline near the lower Lena River, northeast Siberia, Russia. Arctic, Antarctic, and Alpine Research 32:429–437.
- Porinchu, D. and G. MacDonald . 2003. The use and application of freshwater midges (Chironomidae: Insecta: Diptera) in geographical research. Progress in Physical Geography 27:378–422.
- Pourriot, R. and M. Meybeck . 1995. Limnologie générale. Paris, Masson, 956 pp.
- Quinlan, R. and J. P. Smol . 2001. Setting minimum head capsule abundance and taxa deletion criteria in chironomids-based inference models. Journal of Paleolimnology 26:327–342.
- Quinlan, R. , J. P. Smol , and R. I. Hall . 1998. Quantitative inferences of past hypolimnetic anoxia in south-central Ontario lakes using fossil midges (Diptera: Chironomidae). Canadian Journal of Fisheries and Aquatic Sciences 55:587–596.
- Saether, O. A. 1979. Chironomid communities as water quality indicators. Holarctic Ecology 2:65–74.
- Schmäh, A. 1993. Variation among fossil chironomid assemblages in surficial sediments of Bodensee-Untersee (SW-Germany): implications for paleolimnological interpretation. Journal of Paleolimnology 9:99–108.
- Tabachnick, B. G. and L. S. Fidell . 1989. Using multivariate statistics (second edition). New York, Harper Collins, 746 pp.
- ter Braak, C. J F. and P. Šmilauer . 1998. CANOCO for Windows: software for canonical community ordination (version 4). Ithaca, New York, Microcomputer Power.
- Wahl, H. E. , D. B. Fraser , R. C. Harvey , and J. B. Maxwell . 1987. Climate of Yukon. Ottawa, Ontario, Climatological Studies Number 40, Environment Canada, 323 pp.
- Walker, I. R. 1987. Chironomidae (Diptera) in paleoecology. Quaternary Science Reviews 6:29–40.
- Walker, I. R. 1988. Late-Quaternary palaeoecology of Chironomidae (Diptera: Insecta) from lake sediments in British Columbia. Ph.D. dissertation. Burnaby, British Columbia, Simon Fraser University, 204 pp.
- Walker, I. R. 1991. Modern assemblages of arctic and alpine Chironomidae as analogues for late-glacial communities. Hydrobiologia 214:223–227.
- Walker, I. R. 2000. The www field guide to subfossil midges. http://www.ouc.bc.ca/eesc/iwalker/wwwguide/ (last accessed November 2004).
- Walker, I. R. and G. M. MacDonald . 1995. Distributions of Chironomidae (Insecta: Diptera) and other freshwater midges with respect to treeline, Northwest Territories, Canada. Arctic and Alpine Research 27:258–263.
- Walker, I. R. and R. W. Mathewes . 1989a. Much ado about dead Diptera. Journal of Paleolimnology 2:19–22.
- Walker, I. R. and R. W. Mathewes . 1989b. Chironomidae (Diptera) remains in surficial lake sediments from the Canadian Cordillera: analysis of the fauna across an altitudinal gradient. Journal of Paleolimnology 2:61–80.
- Walker, I. R. , A. J. Levesque , R. Pienitz , and J. P. Smol . 2003. Freshwater midges of the Yukon and adjacent Northwest Territories: a new tool for reconstructing Beringian paleoenvironments?. Journal of the North American Benthological Society 22:323–337.
- Walker, I. R. , E. D. Reavie , S. Palmer , and R. N. Nordin . 1993. A palaeoenvironmental assessment of human impact on Wood Lake, Okanagan Valley, British Columbia, Canada. Quaternary International 20:51–70.
- Walker, I. R. , J. P. Smol , D. R. Engstrom , and H. J B. Birks . 1991. An assessment of Chironomidae as quantitative indicators of past climatic change. Canadian Journal of Fisheries and Aquatic Sciences 48:975–987.
- Walker, I. R. , J. P. Smol , D. R. Engstrom , and H. J B. Birks . 1992. Aquatic invertebrates, climate, scale, and statistical hypothesis testing: a response to Hann, Warner, and Warwick. Canadian Journal of Fisheries and Aquatic Sciences 49:1276–1280.
- Walker, I. R. , S. E. Wilson , and J. P. Smol . 1995. Chironomidae (Diptera): quantitative palaeosalinity indicators for lakes of western Canada. Canadian Journal of Fisheries and Aquatic Sciences 52:950–960.
- Warwick, W. F. 1989. Chironomids, lake development and climate: a commentary. Journal of Paleolimnology 2:15–17.
- Wiederholm, T. (ed.),. 1983. Chironomidae of the Holarctic region. Keys and diagnoses. Part 1—Larvae. Entomologica Scandinavica, Supplement 19, 457 pp.
- Wilson, S. E. and K. Gajewski . 2002. Surface sediment diatom assemblages and water chemistry from 42 subarctic lakes in the southwestern Yukon and northern British Columbia, Canada. Ecoscience 9:256–270.