Abstract
We investigated the influence of nitrogen (N) and phosphorus (P) on N2-fixation and abundance of two of the most common N2-fixing arctic lichens, Peltigera aphthosa and P. polydactyla, in two common moist upland tundra types, acidic and non-acidic tundra, at Toolik Lake, Alaska. Acidic tundra has higher N and lower P availability than non-acidic tundra. We measured the abundance of the lichens in control (no fertilization), N- and P-fertilized plots, and N2-fixation using the acetylene reduction assay method on lichens from control and P-fertilized plots from both tundra types. Lichens on N-treated plots were too scarce to include in our N2-fixation estimates. Lichen abundance was lower in plots fertilized with N than in control and P-fertilized plots, while per-biomass N2-fixation rates were higher in P-fertilized plots than in control plots. Per-biomass rates of N2-fixation did not differ between acidic and non-acidic tundra, but both lichen species are more abundant on acidic tundra. Thus, despite per-biomass stimulation of N2-fixation by experimental P addition and reduction in lichen abundance with N fertilization, Peltigera contributes more N to the acidic tundra site, indicating that soil N and P availability are not the primary controls of N2-fixation and abundance of these lichens.
Introduction
Nitrogen-fixation is an important source of new nitrogen (N) to arctic ecosystems because N inputs from atmospheric deposition are very low (CitationAlexander and Schell, 1973; CitationAlexander, 1974; CitationBarsdate and Alexander, 1975; CitationChapin et al., 1991; CitationChapin and Bledsoe, 1992). Yet, surprisingly little research on N2-fixation has been done in upland tundra, despite its widespread distribution in the circumpolar Arctic (CitationBliss and Matveyeva, 1992; CitationWalker, 1998). Because N2-fixation is a physiological process subject to abiotic and biotic controls, determining influences on N2-fixation at the organismal level can offer insights into the important controls of N2-fixation at the ecosystem level (CitationChapin and Bledsoe, 1992).
Lichens are major components of arctic ecosystems; thus, the contribution of N2-fixing lichens to arctic nutrient cycles is likely substantial (CitationNash and Olafsen, 1995). Additionally, N2-fixing lichens may be particularly important in supplying new N in extreme environments where N2-fixing plants are rare (CitationGunther, 1989). N2-fixation in arctic lichens occurs in species that host cyanobacterial symbionts Nostoc or Anabaena, and has been measured in several lichen genera, including Peltigera, Nephroma, and Stereocaulon in the arctic and alpine tundra (CitationAlexander, 1974).
N2-fixation is inhibited by increasing available N (CitationAlexander et al., 1978; CitationChapin et al., 1991; CitationVitousek and Howarth, 1991; CitationChapin and Bledsoe, 1992; CitationVitousek and Field, 1999). Phosphorus (P) stimulates N2-fixation, and low P availability constrains N2-fixation (CitationChapin et al., 1991; CitationChapin and Bledsoe, 1992; CitationCrews, 1993; CitationHendzel et al., 1994; CitationBowman et al., 1996; CitationThompson and Vitousek, 1997; CitationKurina and Vitousek, 1999; CitationVitousek, 1999; CitationVitousek and Field, 1999; but see CitationAlexander et al., 1978). These studies support the suggestion that N2-fixation may be limited by P, and that the ratio of N to P availability is important in regulating N2-fixation (CitationVitousek and Howarth, 1991). In spite of substantial work at other sites, the influence of N or P availability on N2-fixation in upland tundra has not previously been investigated. Furthermore, it is unclear whether N and P have similar influences over growth, abundance, and N2-fixation activity of lichens.
Here we investigate the influence of N and P on abundance and N2-fixation of two N2-fixing arctic lichens, Peltigera aphthosa and P. polydactyla, in moist acidic and moist non-acidic tundra near Toolik Lake, Alaska. Moist acidic and non-acidic tundra are the two most common tundra types in the region (CitationWalker, 1998), and they differ greatly in pH, plant species composition, biomass (CitationWalker et al., 1994; CitationWalker et al., 1995), and N and P availability (CitationHobbie and Gough, 2002). We focus on these particular lichens because previous studies have demonstrated significant N2-fixation in these species at other sites (CitationAlexander, 1974; CitationChapin and Bledsoe, 1992), and lichen surveys indicated that these two species are more abundant and have higher rates of N2-fixation than other N2-fixing lichens in tundra near Toolik Lake.
We measured the influence of N and P availability on lichen abundance by comparing lichen cover among plots receiving either no fertilization (control), or N or P fertilization. We also compared lichen cover between acidic and non-acidic tundra. We compared lichen N2-fixation rates in the two species between control and P fertilized plots at both sites. N treatment plots were not included in the N2-fixation comparison because lichens were too scarce. Specifically, we address the following hypotheses:
Fertilization with N causes a decline in lichen abundance and therefore a decline in the contribution of lichens to N2-fixation per unit area of tundra.
Rates of N2-fixation by lichens are stimulated by fertilization with P.
Lichens from acidic tundra, where N availability is greater, have lower rates of N2-fixation and are less abundant than lichens from non-acidic tundra where P availability is higher; thus the contribution of lichens to N2-fixation per unit area of tundra is lower in acidic tundra compared to non-acidic tundra.
Site Description
Research was conducted at the Arctic Long Term Ecological Research (LTER) site at Toolik Lake, in the northern foothills of the Brooks Range, Alaska (68°38′N, 149°38′W). We studied lichens on moist acidic and moist non-acidic tundra adjacent to Toolik Lake. Because the two sites are <2 km apart, they experience the same climate conditions. However, they differ considerably in substrate age, pH, vegetation, and N and P availability (CitationHobbie and Gough, 2002). Moist acidic tundra is found on older glacial surfaces, while moist non-acidic tundra is found on younger surfaces or surfaces that receive relatively high deposition of loess (M. D. CitationWalker et al., 1994; CitationD. A. Walker et al., 1998). The acidic tundra site investigated here was deglaciated approximately 50,000–120,000 years ago, while the non-acidic site was deglaciated approximately 11,500–25,000 years ago (CitationHamilton, 2003). Moist acidic tundra has a soil pH of 3–4. The vegetation is mostly comprised of sedges, dwarf shrubs, mosses, and fruticose lichens. Dominant species include the vascular plants Eriophorum vaginatum, Betula nana, Carex bigelowii, Ledum palustre, and Vaccinium vitis-idea; the mosses Sphagnum spp. and Hylocomium splendens; and the lichens P. aphthosa, P. polydactyla, and (in relatively low abundance) Nephroma arcticum. Non-acidic tundra has a circumneutral pH (6–7) and is dominated by dwarf shrubs, sedges, and lichens. Forbs are more abundant and diverse in non-acidic than acidic tundra. Major species include the vascular plants Eriophorum angustifolium ssp. triste, Carex bigelowii, Dryas integrifolia, and Salix reticulata; the moss Tomenthypnum nitens; and the lichens P. aphthosa and P. polydactyla (CitationWalker et al., 1994). Net N mineralization rates are higher in acidic tundra than in non-acidic tundra, while cation exchange capacity, extractable calcium, and extractable P in the mineral soil are higher in non-acidic tundra (CitationHobbie and Gough, 2002). Plant biomass is significantly greater in acidic tundra than in non-acidic tundra in this region (CitationWalker et al., 1995; S. E. Hobbie, L. Gough, and G. R. Shaver, unpublished data). N limits production in acidic tundra, while N and P together strongly limit production in non-acidic tundra (CitationShaver et al., 1986; CitationGough and Hobbie, 2003).
Methods
FERTILIZATION EXPERIMENTS
We studied lichens in plots treated with N or P fertilizer in both acidic and non-acidic tundra. Treatment plots are 5 × 20 m and have received either no fertilization (control) or fertilization as single annual dry applications of granular fertilizer immediately following snowmelt, with N as NH4NO3 or P as P2O5 at a rate of 10 g N m−2 y−1 and 5 g P m−2 y−1, respectively (CitationBret-Harte et al., 2001). There are four blocks at the acidic tundra site and three blocks at the non-acidic site. Fertilization began in 1989 on acidic tundra and in 1997 on non-acidic tundra as part of the core LTER experiments.
LICHEN COVER SURVEYS
Lichen abundance was quantified by estimating percent lichen cover at ambient lichen field moisture within the experimental fertilization plots and outside of the fertilization plots at acidic and non-acidic study sites. Percent lichen cover was estimated using a 1-m2 quadrat that was subdivided into a 25-box grid and used to estimate the percent of the quadrat area that was covered by the two lichens. Within each of the 21 fertilization plots, we surveyed six 1-m2 quadrats. Peltigera area values from six quadrats were averaged for each plot, and the averages for the plots were compared among treatments and between sites and species (21 plots × 2 species = 42 observations). Outside of the fertilization plots, percent cover in 1-m2 quadrats was determined every 5 m along eight 50-m transects at each site (10 quadrats per transect × 8 transects × 2 sites × 2 species = 320 observations). These transects were parallel to the slope and were approximately 5 m apart. Because this is a nondestructive measure, we assume that any differences in size due to variability in ambient lichen moisture on measurement days are negligible, certainly less than the precision of the visual percent cover measurement.
We compared cover among fertilization plots using a three-way ANOVA, with site, treatment, and species as factors, and we used Tukey's HSD posthoc test to make pairwise comparisons among treatments. We compared lichen cover between acidic and non-acidic tundra using two-way analysis of variance (ANOVA) with site and species as factors.
ACETYLENE REDUCTION ASSAYS
N2-fixation in the lichens was estimated using the acetylene reduction assay (ARA) (CitationHardy et al., 1968). Lichens were collected within 24 h of the start of the ARA, placed in plastic bags, and transferred into uncapped 250-mL mason jars within 5 h of collection. The mason jars were modified by punching a hole in each lid and fitting the hole with a silicone Hungate septum. In preparation for the incubation, lichens were hydrated in water from Toolik Lake for 2 min and drained for 1 min to ensure moisture was not limiting and that all lichens were experiencing the same moisture conditions. We used sufficient biomass of lichen to cover the surface of the 24-cm2 mason jar lid. Using a syringe, 20 mL of air were removed from the chamber, and 20 mL of acetylene were injected into the chamber. We incubated the lichens under constant light (285 μmol m−2 s−1, compact fluorescent bulbs) in a water bath that maintained the temperature in the range of 11.5–12.5°C, which falls within one standard deviation of the mean air temperature (9.6 ± 5.1°C) during the growing season (10 June to 15 August) in 2000 and 2001 (CitationArctic LTER database, 2002). The jars were inverted for the incubation, orienting the lichen surface to face the light source. We first did several preliminary experiments. We analyzed acetylene blanks directly from our acetylene supply, and noted minimal contamination of ethylene in our acetylene. That small amount was subtracted from the final measure of ethylene when analyzing data. We incubated lichens with no acetylene and measured no ethylene production in 10 h, and we incubated empty jars with acetylene and measured no ethylene production. After establishing that the rate of N2-fixation is linear between 2 and 10 h of incubation, we incubated lichens for 2 to 6 h, at which time a 5-mL gas sample was removed from the jars and analyzed for ethylene using a gas chromatograph (Shimadzu GC-8A, Shimadzu Scientific Instruments, Inc., Columbia, Maryland) with flame-ionization detection (FID) and a Porapak N column. After the incubation, lichens were dried at 65°C for at least 36 h, weighed, and the rate of acetylene reduction was calculated on a per-unit moist lichen area and per-unit dry mass basis.
On 21 July 2000, 24 lichens were collected to compare acetylene reduction rates between control and P fertilized plots. We were unable to harvest sufficient lichens from the N fertilized plots. The experiment is a 2 × 2 × 2 factorial design with three replicates of each species by site by treatment combination. We compared rates of N2-fixation in lichens from fertilization plots using a three-way ANOVA with site, treatment, and species as factors. The N concentration of each incubated lichen was determined after the conclusion of the experiment in finely ground oven-dried lichen tissue using a Perkin-Elmer CHN Analyzer (Perkin-Elmer, Boston, Massachusetts).
A total of 72 lichens were collected from outside of the treatment plots on 2 July, 19 July, and 3 August 2001 to compare acetylene reduction rates between the acidic and non-acidic tundra sites. Six replicates of each species from each site were collected on each of the three dates (3 dates × 2 species × 2 sites factorial design, with 6 replicates). We compared N2-fixation in lichens from the two sites using a three-way ANOVA with site, species, and date as factors.
Results
FERTILIZATION PLOTS: RESPONSE OF PELTIGERA TO EXPERIMENTAL N AND P
The abundance of both P. aphthosa and P. polydactyla was reduced in the N fertilization plots at both the acidic and non-acidic tundra sites (, ). In fact, lichens were virtually eliminated from plots fertilized with N at the acidic site. In contrast, P addition had no effect on lichen abundance at either site (, ). In the analyses of abundance from the treatment plots, P. aphthosa was significantly more abundant at the non-acidic site than at the acidic site, while the abundance of P. polydactyla did not differ between the two sites (, ). However, the area within the treatment plots was likely too small to adequately characterize these sites for lichen abundance, and the site comparison of abundance outside of the treatment plots better represents true site differences (see below).
Rates of N2-fixation in lichens from plots receiving P were significantly greater than in lichens from control plots (, ) on both a per-lichen area and per-lichen biomass basis, although trends were stronger for N2-fixation expressed per unit lichen mass for P. aphthosa (). Because of this similarity, graphs show only the per unit mass data, though the analyses for both are presented ( and ). Thallus N concentration was higher in lichens fertilized by P (, ), which corresponds with higher lichen N2-fixation in plots receiving P. Though we were unable to characterize the response of N2-fixation rates to N fertilization, clearly the contribution of N by Peltigera to the N fertilized plots is not substantial.
SITE COMPARISON: RESPONSE OF PELTIGERA TO AMBIENT N AND P
While 72 lichens were incubated with acetylene, one gas sample, a replicate of P. aphthosa on non-acidic tundra, was lost due to a leak in an incubation chamber. As a result, our analysis is based on the remaining 71 gas samples.
In contrast to our hypothesis, abundance of both species, but especially P. polydactyla, was greater at the acidic site than at the non-acidic site (, ). However, both species of lichens had similar rates of N2-fixation at the two sites (, ). Thus, despite similar rates of per-biomass N2-fixation at the two sites, the contribution of N by N2-fixation in P. aphthosa and P. polydactyla is greater in acidic tundra than in non-acidic tundra. Though N2-fixation rates were significantly higher on 2 July than 19 July and 3 August 2001, there were no significant interactions of date with species or site (), so we present only the seasonal averages in .
Discussion
Our results present an interesting paradox. With P fertilization, the average N2-fixation rate for both species increased from 0.77 ± 0.19 μmoles acetylene g lichen−1 h−1 to 1.65 ± 0.39 μmoles acetylene g lichen−1 h−1. However, N2-fixation rates did not vary between the more P-rich non-acidic site and the acidic site with higher N availability. Furthermore, Peltigera percent cover is threefold greater at the acidic site, with percent cover values of 6% and 2% at the acidic site and non-acidic site, respectively. Thus, while P fertilization approximately doubles the rate of N2-fixation per gram lichen, the areal contribution of N fixed by Peltigera under ambient nutrient conditions is three times less on the more P-rich non-acidic site.
Potential explanations for our contradictory results include soil moisture, soil pH, micronutrient limitation, and the physiological constraints of Peltigera. CitationChapin et al. (1991) measured a positive response of cyanobacterial N2-fixation to P fertilization in a high-arctic lowland, but were unable to measure differing rates of N2-fixation along a natural P gradient. They attributed this result to differences in moisture among the sites that overrode the influence of P on N2-fixation rates. However, the two sites studied here do not differ significantly in soil moisture (L. Gough, unpublished data). There is an obvious difference in pH between these two sites, though the pH optimum for N2-fixation in P. aphthosa is 5–7 (CitationEnglund, 1978), suggesting that rates of N2-fixation would be higher on non-acidic tundra if pH were an important factor influencing in situ rates of N2-fixation there. N2-fixation may be limited by a micronutrient such as iron or molybdenum at the non-acidic site, but this seems unlikely because N2-fixation rates in lichens at that site responded positively to P fertilization. The most likely explanation is that since lichens do not have a root system or other absorptive organs, their nutrient sources are limited to atmospheric deposition (CitationNash and Gries, 1995; CitationHyvärinen and Crittenden, 1998) and nutrients concentrated at the surface of the substrate at their immediate location. The more abundant P at the non-acidic site is concentrated in the mineral soil (CitationHobbie and Gough, 2002). The two lichens studied here are separated from the mineral soil by a layer of organic peat, and may be unable to access mineral soil P. Lichens on acidic tundra may not be inhibited by N because while N mineralization rates are higher at that site, they still represent quite low rates of N availability (CitationHobbie and Gough, 2002).
Our finding that P fertilization stimulated N2-fixation rates and increased lichen N concentrations at both sites and for both species is consistent with the findings of others (CitationChapin et al., 1991; CitationChapin and Bledsoe, 1992; CitationCrittenden et al., 1994; CitationThompson and Vitousek, 1997; CitationVitousek and Field, 1999; but see CitationAlexander et al., 1978). These results support the interpretation that N2 fixers are limited by P in upland tundra as they are in some other ecosystems (CitationVitousek and Howarth, 1991).
Declines in abundance of N2-fixing lichens with N fertilization have been documented previously in the arctic tundra (CitationCornelissen et al., 2001), as well as in the boreal forest (CitationNohrstedt, 1998), where many of the same lichen species are found. The negative effect of N fertilization on lichens likely arises from shading of lichens or burial of lichens under more abundant leaf litter associated with greater plant biomass (CitationCornelissen et al., 2001; CitationVitousek and Field, 1999).
Contrary to our expectation, both P. aphthosa and P. polydactyla are more abundant in acidic than in non-acidic tundra. One possible explanation for these results is that favorable microsites for lichen establishment and growth are more abundant in acidic tundra. For example, we observed that these lichens commonly occur in Sphagnum spp. mats at the acidic site, perhaps because the moss provides a moist, cool microhabitat. Sphagnum spp. is not present on non-acidic tundra; microhabitats well-suited to the two lichens may be generally less abundant at the non-acidic site.
In summary, while P stimulates N2-fixation in P. aphthosa and P. polydactyla in experimental fertilization studies at Toolik Lake, rates of N2-fixation do not differ between lichens from acidic and non-acidic tundra despite differences in N and P availability at the two sites. Since the two lichens are more abundant on acidic tundra, the overall contribution of N2 fixed by lichens is greater on acidic tundra, perhaps contributing to the overall higher N availability and productivity in acidic versus non-acidic tundra. However, further studies are necessary to determine the factors that control the abundance of Peltigera in tundra communities. Such studies will be critical for understanding the contribution of N2-fixing lichens to N budgets of arctic tundra.
FIGURE 1. Percent cover for Peltigera aphthosa and P. polydactyla in control (C), phosphorus (P), and nitrogen (N) treatments on acidic and non-acidic tundra. Quadrats were averaged within plots, and data presented are plot means for each treatment, with standard error bars (n = 4 for acidic tundra or 3 for non-acidic tundra)
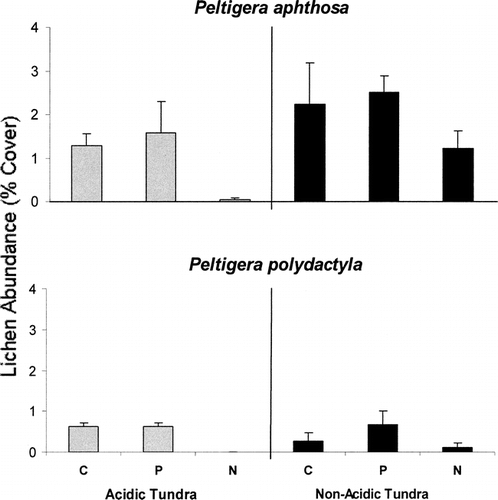
FIGURE 2. Acetylene reduction rates for Peltigera aphthosa and P. polydactyla in control (C) and phosphorus (P) treatments on acidic and non-acidic tundra. Values are means with standard error bars (n = 3)
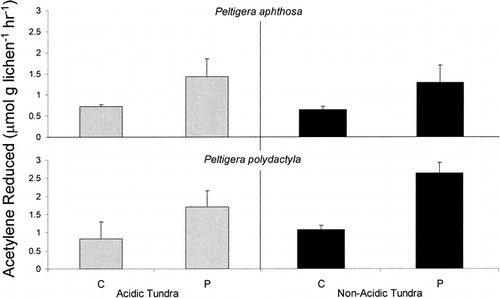
FIGURE 3. Nitrogen concentrations in Peltigera aphthosa and P. polydactyla from control (C) and phosphorus (P) treatments on acidic and non-acidic tundra. Values are means with standard error bars (n = 3)
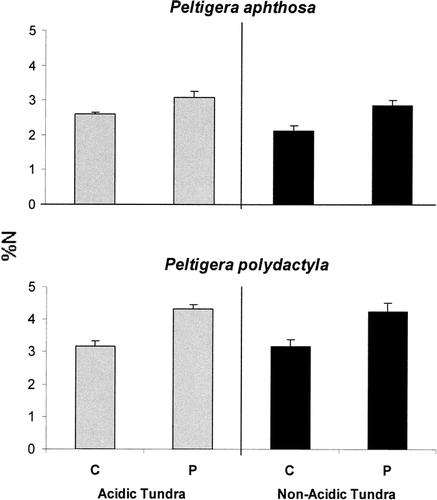
TABLE 1 Three-way ANOVA comparing abundance of Peltigera aphthosa and P. polydactyla between sites, and among control (C), nitrogen (N), and phosphorus (P) treatments, with Tukey's HSD posthoc test. Three-way ANOVA comparing N2-fixation rates per unit area and per unit mass between P. aphthosa and P. polydactyla, acidic and non-acidic tundra, and control and P treatments
TABLE 2 Two-way ANOVA comparing abundance of Peltigera aphthosa and P. polydactyla between moist acidic and moist non-acidic tundra. Three-way ANOVA comparing N2-fixation rates per unit area and per unit mass between P. aphthosa and P. polydactyla, between moist acidic and moist non-acidic tundra, and among the three sampling dates
TABLE 3 Three-way ANOVA comparing lichen N concentration between Peltigera aphthosa and P. polydactyla and between the control (C) and phosphorus (P) treatments at the acidic and non-acidic sites
Acknowledgments
We are grateful to the Toolik Lake Field Research Station and the Toolik Lake Long Term Ecological Research Program for logistic support, and to Kei Koba, Tiffany Miley, Jacques Finlay, Jim Laundre, Gus Shaver, and Lauren McSherry for field assistance and/or use of resources. We also wish to thank two anonymous reviewers who provided helpful comments on the manuscript. This research was supported by a Research Experience for Undergraduates supplement to a grant from the National Science Foundation, OPP-9902695, to S. E. Hobbie. Establishment and maintenance of the fertilization treatments was funded by the Toolik Lake LTER, DEB-9810222.
Notes
Revised ms submitted April 2005
References Cited
- Alexander, V. 1974. A synthesis of the IBP tundra biome circumpolar study of nitrogen fixation. In: Holding, A. J., Heal, O. W., MacLean Jr., S. F., and Flanagan, P. W. (eds.), Soil Organisms and Decomposition in Tundra. Stockholm: Tundra Biome Steering Committee, 109–121.
- Alexander, V. , M. Billington , and D. M. Schell . 1978. Nitrogen fixation in Arctic and Alpine tundra. In: Tieszen, L. L. (ed.), Vegetation and Production Ecology of an Alaskan Arctic Tundra. New York: Springer-Verlag, 539–558.
- Alexander, V. and D. M. Schell . 1973. Seasonal and spatial variation of nitrogen fixation in the Barrow, Alaska tundra. Arctic and Alpine Research 5:77–88.
- Arctic LTER database, 2002. http://ecosystems.mbl.edu/arc. Cited 18 May 2002.
- Barsdate, R. J. and V. Alexander . 1975. The nitrogen balance of Arctic tundra: Pathways, rates, and environmental implications. Journal of Environmental Quality 4:111–117.
- Bliss, L. C. and N. V. Matveyeva . 1992. Circumpolar Arctic vegetation. In: Chapin, F. S. I., Jeffries, R. L., Reynolds, J. F., Shaver, G. R., and Svoboda, J. (eds.), Arctic Ecosystems in a Changing Climate: An Ecophysiological Perspective. San Diego: Academic Press, 59–89.
- Bowman, W. D. , J. C. Schardt , and S. K. Schmidt . 1996. Symbiotic N2 fixation in alpine tundra: Ecosystem input and variation in fixation rates among communities. Oecologia 108:345–350.
- Bret-Harte, M. S. , G. R. Shaver , J. P. Zoerner , J. F. Johnstone , J. L. Wagner , A. S. Chavez , R. F. Gunkelman , S. C. Lippert , and J. A. Laundre . 2001. Developmental plasticity allows Betula nana to dominate tundra subjected to an altered environment. Ecology 82:18–32.
- Chapin, D. M. , L. C. Bliss , and L. J. Bledsoe . 1991. Environmental regulation of nitrogen fixation in a high Arctic lowland ecosystem. Canadian Journal Of Botany 69:2744–2755.
- Chapin, D. M. and C. S. Bledsoe . 1992. Nitrogen fixation in arctic plant communities. In: Chapin, F. S. I., Jeffries, R. L., Reynolds, J. F., Shaver, G. R., and Svoboda, J. (eds.), Arctic Ecosystems in a Changing Climate: An Ecophysiological Perspective. San Diego: Academic Press, 301–319.
- Cornelissen, J. H C. , T. V. Callaghan , J. M. Alatalo , A. Michelsen , E. Graglia , A. E. Hartley , D. S. Hik , S. E. Hobbie , M. C. Press , C. H. Robinson , G. H R. Henry , G. R. Shaver , G. K. Phoenix , D. G. Jones , S. Jonasson , F. S. Chapin , U. Molau , C. Neill , J. A. Lee , J. M. Melillo , B. Sveinbjornsson , and R. Aerts . 2001. Global change and arctic ecosystems: is lichen decline a function of increases in vascular plant biomass?. Journal of Ecology 89:984–994.
- Crews, T. E. 1993. Phosphorus regulation of nitrogen fixation in a traditional Mexican agroecosystem. Biogeochemistry 21:141–166.
- Crittenden, P. D. , I. Kaluka , and E. Oliver . 1994. Does nitrogen supply limit the growth of lichens?. Cryptogamic Botany 4:143–155.
- Englund, B. 1978. Effects of environmental factors on acetylene reduction by intact thallus and excised cephalodia of Peltigera aphthosa Willd. In: Granhall, U. (ed.), Ecological Bulletins: Environmental Role of Nitrogen-fixing Blue-green Algae and Asymbiotic Bacteria. Stockholm, 234–246.
- Gough, L. and S. E. Hobbie . 2003. Responses of moist non-acidic arctic tundra to altered environment: Productivity, biomass, and species richness. Oikos 103:204–216.
- Gunther, A. J. 1989. Nitrogen fixation by lichens in a subarctic Alaskan watershed. Bryologist 92:202–208.
- Hamilton, T. D. 2003. Glacial geology of the Toolik Lake and Upper Kuparuk River region. In: Walker, D. A. (ed.), Biological Papers of the University of Alaska. No. 26. Fairbanks: University of Alaska Printing Services.
- Hardy, R. W F. , R. D. Holsten , E. K. Jackson , and R. C. Burns . 1968. Acetylene-ethylene assay for N2 fixation: Laboratory and field evaluation. Plant Physiology 43:1185–1207.
- Hendzel, L. L. , R. E. Hecky , and D. L. Findlay . 1994. Recent changes of N2 fixation in Lake 227 in response to reduction of the N/P loading ratio. Canadian Journal Of Fisheries And Aquatic Sciences 51:2247–2253.
- Hobbie, S. E. and L. Gough . 2002. Foliar and soil nutrients in tundra on glacial landscapes of contrasting ages in northern Alaska. Oecologia 131:453–462.
- Hyvärinen, M. and P. D. Crittenden . 1998. Relationships between atmospheric nitrogen inputs and the vertical nitrogen and phosphorus concentration gradients in the lichen Cladonia portentosa . New Phytologist 140:519–530.
- Kurina, L. M. and P. M. Vitousek . 1999. Controls over the accumulation and decline of a nitrogen-fixing lichen, Stereocaulon vulcani , on young Hawaiian lava flows. Journal of Ecology 87:784–799.
- Nash, T. H. and C. Gries . 1995. The response of lichens to atmospheric deposition with an emphasis on the Arctic. Science Of The Total Environment 161:737–747.
- Nash, T. H. and A. G. Olafsen . 1995. Climate change and the ecophysiological response of Arctic lichens. The Lichenologist 27:559–565.
- Nohrstedt, H. O. 1998. Residual effects of N fertilization on soil-water chemistry and ground vegetation in a Swedish Scots Pine forest. Environmental Pollution 102:77–83.
- Shaver, G. R. , F. S. Chapin , and B. L. Gartner . 1986. Factors limiting seasonal growth and peak biomass accumulation in Eriophorum vaginatum in Alaskan tussock tundra. Journal of Ecology 74:257–278.
- Thompson, M. V. and P. M. Vitousek . 1997. Asymbiotic nitrogen fixation and litter decomposition on a long soil-age gradient in Hawaiian montane rain forest. Biotropica 29:134–144.
- Vitousek, P. M. 1999. Nutrient limitation to nitrogen fixation in young volcanic sites. Ecosystems 2:505–510.
- Vitousek, P. M. and C. B. Field . 1999. Ecosystem constraints to symbiotic nitrogen fixers: a simple model and its implications. Biogeochemistry 46:179–202.
- Vitousek, P. M. and R. W. Howarth . 1991. Nitrogen limitation on land and in the sea: How can it occur?. Biogeochemistry 13:87–115.
- Walker, D. A. 1998. The Geobotanical Atlas of the Toolik Lake and Kuparuk River Region, Alaska. URL http://www.geobotany.uaf.edu/arcticgeobot/index.html. Cited 9 June 2002.
- Walker, D. A. , N. A. Auerbach , J. G. Bockheim , F. S. Chapin , W. Eugster , J. Y. King , J. P. McFadden , G. J. Michaelson , F. E. Nelson , W. C. Oechel , C. L. Ping , W. S. Reeburg , S. Regli , N. I. Shiklomanov , and G. L. Vourlitis . 1998. Energy and trace-gas fluxes across a soil pH boundary in the arctic. Nature 394:469–472.
- Walker, D. A. , N. A. Auerbach , and M. M. Shippert . 1995. NDVI, biomass, and landscape evolution of glaciated terrain in northern Alaska. Polar Record 31:169–178.
- Walker, M. D. , D. A. Walker , and N. A. Auerbach . 1994. Plant communities of a tussock tundra landscape in the Brooks Range foothills, Alaska. Journal Of Vegetation Science 5:843–866.