Abstract
An alpine fellfield community on granite substrate (elevation 3750 m) near the University of California Barcroft Laboratory in the White Mountains of eastern California was studied during the 2000 growing season to determine whether classic perennial life forms can be treated as plant functional groups. A series of 1-m2 quadrat samples were measured to determine common species. The four species with greatest cover were Penstemon heterodoxus var. heterodoxus, Trifolium andersonii var. beatleyae, Poa glauca subsp. rupicola, and Eriogonum ovalifolium var. nivale. These and four additional common perennial species were selected for ecophysiological studies representing four distinct ecological life forms: chamaephytes, cushion plants (including mat formers), herbaceous dicot perennials, and graminoid perennials. Summer midday leaf temperatures for species with foliage held close to ground surface were up to 20°C higher than air temperatures, whereas on upright species, leaves away from the ground surface closely matched ambient temperatures. For the eight species, peak values of mean maximum photosynthetic rates ranged from 11.5–25.5 μmol CO2 m−2 s−1, typical of published values, although chamaephytes in the study showed higher rates comparable to herbaceous perennials. Water-use efficiency, as estimated by a ratio of internal to ambient CO2, was relatively high (c i:c a ratios of 0.43–0.59) compared to published data. During the stressful end of the growing season, neither predawn nor midday shoot water potentials ever reached low levels, presenting conflicting evidence for the role of soil moisture as a limiting factor. Overall, the data on plant functional attributes showed no strong patterns of differences between categories of life forms in the fellfield community, suggesting that classical life forms in this habitat do not represent plant functional groups.
Introduction
The concept of plant functional groups has been widely used as an approach for predicting community responses to environmental disturbance and global climate change (CitationSmith et al., 1997; CitationLavorel et al., 1997; CitationMcIntyre et al., 1999). This concept has been applied to a variety of plant communities to assess relationships between diversity, resilience, and ecosystem function. In-depth analyses have been performed on mixed forests (CitationVanclay et al., 1997), mediterranean-climate grasslands (CitationHooper and Vitousek, 1998; CitationLavorel et al., 1999), arid savannas (CitationWalker et al., 1999; CitationMcIntyre and Lavorel, 2001; CitationWalker and Landridge, 2002), and tidal marshes (CitationSullivan and Zedler, 1999), as well as for experimental studies of small artificial ecosystems (CitationWardle et al., 2000; CitationTilman et al., 2001).
Although life forms are certainly discussed and studied in modern treatments of alpine vegetation (e.g., CitationKörner, 1999; CitationBillings, 2000), comparatively little attention has been given to these as plant functional groups. Herbaceous perennials of a variety of forms and architectures form the dominant community cover in most temperate alpine ecosystems. These herbaceous perennials include cushion plants, mats, broad-leaved rosette plants, and perennial graminoids (CitationBillings, 2000). Also present with lower species richness are chamaephytes (subshrubs) with a low form of woody growth. Other plant life forms such as taller woody phanerophytes and therophytes (annuals) are rare in most alpine habitats.
The problems of survival for alpine plants are many, suggesting that there should be strong functional traits that increase species fitness. Environmental stresses associated with temperate alpine ecosystems include extreme winter temperatures, short growing season, low nutrient availability, high winds, low partial pressures of CO2, high UV irradiance, and water availability (CitationKörner, 1999; CitationBillings, 2000; CitationBowman and Seastedt, 2001). Species dominance and life form distribution has been shown to vary across a variety of habitats along alpine moisture gradients from wet meadows to fellfields to dry meadows and rocky slopes (CitationOberbauer and Billings, 1981; CitationChapin and Körner, 1995), while carbon isotope ratios as a measure of integrated water use efficiency have been used to identify functional groups within boreal plants (CitationBrooks et al., 1997).
In our study, we focused on the alpine fellfield habitat and asked if classical plant life forms (sensu CitationRaunkiaer, 1934) operate ecologically as functional plant groups. We examined a series of morphological traits and functional attributes of eight fellfield species from alpine habitats of the White Mountains in eastern California. The functional attributes that we investigated for each species and life form were the elevational range of occurrence, root morphology, midday leaf temperatures, mean maximum photosynthetic rate, water use efficiency and plant/water relations. If classical plant life forms are equivalent to plant functional groups, then we should expect to see patterns of similarity in morphological and ecophysiological traits.
The White Mountains lie east of the Sierra Nevada across the Owens Valley that separates the two ranges (CitationLloyd and Mitchell, 1973; CitationHall, 1991). The White Mountains are a relatively small and narrow range extending 60 km from their northern end in Nevada across into California and Westgard Pass in the south, with a width averaging only 20–25 km (). However, the range rises sharply from elevations of 1250 m in the Owens Valley to a high point of 4343 m at White Mountain Peak over a lateral distance of less than 10 km. Lying as it does in the rain shadow of the Sierra Nevada, the White Mountains average only about a third of the rainfall received at similar elevations on the west side of the Sierra Nevada. The Barcroft Laboratory (3801 m) of the White Mountain Research Station receives a mean annual precipitation of only 478 mm, with the great majority of this falling as winter snow (CitationRundel et al., 2005).
Despite an alpine habitat of only 106 km2, the White Mountains have a surprisingly rich alpine flora of 163 vascular plant species (CitationRundel et al., 2005). The greatest richness of alpine species (67 species present, 41% of the flora) occur in fellfield and associated drainage channel habitats intermediate along a moisture gradient between wet sites with saturated soils and dry slopes (CitationMooney et al., 1962; CitationMitchell et al., 1966). Flanking communities along a moisture gradient from wet (moist meadows) to dry (open flats that become relatively dry in summer) hold 24% and 21% of the flora, respectively. Only a small number of species typically are found on wet sites with saturated soils, aquatic habitats, or dry slopes.
Materials and Methods
COMMUNITY DOMINANCE
With an interest in focusing on the ecological relationships and adaptive strategies of alpine species in fellfield habitats, we sampled mean species cover and frequency of perennials along a gradient from mesic to more xeric fellfield sites to gain an understanding of relative species dominance and frequency. Our field measurements were carried out at a site with granitic substrate (elevation 3750 m) approximately 1 km south of the Barcroft Laboratory.
Each of four sites along this fellfield gradient was sampled using ten 1.0 × 1.0 m quadrats to obtain mean percent cover for large rocks, soil, and total vegetation for the sampled areas. Coverage of each perennial species was estimated within a quadrat and assigned a value of 0–5: 5 = 50–100% coverage, 4 = 25–50% coverage, 3 = 10–25% coverage, 2 = 2–10% coverage, 1 = <2% coverage, and 0 = not present. Mean cover for each species was the average for cover values of the ten quadrats, and relative frequency was the proportion of quadrats in which that species occurred.
COMPARATIVE SPECIES STUDIES
Eight fellfield species were selected for further investigation from data collected on species coverage and other observations to provide species in each of four life forms: chamaephyte, cushion plant (including prostrate, rooted mats), herbaceous dicot perennial, and graminoid perennial. Multiple individuals of each species were excavated to examine the below-ground rooting architecture.
Because microclimate conditions can vary significantly over short distances in fellfield habitats, we measured diurnal courses of temperature for air (1 m above the soil surface), surface soil, rock surface, and leaf surfaces for each of our eight study species. Using an infrared thermometer (Everest Scientific Model 210), these temperatures were monitored at 90- to 120-minute intervals on 11–12 July 2000 to determine the thermal environments present.
Comparative ecophysiological measurements were made under field conditions during the summer of 2000 to examine possible ecophysiological traits associated with putative plant functional groups. Four of the species selected were Penstemon heterodoxus A. Gray var. heterodoxus (Scrophulariaceae), Trifolium andersonii A. Gray var. beatleyae (J. M. Gillett) Isely (Fabaceae), Poa glauca M. Vahl. subsp. rupicola (Nash) W. A. Weber (Poaceae), and Eriogonum ovalifolium Nutt. var. nivale (Canby) M. E. Jones (Polygonaceae). These were the only species occurring at every site in sampled quadrats, and were therefore justifiable as representative taxa to be used for the experimental field measurements. Four other characteristic and widespread, but less abundant, fellfield species were also used, so that generally two species would be used for each possible plant functional type: Ivesia lycopoidiodes A. Gray subsp. scandularis (Rydb.) Keck (Rosaceae), Linanthus nuttallii (A. Gray) Milliken subsp. pubescens R. Patterson (Polemoniaceae), Carex helleri Mackenzie (Cyperaceae), and Chrysothamnus viscidiflorus (Hook.) Nutt. subsp. viscidiflorus (Asteraceae).
Among the measurements obtained were maximum photosynthetic capacity (P max, μmol CO2 m−2 s−1), water-use efficiency (WUE) as measured by the ratio of internal to ambient CO2 (c i:c a), and pre-dawn and midday water potential (MPa) at the end of the active growing season. To assess photosynthetic capacity and WUE at the peak of the growing season, P max and c i:c a were obtained at mid-morning, midday, and mid-afternoon on 24 August 2000 using at least two sampled shoots on each of three individuals for each study species. These data were collected with a LICOR-6400 portable photosynthesis system (LICOR, Inc., Lincoln, NB, U.S.A.). Predawn and midday water potentials were measured using a Scholander-type pressure chamber on 18 September 2000 at the end of the active growth season to assess the significance of water stress in limiting growth in each of these life forms. Data on water potential was not taken for the two graminoid species.
Results
COVER AND FREQUENCY
Measurements of species cover and frequency across a moisture gradient of fellfield habitats encountered a total of 20 plant species (). Individual sites had a range of 10–13 species in each set of ten 1.0-m2 quadrats sampled (). Sites along a rocky runoff channel, on a gravelly flat, and a rocky flat all had plant coverage about 70%, with open soil coverage varying from 12% to 26% and rock cover ranging from 4% to 20%. A rocky hillside with old frost polygons had a high rock and soil exposure and the lowest plant coverage with only 43% (). Four species had consistently high relative cover across this gradient: Penstemon heterodoxus var. heterodoxus, Trifolium andersonii var. beatleyae, Poa glauca subsp. rupicola, and Eriogonum ovalifolium var. nivale.
PLANT FUNCTIONAL GROUPS AND SPECIES DESCRIPTIONS
The eight species selected for study represented four distinct ecological life forms, and these life forms were hypothesized to represent putative plant functional groups: (a) chamaephytes (Chrysothamnus viscidifolius subsp. viscidifolius and Linanthus nuttallii subsp. pubescens); (b) cushion plants (Trifolium andersonii var. beatleyae, Penstemon heterodoxus var. heterodoxus, and Eriogonum ovalifolium var. nivale); (c) herbaceous dicot perennial (Ivesia lycopoidiodes subsp. scandularis); and (d) graminoid perennials (Poa glauca subsp. rupicola and Carex helleri). Six of these species are illustrated in .
Chrysothamnus viscidiflorus typically is the tallest perennial of all species inhabiting typical White Mountain fellfields, where this chamaephyte with ascending branches rarely exceeds 20–50 cm in height, although it occurs taller at lower elevations. It has sessile, narrowly linear, slightly resinous leptophylls generally less than 40 mm in length and less than 3 mm in width. Linanthus nuttallii subsp. pubescens is classified as a chamaephyte rooted only at the plant base but has prostrate lateral shoots and, thus, more closely resembles a low subshrub. This woody mat bears bright green, crowded and overlapped leptophylls (mean blade width = 1.2 mm) generally with 5 short and extremely narrow lobes, and shoots senesce brown during late summer drought.
Eriogonum ovalifolium var. nivale is a very low, compact, evergreen cushion plant forming a grayish mound 2–5 cm above the surface with dense foliage. Its leptophylls have roundish, wool-covered, ascending to erect blades (mean blade width = 3.5 mm) in tight helical clusters. Both Trifolium andersonii var. beatleyae and Penstemon heterodoxus var. heterodoxus in the alpine fellfield are prostrate mats that form shallow, adventitious roots along buried stems, and they generally have some leaves year-round although they lose most leaves by the end of the summer growing season. The pinnately compound leaves of T. andersonii var. beatleyae generally have 5 or 7 minute, pubescent leaflets (mean leaflet blade width = 1.8 mm) positioned less than 10 mm from the ground. What appear to be single cushions 5–15 cm in diameter of this species are often found on excavation to be formed of multiple small heads of individual plants growing together. Penstemon heterodoxus, a more mat-forming species, has larger, simple leptophylls (mean blade width = 7.0 mm). The glabrous green leaves remain close to the soil except when erect flowering shoots (to 20 cm) are formed.
Ivesia lycopodioides subsp. scandularis produces a basal rosette of highly dissected, ascending green leaves from a buried caudex. Each segment of the leaf is minute (mean segment area <1.0 mm2), but overall a single leaf up to 15 cm in length has >200 segments and, thus, a significant leaf area. This species becomes deciduous as the summer growing season ends and thereafter remains leafless until spring growth is initiated.
Graminoid perennials in White Mountain fellfield are rhizomatous herbs that have grass-like leaves several millimeters wide. Unlike the dicots described above, all of which have amphistomatic leaves with isolateral mesophyll, the two graminoids exhibit bifacial hypostomatic leaves. Poa glauca subsp. rupicola is a low species with relatively few and narrow basal and lower cauline leaves, whereas Carex helleri generally forms a small, dense tussock of bright green leaves arising from the base and 3-angled stems, which rarely reach 30 cm when in fruit.
FUNCTIONAL ATTRIBUTES
Four of the study species had well-developed taproots (), assumed to have carbohydrate storage, but rooting morphology was only weakly associated with life form. Linanthus nuttallii subsp. pubescens, two cushion plants (Trifolium andersonii var. beatleyae and Eriogonum ovalifolium var. nivale), and Ivesia lycopodioides subsp. scandularis all had taproots extending to depths of about 20–40 cm. Chrysothamnus viscidiflorus and Penstemon heterodoxus each had spreading woody root systems lacking a taproot, and T. andersonii var. beatleyae also had spreading adventitious roots. The two graminoids (Poa glauca subsp. rupicola and Carex helleri) formed dense mats of fibrous roots.
Although all of these species are characteristic of alpine fellfields and reach upper elevations of 3900–4300 m, they vary significantly in their presence at lower elevations in plant communities below alpine habitats. Ivesia lycopodioides subsp. scandularis, Poa glauca subsp. rupicola, and Carex helleri are essentially restricted to alpine habitats and exhibit elevational amplitudes of only 650–850 m over their distribution range in the White Mountains ().
At the other extreme, Eriogonum ovalifolium var. nivale and Chrysothamnus viscidifolius extend in distribution from pinyon-juniper habitats up into the alpine, with an elevational amplitude of 2250–2450 m over their range of occurrence. The other species are intermediate in their ecological habitat plasticity. Linanthus nuttallii subsp. pubescens and Trifolium andersonii var. beatleyae reach from montane habitats into the alpine with an elevational amplitude of about 1600 m, while Penstemon heterodoxus extends from the subalpine into the alpine with an elevational range of 1000 m.
The life form architecture and height of individual species produced a large range of functional leaf temperatures for photosynthesis. Under late morning conditions at 11:00 a.m. in the middle of the active growing season in August 2000, air temperatures averaged 16°C at our site, while adjacent surfaces of large boulder had heated to 32°C and soil surfaces reached 58°C. Four species with fine foliage held upright away from the ground surface (Chrysothamnus viscidiflorus, Linanthus nuttallii subsp. pubescens, Ivesia lycopodioides subsp. scandularis, and Carex helleri) all had leaf temperatures at or within a few degrees of air temperature () and maintained this pattern over the course of the day. This group included a mix of life forms: two chamaephytes, a dicot herbaceous perennial, and a graminoid herbaceous perennial. Poa glauca subsp. rupicola, with upright foliage but with the majority of its actively photosynthesizing tissue in a tight rosette, operated at about 10°C above ambient temperature. The three prostrate cushion and mat-forming species, Trifolium andersoni var. beatleyae, Eriogonum ovalifolium var. nivale, and Penstemon heterodoxus, with their photosynthetic tissue held at or very close to ground level, had shoot temperatures as much as 15–20°C or more above ambient temperature (). This temperature differential dropped to 8–14°C during the afternoon.
Mean maximum photosynthetic rates in the eight study species did not show any strong patterns correlated with form. All of these species had high to moderately high P max, with a range from a low of 11.5 μmol m−2 s−1 in Ivesia lycopoidioides subsp. scandularis to a high rate of 25 μmol m−2 s−1 in Poa glauca subsp. rupicola and Trifolium andersonii var. beatleyae (). Most of the species fit a range from 14 to 18 μmol m−2 s−1.
For the eight species studied, midday water potentials at the end of the active growing season showed only a small range of variation from −1.8 to −2.4 MPa (). Predawn water potentials ranged from −1.3 to −1.5 MPa in all species except Penstemon heterodoxus, for which ψpredawn was substantially higher at −0.8 MPa. All fellfield species studied reached their peak photosynthetic activity from midmorning to noon, and then declined slowly in the afternoon (Gibson et al., unpublished data).
Water-use efficiency as estimated by c i:c a under midmorning conditions at the peak period of growth in August 2000 did not show any pattern of strong differentiation between life forms or even between species (). The overall range of values found indicated a common trait of relatively high WUE, with the lowest mean c i:c a ratio of 0.43 indicating somewhat higher WUE in Poa glauca subsp. rupicola and the highest mean c i:c a of 0.59 in Linanthus nuttallii subsp. pubescens.
Discussion
Clear associations between plant life form and functional attributes of the eight fellfield species studied are not present in our data. In elevational range of occurrence, there is a weak correlation with chamaephytes and cushion plants having broader ranges than herbaceous perennials, but the widest ranging of our eight study species include one chamaephyte and one cushion. If the entire alpine flora of the White Mountains is considered, shrubs (phanerophytes), chamaephytes, and annuals are all far more likely to have broad elevational ranges of occurrence than are broad-leaved herbaceous perennials, perennial graminoids, and cushion plants and mats (CitationRundel et al., 2005).
Similarly, there is a correlation between life form and root morphology, with fibrous roots associated with perennial graminoids as would be expected, and cushion plants and the chamaephytes exhibiting characteristic tap roots, and mat-forming cushions having also shallow, spreading, adventitious roots arising along stems to take advantage of temporary surface soil moisture.
Midday leaf temperatures are a function of the architectural position of the leaves with respect to the ground surface rather than with life form, with the exception of the three cushions, which by definition hold their photosynthetic tissues close to the soil surface.
Among the chamaephytes, for example, the two upright species (Chrysothamnus viscidiflorus and Linanthus nuttallii subsp. pubescens) have leaves that track ambient air temperature, while the mat-forming Penstemon heterodoxus has leaves that are heated significantly over air temperatures at midday. This ability of some plant architectures to promote high leaf temperatures is a significant component of the ability of the C4 grass Muhlenbergia richardsonis (Trin.) Rydb. to grow successfully at elevations up to nearly 4000 m in the White Mountains (CitationSage and Sage, 2002).
The maximum rates of net photosynthesis measured in this study in the White Mountains are similar to those reported in the literature in alpine plant species in Europe and the western United States. Using data published in the past two decades, herbaceous perennials have been reported to have photosynthetic capacities under field conditions of 7–25 μmol m−2 s−1, with mean values of P max about 16 μmol m−2 s−1 (CitationChapin and Oechel, 1983; CitationKörner and Diemer, 1987; CitationRawat and Purohit, 1991; CitationBowman et al., 1995). Our six fellfield herbaceous perennials had a very similar mean photosynthetic rate of 17 μmol m−2 s−1, with a range of 11.5–25.5 μmol m−2 s−1 among species.
Dwarf alpine subshrubs and shrubs have been reported to have lower photosynthetic capacities of 6–13 μmol m−2 s−1 (CitationNakano and Ishida, 1994; CitationBowman and Conant, 1994), although some of these low values can be attributed to the fact that many measurements came from species of Ericaceae, which characteristically have low rates of net carbon assimilation. The two chamaephytes in our study, Chrysothamnus viscidflorus and Linanthus nuttallii subsp. pubescens, had relatively high P max of 18.0 and 16.4 μmol m−2 s−1, respectively, with a mean similar to that measured in the Barcroft herbaceous perennials.
Given the relatively low precipitation experienced in the alpine region of the White Mountains, it would be logical to hypothesize that moisture availability was a limiting factor for plant growth; however, evidence supporting this hypothesis is equivocal. Qualitative phenological observations are clearly consistent with drought as a limiting factor. Our observations indicate that vegetation in dry meadows and rocky flats in the White Mountains typically ceases growth and dries up by early August in most years, at a time when favorable temperatures for growth still exist. The phenological cycle for fellfield species is much more variable, but again suggests limitations of water availability. Following a winter with relatively good snowfall, flowering in this community in the summer 2000 peaked in mid-August, 6–8 weeks later than its peak in the drier summer 2001. Arguing against water as a strong limiting factor are the relatively modest water predawn and midday water potentials measured in mid-September 2000 near the end of the summer growing season. These water potentials are generally comparable with or even higher than those reported from much more mesic alpine habitats in the central Rocky Mountains (CitationOberbauer and Billings, 1981), and far higher than those reported for high elevation Andean perennials in northern Chile (CitationRundel et al., 2003).
Limited water availability need not be associated with low precipitation in alpine habitats if leaf area index (LAI) is relatively low. Studies by CitationSveshnikova (1973) in the arid Pamir Range of central Asian found little evidence of plant water stress despite only about 300 mm of annual precipitation. She recorded osmotic potentials that remained above −2 MPa during the growing season and peak transpirations occurring from 12:00–16:00 hours. However, we have documented high plant cover in fellfield habitats (), suggesting that LAI is not strongly constrained by available soil moisture.
WUE has been used to separate plant functional groups for boreal plants using carbon isotope ratios as an integrating measure of CO2 and water fluxes (CitationBrooks et al., 1997). They found that correlations between life form and carbon isotope ratio were related to differences in plant stature and leaf longevity. Within our study species, the measure of c i:c a ratio for midmorning photosynthesis in the peak of the growing system does not suggest any significant differences between life forms and this measure, given that all species were able to maintain relatively high WUE with values of 0.43–0.59. These ratios show a higher mean WUE than present in semi-woody shrubs growing at 3550 m in high elevation Andean perennials of northern Chile where mean annual rainfall is only about 160 mm (CitationRundel et al., 2003).
Conclusions
If many of the fellfield plant species share similar functional attributes, then why do some occur in great abundance while others are relatively rare? There are several possible answers to this basic question of diversity and ecosystem function. One possibility, of course, is that there are other functional attributes that we were not measuring. These could range from components of temperature response in carbon allocation at the physiological level to reproductive traits related to pollination, seed dispersal, germination conditions, and seedling ecology. A second hypothesis, presented by CitationWalker et al. (1999), suggests that uncommon species might well be functional analogs of dominant species, but differ in terms of their abilities to respond to environmental stresses and disturbance. If changing conditions cause dominant species to decline, there is a pool of functionally equivalent minor species present to substitute for their place in the community. Thus, uncommon species confer resilience on the community with respect to ecosystem function.
FIGURE 1. Regional location of the White Mountains in eastern California and adjacent Nevada. Alpine habitats over 3500 m elevation are shown by the stippled area
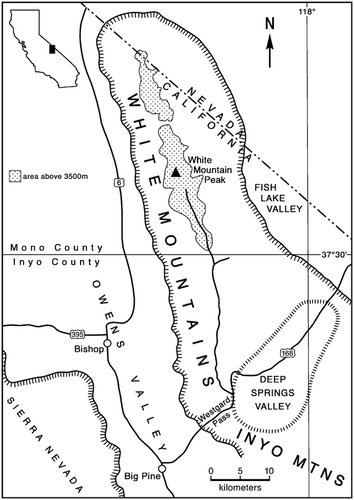
FIGURE 2. Fellfield habitats at 3750 m elevation in the White Mountains of eastern California: (a) landscape view of field site; (b) general form and cover of a fellfield plant community
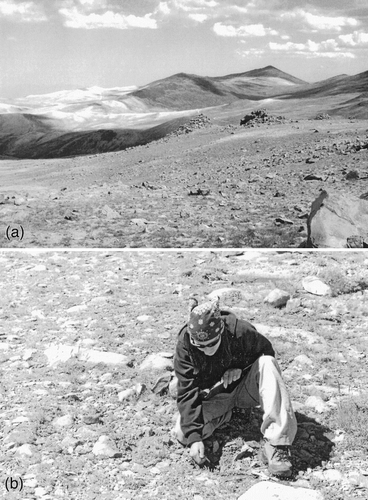
FIGURE 3. Common fellfield plant species: (a) Eriogonum ovalifolium var. nivale, cushion; (b) Linanthus nuttallii subsp. pubescens, chamaephyte; (c) Penstemon heterodoxus var. heterodoxus, mat-forming cushion; (d) Trifolium andersonii var. beatleyae, cushion; (e) Ivesia lycopoidiodes subsp. scandularis, herbaceous dicot perennial; and (f) Carex helleri, herbaceous graminoid perennial
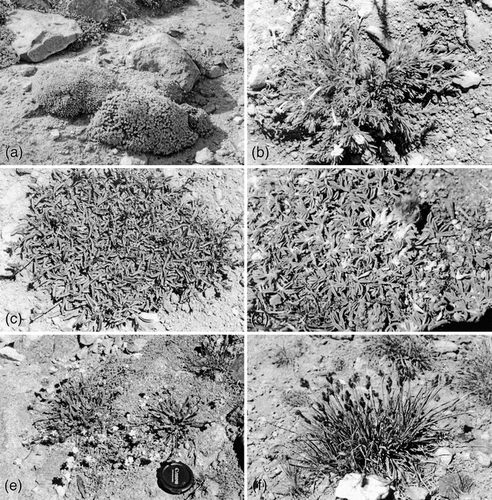
TABLE 1 Cover of perennial alpine species at the White Mountains study site where ecophysiological experiments were conducted (3750 m elevation) for a moisture gradient of four fellfield habitats. Each habitat was sampled using ten 1 × 1 m quadrats to obtain mean percent cover for large rocks, soil, and total vegetation for the sampled 10 m2. Cover of each perennial species was estimated within a quadrat: 5 (50–100%), 4 (25–50%), 3 (10–25%), 2 (2–10%), 1 (<2%). Mean cover for each species is the average for cover values of the ten quadrats, and relative frequency is the proportion of quadrats in which that species occurred
Table 2 Functional attributes of eight common fellfield plants at 3750 m elevation in the White Mountains in 2000. Diurnal cycles of ambient and shoot temperatures (T shoot) were measured on 11–12 July. Data on mean maximum photosynthetic capacity (P max) and ratio of internal to ambient CO2 level (c i:c a ratio) were obtained on 24 August. Leaf water potential (ψ) was measured at the end of the growing season on 18 September
Acknowledgments
We gratefully acknowledge the financial and logistical support offered to this project by the White Mountains Research Station. In particular we thank Catherine Kleier, Frank Powell, Dave Trydal, Mike Morrison, Tyler Rundel, and Erin Gibson for the assistance with various parts of this research.
Notes
Ms submitted September 2003
References Cited
- Billings, W. D. 2000. Alpine vegetation. In: Barbour, M. G., and Billings, W. D. (eds.) North American Terrestrial Vegetation. Second edition. Cambridge: Cambridge University Press, 536–572.
- Bowman, W. D. and R. T. Conant . 1994. Shoot growth dynamics and photosynthetic response to increased nitrogen availability in the alpine willow. Salix glauca. Oecologia 97:93–99.
- Bowman, W. D. and T. R. Seastedt . 2001. Structure and Function of an Alpine Ecosystem: Niwot Ridge, Colorado. Oxford: Oxford University Press.
- Bowman, W. D. , T. A. Theodose , and M. C. Fisk . 1995. Physiological and production response to increases in limiting resources in alpine tundra: implications for differential community response to environmental change. Oecologia 101:217–227.
- Brooks, J. R. , L. B. Flanagan , N. Buchmann , and J. R. Ehleringer . 1997. Carbon isotope composition of boreal plants: functional grouping of life forms. Oecologia 110:301–311.
- Chapin, F. S. and C. Körner . (eds.),. 1995. Arctic and Alpine Biodiversity: Patterns, Causes and Ecosystem Consequences. Berlin: Springer.
- Chapin, F. S. and W. C. Oechel . 1983. Photosynthesis, respiration, and phosphate absorption by Carex aquatilis ecotypes along latitudinal and local environmental gradients. Ecology 64:743–751.
- Hall, C. A. (ed.),. 1991. Natural History of the White-Inyo Range, Eastern California. Berkeley, University of California Press.
- Hooper, D. U. and P. M. Vitousek . 1998. Effects of plant composition and diversity on nutrient cycling. Ecological Monographs 68:121–149.
- Körner, C. 1999. Alpine Plant Life: Functional Plant Ecology of High Mountain Ecosystems. Berlin: Springer.
- Körner, C. and M. Diemer . 1987. In situ photosynthetic responses to light, temperature and carbon dioxide in herbaceous plants from low and high altitude. Functional Ecology 1:179–194.
- Lavorel, S. , S. McIntyre , J. Landsberg , and T. D A. Forbes . 1997. Plant functional classifications: from general groups to specific groups based on response to disturbance. Trends in Ecology and Evolution 12:474–478.
- Lavorel, S. , S. McIntyre , and K. Grigulis . 1999. Plant response to disturbance in a Mediterranean grassland: how many functional groups?. Journal of Vegetation Science 10:661–672.
- Lloyd, R. M. and R. S. Mitchell . 1973. A Flora of the White Mountains of California. Berkeley: University of California.
- McIntyre, S. and S. Lavorel . 2001. Livestock grazing in subtropical pastures: steps in the analysis of attribute response and plant functional types. Journal of Ecology 89:209–226.
- McIntyre, S. , S. Lavorel , J. Landsberg , and T. D A. Forbes . 1999. Disturbance response in vegetation: towards a global perspective on functional traits. Journal of Vegetation Science 10:621–630.
- Mitchell, R. S. , V. C. LaMarche , and R. M. Lloyd . 1966. Alpine vegetation and active frost features of Pellsier Flats, White Mountains, California. American Midland Naturalist 75:516–525.
- Mooney, H. A. , G. St. Andre , and R. D. Wright . 1962. Alpine and subalpine vegetation patterns in the White Mountains of California. American Midland Naturalist 68:257–273.
- Nakano, T. and A. Ishida . 1994. Diurnal variation of photosynthetic rates and xylem pressure potentials in four dwarf shrubs (Ericaceae) in an alpine zone. Proceedings NIPR Symposium on Polar Biology 7:243–255.
- Oberbauer, S. F. and W. D. Billings . 1981. Drought tolerance and water use by plants along an alpine topographic gradient. Oecologia 50:325–331.
- Raunkiaer, C. 1934. The Life Forms of Plants and Statistical Plant Geography. Oxford: Clarendon.
- Rawat, A. S. and A. N. Purohit . 1991. CO2 and water vapour exchange in four alpine herbs at two altitudes and under varying light and temperature conditions. Photosynthetic Research 28:99–108.
- Rundel, P. W. , A. C. Gibson , G. S. Midgley , S. J E. Wand , B. Palma , C. Kleier , and J. Lambrinos . 2003. Ecological and ecophysiological patterns in a pre-altiplano shrubland of the Andean Cordillera in northern Chile. Plant Ecology 169:179–193.
- Rundel, P. W. , A. C. Gibson , and M. R. Sharifi . 2005. The alpine flora of the White Mountains, California. Madroño (in press).
- Sage, R. F. and T. L. Sage . 2002. Microsite characteristics of Muhlenbergia richardsonis (Trin.) Rydb., an alpine C4 grass from the White Mountains, California. Oecologia 132:501–508.
- Smith, T. M. , H. H. Shugart , and F. I. Woodward . (eds.),. 1997. Plant Functional Types: Their Relevance to Ecosystem Properties and Global Change. Cambridge: Cambridge University Press.
- Sullivan, G. and J. B. Zedler . 1999. Functional redundancy among tidal marsh halophytes: a test. Oikos 84:246–260.
- Sveshnikova, V. M. 1973. Water regime of plants under the extreme conditions of high mountain deserts of Pamirs. In: Slayter, R. O. (ed.), Plant Response to Climatic Factors. UNESCO: Proceedings of the Uppsala Symposium (1970), 555–561.
- Tilman, D. , P. B. Reich , J. Knops , D. Wedlin , T. Mielke , and C. Lehman . 2001. Diversity and productivity in a long-term grassland experiment. Science 294:843–845.
- Vanclay, J. K. , A. N. Gillison , and R. J. Keenan . 1997. Using plant functional attributes to quantify site productivity and growth patterns in mixed forests. Forest Ecology and Management 94:149–163.
- Walker, B. , A. Kinzig , and J. Langridge . 1999. Plant attribute diversity, resilience and ecosystem function: the nature and significance of dominant and minor species. Ecosystems 2:95–113.
- Walker, B. H. and J. L. Langridge . 2002. Measuring functional diversity in plant communities with mixed life forms: a problem of hard and soft attributes. Ecosystems 5:529–538.
- Wardle, D. A. , K. I. Bonner , and G. M. Barker . 2000. Stability of ecosystem properties in response to above-ground functional group richness and composition. Oikos 89:11–23.