Abstract
We have reconstructed a continuous high-resolution penguin population record over the past ∼1300 years from the geochemical and 14C dating data in the ornithogenic lake sediments at Ardley Island in the Antarctic. The concentrations of F, P, Sr, Se, S, Cu, Zn, and Ca in the sediments are notably enriched and significantly correlated with each other, and they have a consistent pattern of change versus depth. These elements, as previously reported, are biomarkers of penguin droppings and population. Over the past ∼1300 years, the penguin population at Ardley Island, as measured by these biomarkers’ concentrations, has a decreasing trend, especially during the past century when the penguin population has dropped steeply. Compared with the paleoclimatic records and glacial advance and retreat history in the study area, the pronounced penguin population changes in the absence of human activities appear to be related to climatic changes. The steep decline of the penguin populations in the past century, however, seems to be caused by the abnormally warming climate in the Antarctic Peninsula.
Introduction
The populations of Antarctic seabirds, especially penguins, have been extensively studied over the last several decades as reliable bio-indicators of the natural and environmental variability in the Southern Ocean marine ecosystem (e.g., CitationFraser et al., 1992; CitationKaiser, 1997; CitationTatur et al., 1997; CitationSmith et al., 1999; CitationCroxall et al., 2002; CitationWeimerskirch et al., 2003). A number of penguin monitoring programs in the Antarctic ecosystems are currently underway and are expected to provide data for studying penguins' response to climatic and environmental variability. Currently available data and evidence, however, are not very consistent with each other and remain equivocal and debatable, and one of the major reasons is the difficulty in obtaining long-term and continuous in situ data (CitationCroxall et al., 2002; CitationWeimerskirch et al., 2003; CitationZhu et al., 2005).
Abandoned penguin colonies have become an important way for estimating historical penguin populations and for other paleoenvironmental research. The penguin relics in these colonies, marked by the presence of nest stones, ornithogenic soils, food, and chicks, can provide considerable information about the past population, diet, and migration of penguin species (CitationBaroni and Orombelli, 1994; CitationEmslie et al., 1998; CitationEmslie and McDaniel, 2002; CitationPolito et al., 2002). Furthermore, these data can be compared with other paleoclimatic records to determine whether the population changes are related to climate change. These older relics, however, are generally not well preserved and difficult to obtain. For example, the old ornithogenic soil surfaces containing penguin remains have possibly been entirely eroded and scoured by glacial advances, or dissolved in the wetter climatic zone of maritime Antarctica (CitationTatur et al., 1997; CitationEmslie, 2001).
In more recent studies, CitationSun et al. (2000, Citation2001a, Citation2004) reported that the remnants of ancient penguin droppings in the lake sediments near penguin colonies can be identified by their geochemical characteristics and provide continuous information about historical penguin population changes. In this paper, we performed detailed geochemical analyses on an ornithogenic lake sediment core from Ardley Island in the Antarctic in order to reconstruct a continuous, high-resolution record of the penguin population on that island over the past ∼1300 yr B.P.
Study Area
Ardley Island (62°13′S, 58°56′W), declared as an Antarctic Specially Protected Area by the Protocol on Environmental Protection to the Antarctic Treaty and ratified in 1991, is 2.0 km in length and 1.5 km in width (). The island is about 500 m east of the coast of Fildes Peninsula, Maxwell Bay, King George Island, and connected to the Fildes Peninsula through a sandy dam. The Great Wall Station of China is located about 0.5 km to the west (). The study area has a cold oceanic climate, characteristic of maritime Antarctica. According to the meteorological records from the Great Wall Station, the mean annual precipitation is about 630 mm, the annual average relative humidity is about 90%, and the mean annual air temperature is around −2.6°C with a winter low at −26.6°C and a summer high at 11.7°C. It is free of snow and ice during the summer. Geologically, it consists mainly of Tertiary andesitic and basaltic lavas and tuffs together with raised beach terraces. The topography is plain, with the highest elevation at 70 m, and 70% of Ardley Island is covered by vegetation.
Ardley Island is one of the most important penguin colonies in the maritime Antarctic region. The site has the largest concentration of Gentoo penguins (Pygoscelis papua) within the South Shetland Islands. The average number of breeding pairs is estimated at about 4000. In addition, there are also about 1200 breeding pairs of Adélie penguins (Pygoscelis adeliae) and a small number of Chinstrap penguins (Pygoscelis antarctica) (cited from CitationSun and Xie, 2001). The inter-season fluctuation in the numbers and the breeding success of each penguin species is available from modern census data collected since 1979. In addition to these census data, ancient penguin waste products, accumulated in small and shallow lakes on the island, have recently been proposed as a means to examine ancient penguin populations and paleoclimate (CitationSun et al., 2000). In the catchment area of the investigated Y4 lake, there are some abandoned colonies of penguins, which are a potential eutrophying factor in this area (CitationTatur and Myrcha, 1984); the penguin droppings probably have been transferred and deposited into the lake by ice or snowmelt water.
Materials and Methods
SAMPLING
A lake sediment core (Y4), 34 cm long, was collected by driving PVC pipe, 12 cm in diameter, into the soft substrate of the Y4 lake floor at an altitude of 28 m during the austral summer of 1999/2000. For the purpose of comparison, we also collected a 66-cm-long weathered sediment sequence from the front margin of Nelson Ice Cap (as marked by N1 in ), a 67.5-cm-long sediment core (named as Y2) amended by penguin droppings, and a 54-cm-long lake core from a lake named G using the same method. All the sampling sites are shown in . These sediment cores were transported directly to the home laboratory without slicing, and were preserved in cold storage prior to being analyzed. In the laboratory, continuous subsampling for elemental analyses was done in 2-cm slices for the sediment core Y4. The lithologic section is illustrated in . This sediment core discharges an unpleasant smell, and some ancient penguin bones, identified with reference to modern penguin bones from the Fildes Peninsula on the King George Island, are well preserved in it (shown in ). For the sediment core N1 and Y2 and the Xihu lacustrine sediments, detailed information about their sediment patterns and geochemical characteristics have been described by CitationLiu et al. (2004), CitationSun et al. (2000, Citation2001a), and CitationZhao (1991), respectively.
ELEMENTAL ANALYSIS
All the Y4 subsamples were ground to 200 mesh size after drying in open air. About 0.1–0.5 g of each powder sample was taken, precisely weighed, and then digested by multi-acid in a Pt crucible with electric heating. The digested samples were analyzed for the following 24 elements: Si, Al, Fe, S, F, Sr, Ba, P, Ti, Cr, V, As, Se, K, Na, Ca, Mg, Mn, Pb, Ni, Cu, Zn, Ga, and Ge. Concentrations of Si, Al, and Fe2O3 were determined by various wet chemical methods. FeO was measured by the potassium dichromate volumetric method. S was analyzed by the KI volume method after combustion in a SRJK-2 high-temperature furnace. F was measured by ion selective electrode (ISZ). Trace elements Sr and Ba were determined using inductively coupled plasma-atomic emission spectrometry (ICP-AES) (model Atom Scan Advantage from Thermo Elemental) after digestion by aqua regia/HF/HClO4. Abundance of P2O5, TiO2, Cr, and V was determined by ultraviolet visible spectrophotometry (UVS). Concentrations of As and Se were determined by atomic fluorescent hydrogenation (AFS) (XDY-1 atomic fluorescent spectrometry). Atomic absorption spectrophotometry (AAS) (model PZ-1100) was used to determine K, Na, Ca, Mg, Mn, Ni, Cu, Zn, Pb, and Ge. Precision and accuracy of our results was monitored by analyzing standard sediment reference materials with study samples in every batch of analysis. The analytical values for the major elements and trace elements are within ±0.5% and ±5% of the certified ones, respectively.
RADIOMETRIC AND RADIOCARBON ANALYSIS
Conventional radiocarbon dating was carried out on two samples of bulk sediments from the core Y4. For the sediment core G, conventional radiocarbon dating was performed on one sample of moss-rich sediments as well as handpicked moss remains (Amblystegiaceae, Drepanocladus aduncus) at four different depths (). Using tweezers and a needle, the moss materials were separated under a binocular microscope, and mechanically cleaned from other sediment particles. 14C measurement was conducted on organic carbon components by using a Quantalas-1220 liquid scintillometer, after pretreatment with dilute HCl to remove the minute content of carbonate which may have been included in the samples. The conventional radiocarbon ages were reported here as B.P. (before present, conventionally before 1950). The quoted errors in the dates are based on the reproducibility of measurement.
For the G sediment core, the top 10 cm of sediments were sectioned into 10 samples at 1-cm intervals. About 3 g of each subsample was dried and then ground to fine powder in the laboratory. The analysis for recent radionuclides (210Pb, 226Ra, and 137Cs) of these subsamples was performed by direct gamma spectrometry using Ortec HPGe GWL series, well-type, coaxial, low background, intrinsic germanium detectors. 210Pb activity was determined via its gamma emissions at 46.5 keV, and 226Ra by the 295 and 352 keV γ-rays emitted by its daughter isotope 214Pb following 3 weeks storage in sealed containers to allow radioactive equilibration. 137Cs was measured by its emissions at 662 keV. The absolute efficiencies of the detectors were determined using calibrated sources and sediment samples of known 210Pb activity. Corrections were made for the effect of self-absorption of low energy γ-rays within the sample. Supported 210Pb in each sample was assumed to be in equilibrium with in situ 226Ra. Unsupported 210Pb activity at each depth was calculated by subtracting 226Ra activity from total 210Pb activity. A more detailed description of the equipment and method was given by CitationWu et al. (2004). In this study, both the radiometric and conventional radiocarbon dating were performed at Nanjing Institute of Geography & Limnology, Chinese Academy of Sciences (CAS).
Results and Discussion
CHRONOLOGY
As reviewed by CitationBjörck et al. (1991a) and CitationIngólfsson et al. (1998), there are a number of problems associated with dating Antarctic lacustrine deposits that can result in inaccurate chronologies. In particular, marine sources can contaminate freshwater sediments, and therefore dates can be influenced by the Antarctic marine reservoir effect, which yields older radiocarbon dates (CitationHodgson et al., 2001). As discussed in this section, marine material from the input of penguin droppings contaminated lake Y4, and thus the radiocarbon dates for the bulk organic carbon in the sediments require a reservoir correction. However, the reservoir effect for marine-originated penguin droppings in the study area is unknown to date. Because there was no terrestrial material suitable for 14C dating in the Y4 lake sediments, we only determined two conventional 14C dates on bulk organic materials from the surface and base of the core Y4 (). Assuming that the surface date could be used to estimate the local marine reservoir effect (CitationMcMinn, 2000; CitationHodgson et al., 2001), the base date can be reasonably corrected by subtracting the surface from it to give the reservoir-corrected date of about 1300 yr B.P. We cannot establish a more high resolution chronology for this core since no other radiocarbon dates were available in the present study.
The adjacent lake G contains large amounts of aquatic mosses. Since moss remains in lake sediments can give the most reliable 14C ages in the Antarctic Peninsula (CitationBjörck et al., 1991a, Citation1991b), ages of mosses in lake G from conventional radiocarbon dating were taken to be those of the sediments.
As shown in , 137Cs is present in the uppermost 3-cm sediment layer of the lake G core, with a peak activity at the depth of 1.5 cm, likely indicating that the 1965 fallout maximum from the atmospheric testing of nuclear weapons pertains to this depth (CitationAppleby et al., 1995). Significant levels of unsupported 210Pb were detected in the top 5 cm, and the total 210Pb activities were in equilibrium with the 226Ra activity below 6 cm, which produces supported 210Pb. The oldest 210Pb-datable sediments in the core are located at 4.5 cm and correspond to an age of 134 yr B.P. based on a constant rate of supply (CRS) dating model (CitationAppleby et al., 1995). Further information on 210Pb and 137Cs chronology has been published elsewhere (CitationSun et al., 2001b).
Five samples from the lake G core were measured for radiocarbon dates, out of which four are aquatic mosses (Amblystegiaceae, Drepanocladus aduncus) extracted from the bulk sediments, and one is moss-rich sediments (G-08 listed in ). All the radiocarbon dates were transformed into calibrated radiocarbon years before present (cal. B.P.) using the INTCAL98 calibration data set (CitationStuiver et al., 1998) in the CALIB 4.4 program (CitationStuiver and Reimer, 1993); the results are shown in . All the 14C dates, the 137Cs peak, and the oldest 210Pb date are illustrated in versus depth. As can be seen from , the radiocarbon date of the moss-rich sediment sample G-08 is apparently too old in comparison with the 210Pb dating results, and this is consistent with the results of CitationBjörck et al. (1991a, Citation1991b) and CitationShen et al. (1998), who suggested that the dates on aquatic moss samples were more reliable than those of the bulk sediments themselves. If G-08 is removed from the age-depth modeling, we see a good correlation between the dates and the sediment depth with a slope or average sedimentation rate of 0.027 cm a−1, in good agreement with 0.029 cm a−1 of the adjacent lake Y2 as reported by CitationSun et al. (2000). Because the lakes Y2, G, and Y4 are in the same geographical area and have similar limnological features, it is reasonable to believe that they should have consistent average sedimentation rates. As shown in , the basal date for the sediment core Y4 (sample Y4-34) is in line with the age-depth model of the G lake sediment profile. Therefore, we could use this age-depth model to establish the chronology of the sediment core Y4.
GEOCHEMICAL CHARACTERISTICS
According to the results of CitationSun et al. (2000, Citation2001a, Citation2004), nine “bio-elements”—sulfur (S), phosphorus (as P2O5), calcium (as CaO), copper (Cu), zinc (Zn), selenium (Se), strontium (Sr), barium (Ba), and fluorine (F)—were enriched and significantly correlated with each other in the soils or sediments amended by penguin guanos. These element levels in the sediments or soils impacted by penguin guanos are significantly higher than those in the unaffected ones (CitationTatur et al., 1997; CitationSun et al., 2000; CitationLiu et al., 2004). They have not been reported together in high concentration in any organic material except penguin droppings, and they were typical elements of penguin guanos. Without remnants of penguin guanos, these elements cannot be accumulated together since their chemical properties are very different (CitationSun et al., 2000, Citation2001a; CitationSun and Xie, 2001). Moreover, these typical elements from penguin guanos are almost immobile in the sediments of Antarctic lakes and depressions, according to CitationSun et al. (2000, Citation2001a). Therefore, the assemblage of P, Sr, Cu, Zn, Se, Ca, F, Ba, and S is an important geochemical marker for penguin droppings or the soils and sediments impacted by them in the maritime Antarctic.
gives the arithmetic mean, variable range, and coefficient of variation (CV) for the chemical concentrations of elements in the Y4 lake sediments. For comparative purposes, the average element concentrations in the local bedrock, N1 proglacial sediments, Y2 lake sediments, and Xihu lacustrine sediments are also given in this table. As seen from the , the mean concentrations of major elements, with the exception of Ca, in Y4 and Y2 were obviously lower than those in local bedrock and N1, but the concentrations of the minor elements, such as Zn, Cu, P, S, Se, Sr, and F, in Y4 and Y2 were much higher. These significant differences in the mean element concentrations among the local bedrock, N1, Y2, Y4, and Xihu lake sediments may be attributed to their different sediment patterns. The Y2 lake sediments were found to be impacted by penguin droppings, thus resulting in remarkable enrichment of S, P, Ca, Cu, Zn, Se, Sr, Ba, and F and significant intercorrelations (CitationSun et al., 2000, Citation2001a; CitationSun and Xie, 2001). The Xihu lacustrine sediments were not amended by penguin droppings, but affected by biogenic materials such as moss, lichens, etc. (CitationZhao, 1991). The N1 proglacial sediments were not affected by penguin droppings or by biological action, and they were considered to be the naturally weathered products of the local bedrocks (CitationLiu et al., 2004). The Y4 lake sediments, having remarkably higher concentrations of F, P, Sr, Se, S, Cu, Zn, and Ca, are apparently similar to the Y2 lake sediments and very likely impacted by penguin droppings.
The impact of penguin droppings on Y4 is also supported by the vertical concentration profiles of major and minor elements versus depth and the results of clustering and correlation analysis. As illustrated in , the vertical distributions of F, P, Sr, Se, S, Cu, Zn, and Ca concentrations display almost the same pattern, but opposite that of lithophile elements such as Na, K, Al, Mg, Ti, Si, Fe, etc. The results of R-clustering performed on the intercorrelation coefficients of 24 element concentrations in Y4 are given in . As seen from the dendrogram, Sr, Cu, P, Zn, S, F, Ca, Se, As, Mn, and Cr belong to the same group, and the rest of the elements belong to the other group. A Pearson correlation analysis also gave significantly positive correlations among the concentrations of Sr, Cu, P, Zn, S, F, Ca, and Se in Y4 (). All these results indicated that Sr, Cu, P, Zn, S, F, Ca, and Se are likely to be of the same origin.
As indicated above, Antarctic penguin droppings are notably rich in P, Sr, Ba, Cu, Zn, Se, Ca, F, and S, and the assemblage of these bio-elements is an important geochemical marker or biomarker for the lake sediments impacted by penguin droppings or guano soil (CitationSun et al., 2000, Citation2001a; CitationSun and Xie, 2001). For Y4, nevertheless, Ba does not belong to this assemblage probably due to the difference of its geochemical behavior in Y2 and Y4. In Y2, Ba was considered to be associated with a bio-source, but in Y4, it seems to be a lithogenic element. gives the correlation coefficients of Ba and other elements in Y4 and shows that Ba has significantly positive correlations with lithophile elements such as Na, K, Fe, Al, Si, and V, but negative with P, Cu, Zn, Se, Ca, F, and S. Further investigations suggested that Ba is mainly controlled by minerals such as potassium feldspars. As illustrated in , there is a strong correlation between K and Ba concentrations. This correlation may be attributed to the mineralogical control of K-feldspar as a result of poor chemical weathering processes under extremely low temperatures of the Antarctic (CitationLiu et al., 2004), since K-feldspar is the main carrier for these elements (CitationKim et al., 1998). Furthermore, we found excellent correlation between Ba/Mg versus Al/Mg ratios (), indicating the presence of feldspars (CitationKim et al., 1999).
Therefore, the assemblage of P, Cu, Sr, Zn, Se, Ca, F, and S is the biomarker of the amendment by penguin droppings or guano soil in Y4. Penguin rookeries are present around the lake Y4, and its excreta are probably deposited in the lake by catchment snow-melt or directly in the water, as evidenced by the presence of ancient penguin bones in the sediments. The input of penguin droppings enriched the Y4 sediments with the bio-elements of P, Cu, Sr, Zn, Se, Ca, F, and S and resulted in the dilution of the lithophile elements. Additionally, as shown in , the contents of the bio-elements in Y4 are much higher than those in Y2, probably indicating a greater influence of penguin droppings.
RECONSTRUCTION OF HISTORICAL PENGUIN POPULATION OVER THE PAST ∼1300 YEARS
With the evidences that geochemical characteristics of Y4 are controlled mainly by the influence of penguin droppings or guano soil, Q-mode factor analysis (CitationSun et al., 2000), a method for decomposing multiple factors, was utilized to analyze the concentration of P, Cu, Sr, Zn, Se, Ca, F, and S in Y4. The results revealed that more than 99% of the variance in the data could be explained by two factors (51.54% for factor1 and 48.43% for factor2). As illustrated in , the change pattern of the first factor is very close to the profiles of the bio-element concentrations versus depth. Therefore, this factor can be used to measure the input of penguin droppings in the sediments and thus penguin population for the last ∼1300 years. The profile of factor 1, as given in , shows that the historical penguin population, while relatively stable, has a decreasing trend. Two troughs appear around 1000 yr B.P. and during the period of ∼450–200 yr B.P. Especially, in the past century, the penguin population declined steeply, and the trend still continues. This is consistent with the recently documented decreases in the total number of penguins on Ardley Island (citing from CitationXie, 2001). The trough of ∼450–200 yr B.P., as shown in , is comparatively shallow, but the t-test result showed that there existed significant differences between the data within the period of ∼450–200 yr B.P. and the rest (t: −3.85; p: 0.00093; confidence level: 95%). Therefore, we suggested that the fluctuation of the factor 1 curve during the period of ∼450–200 yr B.P. did represent a significant decline of the penguin population.
What is responsible for the declining penguin population? In order to answer this question, we tentatively compared the relative penguin populations with the paleoclimatic records and glacial advance and retreat history. According to the work of CitationSun et al. (2000), CitationSun and Xie (2001), CitationXie (2001), and CitationZhao (1991, Citation1997), the period of 1100–1300 yr B.P. was characterized by warm and humid climatic conditions with relatively high precipitation. After that, there was a glacial advance at about 1000 yr B.P. in the South Shetland Islands (CitationBarsch and Mäusbacher, 1986), and this is in agreement with the decreasing penguin populations around 1000 yr B.P.
The trough of the relative penguin population from ∼450 to 200 yr B.P. coincides well with the onset of the Little Ice Age. The Little Ice Age, a widespread cooling climate event that also occurred on the Antarctic Peninsula, has been supported by evidence from ice cores, marine sediments, lake sediments, and penguin occupation history (CitationKreutz et al., 1997; CitationGoodwin, 1998; CitationEmslie et al., 1998; CitationSmith et al., 1999; CitationEmslie and McDaniel, 2002). The ice-core and oxygen isotope record from Siple station indicate that the Antarctic Peninsula did experience colder climates than today during the Little Ice Age (CitationEmslie, 2001; citing CitationMosley-Thompson et al., 1990). CitationEmslie et al. (1998), based on the investigation of the penguin occupation history on the Antarctic Peninsula, suggested that a brief gap occurred during the period of the Little Ice Age (A.D. 1500–1850). During climatic deterioration, Ardley Island could have been covered with more ice or a glacier, making it harder for penguins to nest and breed and thus leading to a reduction in the populations of breeding penguins (CitationSmith et al., 1999; CitationEmslie et al., 1998; CitationEmslie, 2001; CitationEmslie and McDaniel, 2002). Other causes for the reduction of penguin populations may include changes in marine food availability and/or sea-ice conditions that precluded breeding penguins from existing in the study area (CitationFraser et al., 1992), since lower surface air temperature could increase sea ice extent in the Antarctic Peninsula (CitationSmith et al., 1999).
With the available data in the present study, the cause for the significant decrease in the penguin population over the past ∼100 years, however, cannot be fully understood. According to reliable climatic records and other evidences, the recent warming on the Antarctic Peninsula has resulted in a profound climatic change, an order of magnitude greater than global mean warming (CitationFraser et al., 1992; CitationStark, 1994; CitationKing, 1994; CitationTurner et al., 2002). This abnormally warming climate period may hold more moisture and cause heavier snowfall (CitationKaiser, 1997). Moreover, as discussed by Zhu et al. (unpublished data), the north, north-west, and west winds are more frequent than the east wind on Ardley Island; therefore, more snow should have accumulated on the northwest, west, and southwest sides of the island over the past ∼100 years, making it harder for penguins to breed. Therefore, we speculated that the sharp reduction of penguin populations in the past century in the catchment of Y4 lake is possibly due to interactions between the effects of increased snow deposition and decreasing egg and/or chick survival due to predation and flooding (CitationKaiser, 1997; CitationPatterson et al., 2003). To confirm this hypothesis, however, sampling of additional sediment cores from the northwest, west, and southwest sides of the Ardley Island is apparently necessary.
CONCLUSIONS
Compared with the local bedrock, natural weathered sediments, Xihu lake sediments, and ornithogenic lake sediments, the Y4 lake core from the Antarctic Ardley Island has a distinct geochemistry, characterized by notably high concentrations of F, P, Sr, Se, S, Cu, Zn, and Ca and significantly positive inter-correlation. The vertical distribution patterns of F, P, Sr, Se, S, Cu, Zn, and Ca concentrations are almost identical, but opposite to those of lithophile elements such as Na, K, Al, Mg, Ti, Si, Fe, etc. The assemblage of P, Cu, Sr, Zn, Se, Ca, F, and S suggests that the Y4 lake sediments have been impacted by penguin droppings or guano soil, and this is further confirmed by the presence of well-preserved penguin bones in the sediments. Using Q-mode factor analysis, we have determined the factor responsible for the input of penguin droppings, and its changes are considered to be representative of the population dynamics of penguin species. Our results show that the penguin population on the Ardley Island over the past ∼1300 years, while relatively stable, has a decreasing trend, especially in the past century, when the penguin population declined steeply. The comparison between the historical penguin population changes in Y4 and the paleoclimatic records suggests that the decline, prior to the past two centuries, is likely due to climatic deterioration. The cause for the significant decrease in penguin population over the past ∼100 years, however, remains to be further studied.
FIGURE 1. Studied area and sampling sites for the cores Y4, N1, and Y2. In the top panel, marker A shows lakes, marker B shows network of meltwater channels. In the bottom panel, the contour interval is 10 m
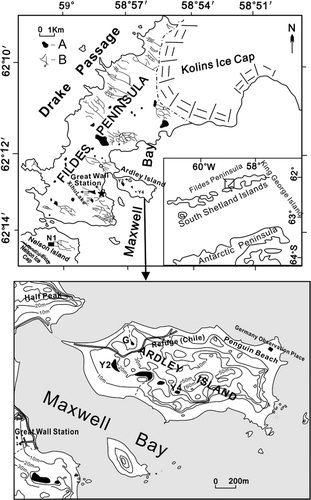
FIGURE 2. (a) Sediment lithostratigraphy for the core Y4; (b) 210Pb, 137Cs versus depth in the sediment core G. Highest peak value of 137Cs corresponds to the 1965 fallout maximum from the atmospheric testing of nuclear weapons, in good agreement with the result of CRS 210Pb model (CitationSun et al., 2001b). (c) Linear depth-age model for the core G using the radiometric and calibrated ages, except one with an age of 666 cal. yr B.P. (G-08). The linear sedimentation rate (S) is 0.027 cm a−1
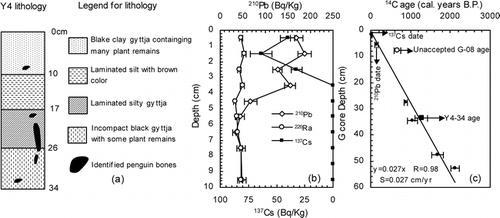
FIGURE 6. The change of penguin populations during the past ∼1300 years obtained by Q-factor analysis for the sediment core Y4 on the Ardley Island. The factor 2 is opposite to the factor 1; variation in loadings of factor 1 represents the variation in penguin population during the past 1300 years. At the top of the graph, the climatic optimum is based on the work of CitationSun et al. (2000), CitationSun and Xie (2001), CitationXie (2001), and CitationZhao (1991, Citation1997); the period of glacial advance is from CitationBarsch and Mäusbacher (1986); the Little Ice Age is derived from the work of CitationKreutz et al. (1997), CitationGoodwin (1998), CitationEmslie et al. (1998), CitationSmith et al. (1999), and CitationEmslie and McDaniel (2002). The abnormally warming climate period is from the work of CitationFraser et al. (1992), CitationStark (1994), CitationKing (1994), and CitationTurner et al. (2002). With regard to the correlation between the climatic change and penguin population fluctuation, see text for detailed discussion
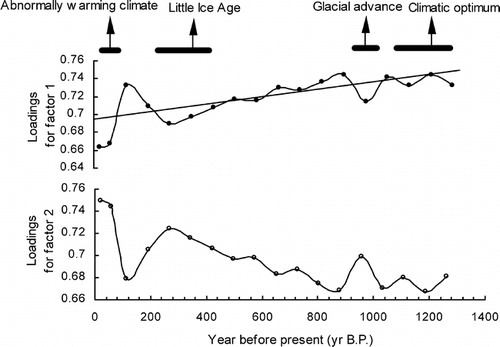
TABLE 1 14C conventional and calibrated ages of the samples from G and Y4 lake sediment cores
TABLE 2 Chemical element concentrations in the proglacial sediments (N1), the local bedrocks, and the lake sediments Y4, Y2, and Xihu
TABLE 3 The correlation coefficients between the eight elements in the Y4 lake sediments
TABLE 4 The correlation coefficients between element Ba and lithological elements and bio-elements in the Y4 lake sediments
Acknowledgments
We would like to thank Polar Office of National Oceanic Bureau of China for support and assistance. This study was supported by the National Natural Science Foundation (grant no. 40476001 and 40231002), China Postdoctoral Science foundation, K. C. Wang Educational Foundation, Hong Kong, and the Commonweal Science Foundation from Ministry of Science and Technology (no. 2002DEA30027-9). We thank two anonymous reviewers for their constructive comments to improve this manuscript.
Notes
Revised ms submitted March 2005
References Cited
- Appleby, A. G. , V. J. Jones , and J. C. Ellis-Evans . 1995. Radiometric dating of lake sediments from Signy Island (maritime Antarctic): evidence of recent climatic change. Journal of Paleolimnology 13:179–191.
- Baroni, C. and G. Orombelli . 1994. Holocene glacier variations in the Terra Nova Bay area (Victoria Land, Antarctica). Antarctic Science 6:497–505.
- Barsch, D. and R. Mäusbacher . 1986. New data on the relief development of the Southern Shetland Islands, Antarctica. Interdisciplinary Science Review 11:211–218.
- Björck, S. , C. Hjort , O. Ingolfsson , and G. Skog . 1991a. Radiocarbon dates from the Antarctic Peninsula region—problems and potential. In Lowe, J. J. (ed.), Radiocarbon dating: recent applications and future potential . Quaternary Proceedings 1:55–65.
- Björck, S. , N. Malmer , C. Hjort , P. Sandgren , O. Ingolfsson , B. Wallen , R. I L. Smith , and B. L. Jonsson . 1991b. Stratigraphic and paleoclimatic studies of a 5500-year-old moss bank on elephant island, Antarctica. Arctic and Alpine Research 23:361–374.
- Croxall, J. P. , P. N. Trathan , and E. J. Murphy . 2002. Environmental change and Antarctic seabird populations. Science 279:1510–1514.
- Emslie, S. D. 2001. Radiocarbon dates from abandoned penguin colonies in the Antarctic Peninsula region. Antarctic Science 13:289–295.
- Emslie, S. D. and J. D. McDaniel . 2002. Adelie penguin diet and climate change during the middle to late Holocene in northern Marguerite Bay, Antarctic Peninsula. Polar Biology 25:222–229.
- Emslie, S. D. , W. R. Fraser , R. C. Smith , and W. Walker . 1998. Abandoned penguin colonies and environmental change in the Palmer Station areas, Anvers Island, Antarctic Peninsula. Antarctic Science 3:257–268.
- Fraser, W. R. , W. Z. Trivelpiece , D. G. Ainley , and S. G. Trivelpiece . 1992. Increases in Antarctic penguin populations: reduced competition with whales or a loss of sea ice due to environmental warming?. Polar Biology 11:525–531.
- Goodwin, I. D. 1998. Did changes in Antarctic ice volume influence late Holocene sea-level lowering?. Quaternary Science Reviews 17:319–332.
- Hodgson, D. A. , P. E. Noon , W. Vyverman , C. L. Bryant , D. B. Gore , P. Appleby , M. Gilmour , E. Verleyen , K. Sabbe , V. J. Jones , J. C. Ellis-Evans , and P. B. Wood . 2001. Were the Larsemann Hills ice-free through the Last Glacial Maximum?. Antarctic Science 13:440–454.
- Ingólfsson, O. , C. Hjort , P. A. Berkman , S. Bjorck , E. Colhoum , I. D. Goodwin , B. Hall , K. Hcrakawa , M. Melles , P. Moller , and M. L. Prentice . 1998. Antarctica glacial history since the Last Glacial Maximum: an overview of the record on land. Antarctic Science 10:326–344.
- Kaiser, J. 1997. Is warming trend harming penguins?. Science 276:1790.
- Kim, G. , H. Yang , and Y. Kodama . 1998. Distribution of transition elements in the surface sediments of the Yellow Sea. Continental Shelf Research 18:1531–1542.
- Kim, G. , H. S. Yang , and T. M. Church . 1999. Geochemistry of alkaline earth elements (Mg, Ca, Sr, Ba) in the surface sediments of the Yellow Sea. Chemical Geology 153:1–10.
- King, J. C. 1994. Recent climate variability in the vicinity of the Antarctic Peninsula. International Journal of Climatology 14:357–369.
- Kreutz, K. J. , P. A. Mayewski , L. D. Meeker , M. S. Twickler , S. I. Whitlow , and I. I. Pittalwala . 1997. Bipolar changes in atmospheric circulation during the Little Ice Age. Science 277:1294–1296.
- Liu, X. D. , L. G. Sun , and X. B. Yin . 2004. Textural and geochemical characteristics of proglacial sediments: a case study in the foreland of Nelson Ice Cap, Antarctica. Acta Geologica Sinica 78:970–981.
- McMinn, A. 2000. Late Holocene increase in sea ice extent in fjords of the Vestfold Hills, eastern Antarctica. Antarctic Science 12:80–88.
- Mosley-Thompson, M. , L. G. Thompson , P. M. Grootes , and N. Gundestrup . 1990. Little Ice Age (Neoglacial) paleoenvironmental conditions at Siple Station, Antarctica. Annals of Glaciology 14:199–204.
- Patterson, D. L. , A. L. Easter-Pilcher , and W. R. Fraser . 2003. The effects of human activity and environmental variability on long-term changes in Adélie penguin populations at Palmer Station, Antarctica. In Huiskes, A. H. L., Gieskes, W. W. C., Rozema, J., Schorno, R. M. L., Van der Vies, S. M., and Wolff, W. J. (eds.), Antarctic Biology in a Global Context. Leiden, The Netherlands: Backhuys Publishers, 301–307.
- Polito, M. , S. D. Emslie , and W. Walker . 2002. A 1000-year record of Adélie penguin diets in the Southern Ross Sea. Antarctic Science 14:327–332.
- Shen, C. D. , T. S. Liu , W. X. Yi , Y. M. Sun , M. T. Jiang , J. Beer , and G. Bonani . 1998. C-14 dating of terrestrial moss in Tern Lake deposits, Antarctica. Radiocarbon 40:2 849–854.
- Smith, R. C. , D. Ainley , K. Baker , E. Domack , S. Emslie , B. Fraser , J. Kennett , A. Leventer , E. M. Thompson , S. Stammerjohn , and M. Vernet . 1999. Marine ecosystem sensitivity to climate change. Bioscience 49:393–404.
- Stark, P. 1994. Climatic warming in the central Antarctic Peninsula area. Weather 49:215–220.
- Stuiver, M. , P. J. Reimer , E. Bard , J. W. Beck , G. S. Burr , K. A. Hughen , B. Kromer , F. G. McCormac , J. van der Plich , and M. Spurk . 1998. INTCAL98 radiocarbon age calibration, 24,000–0 cal B.P. Radiocarbon 40:1041–1083.
- Stuiver, M. and P. J. Reimer . 1993. Extended 14C data-base and revised CALIB 3.0 14C age calibration program. Radiocarbon 35:215–230.
- Sun, L. G. and Z. Q. Xie . 2001. Relics: penguin population programs. Science progress 84:31–44.
- Sun, L. G. , Z. Q. Xie , and J. L. Zhao . 2000. A 3,000-year record of penguin populations. Nature 407:858.
- Sun, L. G. , Z. Q. Xie , and J. L. Zhao . 2001a. The sediments of lake on the Ardley Island, Antarctica: identification of penguin-dropping soil. Chinese Journal of Polar Science 12:1 1–8.
- Sun, L. G. , Z. Q. Xie , J. L. Zhao , and L. Xiang . 2001b. 210Pb, 137Cs dating of lake sediments from Ardley Island (maritime Antarctic) and their environment implications. Chinese Journal of Lake Sciences 13:93–96. (in Chinese with English Abstract).
- Sun, L. G. , R. B. Zhu , X. B. Yin , X. D. Liu , Z. Q. Xie , and Y. H. Wang . 2004. A geochemical method for the reconstruction of the occupation history of a penguin colony in the maritime Antarctic. Polar Biology 27:670–678.
- Tatur, A. and A. Myrcha . 1984. Ornithogenic soils on King George Island (maritime Antarctic zone). Polish Polar Research 5:31–60.
- Tatur, A. , A. Myrcha , J. Fabiszewaki , and J. Niegodzisz . 1997. Formation of abandoned penguin colony ecosystem in maritime Antarctic. Polar Biology 17:405–417.
- Turner, J. , J. C. King , T. A. Lachlan-Cope , and P. D. Jones . 2002. Recent temperature trends in the Antarctic. Nature 418:291–292.
- Weimerskirch, H. , P. Inchausti , C. Guinet , and C. Barbraud . 2003. Trends in birds and seal populations as indicators of a system shift in the Southern Ocean. Antarctic Science 15:249–256.
- Wu, J. L. , M. K. Gagan , X. Z. Jiang , W. L. Xia , and S. M. Wang . 2004. Sedimentary geochemical evidence for recent eutrophication of Lake Chenghai, Yunnan, China. Journal of Paleolimnology 32:85–94.
- Xie, Z. Q. 2001. Lake sediment and change of ecological environment of penguin on Ardley Island in Antarctica. Ph.D. thesis, University of Science and Technology of China, 96 pp. (in Chinese with English abstract).
- Xie, Y. Y. , P. Guan , and S. J. Yang . 1992. Geochemistry of sediments and environment in Xihu Lake of Great Wall Station of China, Antarctica. Science in China (Ser. B) 35:758–768.
- Zhao, J. L. 1991. The characteristics of the modern environmental geochemistry and natural environmental evolution in the region of Antarctic Great Wall Station. Beijing: Science Press, 91 pp. (in Chinese with detailed English summary).
- Zhao, J. L. 1997. Climatic changes in the regions of Antarctic Great Wall Station, Southern Chile and South Georgia Island. Chinese Journal of Polar Science 8:27–32.
- Zhu, R. B. , L. G. Sun , X. B. Yin , Z. Q. Xie , and X. D. Liu . 2005. Geochemical evidence for rapid enlargement of gentoo penguin colony on Barton Peninsula in the maritime Antarctic. Antarctic Science 17:11–16.