Abstract
A complex sequence of sawtooth moraines occurs on the proglacial foreland at Lambatungnajökull, southeast Iceland. These features reflect the pattern of deglaciation of a highly crevassed ice margin. The whole sequence documents a period of overall glacier recession exceeding 450 m. Dates have been interpolated for the formation of these moraines through the examination of aerial photographs and the application of lichenometry. The moraines formed annually between AD ∼1932 and 1950. Retreat rates were greatest between 1933 and 1939 and slowest between 1942 and 1944, as reflected by the spacing of annual moraine ridges. Differences in glacier recession rate from year to year are related to variations in mean summer air temperature. A rise in mean summer temperature of 1°C results in a retreat rate of ∼30 m yr−1. It is shown that annual moraine spacings are a suitable geomorphological proxy for annual net glacier balance and a potentially valuable source of paleoclimatic information.
Introduction
The former extent of glaciers is an important source of information regarding past climates. It has been shown that temperate glaciers and small ice caps are sensitive indicators of climatic change (e.g., CitationNye, 1965; CitationRosqvist and Østrem, 1989; CitationKlok and Oerlemans, 2003). Many studies have pointed towards the importance of air temperature and precipitation in determining volume changes, and in turn glacier-front fluctuations, particularly on a timescale of decades to centuries (e.g., CitationHoinkes, 1968; CitationOerlemans, 1989; CitationJohannesson and Sigurðsson, 1998). Less well studied is the direct link between small-scale snout fluctuations and variations in climate from year to year. This paper aims to test the relationship between air temperatures and annual glacier recession during two decades of the 20th century. If a quantitative correlation can be found between glacier retreat and ablation-season temperature, the possibility of reconstructing former air temperatures from known annual ice-margin fluctuations becomes a realistic one.
Twentieth-century annual moraine sequences have been identified adjacent to several glaciers in Iceland (CitationThorarinsson, 1967; CitationPrice, 1970; CitationSharp, 1984; CitationBoulton, 1986; CitationKruger, 1995). Numerous workers have described how these minor moraines are created, relating the method of formation to summer retreat and subsequent winter readvance during a period of overall glacier recession. Working on the subantarctic island of South Georgia, CitationTimmis (1986) found that annual ice-front recession was proportional to the net glacier balance during a given year. Annual moraine spacings corresponded well with the measurements of net balance over a 5-yr period. CitationKruger (1995) made a general comparison between annual retreat of the northern margin of Mýrdalsjökull in southern Iceland and mean summer air-temperature trends in Stykkisholmur, since 1906. His findings implied that these minor ice-front fluctuations were in tune with changes in air temperatures during the ablation season. This study aims to build on this work by identifying a suitable geomorphological proxy for annual net glacier balance and exploring its connection with air temperature measurements over a period of years.
Study Area
Lambatungnajökull, an outlet glacier of Vatnajökull in southeast Iceland (), is a temperate glacier approximately 10 km in length. It flows in a relatively narrow, steep-sided trough trending northwest–southeast, gradually curving around to flow east in its lower reaches (). Unlike many other glaciers in Iceland that terminate as a broad lobe on a level outwash plain, Lambatungnajökull's terminus is presently confined by valley walls to the north and south (). However, supraglacial debris is notably absent.
Although the ice flow is currently restricted to one valley, Skyndidalur (), there is evidence to suggest that during previous periods of glacier expansion the ice breached a col in the valley side and flowed south into the neighboring valley of Hoffellsdalur. This latter valley is now ice free but an extensive system of moraines reflects the various stages of glacial growth and decay (CitationBradwell, 2001a). The main valley also exhibits a wealth of geomorphological evidence relating to the former extent of the glacier.
Information regarding the former extent of Lambatungnajökull is limited. The main reference in the scientific literature to glacier fluctuations at Lambatungnajökull is by Sigurdur CitationThorarinsson (1943) who visited the area briefly in the summer of 1938. He devoted one page of his 200-page study of Icelandic glaciers to the former extent of the ice-flow. Thorarinsson stated (1943: 6) that “the ice was just leaving the pass from Hoffellsdalur to Skyndidalur” during the autumn of 1938. With the aid of a map (1943: ) he partially sketched the glacier's maximum extent in historical time, which he believed to have been in ∼AD 1890. The best sources of information regarding ice-front fluctuations in the last 50 yr are aerial photographs taken in AD 1945, 1957, 1967, 1982, 1989, and 1998.
THE LANDFORMS
Geomorphological mapping at Lambatungnajökull in 1999 revealed a distinctive landform assemblage on the proglacial foreland in Skyndidalur, approximately 500 m from the present-day glacier margin (). The assemblage is characterized by low-elevation ridges (<3 m) and elongated hummocks forming a remarkably regular series of sawtooth, in places, chevron-shaped ridges. The zigzag landforms possess distinct lineations which trend east–west before sharply turning at angles of between 30 and 60 degrees and continuing for some distance. Most of the ridges are asymmetrical in cross profile with a slightly steeper distal slope. The ridges nearest to the ice margin are crenulated features with connected arcuate sections. The complete sequence covers an area of approximately 400 m × 500 m and consists of up to 20 individual, subparallel, lineations.
All the ridges in question are composed of gray-brown, poorly sorted, clay-rich diamict. Clasts ranging from 1 to 50 cm are set within a soft, clay-sand matrix. Large cobbles and boulders are notably absent. Most of the matrix-supported sediment is subangular to subrounded gravel and cobbles (6–240 mm). Clast lithologies reflect the local geology and can be subdivided into vesicular and porphyritic basalt, dolerite, pyroclastic agglomerate, and tuffaceous sediment. The diamict is homogeneous, although elongate clasts have a tendency to be vertically orientated.
LANDFORM GENESIS AND INTERPRETATION
In 1999, the ice-front at Lambatungnajökull exhibited a simple, arcuate form. Although the lateral margins are steep and highly crevassed, the glacier snout slopes gently and is free from crevassing. There is evidence to suggest, however, that the ice margin was previously irregular in form and intensely crevassed. A vertical aerial photograph from 1945 (US Army Mapping Service: #51/1210) shows an intricate ice margin with sharp promontories, tight embayments, and deep crevasses. This snout morphology is similar to that of several nearby valley glaciers that terminate on broad outwash plains. Skeiðarárjökull, Fjallsjökull, Breiðamerkurjökull, and Skálafellsjökull, have all exhibited distinctive crenulated margins during the last 50 yr (CitationThorarinsson, 1967; CitationPrice, 1970; CitationSharp, 1984; CitationBoulton, 1986). All four of these glaciers possess splaying, radial crevassing at their margins as a result of diverging flow. Furthermore, the forelands of these glaciers exhibit sequences of closely spaced minor moraines. Sawtooth moraines, characterized by downvalley-pointing teeth and upvalley-pointing notches, can be seen as a special form of minor moraine. These features, representing the former position of a crevassed ice-margin, were described at Fjallsjökull (CitationPrice, 1970) but not formally named until some years later (CitationMatthews et al., 1979). At Skeiðarárjökull, Breiðamerkurjökull and Skálafellsjökull these minor moraines reflect fluctuations of the ice margin from year to year and are good examples of annual moraines (CitationThorarinsson, 1967; CitationSharp, 1984; CitationBoulton, 1986).
The simplest mechanism of minor-moraine formation involves the extrusion of subglacial till from beneath the snout of the glacier during minor, winter, readvance events (CitationSharp, 1984), although the bulldozing of existing sediment into discrete ridges may generate a similar landform (CitationMatthews et al., 1979). A further mechanism involves basal freezing and the subsequent movement of lodgment till slabs at thin ice margins in cold continental climates (CitationKruger, 1995). At Lambatungnajökull the moraines are low-elevation, asymmetrical, ridges composed of clay-rich till with vertical clasts and, therefore, are likely to have resulted from subglacial squeezing, as reported elsewhere in southeast Iceland (CitationThorarinsson, 1967; CitationSharp, 1984). It is hypothesized that the ridges formed annually.
The particular subglacial dynamics at Lambatungnajökull may well explain the peculiar morphology of the minor moraines seen on the proglacial foreland. Located 350 m in front of the present-day ice margin is a streamlined, basaltic, bedrock hummock around 5 m high and 25 m in length (). This ice-molded roche moutonnée was exposed by the retreating glacier terminus between 1957 and 1967, according to aerial photographic evidence. However, in the decades prior to this time, relatively thin ice flowed over the bedrock prominence causing deep crevasses to open where stresses were highest, immediately up-ice of the obstruction. The resulting crevasse pattern determined the jagged snout morphology reflected in the irregular, chevron-shaped, moraine ridges preserved on the foreland (ridges a-n; ). After a period of several years the ice no longer flowed over the roche moutonnée and the morphology of the ice-margin became more regular and less crevassed. Ridges o-q probably formed during this time ().
Methods
CHRONOLOGY
In order to determine the 20th-century deglacial chronology of Lambatungnajökull a site-specific lichenometric dating (age-size) curve was constructed (CitationBradwell, 2001b). There are several surfaces of known age on the glacier foreland dating from the time interval covered by aerial photography. However, lichenometry is deemed inapplicable on surfaces less than 30 yr old due to the uncertain nature of lichen colonization and the initial growth phase (CitationBeschel, 1950, Citation1961; CitationArmstrong, 1983; CitationInnes, 1988). The only surfaces at Lambatungnajökull which can be used in the construction of a lichenenometric dating curve are those seen in contact with the ice margin in the 1945, 1957, and 1967 aerial photographs. Older surfaces at Hoffellsjökull, 15 km to the southwest, were used to constrain the dating curve over the early part of the 20th century.
Measurements of approximately 300 thalli of the lichen Rhizocarpon section Rhizocarpon were made on each of five surfaces of known age—three on the proglacial foreland at Lambatungnajökull and two at Hoffellsjökull (). The exact locations of these dated surfaces are given in CitationBradwell (2001b). Rhizocarpon geographicum (L). DC. was the most commonly measured lichen although other species within section Rhizocarpon were also probably included. The maximum diameter of every thallus over 5 mm was recorded within a fixed area, normally 30 m2. Ambiguous, coalescent thalli were avoided and, where possible, readings were only taken from boulders of similar lithology, namely Tertiary porphyritic basalt. No anomalously large thalli were identified in any of the five populations (CitationBradwell, 2001b). The diameter of the largest lichen in each of the five populations was plotted against the respective age of the substrates (), determined from aerial photographs taken in 1945, 1957, and 1967 and detailed surveys performed in 1903 (Danish General Staff) and 1930 (CitationEirikson, 1932). CitationThorarinsson's (1943) sketch map of Lambatungnajökull's ice margin in ∼1890 has not been used for calibrating the lichenometric dating curve because it is not accurate enough. Detailed inspection of recently uncovered ground adjacent to the ice margin, revealed between July 1982 and September 1989 (evidenced from aerial photographs), has provided a lichen colonization time of not less than 10 yr, and not more than 17 yr, at Lambatungnajökull. A lichenometric dating (age-size) curve for the study area covering the last 96 yr was generated using these control points ().
Aerial photographs taken by the U.S. Army in 1945 provide a crucial link in the reconstruction of Lambatungnajökull's recent fluctuations and provide a minimum age for the formation of the sawtooth moraine sequence. Close examination of the aerial photographs taken in August 1945 show the glacier to be almost in contact with ridge “m” (). From this observation it can be inferred that ridge “m” probably formed in the late winter or spring of 1945, a few months prior to the photograph being taken. Ridge “n” had not yet formed when the aerial survey was carried out in 1945, although the outline of the ice-margin does strongly reflect the morphology of the moraine ridge seen today. Lichen measurements from ridge “m” have been used to help constrain the lichen age-size curve ().
If lichen measurements from the most distal ridge (S9; ) can provide an age for its formation, the majority of the moraine sequence would be bracketed by two dates thus allowing the annual moraine hypothesis to be tested. To determine the age of the sawtooth moraine sequence, measurements of approximately 300 thalli of the lichen Rhizocarpon section Rhizocarpon were made within a fixed area on the proximal flank of end moraine S9. The same method used to construct the dating curve was employed in the lichenometric survey. The lichenometric measurements were restricted to only the slope proximal to the glacier, as distal slopes may include older, reworked, debris.
Results
The size-frequency distribution from moraine S9 indicates that the lichens comprise a single, rather than a composite, population (). No anomalously large lichens were recorded. The diameter of the largest lichen in the population was therefore applied to the lichenometric dating curve (). The result gives a close approximation of the minimum age of moraine S9. A largest-lichen diameter (within a fixed area) of 31 mm implies that the proximal flank of ridge S9 was exposed around AD 1932 (). Although the dating technique has a precision of probably no more than 2 or 3 yr (CitationInnes, 1988), the exact correspondence between the number of years elapsed between 1932 and 1945, and the number of ridges formed (13) strongly indicates that the sawtooth ridges are annual moraines. The whole sequence of 17 moraine ridges, assuming annual formation, therefore formed between ∼AD 1932 and 1950 ().
Discussion
Rapid retreat of Lambatungnajökull during the 1930s and 1940s, coupled with a debris-free glacier surface, has resulted in the formation of an unbroken sequence of annual moraine ridges. The presence of a confined meltwater system has ensured the preservation of these minor moraines up until the present day.
OTHER SUPPORTING EVIDENCE
The small lobe of Lambatungnajökull that breached the col at the head of Hoffellsdalur was monitored between 1935 and 1945 (CitationSigurðsson, 1998). The glacier tongue—known as “Hoffellsdalsjökull”—receded every year during this 10-yr period. Annual retreat rates for “Hoffellsdalsjökull,” taken from the database of the Iceland Glaciological Society (CitationSigurðsson, 1998), are shown in along with the equivalent annual recession for the main lobe of Lambatungnajökull, determined in this study. The similarity between the total amounts of ice-front recession over the 10-yr period is clear. The behavior of the Hoffellsdalur lobe is in good agreement with the lobe in Skyndidalur, implying a broadly similar response to climatic forcing between the two arms of the ice-flow. These data also corroborate the age of the sawtooth moraine sequence determined by lichenometry.
CLIMATOLOGICAL IMPLICATIONS
The formation of annual moraines requires a prolonged period of considerable ice-front recession, since the net recession in one ablation season must be greater than the net advance the following winter. Ablation on glacier margins is controlled chiefly by summer air temperature, with higher temperatures resulting in more rapid melting (CitationSutherland, 1984; CitationBraithwaite and Olesen, 1989; CitationPaterson, 1994). Assuming annual formation, distances between successive ridges measured along the former flow line, can be seen to represent the ice-front recession during a given mass-balance year.
Ice-front retreat rates at Lambatungnajökull, deduced in this paper, were compared with mean air temperatures at Holar í Hofn, the nearest weather station, 18 km to the south. Linear regression analyses were performed using winter/spring (February–May), summer (June–September) and autumn/winter (October–January) temperatures over the period 1932–1950 (). Temperatures were expressed as departures from the mean during the reference period 1961–1990. A significant positive correlation exists between the retreat rate of the ice-margin between 1932 and 1950 and mean summer temperatures at Holar í Hofn (r 2 = 0.56; P = 0.05) (). The greatest degree of glacier recession (>30 m yr−1) occurs when summer temperatures are more than 1°C higher than the 1961–1990 average. In contrast, retreat rates are close to zero when summer temperatures are at, or below, the 1961–1990 mean. No significant correlations were found between either February–May or October–January temperature and ice-front retreat rates (). A good match emerges when temperatures are plotted as a time series and compared with the corresponding retreat rate of the ice margin at Lambatungnajökull (). Warmer summers resulted in more rapid glacier recession—between 20 and 60 m yr−1; whereas in cooler summers the ice-front retreated less—only 8 m yr−1 on average in 1942, 1943, and 1944. Assuming a constant relationship between the temperature fluctuations at Holar and the study area, 18 km away, differences in ice-front retreat rate can be largely attributed to variations in mean air temperature during the ablation season (June–September).
One implication of this work is that certain moraine records can be used to study past climatic changes at a higher resolution than the response times of glaciers. This supports the assertions of CitationLowell et al. (1999) who argued that during warm periods ablation is the primary forcing factor on ice-front fluctuations. Even the margins of large ice masses, such as Vatnajökull, can respond to small variations in temperature through increased ablation. Consequently, annual moraine sequences in the geological record, once identified, could yield high-resolution paleoclimatic information.
Conclusions
Ice-front recession of around 470 m occurred between 1932 and 1950, depositing a sequence of 17 annually produced sawtooth moraines on the proglacial foreland at Lambatungnajökull. The distinctive zigzag moraine ridges represent former positions of an intensely crevassed ice margin. Preservation of these features has been aided by a low supraglacial debris supply and a confined meltwater system. Mean summer temperatures (June–September) from 1930 to 1955 represent the warmest period during the Icelandic instrumental record (since 1846) and were responsible for the rapid recession of the ice front between these years. Differences in summer air temperature from year to year account for the variations in ice-margin retreat rate experienced during the 1930s and 1940s. At Lambatungnajökull, a small rise in mean summer temperature of only 1°C results in retreat of ∼30 m yr−1, showing that the glacier margin is sensitive to even the smallest changes in climate. Hence, annual moraine spacings are a suitable geomorphological proxy for annual net glacier balance and a potentially valuable source of paleoclimatic information.
FIGURE 1. Map of southeast Iceland showing the location of the study area (boxed). Other glaciers referred to in the text are also shown
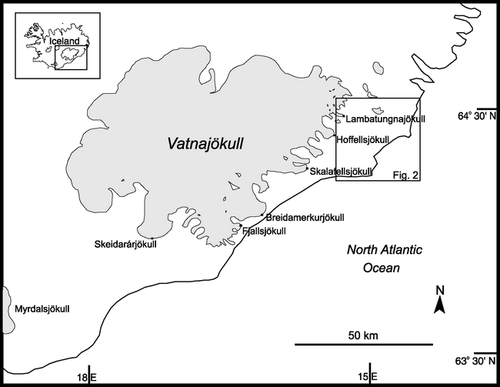
FIGURE 2. Topographic map of the Hornafjördur district, southeast Iceland. Contours are at 100-m vertical intervals. The area within the small rectangle is shown in detail in . The location of the weather station at Holar í Hofn is also shown
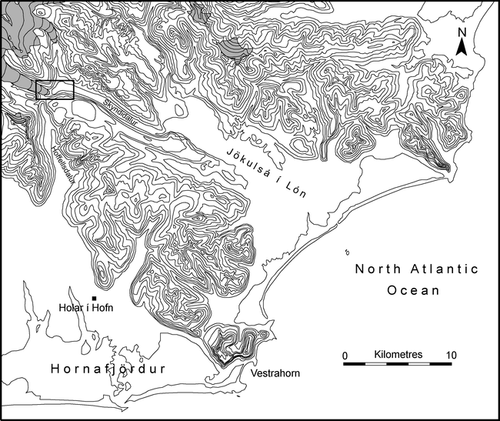
FIGURE 3. The terminus of Lambatungnajökull in 1998, viewed from the east. This photograph was taken from approximately where the ice margin would have been in ∼1890, according to CitationThorarinsson (1943)
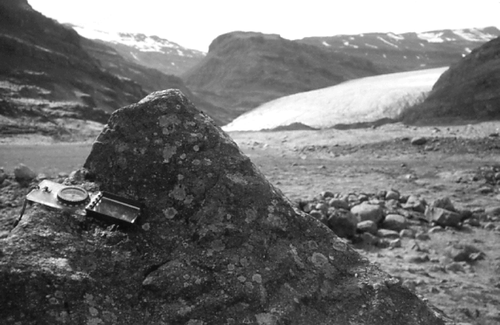
FIGURE 4. Map showing the sawtooth moraine sequence in front of Lambatungnajökull in relation to former ice-margin positions (Compiled from air photographs taken in 1945 and 1967; photos held at Landmaelingar Islands)
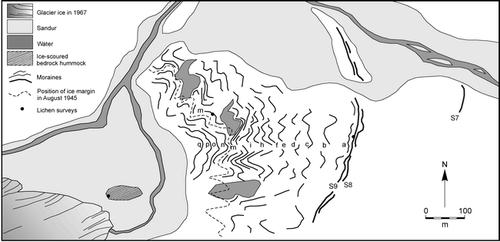
FIGURE 5. Lichenometric dating (age-size) curve for Lambatungnajökull. (Using single largest Rhizocarpon section Rhizocarpon lichen within a fixed area.) Numbers refer to calibration sites in . The age of moraine S9 has been interpolated using this curve and is shown as a dashed line
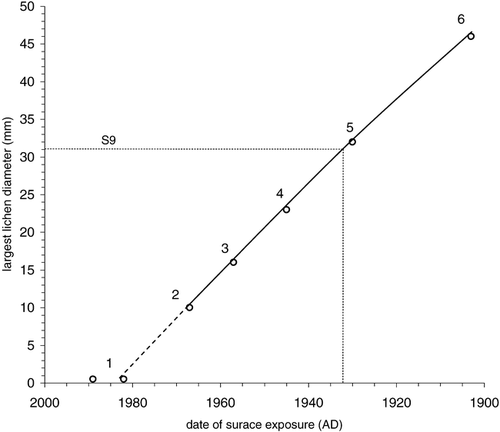
TABLE 1 Lichenometric data used to calibrate dating curve. (Note: survey conducted in 1999.) Refer to CitationBradwell (2001b) for more detailed descriptions of calibration sites
TABLE 2 Sawtooth annual moraine spacings (average) and respective formation dates, deduced from aerial photography and lichenometric data
TABLE 3 Comparative ice-front recession of the two lobes of the glacier. Figures for Lambatungnajökull in Skyndidalur are derived from this study. Figures for the “Hoffellsdalsjökull” lobe are taken from CitationSigurðsson (1998)
Acknowledgments
Thanks go to David Sugden, Andrew Dugmore, Jeremy Everest, Steve Roberts, and Sara Strachan for their thought-provoking discussions in the field and in Edinburgh. The author is grateful to the Department of Geography, University of Edinburgh and The Royal Geographical Society Dudley Stamp Memorial Trust for financial assistance. Michael Kaplan and Parker Calkin provided helpful comments on the manuscript.
References Cited
- Armstrong, R. A. 1983. Growth curve of the lichen. Rhizocarpon geographicum. New Phytologist 94:619–622.
- Beschel, R. E. 1950. Flechten als Altersmasstab Rezenter Moränen. Zeitschrift für Gletscherkunde und Glazialgeologie 1:2 152–161.
- Beschel, R. E. 1961. Dating rock surfaces by lichen growth and its application to glaciology and physiography (lichenometry). In Raasch, G. O. (ed.), Geology of the Arctic. Vol. 2. Toronto: University of Toronto Press, 1044–1062.
- Boulton, G. S. 1986. Push moraines and glacier-contact fans in marine and terrestrial environments. Sedimentology 33:677–698.
- Bradwell, T. 2001a. Glacier fluctuations, lichenometry and climatic change in Iceland. PhD thesis, University of Edinburgh: 365 pp.
- Bradwell, T. 2001b. A new lichenometric dating curve for southeast Iceland. Geografiska Annaler 83A:91–101.
- Braithwaite, R. J. and O. B. Olesen . 1989. Calculation of glacier ablation from air temperature, west Greenland. In Oerlemans, J. (ed.), Glacier Fluctuations and Climatic Change. Dordrecht: Kluwer Academic Press, 353–371.
- Eiríkson, H. H. 1932. Observations and measurements of some glaciers in Austur-Skaftafellssýsla, in the Summer of 1930. Vindefelag Islendinga, 12. Reykjavik.
- Hoinkes, H. C. 1968. Glacier variation and weather. Journal of Glaciology 7:3–17.
- Innes, J. L. 1988. The use of lichens in dating. In Galun, M. (ed.), CRC Handbook of Lichenology. Volume III. Boca Raton: CRC Press, Inc., 75–91.
- Johannesson, T. and O. Sigurðsson . 1998. Interpretation of glacier variations in Iceland 1930–1995. Jokull 45:27–33.
- Klok, E. J. and J. Oerlemans . 2003. Deriving historical equilibrium-line altitudes from a glacier length record by linear inverse modelling. The Holocene 13:343–351.
- Kruger, J. 1995. Origin, chronology and climatological significance of annual moraine ridges at Mýrdalsjökull, Iceland. The Holocene 5:420–427.
- Lowell, T. V. , R. K. Hayward , and G. H. Denton . 1999. Role of climate oscillations in determining ice-margin position: hypothesis, examples and implications. In Mickelson, D. M. and Attig, J. W., (eds.), Glacial Processes Past and Present. Boulder, Colorado: Geological Society of America Special Paper, 337: 193–203.
- Matthews, J. A. , R. Cornish , and R. A. Shakesby . 1979. ‘Saw-tooth’ moraines in front of Bødalsbreen, southern Norway. Journal of Glaciology 22:535–546.
- Nye, J. F. 1965. The frequency response of glaciers. Journal of Glaciology 5:567–587.
- Oerlemans, J. 1989. On the response of valley glaciers to climatic change. In Oerlemans, J. (ed.), Glacier Fluctuations and Climatic Change. Dordrecht: Kluwer Academic Press, 353–371.
- Paterson, W. S B. 1994. The Physics of Glaciers. 3rd ed. Oxford: Oxford University Press. 480 pp.
- Price, R. J. 1970. Moraines at Fjallsjökull, Iceland. Arctic and Alpine Research 2:27–42.
- Rosqvist, G. and G. Østrem . 1989. The sensitivity of a small ice-cap to climatic fluctuations. Geografiska Annaler 71A:99–103.
- Sharp, M. 1984. Annual moraine ridges at Skálafellsjökull, south-east Iceland. Journal of Glaciology 30:82–93.
- Sigurdsson, O. 1998. Glacier variations in Iceland 1930–1995. Jokull 45:3–26.
- Sutherland, D. G. 1984. Modern glacier characteristics as a basis for inferring former climates with particular reference to the Loch Lomond Stadial. Quaternary Science Reviews 3:291–309.
- Thorarinsson, S. 1943. Oscillations of the Icelandic glaciers in the last 250 years. Geografisker Annaler 25:1–54.
- Thorarinsson, S. 1967. Forvitnilegir jökulgarðar við jaðar Skeiðarárjökuls [Washboard moraines in front of Skeiðarárjökull]. Jokull 17:311–312.
- Timmis, R. 1986. Glacier changes in South Georgia and their relationship to climatic trends. Ph.D. thesis, University of East Anglia, U.K.