Abstract
Variations of the diatom distribution in a lake sediment core of Lake Lama, Central Siberia, were used to reconstruct paleotemperatures during the Holocene. The changes of the diatom assemblages revealed a strong association to climatic changes during this period. A transfer function based on weighted averaging partial least squares regression (WA-PLS), derived from a calibration dataset from northwestern Finnish Lapland, was used to infer July air temperatures for the area around Lake Lama. The prediction accuracy of the diatom-temperature model was 0.89°C. The analysis of the modern vs. core assemblages showed the suitability of the model for the lake sediment core. The reconstruction yielded a rapid increase in temperature at the beginning of the early Holocene. There was a period between 8000 and 6500 cal. yr BP with slightly higher temperatures, which were assigned to the Holocene thermal optimum (Hypsithermal), followed by a short and distinct temperature decrease. The period between 4000 and 2800 cal. yr BP again revealed higher temperatures. The inferred temperatures are consistent in their trends with a pollen-based paleotemperature reconstruction of the same core. The range of the diatom-based temperatures was 1.6°C, which is low compared to that of the pollen-based temperatures. This discrepancy may be explained by over- and underestimation of temperatures at the edges of the temperature range by our diatom-based model, and by the large size of this water body which could have moderated the response of diatoms to the temperature signal. The inferred temperature trends from the diatom assemblages at Lake Lama show the potential of aquatic organisms for paleoclimatic reconstructions. This is one of the very few quantitative paleoclimate reconstructions in arctic Russia. Previous research was limited mainly due to the lack of available calibration datasets. Our results also imply that, although this lack might be a drawback, an ecologically sound and reliable reconstruction is still possible and needs to be carried out in other parts of the arctic regions in order to obtain a more detailed knowledge about past regional climate and environmental changes.
Introduction
The quality of predicted climate scenarios largely depends on the complexity and the robustness of the climate models used. The improvement of such models requires the assimilation of known or inferred past climate variables. Since systematic measurements of temperature or precipitation extend back hardly more than one century, estimates of these variables in the past are urgently needed in order to study climatic variability at decadal or centennial time-scales and to understand the mechanisms of climate or climate-related processes.
It has been shown that ice cores, tree rings, and lake sediments often record their local or regional climatic history (CitationBriffa et al., 1992; CitationJouzel et al., 1996; CitationBattarbee, 2000). The biological record in lake sediments especially offers some opportunities to derive quantitative estimates of paleoclimatic or paleoenvironmental variables. If sedimentation in lakes occurred continuously, then they have an advantage over other paleoclimatic archives because of their occurrence under many different environmental situations at continental regions (e.g., CitationBarker et al., 1994; CitationKorhola et al., 2000; CitationLotter et al., 2000; CitationPienitz et al., 2000).
To date, the most reliable indicators of past climatic and environmental changes found in lake sediments are pollen, plant macrofossils, and remains of aquatic organisms. These indicators have been used to infer temperature and precipitation as well as salinity, pH, or nutrient status of lakes during the Holocene and Late Pleistocene (e.g., CitationBirks et al., 1990; CitationCheddadi et al., 1997; CitationHall et al., 1997; CitationSeppä and Weckström, 1999; CitationBrooks and Birks, 2000). Among them, diatoms are excellent proxies to reconstruct limnological or climatological characteristics of the past (see CitationStoermer and Smol, 1999). Diatoms usually occur in large quantities, are diverse, ubiquitous, and identifiable to a low taxonomic level. Many of the species have narrow ecological tolerances to their environment and they respond rapidly to changing environmental conditions due to their short life cycle. In addition, diatom valves are usually well preserved in lake sediments, for which reason the preserved diatom assemblage may be regarded as a reliable reflection of the diatom communities and their productivity at the time of sedimentation (CitationBattarbee, 2000).
The majority of the diatom studies have so far focused on quantitative reconstruction of the element concentrations in the lake water, such as phosphorus, of the salinity or pH (as reviewed in CitationSmol and Cumming, 2000). More recently, relationships between diatom assemblages and both water and air temperatures have been established by comparing the diatoms indentified to the respective modern climate data in order to determine the autecological limits of taxa within assemblages. Using canonical community ordinations, CitationPienitz et al. (1995), CitationWeckström et al. (1997a, Citation1997b), CitationLotter et al. (1997), CitationKorhola et al. (2000), and CitationRosén et al. (2000), among others, have demonstrated that there is a statistically significant relationship between summer surface-water or air temperatures and diatom assemblages in lakes distributed along distinct climatic gradients in Canada, the central European Alps, and Fennoscandia. They have developed calibration models which should allow reconstruction of water or air temperatures with an accuracy of approximately 0.8 to 2.0°C (for more details of these models, see CitationRosén et al., 2000). Although there are distinct relationships between diatom assemblages and lake water/summer air temperatures, the applicability of these models is still questioned. As pointed out by CitationSmol and Cumming (2000) as well as by CitationAnderson (2000), the high explanatory power of temperature might be partially dependent on other climate-related variables like the duration of ice cover or water chemistry. They argue that water or air temperature captures most of the variance among all other interrelated environmental variables. The estimated temperature optima for the diatom species should, therefore, be interpreted carefully, since most of the studies include an incomplete temperature gradient as well as only a small number of sites. Consequently, CitationSmol and Cumming (2000) made the recommendation to infer paleoclimatic trends from the diatom data, rather than to reconstruct absolute temperatures. Ideally, one should evaluate the reconstructed values using other independent indicators to reinforce the results, such as pollen-derived calibrated temperatures.
The empirical relationship between diatom assemblages and temperature—as well as other environmental variables—is established by using “transfer function” methodologies. Most often, the transfer function is based on nonlinear regression methods. In paleolimnological applications, weighted averaging (WA) and WA partial least squares (WA-PLS) regression (the latter also accounts for the residual species structure) have been used frequently (e.g., Citationter Braak and Juggins, 1993; CitationBirks, 1998), although Bayesian methods have recently also been introduced with some innovative aspects (CitationVasko et al., 2000; CitationToivonen et al., 2001; CitationKumke et al., 2004). The WA and WA-PLS methods provide simple and reliable tools to obtain quantitative past estimates for several variables derived from aquatic organisms. However, to obtain reliable parameter estimates in WA and WA-PLS, the species response along a gradient should follow a Gaussian distribution, which is ecologically sound, but not necessarily true (CitationHuisman et al., 1993; CitationAustin et al., 1994). In such cases, Bayesian methods are more flexible.
There are few examples of calibration datasets involving diatoms for central and northern Siberia. Several reasons exist for the insufficient amount of modern calibration datasets in this part of the continent, but the most important is caused by logistical constraints. The vast size of Siberia also limits the spatial applicability of the existing datasets to a certain extent. Most of the work done so far is related to the program PACT (Paleoecological Analysis of Circumpolar Treeline) and resulted in the generation of three regional calibration datasets of about 25 to 30 lakes each (CitationDuff et al., 1999; CitationLaing et al., 1999; CitationPorinchu and Cwynar, 2000). According to CitationDuff et al. (1999), the major gradients represented by their lakes were related to the ionic composition of the lake water. Regional differences existed in the water chemistry of the three datasets as a result of the regional geology and hydrology. Moreover, CitationLaing and Smol (2000) investigated the pooled regional datasets and showed that water temperatures explained a significant amount of the variation in diatom taxonomy. CitationPorinchu and Cwynar (2002) used chironomids for inferring qualitatively paleoclimate during the Holocene from a lake sediment core near the Lower Lena river valley, northeastern Siberia. More recently, CitationLaing and Smol (2003) interpreted climate and environmental changes from a lake sediment core during the last 5000 yr at Taymyr Peninsula, central Siberia, using diatom assemblages. Whereas all the studies mentioned were related to PACT, there are also a few paleolimnological studies in the European part of arctic Russia, tracking the pollution history of these areas (e.g., CitationSolovieva and Jones, 2002; CitationSolovieva et al., 2002; CitationIlyashuk et al., 2003).
In the present study, we used a calibration dataset from northwestern Finnish Lapland (CitationKorhola et al., 2000) to reconstruct paleotemperatures using diatom assemblages from Lake Lama. To the best of our knowledge, this study is the first quantitative application of a temperature model to aquatic organisms in Siberia. The specific aims are (1) to obtain estimates of July air temperatures during the Holocene as derived from diatoms, (2) to interpret and discuss the variability of the diatom assemblages as recorded in a lake sediment core, and (3) to evaluate this reconstruction by comparison with other regional paleoclimatic reconstructions.
Study Area
Lake Lama (69°33′N, 90°13′E) is located at the northwestern foothills of the Putoran Plateau, near the city of Norilsk, central Siberia. The lake is of tectonic origin, has an approximate size of about 80 km × 13 km and is up to 254 m deep. Lake Lama consists of an eastern and a western basin, which are connected by a north-south trending sub-basin (see ). More than 10 large rivers enter Lake Lama, the largest being the Mikchanda river forming a delta on its northwestern shore. The lake drains towards west via Lake Melkoye into Lake Pyasino. Lake Lama is surrounded by steeply sloped mountains to the north and south, which reach altitudes up to 1234 m a.s.l. The inundated shoreline area reaches up to 10 m in width and is covered with pebbles, gravel, and some sand due to high annual lake-level fluctuations. The geology of the catchment area is described in CitationLightfoot et al. (1990). The present mean July air temperatures and the annual precipitation vary between 10 to 15°C and 300 to 500 mm, respectively. Other main climatological and hydrological characteristics are given in CitationKienel and Kumke (2002). The forest-tundra vegetation in the embankment zone is dense and dominated by taiga trees (Betula nana, Larix, and less Picea) and shrubs (e.g., CitationKienel, 1999). Alnus fruticosa and Salix dominate the brushwood (shrubs). The larch treeline occurs at an altitude between 200 and 400 m a.s.l. Peat formation (hummocky tundra) is found in depressions and on beach terraces.
Methods
SAMPLING AND LABORATORY ANALYSES
In 1993, a lake sediment core (PG1111) with a length of 10.6 m was taken from the western basin of Lake Lama applying gravity and piston coring for the upper and lower parts of the sediment, respectively (see ). The core was subsampled for diatoms at 10-cm intervals. Diatoms were found from the top of the core to a depth of 7.0 m. Additional details of all sampling work related to this sediment core are described in CitationHarwart et al. (1999). Diatom analysis was carried out on freeze-dried subsamples of 0.5 g sediment. The calcareous and organic components were removed by boiling with HCl (10%) and H2O2 (30%). After termination of the reaction, 5 ml HNO3 (concentrated) was added and the sample was left at 120°C for 2 h. Repeated washing with distilled water (up to 10 times) removed finer particles and residual acid. The samples were allowed to settle down for 24 h between washing. Preparation of slides was carried out with 0.4 μl aliquots using the evaporation tray method (CitationBattarbee, 1973). For mounting permanent slides, the medium MOUNTEX (MERCK, RI = 1.67) was used. Diatoms were identified using a ZEISS Axioplan microscope (equipped with differential interference contrast) at 1000× magnification. The counts included 400 to 500 valves per sample with exception of four diatom-poor samples where at least 100 valves were counted. Reference texts used for the diatom taxonomy are given in CitationKienel (1999) and CitationKienel and Kumke (2002).
A reliable age chronology of the sediment core was achieved by obtaining Accelerator Mass Spectrometry (AMS) radiocarbon ages on macrofossils from 24 samples. The deepest core sample for dating had a mean depth of 8.19 m and a radiocarbon age of 11,100 ± 610 yr BP (). All radiocarbon ages were calibrated using the program CALIB 4.1 which is based on the INTCAL98 calibration curve (CitationStuiver et al., 1998).
STATISTICAL ANALYSES AND CALIBRATION MODEL
In order to obtain local diatom assemblage zones (LDAZ) from the fossil assemblages, constrained cluster analysis using a constrained optimal sum of squares partitioning algorithm with untransformed species percentage data (CitationBirks and Gordon, 1985) was carried out. The number of statistically significant zones was calculated using the broken-stick model and the associated approach described in CitationBennett (1996).
To establish an age-depth relationship, a squared polynomial regression model was fitted to 24 radiocarbon ages. The regression model fits quite well to the radiocarbon ages (R 2 = 0.75), though cross-validation yielded an error of about 400 yr.
Rates of change (RC) in the fossil diatom composition per unit time were determined by calculating the chord distance between adjacent objects. The coefficients were standardized to 100 yr. The chord distance was used because of its statistical properties concerning the weighting of species with low percentages (CitationOverpeck et al., 1985).
The transfer function applied for the paleotemperature reconstruction was derived using the northern Fennoscandian calibration dataset described in CitationKorhola et al. (2000). Only the main elements of this dataset and associated calibration function used are described here. The dataset includes 38 small (i.e. 1 to 115 ha) subarctic lakes distributed along a steep climatic gradient in northern Fennoscandia and 324 diatom taxa. The majority of the lakes is shallow (i.e. 1 to 25 m) with a simple bathymetry, nonstratified, oligotrophic, have low electrical conductivities, are slightly acidic to circumneutral, and range from clearwater to polyhumic lakes (e.g., CitationWeckström et al., 1997a). The geographic location of the lakes spans from the spruce forest to the tundra zone (CitationKorhola et al., 2000). The ice-free period of the lakes generally ranges from May or June until September. Mean July air temperatures were estimated for each lake using 1961–1990 Climate Normals data from 11 nearby climate stations. After applying consistent regional lapse rates and a linear interpolation procedure, this yielded a July air temperature gradient of 7.9 to 14.9°C for the dataset (CitationOlander et al., 1999). A more detailed description of the Fennoscandian lakes used in this study is given in CitationWeckström (2001). The transfer function between diatoms and mean July air temperature was generated using a two-component WA-PLS model. The transfer function yielded a coefficient of determination of 0.78, a root mean square error of prediction (RMSEP) of 0.89°C, and a maximum bias of 0.64°C between the calculated and the diatom-predicted temperatures (all values are based on cross-validation statistics). To give a visual impression of the performance of the transfer function, the results of the WA-PLS model are shown in together with the respective residual data.
In order to evaluate the reconstructed paleotemperatures, a principal components analysis (PCA) was performed with the fossil species from each sample of the sediment core. The PCA is basically an eigenanalysis from which eigenvalues λk and eigenvectors u k are derived. The eigenvectors corresponding to the largest λ represent most of the variance in the species. The position of the objects f k (i.e., the sample scores) at each principal axis k are a linear combination of the centered values for each species and u k. The values of f k were scaled to the square root of (n − 1)λk. To reduce the skewness, the species were log-transformed prior to the ordination. The sample scores of the first few PC axes were compared to the reconstructed temperatures, because the variability of f k is, at least theoretically, determined by the first few ecological gradients of the species. Ideally, f k should be dominated mainly by the variable being reconstructed.
In addition, an analysis of analogs between the samples of the calibration dataset and the diatoms from core samples was carried out. The analog analysis involves the computation of dissimilarities between the assemblages of the calibration data and the core data. We used the chord distance as a distance coefficient. The calculation of modern analogs was carried out using the software ANALOG (Birks and Line, unpublished program) and paleotemperatures were calculated with the software CALIBRATE (CitationJuggins and ter Braak, 1997). The PCA and the cluster analysis were performed using the programs CANOCO 4.0 (Citationter Braak and Smilauer, 1998) and ZONE (CitationLotter and Juggins, 1991), respectively.
Results
DIATOM DISTRIBUTION
We identified 184 diatom taxa from 74 samples in the core PG1111, 77 of them having a maximum relative abundance <1%. In , the most common diatom species are presented in relation to four local diatom assemblage zones (LDAZ) that are interpreted as statistically significant and chronologically clustered. LDAZ II was further subdivided into IIa and IIb due to some major taxa changes, for example in Cyclotella comensis Grunow in Van Heurck 1882 and C. ocellata Pantocsek 1901.
The species chosen in contributed more than 35% to the first two principal axes of the PCA, shown in . For example, Aulacoseira subarctica (O. Müller) Haworth 1988 was abundant in many samples associated with LDAZ I, IIa, and IIb with a considerable variability. Towards the end of LDAZ IIb, the proportions of A. subarctica decreased strongly; this species occurred in very low frequencies during the middle and late Holocene. LDAZ I is characterized by the highest abundance of Stephanodiscus medius Håkansson 1986 and high percentages of C. rossii Håkansson 1990, Nitzschia angustata var. angustata (W. Smith) Grunow in Cleve and Grunow 1880, and N. lacuum Lange-Bertalot 1980. The latter two species were most abundant during LDAZ I and decreased to an approximately steady level during LDAZ IIa. That is also evident from the PCA, where N. angustata var. angustata and N. lacuum are closely associated with the sample depths of LDAZs I and IIa (). The same holds true for C. rossii with the exception that the species reached its highest abundance at approximately 6500 cal. yr BP and had high abundance during LDAZ IV. The distribution of C. ocellata is characterized by its almost exclusive occurrence up to 20% during LDAZ IIb. The planktonic species C. comensis and C. radiosa (Grunow) Lemmermann 1900 had their highest frequencies during the first half of LDAZ III. In particular, C. radiosa occurred with up to 10% during LDAZ III, whereas its abundance during other zones was either low or the species was absent. The taxon C. comensis was present throughout the sediment sequence except for the lowest two samples in LDAZ I. Both C. comensis and C. radiosa have their greatest fit to the second PCA axis, but also contribute to the first and to the third axes (). The Shannon diversity index H′ (CitationShannon and Weaver, 1949) was highly variable during the lower part of the core () with maximum values (H′ ≈ 3.72) occurring in LDAZ I and two minimum peaks (H′ ≈ 2.45) between 9000 and 8000 cal. yr BP. The Shannon diversity index increased from about 6500 cal. yr BP towards generally large values between H′ = 3.3 and H′ = 3.7 during the late Holocene (LDAZ IV).
The sample scores of PC axis 1 () decrease more or less steadily from their highest values at the beginning of the Holocene until 7000 cal. yr BP, where they receive the lowest scores. At this time, the sample scores are mainly determined by the increased abundance of C. comensis and Fragilaria pinnata var. pinnata Ehrenberg 1843. The following rise of the sample scores is mainly caused by the peak of C. rossii. The positive sample scores of PC axis 2 are associated with the large abundances of C. comensis and C. radiosa. During LDAZ IIb, the scores of PC axis 2 have their highest values due to the occurrence of C. ocellata which fits very well into that direction of species space.
The rates of change in species composition () have similar patterns as the sample scores of PC axis 1 in particular during the early and middle Holocene. The rates of compositional turnover were relatively high during LDAZs I and II. However, the most distinctive RCs occurred at the transition between LDAZs IIa and IIb as well as at approximately 6400 cal. yr BP. Species that affect the rates of change during this time are those negatively associated with the first principal axis, for example C. comensis, F. pinnata var. pinnata, and Hippodonta costulata (Grunow in Cleve & Grunow) Lange-Bertalot, Metzeltin and Witkowski 1996. The sample scores of PC axis 1 had their minimum values at 6400 cal. yr BP. The RC peak at the LDAZ IIa/IIb boundary is mainly caused by species which covary positively and strongly with PC axis 1 and less strongly with PC axis 2. These include particularly A. subarctica and C. schumannii (Grunow) Håkansson 1990. However, since the RC calculation is based on the dissimilarities of all species between adjacent samples, the species listed comprise only some representatives of the changes in the diatom assemblages.
INFERRED JULY AIR PALEOTEMPERATURES
The reconstructed July air temperatures using the WA-PLS 2-component model range from 10.2 to 11.6°C during the Holocene with a mean of 11.0°C. The deviations from the mean () indicate lower temperatures during LDAZs I and IIa, which corresponds roughly to the latest part of the Younger Dryas given the error of the age model of approximately 400 yr. At the beginning of LDAZ IIb, there is a distinct and abrupt increase in temperature of about 1.2°C which occurs simultaneously with the large peak in the RC values. From LDAZ IIb to the middle of LDAZ III, the majority of the inferred temperatures have positive deviations from the mean with some peaks up to 0.5°C. The most striking changes were reconstructed at about 6400 and 4500 cal. yr BP, where the inferred temperatures decrease. The temperature decline at 6400 cal. yr BP occurs alongside with a major change in the diatom composition as indicated by the RC value. During the period from about 4000 cal. yr BP to 2800 cal. yr BP, the inferred temperatures are constantly above the average.
In order to examine the reconstruction results in terms of the species fit, modern analogs were calculated for the core data. The second and the fifth percentile of the modern analog distribution were used to determine close and poor analogy of the core diatom assemblages, respectively (CitationBirks et al., 1990). Using these thresholds, the diatom assemblages of the sediment core at Lake Lama have no or poor analogies with the assemblages of the calibration dataset. This result, however, only indicates that there are differences between the diatom assemblages in the lakes of the calibration dataset and the past assemblages in the sediment core. It does not assess the goodness of the prediction since the modern analog approach is not based on any species response model. The lack of analogs does not affect the reconstruction when using the weighted averaging techniques as long as there are sufficient species with large frequencies in the fossil dataset for which the temperature optima can be estimated from the calibration dataset (CitationBirks, 1998). lists those taxa identified from the core samples, which also occurred in at least 20% of the lakes sampled for the modern calibration dataset; their effective number of occurrence N2 (CitationHill, 1973) and maximum abundance N are included. The data in represent the overlap between fossil and calibration dataset with the constraint that the taxa are abundant in the calibration dataset in at least eight lakes. Some of the taxa, for example, A. subarctica, C. comensis, or C. schumannii, are frequently abundant but in less than 20% of the lakes. From the 184 species recorded in the fossil data, 92 were also present in the calibration dataset. Almost 50% of the remaining 92 species were represented in less than 1% abundance. Of the important species in terms of high N2 values and species variation, identified from the core PG1111, only C. ocellata and Stephanodiscus medius are absent from the calibration dataset. However, both species, especially C. ocellata, rather contribute to the second PC axis, suggesting that they might be more related to secondary gradients. As C. ocellata and S. medius have enhanced phosphorus requirements (e.g., European Diatom Database at http://craticula.ncl.ac.uk:8000/Eddi) the second PC axis may be associated with nutrient availability. The first PC axis, which is the major hypothetical gradient in PCA, is significantly correlated with the inferred temperatures (r = −0.38, P ≤ 0.05). The higher PC axes were not correlated with the inferred temperatures; therefore secondary gradients cannot be explained by inferred temperature changes.
Discussion
To date, our reconstruction is the first attempt to infer quantitatively paleotemperatures using aquatic organisms in Siberia. This temperature reconstruction is justified by the changes of especially those diatom taxa with temperature preferences. The indirect gradient analysis of the fossil assemblages (i.e. the PCA) has shown, that the major part of the variability in the diatom assemblages is mainly driven by temperature-indicative taxa. This is supported by the significant correlation of the principal components of the first major axis with the reconstructed temperatures. However, there are a few temperature reconstructions available from pollen in central Siberia which may be used for comparison. CitationTarasov et al. (1999) presented some quantitative paleoclimate reconstructions using plant functional types (PFT) for a time slice of 6000 14C yr BP at several sites in northern Eurasia. Their reconstructions indicated up to 2°C higher temperatures at 6000 14C yr BP for a region southwest of Taymyr Peninsula although their results failed to be statistically significant at some of the sites. CitationTarasov et al. (1999) also found that the PFT method often yields rather large error bars.
Our results show the highest positive July temperature deviations between approximately 8000 and 6500 cal. yr BP as well as between 4000 and 2800 cal. yr BP. The diatom-reconstructed temperature deviations are generally less pronounced than the pollen-based paleotemperature changes inferred by CitationTarasov et al. (1999). The results of CitationTarasov et al. (1999) and especially pollen-based reconstructions derived from CitationAndreev and Klimanov (2000) suggest that the so-called Holocene thermal optimum (Hypsithermal) occurred regionally during quite different times in northern Russia. For example, the sites studied by CitationAndreev and Klimanov (2000) have prolonged intervals of positive paleotemperature deviations during the Atlantic chronozone (between 8000 and 4500 14C yr BP). The qualitative inferences of CitationPorinchu and Cwynar (2002) for northeastern Siberia, on the other hand, suggest a Holocene warming period between 10000 and 6400 14C yr BP based on the increased occurrences of warm water chironomid taxa.
The quantitative paleotemperature reconstruction of CitationAndreev et al. (2004) derived from the pollen record of the sediment core PG1111 at Lake Lama has similar variations as our diatom-based reconstruction. Comparisons of the pollen spectra and the diatom assemblages were carried out based on Mantel tests (see CitationLegendre and Legendre, 1998) and the results of the RC analyses. The standardized Mantel test revealed a significant correlation (r = 0.51, P ≤ 0.05) between the dissimilarity matrices of the pollen spectra and the diatom assemblages using the chord distance. A correlation of the RC results of both records (not presented in CitationAndreev et al., 2004) was estimated with r = 0.88 at P ≤ 0.05 using the chord distance. These results indicate that variations in the fossil diatom assemblages and the pollen spectra took place synchronously and in a similar magnitude. The diatom-inferred July temperatures are significantly correlated (r = 0.25, P ≤ 0.05) with the pollen-reconstructed July temperatures (CitationAndreev et al., 2004). Although the temperature correlation is distinctly weaker than the correlations of the respective RCs or the dissimilarity structures, the main trends are similar in both reconstructions. For example, the negative temperature deviations associated with the Younger Dryas (i.e., the inferred temperatures during LDAZ I and IIa) as well as the first Holocene warming (inferred for the beginning of zone IIb) were similarly interpreted by both diatoms and pollen. CitationAndreev et al. (2004) were not able to distinguish a period of maximum warming from their reconstruction, but they assume a thermal optimum period between 8000 and 4500 14C yr BP based on their temperature curve. Similarly, from our diatom reconstruction we might emphasize a Holocene thermal maximum between 8000 and 6500 cal. yr BP, but there is no consistent positive temperature deviation over a longer time period. Reconstructed temperature maxima for Lake Lama occurred at about 8000, 6500, 4000, and about 3200 cal. yr BP. A smoothed paleotemperature curve shows the most pronounced positive temperature deviations over a long time period to occur between 4000 and 2800 cal. yr BP (). The pollen-based temperatures were also higher than present during this period (CitationAndreev et al., 2004).
The overall range of our reconstructed temperatures is rather low compared to that of the pollen reconstructions of Lake Lama. The temperature range reconstructed by CitationAndreev et al. (2004) is up to 6°C for the Younger Dryas and Holocene part of their pollen record whereas the maximum range of our diatom-based reconstruction is 1.6°C. In addition, the mean of the pollen-based temperatures is 1.7°C higher than that of the diatom-based temperatures. The reasons for these differences may be twofold. First, changes in water temperature (which actually affect diatoms) might have been smaller than in air temperature due to the large water body of Lake Lama compared to the smaller lakes in the calibration dataset. The response of the diatom assemblages would, therefore, have been comparatively moderate. Near shore, the response of water temperature to changes in air temperature can be expected to be stronger. The reflection in the diatom assemblages there would pertain mainly to periphytic species. Because the periphytic diatoms occur with many species with low frequencies in the fossil assemblages, their influence on the reconstructed temperatures is low. Second, the cross-validation of the transfer function showed that the predicted temperatures below the mean overestimated the observed temperatures and the predicted temperatures above the mean underestimated the observed values (see ). This resulted in a predicted temperature range of 5.1°C compared to 7.0°C of the observed temperature range. Thus, the range of the model predictions is likely to be too small when applied to fossil data which is a common problem in inverse approaches and hence in WA-PLS (CitationBirks, 1995; CitationKorhola et al., 2000; CitationVasko et al., 2000). This would largely explain the discrepancy between the considerably distinct RCs (e.g., at the LDAZ IIa/IIb boundary and at 6400 cal. yr BP) and the rather small inferred temperature changes. The inferred mean July paleotemperature range (about 1.3°C) of the reconstruction by CitationKorhola et al. (2000) in the Lake Tsuolbmajavri, Finnish Lapland, using the same transfer function support this interpretation. This problem could possibly be avoided in future studies by the application of reconstruction methods that are based on classical calibration (see CitationVasko et al., 2000).
The calibration dataset per se used in this paleoclimatic reconstruction is sufficient and reliable for several reasons. The temperature gradient in the modern dataset exceeds the range of the present mean July temperatures (10 to 15°C) of the region around Norilsk. Present throughout the lake sediment core are many diatom species also represented in the calibration dataset. These taxa are indicative of temperature, i.e., species whose estimated optima and tolerances are within the range of the temperatures of the dataset and whose variance is largely captured by temperature. Most of the taxa also contribute to the first PC axis (), including C. rossii, F. pinnata var. pinnata, and N. angustata var. angustata. Only A. subarctica was present in less than 20% of the lakes of the calibration dataset and S. medius was even absent. The estimated temperature optima of the species attributed to the first PC axis agree well with the paleotemperatures. For example, C. rossii and N. angustata var. angustata have optima distinctly less than the average (i.e., u = 10.0 and 8.7°C, respectively) in the modern training dataset and the optimum of F. pinnata var. pinnata was estimated to be slightly above the average temperature of 11.1°C (u = 11.6°C). The relatively high optimum value for the latter species, compared to others in this particular calibration dataset, may be somewhat surprising, since F. pinnata is regarded as a rather cold water taxon and typical for tundra lakes (e.g., CitationRosén et al., 2000; CitationLaing and Smol, 2003). In addition, earlier analyses with a Fennoscandian dataset, in which 17 sites are the same as in the current one, indicated that the abundance of that species is more frequent at the lower end of the temperature gradient (CitationWeckström et al., 1997b). This disparity may be attributed to some degree to the method of sampling for the calibration datasets: surface samples are taken from the deepest point of the lake where in most of the cases planktonic species dominate the diatom assemblage. Consequently, tychoplanktonic or periphytic species, such as F. pinnata are underrepresented leading to less reliable optima of environmental variables. Regardless of the low representation of Aulacoseira subarctica in the calibration dataset, its association with lower temperatures in the reconstruction is confirmed by results of autecological studies. The nordic-alpine species (CitationKrammer and Lange-Bertalot, 1991) is favored by low-light conditions, turbulent waters and low temperatures (e.g., CitationLund, 1971; CitationInterlandi and Kilham, 1999).
The reconstruction may have some disadvantages. First, lake sizes and bathymetries of the 38 lakes of the calibration dataset are very different from that of Lake Lama. This may have caused some differences in the species composition resulting in a lack of close analogy. The most noticeable differences are the higher number and partly larger proportions of periphytic species in the calibration dataset compared to PG1111. However, as discussed above, the species composition of modern and fossil dataset is comparable especially concerning the species whose occurrence is largely determined by the climatic gradient. A second disadvantage is the lack of regional calibration datasets in arctic Russia. The only available modern dataset from this region (CitationLaing and Smol, 2000) suffers from a lack of comparable diatom species apart from the subset containing the Taymyr lakes. As a consequence, this dataset was regarded as not appropriate for establishing an applicable inference model for Lake Lama. However, the alkalinity reconstruction of CitationLaing et al. (1999) indicated that the use of transfer functions from other regions is justified as long as species compositions and environmental gradients (water chemistry in their case) are comparable in both datasets. This assumption held true after we tested our Lake Lama dataset against the Fennoscandian dataset. Third, we cannot neglect the comments of CitationSmol and Cumming (2000) on the validity of reconstructing paleotemperatures using diatoms, because of the influence of other environmental variables. In the case of Lake Lama, the inferred paleotemperatures largely reflect the changes in the diatom compositional changes of the core PG1111. If the results are compared to the results of the pollen-based reconstruction, both the species compositions of diatoms and pollen as well as the inferred temperatures follow the same general patterns. Thus, the inferred Holocene temperature trends seem to be more reliable than their absolute values.
Conclusions
Diatom assemblages from Lake Lama, central Siberia, showed distinct responses to climatic changes during the Holocene. This is evident from indirect gradient analysis in which the major part of the variation of diatom taxa is related to species indicative to temperature changes. Using a transfer function derived from diatoms and July air temperatures of 38 lakes in northwestern Finnish Lapland (CitationKorhola et al., 2000), paleotemperatures were inferred from a lake sediment core of Lake Lama. The reconstructed temperatures were strongly correlated with the diatom assemblage changes indicating that these changes can be explained largely by the established diatom-temperature relationship. Moreover, the inferred paleotemperatures were correlated with the first principal axis of the fossil diatom distribution, thus demonstrating that most of the variation in the diatoms can be explained by the reconstructed temperatures. For example, the PCA biplot analysis has shown that the occurrence of A. subarctica, C. rossii, and N. angustata var. angustata are attributed to cooler climatic conditions, whereas F. pinnata var. pinnata may be related to increased paleotemperatures. Most of the taxa in the fossil dataset contributing to the major direction of variation were also present in at least 20% of the lakes within the calibration dataset. From this it can be concluded that the application of the Fennoscandian transfer function to the diatom assemblages of a central Siberian lake is justified. Further support for the reconstructed temperature trends come from an independent pollen-based paleotemperature reconstruction of the same sediment core from Lake Lama (CitationAndreev et al., 2004). A comparison between both reconstructions has shown that the major patterns in the variation of paleotemperatures are similar. For example, the diatom-based temperatures increase distinctly at the beginning of the early Holocene. Further, there are positive temperature deviations between ca. 8000 and 6500 cal. yr BP as well as between ca. 4000 and 2800 cal. yr BP. The period between 8000 and 6500 cal. yr BP might be attributed to the Holocene thermal optimum period, although the positive temperature deviations are not well pronounced. The pollen-based reconstructions support this hypothesis.
Our study of a sediment core from Lake Lama provides the first paleotemperature reconstruction for arctic Russia based on aquatic organisms. The results obtained imply that the use of diatoms is an alternative and an important extension to the few existing approaches suitable to studying the paleoclimate of this large area. However, our approach also shows that although the application of the Fennoscandian transfer function worked quite well for Lake Lama, there is a need to develop regional calibration datasets. Such datasets should preferably consist of a variety of paleolimnological indicators in order to circumvent the problems outlined in this paper.
FIGURE 1. Bathymetric map of Lake Lama, central Siberia, and the coring location of PG1111. The isolines refer to the water depths (m). The inset shows the Taymyr Peninsula with the Norilsk region in the south and the associated vegetation zones
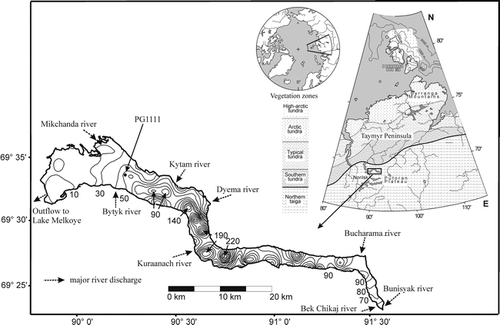
FIGURE 2. Relationship between observed and diatom-inferred mean July air temperature for Fennoscandia using a two-component weighted averaging partial least squares (WA-PLS) model with leave-one-out cross-validation (CitationKorhola et al., 2000)
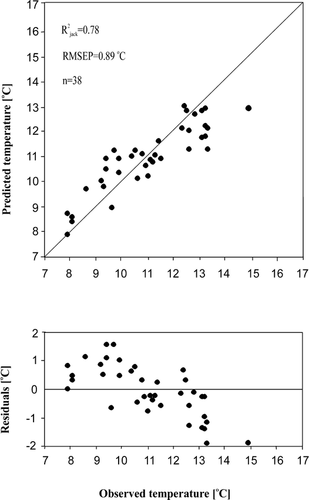
FIGURE 3. Distribution of selected diatom taxa in the sediment core PG1111, Lake Lama. The species chosen fit at least 35% into the major plane of species space. Note that the percentage scale for each taxon is different for the sake of better visibility. The roman numerals are the local diatom assemblage zones (LDAZ) as estimated by constrained cluster analysis. The arrows indicate those samples with a lower number of valves counted
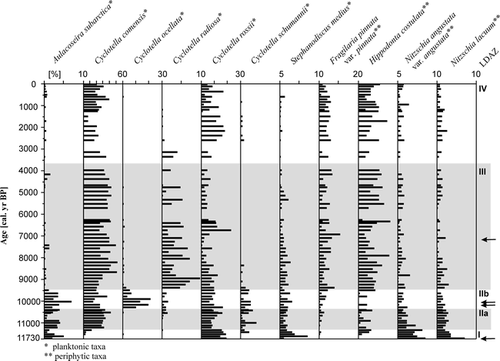
FIGURE 4. Distance biplot of the PCA for PG1111. The objects and species plotted fit at least 20% and 35% into species space, respectively
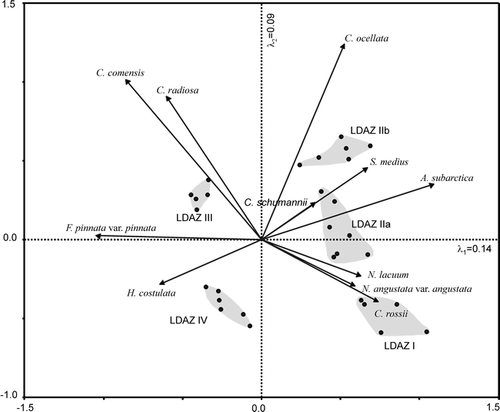
FIGURE 5. Object scores of the first two principal axes, the Shannon diversity index, estimated rates of change (RC), and inferred July air temperatures (°C) derived from the diatom assemblages of core PG1111. The inferred July air temperatures are given as deviations from the mean (points) and as their three-point moving average (solid line)
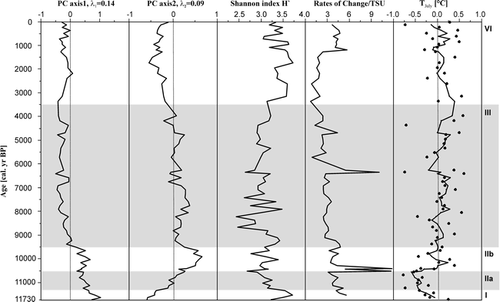
TABLE 1 AMS radiocarbon dates from the sediment samples of Lake Lama, Central Siberia (modified after CitationAndreev et al., 2004)
TABLE 2 Summary statistics of the species occurrences N and the effective species number N2 in the calibration dataset and the fossil data set for those species which occurred in at least 20% (N = 8) of the lakes in the calibration dataset
TABLE 2 (Cont.)
Acknowledgments
This is a contribution to the project “Natural climate variations from 10,000 years to the present day” (KIHZ), a joint effort of geoscientists and modelers from the German National Research Centers and Universities to analyze the dynamics of natural climate variability. KIHZ is funded by the Federal Ministry of Education and Research (BMBF). Additional financial support was achieved from the Academy of Finland. We thank the participants of the expedition Taymyr 93 from the Alfred-Wegener-Institute for Polar and Marine Research, Potsdam, for providing the sample material and all members of the CHILL-10,000 program who contributed to the preparation of the calibration dataset. We also would like to thank N. Hultzsch, C. Yansa, J. P. Smol, and an anonymous reviewer for their helpful comments on earlier drafts of the manuscript.
References Cited
- Anderson, N. J. 2000. Diatoms, temperature and climatic change. European Journal of Phycology 35:307–314.
- Andreev, A. A. and V. A. Klimanov . 2000. Quantitative Holocene climatic reconstruction from Arctic Russia. Journal of Paleolimnology 24:81–91.
- Andreev, A. A. , P. E. Tarasov , V. A. Klimanov , M. Melles , O. M. Lisitsina , and H-W. Hubberten . 2004. Vegetation and climate changes around the Lama Lake, Taymyr Peninsula during the Late Pleistocene and Holocene reconstructed from pollen records. Quaternary International 122:69–84.
- Austin, M. P. , A. O. Nicholls , M. D. Doherty , and J. A. Meyers . 1994. Determining species response functions to an environmental gradient by means of a ß-function. Journal of Vegetation Science 5:214–228.
- Barker, P. , N. Roberts , H. Lamb , S. van der Kaars , and A. Benkaddour . 1994. Interpretation of Holocene lake-level change from diatom assemblages in Lake Sidi Ali, Middle Atlas, Morocco. Journal of Paleolimnology 12:223–234.
- Battarbee, R. 1973. A new method for the estimation of absolute microfossil numbers, with reference especially to diatoms. Limnology and Oceanography 18:647–653.
- Battarbee, R. 2000. Paleolimnological approaches to climate change, with special regard to the biological record. Quaternary Science Reviews 19:107–124.
- Bennett, K. D. 1996. Determination of the number of zones in a biostratigraphical sequence. New Phytologist 132:155–170.
- Birks, H. J B. 1995. Quantitative palaeoenvironmental reconstructions. In Maddy, D. and Brew, J. S. (eds.), Statistical Modelling of Quaternary Science Data, Technical Guide. 5th ed. Cambridge: Quaternary Science Association, 161–254.
- Birks, H. J B. 1998. Numerical tools in palaeolimnology—Progress, potentialities, and problems. Journal of Paleolimnology 20:307–332.
- Birks, H. J B. and A. Gordon . 1985. Numerical Methods in Quaternary Pollen Analysis. London: Academic Press. 317 pp.
- Birks, H. J B. , J. Line , S. Juggins , A. Stevenson , and C. J F. ter Braak . 1990. Diatoms and pH reconstruction. Philosophical Transactions of the Royal Society of London B 327:263–278.
- Briffa, R. , P. Jones , and F. Schweingruber . 1992. Tree-ring density reconstructions of summer temperature patterns across western North America since 1600. Journal of Climate 5:735–754.
- Brooks, S. and H. J B. Birks . 2000. Chironomid-inferred late-glacial and early-Holocene mean July air temperatures for Kråkenes Lake, western Norway. Journal of Paleolimnology 23:77–89.
- Cheddadi, R. , G. Yu , J. Guiot , S. Harrison , and I. C. Prentice . 1997. The climate of Europe 6000 years ago. Climate Dynamics 13:1–9.
- Duff, K. , T. E. Laing , J. P. Smol , and D. Lean . 1999. Limnological characteristics of lakes located across arctic treeline in northern Russia. Hydrobiologia 391:205–222.
- Hall, R. I. , P. R. Leavitt , J. P. Smol , and N. Zirbhelt . 1997. Comparison of diatoms, fossil pigments and historical records as measures of lake eutrophication. Freshwater Biology 38:401–417.
- Harwart, S. , B. Hagedorn , M. Melles , and U. Wand . 1999. Lithological and biochemical properties in sediments of Lama Lake as indicators for the late Pleistocene and Holocene ecosystem development of the southern Taymyr Peninsula, Central Siberia. Boreas 28:167–180.
- Hill, M. 1973. Diversity and evenness: a unifying notation and its consequences. Ecology 54:427–432.
- Huisman, J. , H. Olff , and L. F M. Fresno . 1993. A hierarchical set of models for species response analysis. Journal of Vegetation Science 4:37–46.
- Ilyashuk, B. , E. Ilyashuk , and V. Dauvalter . 2003. Chironomid responses to long-term metal contamination: a paleolimnological study in two bays of Lake Imandra, Kola Peninsula, northern Russia. Journal of Paleolimnology 30:217–230.
- Interlandi, S. J. and S. S. Kilham . 1999. Responses of phytoplankton to varied resource availability in large lakes of the Greater Yellowstone Ecosystem. Limnology and Oceanography 44:668–682.
- Jouzel, J. , C. Waelbroeck , B. Malaize , M. Bender , J. Petit , M. Stievenard , N. Barkov , J. Barnola , T. King , V. Kotiyakov , V. Lipenkov , C. Lorius , D. Raynaud , C. Ritz , and T. Sowers . 1996. Climatic interpretation of the recently extended Vostok ice records. Climate Dynamics 12:513–521.
- Juggins, S. and C. J F. ter Braak . 1997. CALIBRATE—a program for species-environment calibration by [weighted averaging] partial least squares regression (0.81). University of Newcastle.
- Kienel, U. 1999. Late Weichselian to Holocene diatom successions in a sediment core from Lama Lake, Siberia and presumed ecological implications. In Kassens, H., Bauch, H., Dmitrenko, I., Eicken, H., Hubberten, H.-W., Melles, M., Thiede, J., and Timokhov, L. (eds.), Land-Ocean Systems in the Siberian Arctic: Dynamics and History. Berlin: Springer-Verlag, 377–405.
- Kienel, U. and T. Kumke . 2002. Combining ordination techniques and geostatistics to determine the patterns of diatom distributions at Lake Lama, Central Siberia. Journal of Paleolimnology 28:181–194.
- Korhola, A. , J. Weckström , L. Holmström , and P. Erästö . 2000. A quantitative Holocene climatic record from diatoms in northern Fennoscandia. Quaternary Research 54:284–294.
- Krammer, K. and H. Lange-Bertalot . 1991. Süsswasserflora von Mitteleuropa: Bacillariophycea 3. Teil: Centrales, Fragilariaceae, Eunotiaceae. Stuttgart: Fischer. 576 pp.
- Kumke, T. , C. Schölzel , and A. Hense . 2004. Transfer functions for paleoclimate reconstructions—theory and methods. In Fischer, H. Kumke, T., Lohmann, G., Flöser, G., Miller, H., von Storch, H., and Negendank, J. F. W. (eds.), The KIHZ project: Towards a Synthesis of Paleoclimate Variability Using Proxy Data and Climate Models. Berlin: Springer-Verlag, 231–246.
- Laing, T. E. , K. Rühland , and J. P. Smol . 1999. Past environmental and climatic changes related to tree-line shifts inferred from fossil diatoms from a lake near the Lena River Delta, Siberia. The Holocene 9:547–557.
- Laing, T. E. and J. P. Smol . 2000. Factors influencing diatom distributions in circumpolar treeline lakes of Northern Russia. Journal of Phycology 36:1035–1048.
- Laing, T. E. and J. P. Smol . 2003. Late Holocene environmental changes inferred from diatoms in a lake on the western Taimyr Peninsula, northern Russia. Journal of Paleolimnology 30:231–247.
- Legendre, P. and L. Legendre . 1998. Numerical Ecology. Amsterdam: Elsevier. 853 pp.
- Lightfoot, P. C. , A. J. Naldrett , N. S. Gorbachev , W. Doherty , and V. A. Fedorenko . 1990. Geochemistry of the Siberian Trap of the Noril'sk area, USSR, with implications for the relative contributions of crust and mantle to flood basalt magmatism. Contributions in Mineralogy and Petrology 104:631–644.
- Lotter, A. , H. J B. Birks , U. Eicher , W. Hofmann , J. Schwander , and L. Wick . 2000. Younger Dryas and Allerød summer temperatures at Gerzensee (Switzerland) inferred from fossil pollen and cladoceran assemblages. Palaeogeography, Palaeoclimatology, Palaeoecology 159:349–361.
- Lotter, A. F. , H. J B. Birks , W. Hofmann , and A. Marchetto . 1997. Modern diatom, cladocera, chironomid, and chrysophyte cyst assemblages as quantitative indicators for the reconstruction of past environmental conditions in the Alps. I. Climate. Journal of Paleolimnology 18:395–420.
- Lotter, A. F. and S. Juggins . 1991. PLOTPROF, TRAN and ZONE. Programs for plotting, editing and zoning of pollen and diatom data. INQUA Commission for the study of the Holocene, Working Group on Data Handling Methods, Newsletter 6.
- Lund, J. W G. 1971. An artificial alteration of the seasonal cycle of Melosira italica subsp. subarctica in an English lake. Journal of Ecology 59:521–533.
- Olander, H. , H. J B. Birks , A. Korhola , and T. Blom . 1999. An expanded calibration model for inferring lakewater and air temperatures from fossil chironomid assemblages in northern Fennoscandia. The Holocene 9:279–294.
- Overpeck, J. , T. Webb III , and I. C. Prentice . 1985. A quantitative interpretation of fossil pollen spectra: dissimilarity coefficients and the method of modern analogs. Quaternary Research 23:87–108.
- Pienitz, R. , J. P. Smol , and H. J B. Birks . 1995. Assessment of freshwater diatoms as quantitative indicators of past climatic change in the Yukon and Northwest Territories, Canada. Journal of Paleolimnology 13:21–49.
- Pienitz, R. , J. P. Smol , W. M. Last , P. R. Leavitt , and B. F. Cumming . 2000. Multi-proxy Holocene palaeoclimatic record from a saline lake in the Canadian Subarctic. The Holocene 10:673–686.
- Porinchu, D. F. and L. C. Cwynar . 2000. The Distribution of Freshwater Chironomidae (Insecta: Diptera) across Treeline near the Lower Lena River, Northeast Siberia, Russia. Arctic, Antarctic, and Alpine Resarch 32:429–437.
- Porinchu, D. F. and L. C. Cwynar . 2002. Late-Quaternary history of midge communities and climate from a tundra site near the lower Lena River, Northeast Siberia. Journal of Paleolimnology 27:59–69.
- Rosén, P. , R. I. Hall , T. Korsman , and I. Renberg . 2000. Diatom transfer functions for quantifying past air temperature, pH and total organic carbon concentration from lakes in northern Sweden. Journal of Paleolimnology 24:109–123.
- Seppä, H. and J. Weckström . 1999. Holocene vegetational and limnological changes in the Fennoscandian tree-line area as documented by pollen and diatom records from Lake Tsuolbmajavri, Finland. Ecoscience 6:621–635.
- Shannon, C. E. and W. Weaver . 1949. The Mathematical Theory of Communication. Urbana: University of Illinois Press. 117 pp.
- Smol, J. P. and B. F. Cumming . 2000. Tracking long-term changes in climate using algal indicators in lake sediments. Journal of Phycology 36:986–1011.
- Solovieva, N. and V. J. Jones . 2002. A multiproxy record of Holocene environmental changes in the central Kola Peninsula, northwest Russia. Journal of Quaternary Science 17:303–318.
- Solovieva, N. , V. J. Jones , P. G. Appleby , and B. M. Kondratenok . 2002. Extent, environmental impact and long-term trends in atmospheric contamination in the Usa basin of East-European Russian Arctic. Water, Air, and Soil Pollution 139:237–260.
- Stoermer, E. F. and J. P. Smol . (eds.),. 1999. The Diatoms: Applications for the Environmental and Earth Sciences. Cambridge: Cambridge University Press. 482 pp.
- Stuiver, M. , P. J. Reimer , E. Bard , J. W. Beck , G. S. Burr , K. A. Hughen , B. Kromer , F. G. McCormac , J. van der Plicht , and M. Spurk . 1998. INTCAL98 Radiocarbon age calibration 24,000–0 cal BP. Radiocarbon 40:1041–1083.
- Tarasov, P. , J. Guiot , R. Cheddadi , A. A. Andreev , L. Bezusko , T. Blyakharchuk , N. Dorofeyuk , L. Filimonova , V. Volkova , and V. Zernitskaya . 1999. Climate in Northern Eurasia 6000 years ago reconstructed from pollen data. Earth and Planetary Science Letters 171:635–645.
- ter Braak, C. J F. and S. Juggins . 1993. Weighted averaging partial least squares regression (WA-PLS): an improved method for reconstructing environmental variables from species assemblages. Hydrobiologia 269/270:485–502.
- ter Braak, C. J F. and P. Smilauer . 1998. CANOCO Reference Manual and User's Guide to Canoco for Windows: Software for Canonical Community Ordination (version 4). Microcomputer Power, Ithaca, New York. 352 pp.
- Toivonen, H. , H. Mannila , A. Korhola , and H. Olander . 2001. Applying Bayesian statistics to organism-based environmental reconstruction. Ecological Applications 11:618–630.
- Vasko, K. , H. Toivonen , and A. Korhola . 2000. A Bayesian multinomial Gaussian response model for organism-based environmental reconstruction. Journal of Paleolimnology 24:243–250.
- Weckström, J. , A. Korhola , and T. Blom . 1997a. Diatoms as quantitative indicators of pH and water temperature in subarctic Fennoscandian lakes. Hydrobiologia 347:171–184.
- Weckström, J. , A. Korhola , and T. Blom . 1997b. The relationship between diatoms and water temperature in thirty subarctic Fennoscandian lakes. Arctic and Alpine Research 29:75–92.
- Weckström, J. 2001. Assessment of diatoms as markers of environmental change in northern Fennoscandia. PhD thesis, University of Helsinki. 195 pp.