ABSTRACT
Holocene lake and catchment environmental history and regional climate are reconstructed from lake sediments at Trettetjørn, a small lake situated close to the present-day treeline in western Norway. Sediments began to accumulate in the lake ca. 8575 (± 115) cal yr BP. Pollen-inferred mean July temperatures (Tjul) fluctuated below 12°C with two cooler phases ca. 8400 and 8200 cal yr BP. The pollen-inferred annual precipitation (Pann) was lowest during the early Holocene and varied around 1600 mm yr−1. At the same time the highest diatom-inferred pH values (6.8) were reconstructed, probably due to input of base cations from the immature catchment soils. Betula pubescens became established around the lake ca. 8270 cal BP, soon followed by Pinus sylvestris. Maximum Tjul of ca. 12.5°C occurred from 7760 to 5200 cal yr BP. The oribatid mite assemblages confirm the vegetation development from semiopen grassland to forest. Inferred Tjul was variable after 5200 cal yr BP and declined markedly around 4175 cal yr BP. At the same time more oceanic conditions are inferred with changes in the vegetation and mite assemblages suggesting the expansion of mires. After ca. 5000 cal yr BP and towards the present, the diatom concentration in the core becomes low and variable, thickness of the valves within the same taxon varies, and sometimes diatom frustules are completely absent from the sediment column. The diatom valve dissolution has affected both the diatom assemblages and the diatom-inferred pH up to the present-day. All proxies suggest that human impact affected the catchment after ca. 2000 cal yr BP followed by more intensive impact after ca. 1555 cal yr BP. The enlargement of the settlement at Upsete during the construction of the Bergen–Oslo railway (1894–1909 AD) is reflected by a charcoal peak and the presence of spheroidal carbonaceous particles coincides with a change in the diatom assemblages.
Introduction
The forest-alpine transition is a striking vegetation boundary that shows considerable sensitivity to climate change (CitationKörner, 1998). Chemical weathering can dominate during phases of dense forest in the catchment whereas physical erosion may prevail during phases with an open catchment (CitationKauppila and Salonen, 1997). Treeline fluctuations also have important impacts on the lake environment. A temporary increase in lake-water productivity and lake-water pH could occur when the leaching of base cations from the catchment is accelerated by the development of organic soils when forest becomes established. On the other hand, if the soils in the catchment undergo a steady depletion of available bases, forest establishment can accelerate natural lake-water acidification through the release of weak organic acids and the storage of cations in the accumulating soils and vegetation (CitationH. H. Birks et al., 2000). As forest soils build up, more decomposed dissolved organic components enter the lake and reduce the light availability for aquatic plants. An increase in organic accumulation into the lake can also occur as tree birch declines in the catchment due to climate change (CitationVelle et al., 2005). A significant change in the degree of wind exposure can occur depending on whether trees are present or absent from the catchment, influencing the timing and extent of lake stratification, circulation patterns, and ice formation.
The potential sensitivity of the treeline to climate change (CitationKörner, 1998) is the reason for this paleoecological study that explores the timing and effects of terrestrial vegetational change and accompanying changes in the aquatic ecosystem at a site situated just above the present-day treeline. An important source of information about the climate during the Holocene is from reconstructions of mean July temperatures (Tjul) based on pollen preserved in lake sediments situated in the treeline area (e.g., CitationSeppä and H. J. B. Birks, 2001; CitationBjune et al., 2004). For reconstruction of local vegetational changes in the lake catchment, plant macrofossil analysis is a valuable tool. Plant macrofossils tend to reflect the past local vegetation whereas pollen analyses give a more regional pattern, especially during treeless conditions (CitationH. H. Birks, 1993; CitationH. H. Birks and H. J. B. Birks, 2000, Citation2003). Oribatid mites from lake sediments have been used to infer climate changes related to terrestrial and aquatic habitat development during deglaciation and the early Holocene (CitationI. W. Solhøy and T. Solhøy, 2000; CitationT. Solhøy 2001). Diatoms are sensitive ecological indicators of lake-water quality change. They have been used in the reconstruction of several variables accompanying catchment vegetational change such as changes in organic matter or lake-water pH following tree establishment or decline (e.g., CitationFord, 1990; CitationPienitz et al., 1999). Each proxy has its individual strengths and weaknesses but by combining information from several proxies we hope to achieve a more detailed picture of past environmental and climatic change.
As the North Atlantic strongly affects the climate in northwest Europe, paleoclimate reconstructions from western Norway thus can be a key in documenting natural climate variability in the past. Paleoclimatic reconstructions from this region can also give insights into possible atmospheric and oceanic circulation changes that are potentially important in relation to recent global warming (e.g., CitationNesje et al., 2000; CitationBjune et al., 2005). Inferred summer temperature reconstructions and terrestrial environmental reconstructions from terrestrial paleoecological records in this region are therefore needed (CitationJansen et al., 2005).
Study Area
Trettetjørn (7°00′E, 60°43′N) is located in the western part of Norway (), 810 m above sea level on the border between the subalpine forest formed by Betula pubescens and the low-alpine zone (CitationMoen, 1998). No trees are present in the catchment, but scattered birch trees are present in the hillsides surrounding the lake. Estimated present-day mean July and January temperatures are 10.7°C and −5.5°C, respectively, and estimated annual precipitation is 1800 mm yr−1 (Arvid Odland, unpublished). The lake is circular with a diameter of about 100 m and a maximum water depth of 7.8 m. The catchment consists of bare bedrock or is covered by thin till where Empetrum nigrum and Vaccinium vitis-idaea dominate on shallow dry ground, while V. myrtillus and V. uliginosum are abundant on deeper and wetter soils. Towards the south, a bog has formed under the steep valley slope. A stream enters the lake in the eastern part of the lake, and a stream may form during snowmelt, draining over the bog surface. There is an outlet over a bedrock threshold draining towards the west. Gabbro dominates the catchment bedrock with the presence of sandstone and phyllite in some areas (CitationBjune et al., 2005). The lake-water pH has an average value of 6.3 during the growing season (n = 11, range: 5.8–6.4). Lowest pH (5.8) is observed during spring snow melt. Conductivity and alkalinity are low (n = 11, range: 8–12 µS cm−1 and 16–66 µeq, respectively). The total carbon content (TOC) is also low (1.7 mg L−1, n = 1) and SiO2 has a relatively wide range during the growing season (n = 11, range: 0.48–61.60 mg L−1). The lake was ice covered from early November 2001 to the middle of May 2002. The following autumn the lake froze in early October and still had 1 m of ice in early May 2003. The lake therefore melted out late (early June) compared to the year before. This was probably due to the dry winter of 2002–2003 when the thin snow cover had little insulating effect on the lake. The prevailing wind direction is from east to west, down the valley, probably suppressing the treeline locally.
Methods
Sediment Sampling and Analyses
One sediment core (the main core) (350 cm) was retrieved from a water depth of 6.8 m in January 2001 using a 110-mm modified piston corer (CitationNesje, 1992) that sampled the entire accumulation of Holocene sediment (272 cm) at the site. Below 272 cm, the core contained minerogenic material of variable size interpreted as a till. One short core covering the upper 30 cm of sediment was retrieved using a HON corer (CitationRenberg, 1991) as the upper part of the CitationNesje (1992) core becomes disturbed during sampling and transport. The short core was sliced in the field into 0.25 cm intervals and stored in plastic bags.
Sediment description follows CitationTroels-Smith (1955). Sediment samples of 0.5 cm3 were taken continuously from the short core or at 1- to 2-cm intervals from the main core using a brass sampler (CitationH. J. B. Birks, 1976) for the percentage loss-on-ignition (LOI) analyses at 550°C (LOI 550) and 950°C (LOI 950). The short core and the main core were correlated by visual inspection of the LOI 550 profiles. For LOI dry weight was determined after drying overnight at 105°C. The samples were then ignited at 550°C for 6 h and then put in a desiccator for cooling to room temperature and weighed (CitationEnell and Larsson, 1986). LOI is calculated as a percentage of dry weight. Bulk density is defined as the weight of a known volume compared with water and was calculated as fresh weight divided by volume.
Ams-radiocarbon Dating and Chronology
Samples were sent for a radiocarbon dating by accelerator mass spectrometry (AMS) to the following laboratories: Beta Analytic Inc., Miami; Radiological Dating Laboratory, Trondheim; and Poznań Radiocarbon Laboratory, Poznań. All dates are from bulk sediments as the sediment sequence did not contain enough terrestrial plant macrofossils for dating except at sediment level 195.5 cm that is dated using terrestrial plant macrofossils. The dating at 269.5 cm is excluded from the age-depth model and disregarded as it probably has a hard-water reservoir effect leading to a too old age (11,680 ± 60 14C yr BP). The dating is far too old compared to the deglaciation history in the region (see discussion below). There is a possibility that also younger dates suffer a hard water effect though to a lesser or very low extent as indicated by the relatively low diatom-inferred pH during the whole sediment sequence (see the result and discussion below). (The diatom-inferred pH was not reconstructed at level 269.5 cm due to the absence of diatoms.)
The dates were calibrated using CALIB 4.3, method A, and the bidecadal data-set (CitationStuiver and Reimer, 1993) (). Age-depth modeling was then performed using a weighted regression procedure in the framework of generalized additive models (CitationHeegaard, 2003; CitationHeegaard et al., 2005). Chronology is presented as calibrated years before present (cal yr BP), where BP is AD 1950. Ages below the lowest radiocarbon dates were estimated by extrapolation of the fitted model. The age-depth model is presented in .
Table 1 AMS-dates from Trettetjørn, showing laboratory number, sample name, depth in the core, conventional 14C age BP with one standard error, and 14C-calibrated age BP 1950 with one standard error.
Pollen Analysis
Subsamples for pollen analysis of 0.5 cm3 were taken in the laboratory at 2- to 4-cm intervals from 53 levels, prepared using standard methods (CitationFægri and Iversen, 1989), and mounted in glycerine. At least 500 terrestrial pollen grains and spores were identified to the lowest possible taxonomic level using keys (CitationFægri and Iversen, 1989; CitationMoore et al., 1991; CitationPunt et al., 1976–95) and an extensive modern pollen reference collection at the Department of Biology, University of Bergen. The pollen sum includes trees, shrubs, herbs, and dwarf shrubs. Pteridophytes are excluded from the sum due to local over-representation of these taxa.
Plant Macrofossil Analysis
Samples of a known volume of sediment, varying from 8 to 28 cm3, from 26 levels were washed through a sieve of mesh diameter 125 µm, soaked in water and 10% KOH for a few minutes to dissolve humics, and sieved again (CitationH. H. Birks, 2001). Macrofossils were picked out from the residue, identified and counted at 12× or 40× magnification under a stereomicroscope. The number of macrofossils was normalized for 25 cm3 of sediment before presentation (CitationH. H. Birks, 2001).
Mite Analysis
The residues sieved for macrofossils were also used for mite analysis. Nineteen levels were analyzed. Mites were sorted, identified, and counted at 16× magnification under a stereomicroscope. Only oribatid mites, both adults and juvenile stages, were considered. The number of mites was normalized for 100 cm3 of sediment comparable to the study by CitationI. W. Solhøy and T. Solhøy (2000). As the sediment core was taken in the center of the lake, more aquatic taxa are expected than terrestrial taxa. The mites found are classified in several broad ecological groups following those established by CitationI. W. Solhøy and T. Solhøy (2000).
Diatom Analysis
Samples of 1 to 0.5 g wet sediment were taken at 2- to 8-cm intervals from 68 levels of the 0.25 cm sliced Renberg core and the Nesje core. In 11 samples the concentrations of diatoms were too low for counting. In several parts of the core, the aim of counting 500 valves was difficult to achieve. In ten of the samples, the number of counted valves therefore varied from ca. 300 to 400. Diatom preparation and concentration estimation followed the method of CitationBattarbee (1986) and CitationBattarbee and Kneen (1982). Floras for identification included CitationKrammer and Lange-Bertalot (1986–91) and CitationCamburn and Charles (2000). As the AL∶PE training set (CitationCameron et al., 1999) used for pH reconstruction follows the nomenclatural conventions in the Surface Water Acidification Programme (SWAP) pH-diatom data set (CitationStevenson et al., 1991), the taxonomy and nomenclature of the Trettetjørn diatom stratigraphy were harmonized to the SWAP data set.
Numerical Analyses
Detrended correspondence analysis (DCA) was used (detrending by segments, nonlinear rescaling, no downweighting of rare taxa) to summarize the main trends in the diatom and mite assemblages through the core. Principal components analysis (PCA) was used (intersample distances, centering by species) for the pollen assemblages as the data-set had a shorter gradient (CitationJongman et al., 1987). The ordinations were performed using the program CANOCO 4.5 (Citationter Braak and Šmilauer, 1998). Pollen and diatom percentages were square root transformed while mite concentrations were log transformed to stabilize their variances prior to ordination.
For reconstruction of mean July temperature (Tjul) and annual precipitation (Pann) a modern pollen-climate training set was used. This includes surface sediments from 191 lakes distributed throughout Norway and northern Sweden (H. J. B. Birks, S. M. Peglar, and A. Odland, unpublished data). The data-set crosses large gradients in both temperature and precipitation. Modern Tjul and Pann values were estimated for each of the 191 lakes using climate data from the 1961–1990 Climate Normals data from nearby meteorological stations by interpolation and adjustments for altitude using a standard lapse rate of −0.57°C per 100 m altitude (CitationSeppä and H. J. B. Birks, 2001). Pollen-climate transfer functions based on the training set were developed using weighted-averaging partial least squares (WA-PLS) regression and the climate reconstructions were made by WA-PLS calibration (Citationter Braak and Juggins, 1993). The resulting two-component models have a good predictive ability as estimated by leave-one-out cross-validation (Citationter Braak and Juggins, 1993). The root-mean-square error of prediction (RMSEP) is 1.03°C (based on leave-one-out cross-validation) for Tjul and 417 mm for Pann. The Pteridophytes are included when reconstructing past climate.
Inferred pH values reconstructed from the subfossil diatom assemblages were made by WA-PLS, applying the AL∶PE training set (CitationCameron et al., 1999) in a three-component WA-PLS model. The diatom percentage data were transformed to square roots. Sample-specific root mean squared errors of prediction were estimated by cross-validation (CitationH. J. B. Birks, 1995). The diatom diversity was estimated as the effective number of taxa (Hill's N2) (CitationHill, 1973).
A LOESS smoother (span = 0.2) (CitationCleveland, 1979) was fitted to the plot of the diatom-inferred pH, Hill's N2 diatom diversity, the axis one sample scores for pollen and diatoms, Tjul, and Pann to highlight the major trends using the program C2 (CitationJuggins, 2003). The pollen and diatom stratigraphies were divided into assemblage zones using optimal sum of squares partitioning (CitationH. J. B. Birks and Gordon, 1985) using the program ZONE (Juggins, unpublished) and the number of zones to be used was assessed by comparison with the broken stick model (CitationBennett, 1996). Diagrams were drawn using TILIA and TILIA GRAPH (CitationGrimm, 1990), except for the quantitative environmental reconstructions that were drawn in C2 (CitationJuggins, 2003).
Results and Discussion
The sediment accumulation rate (based on the age-depth model) is 0.0313 cm yr−1 through the whole sediment sequence. The error estimate in the model is low through the sequence with a maximum error estimate of ±180 yr from 6240 to 5120 cal yr BP. Selected taxa of pollen, spores, and macrofossils are shown in , mites in , and diatoms in . Selected sedimentological variables, diatom-inferred pH, Hill's N2 diversity measure, and concentration for diatoms, DCA sample scores on axis 1 for diatoms and mites, PCA sample scores on axis 1 for pollen, and pollen-inferred Tjul and Pann are shown in .
Figure 3 Selected pollen and spore percentages and macrofossil concentrations from Trettetjørn. The data are presented on a depth basis with a calibrated age scale. Pollen is presented as % of total land pollen (solid silhouettes). The open silhouettes denote a 10× exaggeration of the percentage values. Macrofossils are presented as numbers per 25 cm3 of sediment as histograms. Sphagnum leaves, Drepanocladus, and Nitella are given in abundance categories. Pollen zones (P-) are shown in the right-hand column.
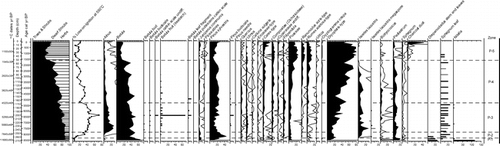
Figure 4 Selected mite concentrations from Trettetjørn. The data are presented on a depth basis with a calibrated age scale. The phases shown in the right-hand column refer to the phases in the text.
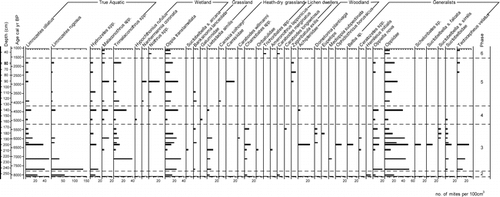
Figure 5 Selected diatom percentages from Trettetjørn. The data are presented on a depth basis with a calibrated age scale. Diatom zones (D-) are shown in the right-hand column.
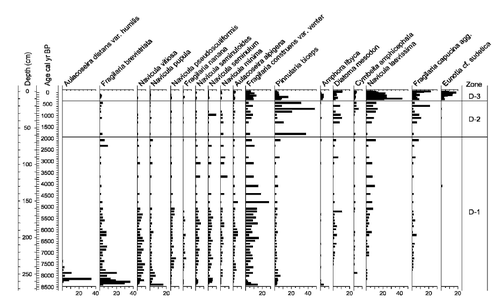
Figure 6 % LOI 550, sediment bulk density, diatom-inferred pH, Hill's N2 for diatoms and diatom concentrations (values per cm3 × 108), DCA sample scores (axis 1) for diatoms, PCA sample scores (axis 1) for pollen, and DCA sample scores (axis 1) for mite assemblages, inferred mean July temperature (Tjul), and annual precipitation (Pann) from Trettetjørn plotted against calibrated age. Diatom inferred-pH is based on a 3-component WA-PLS model, and Tjul and Pann values are based on a 2-component WA-PLS model. For inferred pH, Hill's N2, DCA, PCA, Tjul, and Pann the individual points are joined and a LOESS smoother (span = 0.2) is fitted to highlight the major trends. The phases mentioned in the text are indicated in the right-hand column.
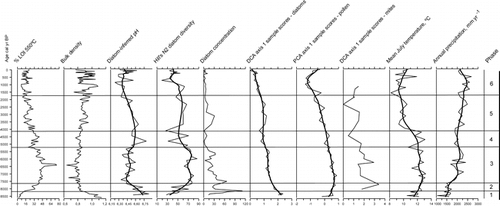
Timing of Compositional Change
The different proxies will have different sensitivities and thresholds to the environmental changes influencing the lake and catchment system. This causes compositional shifts that can occur independently between proxies. In Trettetjørn, there are similarities in the timing of compositional shifts between proxies but also differences in response time occur. This is shown and commented below. The environmental outline is divided into phases 1 to 6 on the bases of the pollen zones and the change in reconstructed July temperatures. These phases are discussed in the text and shown on and .
Environmental History
Phase 1: Deglaciation and Pioneer Phase 8575–8270 Cal Yr Bp (270–260.5 cm)
According to the extrapolation of the age-depth model the basal sediments accumulated in the lake from ca. 8575 (± 115) cal yr BP. If this date represents deglaciation of the area it is late compared to dated sediments from sites nearby. The Finse area lying ca. 1200 m a.s.l. at a distance of 30 km from Trettetjørn, was deglaciated ca. 10,270–9990 cal yr BP (CitationDahl and Nesje, 1994). On the other hand, an age of 11,680 ± 60 14C yr BP of the basal sediment is far too old and by comparison with other pollen diagrams in the area the estimated age for the basal sediments using extrapolation appears to be correct (CitationBjune, 2005). According to Atle Nesje (pers. comm.), the Upsete area was probably deglaciated ca. 9000 cal BP whereas areas at higher altitudes may have melted out earlier.
During the first 300 yr of sediment deposition, the catchment and lake vegetation were sparse as judged from the macrofossil evidence. The earliest pioneers found in Trettetjørn were the submerged aquatic algae Nitella and the moss Drepanocladus. In addition Pediastrum and Botrycoocus had their maximum abundance in the pioneer phase. Nitella is characterized by its rapid colonizing ability into lakes in newly deglaciated or disturbed areas (e.g., CitationH. H. Birks, 2000) while the genus Drepanocladus can grow in a range of different terrestrial and aquatic habitats (CitationJonsgard and H. H. Birks, 1995). The existence of mosses in the earliest sediments suggests that they colonized newly available habitats, also aquatic, as rapidly as the vascular plants.
The mite analysis does not cover all of this phase. However, the development of the pioneer lake flora may explain the existence of aquatic oribatid taxa such as Hydrozetes and Limnozetes even if they are not considered to be pioneer species.
The pollen diagram is dominated by pioneer taxa such as Salix herbacea, Ericaeae-type, and Juniperus communis. The highest pollen percentages are of Betula and Pinus sylvestris most of it probably being a result of long-distance transport (CitationBjune, 2005). Only one Betula pubescens budscale was found in the first part of this phase representing the immigration of birch trees. Inferred Tjul are low and fluctuate below 12°C. Two Tjul minima occur at ca. 8400 and 8200 cal yr BP (CitationBjune et al., 2005) that could be related to the Finse event described by CitationDahl and Nesje (1996). The Pann values are the lowest reconstructed during the Holocene and vary around 1600 mm yr−1. During the Finse event winter precipitation was inferred by CitationDahl and Nesje (1996) to be 175% of the AD 1961–1990 normal with a mean summer temperature of ca. 1.35°C warmer than at present at Finse. According to the Tjul inferred from Trettetjørn the summers were slightly cooler during the Finse event, and a new reconstruction of winter precipitation based on the Tjul suggests that winter precipitation at Hardangerjøkulen increased to ca. 160% of present-day values (CitationBjune et al., 2005).
A large part of the sediment is of inorganic origin, as judged by the loss-on-ignition analyses. The diatom record consists of typical pioneer types such as Fragilaria taxa, characteristic of the deglaciation phase and often related to relatively high pH and turbid conditions. Only F. brevistriata and F. construens var. venter have a high relative abundance. Most of the taxa such as Aulacoseira alpigena, A. distans var. humilis, Navicula minima, N. pseudoscutiformis, N. pupula, N. seminuloides, N. seminulum, N. vitiosa, and Pinnularia biceps were already present in the pioneer phase and their occurrences suggest that the lake and the catchment stabilized very quickly, possibly due to the low amount of glacial-deposits in the catchment and/or a rapid colonization by terrestrial plants. The inferred pH is the highest reconstructed in the lake's history, with a maximum above 6.8, probably reflecting the leaching of base cations from the immature catchment soils a process that may have been accelerated by the developing organic matter in the soils producing organic acids.
Phase 2: Birch Establishment 8270–7760 Cal Yr Bp (260.5–244.5 cm)
Production in the catchment increased and stabilized (as judged by the LOI 550) related to the succession of the terrestrial vegetation from an open dwarf-shrub and shrub vegetation into a semiopen vegetation with scattered trees of birch and pine. During this phase the oribatid species Dometorina plantivaga inhabitant of lichens on trees (CitationGrandjean, 1950; CitationTravé, 1963) and the species Liebstadia similis and the genus Chamobates considered inhabitants of more open areas (CitationT. Solhøy, 1976, Citation1979; CitationMaraun and Scheu, 2000) support the change of the former vegetation into semiopen vegetation around Trettetjørn.
On southwest Hardangervidda the oldest birch megafossils are dated to 7900 cal yr BP at 1122 m a.s.l. by CitationMoe (1979). No megafossils are reported from the western side of Hardangervidda. According to CitationSimonsen (1980) birch reached as high as 800 m a.s.l. in Ulvik in Hardanger between 10,900–9000 cal yr BP. At Vestre Øykjamyrtjørn, a low-alpine maritime site, dwarf and tree birch had been present since the late glacial (J. Bakke and A. E. Bjune, unpublished).
The Tjul rises quickly in this period, suggesting favorable conditions for the establishment of the local flora. The reconstructed July temperature is validated by the presence of birch macrofossils in the sediments. According to CitationOdland (1996) birch needs at least a July temperature of 10°C in western Norway. Pann is still low as in the previous phase, with values fluctuating around 1500 mm yr−1. Hydrozetes and Limnozetes ciliatus and L. rugosus, which are oribatids spending most of their lives submerged in water or floating within aquatic vegetation (CitationBehan-Pelletier, 1989; CitationBehan-Pelletier and Bisset, 1994), indicate the presence of aquatic and shore vegetation. They also may indicate a wetter climate.
A short period of high abundance of Aulacoseira distans var humilis characterizes phase 2 following the dominance of Fragilaria taxa in the pioneer development of the lake flora and can be interpreted as an increase in water transparency following catchment soil stabilization (CitationJones et al., 1989). Aulacoseira distans var. humilis has been found abundantly in sediments from a lake in south Sweden in the late-Pre-Boreal to the early Boreal zone (CitationFlorin, 1977). In addition the taxon occurs rather commonly in lakes from central and northern Sweden and Finland (CitationFlorin, 1980). Isoëtes lacustris (microspores) increase during this phase. In general, isoetids favor shallow stony and silty shores or deeper water in clear-water lakes on a silty and stony bottom (CitationH. H. Birks, 2000).
Phase 3: Stable Vegetation and Summer Temperature Maximum 7760–5200 Cal Yr Bp (244.5–164.5 cm)
Macrofossils indicate the local presence of pine at Trettetjørn from ca. 7700 cal yr BP. The more continental (drier, warmer) climate in the mid-Holocene may have favored pine (CitationKullman, 1992). On the eastern part of Hardangervidda, in more continental areas, pine establishment is dated to 9300 cal yr BP at 1180 m a.s.l. (CitationMoe, 1979) and to 9650 cal yr BP (CitationAas and Faarlund, 1988) by finds of pine megafossils, suggesting an early establishment of pine in this area. In the western, more maritime climate pine establishment is believed to be earlier than in the inland areas (CitationAas and Faarlund, 1988). At Vestre Øykjamyrtjørn pine is present from ca. 9500 cal yr BP, and dominant from ca. 8000 cal yr BP (CitationBjune, 2005) during the same time period as pine became established in the more continental areas. A combination of harsh climate, large amounts of snow, and poorly developed soils may have prevented the establishment of pine at Trettetjørn before ca. 7700 cal yr BP. In Ulvik pine was present from ca. 8900–7800 cal yr BP (CitationSimonsen, 1980). The fact that pine arrived late at Trettetjørn, which is situated on the boundary between an oceanic and a continental climate, is supported by CitationAas and Faarlund (1988) who found an earlier establishment of pine at coastal sites than at inland sites.
The presence of woodland in the catchment may be reflected by the mite fauna associated with lichens living on trees such as the family Achipteriidae and the species Ophidiotrichus borussicus and Dometorina plantivaga indicating woodland (Torstein Solhøy, pers. comm.). The oppidae Medioppia subpectinata appears for the first and only time during this phase. This oribatid mite also indicates wooded and moist environments (CitationSubías and Arillo, 2001).
This phase had the warmest summers of the Holocene. The inferred temperatures indicate stable July temperatures varying between 12 and 13°C. Maximum Tjul are found ca. 7500–7000 cal. BP. After the Finse event at ca. 8200 cal yr BP the glaciers on the Hardangerjøkulen plateau melted away, and in the Finse area only small local glaciers persisted during the summer temperature maximum (CitationDahl and Nesje, 1994). CitationDahl and Nesje (1996) suggest that winter precipitation between ca. 7400–6200 cal. BP was 145 to 150% higher compared to present values, followed by drier conditions until ca. 5700 cal yr BP with winter precipitation of 65% compared to present values. This precipitation pattern is also partly reflected in our study where Pann increases steadily during this period until 6800 cal yr BP reaching above 2000 mm yr−1 probably mostly as an increase in winter precipitation as found in CitationBjune et al. (2005). The Pann then remains at the same level but becomes more variable during the rest of the phase.
The diatom flora continued to change as summarized by the change in the DCA axis one sample scores, mainly due to the decrease in the abundance of Aulacoseira distans var. humilis and Fragilaria taxa. The diversity index N2 reached its highest values for the whole of Holocene reflecting the first appearance of taxa such as Cymbella amphicephala, Diatoma mesodon, and Fragilaria capucina agg. A modern diatom diversity study by CitationWeckström and Korhola (2001) across the boreal coniferous forest to treeless tundra transition in arctic Lapland found taxa diversity to be highest in the mountain birch woodland. In general, a longer growing season allows the development of more complex diatom communities with higher diversity (CitationDouglas and Smol, 1999), as shown also by the diatom flora of Trettetjørn. The planktonic species Fragilaria nanana appeared for the first time in the lake during this phase and disappeared at the end of it. The species has been found to be more abundant in forested lakes and may partly reflect a longer ice-free period compared to alpine lakes and an earlier onset of thermal stratification (CitationLaing et al., 1999). At the start of this phase the inferred pH values decrease, reflecting the local forest development which results in the retention of the base cations in the catchment vegetation and soil and increased input of organic acids to the lake. The concentration of diatom valves is highly fluctuating through the core but there is an overall decrease in concentration during this phase. This could reflect the decrease in pH providing less optimal conditions for the Fragilaria taxa to develop. The presence and increase in Isoëtes lacustris microspores indicate clear, oligotrophic lake-water. This increase in Isoëtes lacustris probably provides habitats for mites, and it is also reflected in the oribatid mite fauna where Hydrozetes, Limnozetes ciliatus, and L. rugosus continue to be frequent in the sediments.
Phase 4: Summer Temperature Variability 5200–4175 Cal Yr Bp (164.5–132.5 cm)
The inferred Tjul during this period suggests that the summer temperatures became more variable. At the start of the phase Cryptogramma crispa spores occur. This fern needs an insulating snow cover during winter (CitationJonsell, 2000) and the presence of C. crispa spores suggests that winter precipitation and snow cover increased. An increase is also seen in Dryopteris-type and Sphagnum spores (however there is no increase in Sphagnum leaves). LOI 550 also decreases and fluctuates around 30%. A slight increase is seen in Pann during this phase, and Tjul fluctuates. The lack of pine megafossils on Hardangervidda after ca. 5300 cal yr BP suggests a lowering of the pine limit and treeline (CitationMoe, 1979). At Trettetjørn pine macrofossils are almost absent after 5200 cal yr BP, suggesting a retreat in the pine treeline in the area. A climate with both lower temperatures and increased snow cover in winter is one explanation for this retreat of pine. During this period glacier expansions are seen across large areas in southern Norway supporting the hypothesis of increased humidity, especially winter precipitation (e.g., CitationDahl and Nesje, 1996; CitationNesje et al., 2001; CitationLie et al., 2004). When pine disappeared birch was the only tree growing in the catchment around Trettetjørn. A few birch fruits and leaf fragments are present in the sediments, but in general the landscape became more open as seen by the increase of pollen of grasses, sedges, and herbs. These features suggest that the climate had changed to more oceanic conditions during this phase, leading to the development and expansion of mires.
During this phase there are no remains of the oribatid mite fauna indicating woodland, which matches with the proposed retreat of the treeline. For the first time, oribatid mites indicative of wetland appear. Hypochthonius rufulus and Nanhermannia coronata are considered to be associated with rather wet habitats (CitationT. Solhøy, 1979). The latter are found in the lowlands up to the pre- and subalpine regions (CitationDalenius, 1962; CitationT. Solhøy, 1979). The pollen analyses suggest the development of mires during this phase, but judging from the oribatid records most probable peat formation had already started during the previous phase. At Trettetjørn today the predominant species from the wettest areas and from the bog around the lake is Trimalaconothrus maior, which is considered an oribatid usually found in association with Sphagnum moss species, a genus typical of oligotrophic bogs. It is, therefore, possible that the fossil Trimalaconothrus belongs to the species Trimalaconothrus maior. After ca. 5000 cal yr BP and towards the present, the diatom concentration in the core becomes low and variable, thickness of the valves within the same taxon varies, and sometimes diatom frustules are completely absent from the sediment column. As the start of the dissolution coincides with the change to cooler, more oceanic conditions we propose that the climate change altered the lake environment in such as way as to cause diatom dissolution. Possible causes of dissolution are further discussed in CitationLarsen et al. (2006).
The diatom assemblages found in Trettetjørn from this phase until the present-day may therefore be biased towards large and more silicified valves and thus would have an important effect on the interpretation of the lake environment in an unpredictable way. The DCA sample scores fluctuate through the phase and into the next, suggesting that the dissolution effect was significant during this time period. A severe effect is especially observed at 150 cm (4735 cal yr BP) where N2 and the diatom concentration are very low, and the inferred pH value is especially high.
Phase 5: Main Summer Temperature Decrease 4175–1555 Cal Yr Bp (132.5–50.5 cm)
The inferred mean July temperatures during this period suggest that the summer temperatures decreased from the start of this phase. The Tjul are lower than at present varying around 10°C. Macrofossils of birch are essentially absent from this time period through the rest of the core, possibly due to a temperature too low for birch to thrive (CitationOdland, 1996) in combination with human impact. The vegetation developed into an open dwarf-shrub and grassland vegetation and the pollen diagram is dominated by taxa such as Ericaceae-type, Calluna vulgaris, Poaceae, Cyperaceae, Ranunculus acris-type, Rumex acetosa-type, and Dryopteris-type.
The PCA scores of the pollen assemblages along axis one follow mainly the same trend as the pollen-inferred mean July temperature through the core. This indicates that the most important floristic change in the pollen data is due to temperature changes. A major temperature shift at 4200 cal yr BP is seen in the pollen diagram as an increase in the Dryopteris-type but not recorded in the PCA axis sample scores. This floristic change is possibly influenced by a second important environmental gradient, uncorrelated with the environmental variable expressed along PCA axis one.
Annual precipitation increases to values above 2000 mm yr−1 which agrees with CitationDahl and Nesje (1996) who show that the glaciers on the Hardangerjøkulen plateau have existed continuously since ca. 4200 cal yr BP, probably in response to an increase in winter precipitation and a decrease in summer temperature. The increase in precipitation is also indicated by the increase of fern spores in the sediments from Trettetjørn.
During this phase of higher precipitation the oribatid record has a higher representation of terrestrial mites than earlier. The oribatid mites found are considered to be indicative of wetlands, such as Nanhermannia coronata and Nothrus (CitationKarppinen, 1955; CitationTarras-Wahlberg, 1961; CitationT. Solhøy 1976, Citation1979). However, there are also oribatid mites indicative of grassland, such as Banksinoma lanceolata (CitationT. Solhøy, 1979) and heathland, such as the family Camisiidae. Thus the vegetation must have included both wetland and heathland during this phase. Due to the few number of oribatid mites recorded, the DCA analysis is highly variable. The disappearance or appearance of a few individuals in the core will have a relatively high influence on the DCA results. However, the DCA analysis do reflects a trend in the change of taxa along the sequence of the core. At the bottom of the core the oribatid fauna is mainly composed of aquatic, woodland dweller and generalist taxa. This fauna changes into a fauna more characteristic of wetland and grassland habitats at the top of the sequence.
Isoëtes lacustris spores are not recorded from the sediments after ca. 2000 cal yr BP. This species is not found in lakes where the water is turbid and brown colored, or where the organic content in the water is high (CitationSeppä and Weckström, 1999). Its decline could be a result of increased humus input into the lake as peat developed around the lake. There are also indications of grazing starting ca. 1700–1600 cal yr BP in the area, as suggested by the increase in Poaceae, Carex-type, Cyperaceae, Ranunculus acris-type, and Rumex acetosa-type pollen (CitationHjelle, 1999). Grazing could cause more turbid conditions in the lake by disturbing soil and increasing inwash and thereby preventing the growth of Isoëtes lacustris. The sediment bulk density becomes highly variable after ca. 2100 cal yr BP. At the same time there is an increase in the abundance of the diatom taxon Fragilaria brevistriata that can tolerate more turbid conditions in the lake-water (CitationJones et al., 1989). In addition, after 2000 cal yr BP there is a significant shift in the diatom zonation. This change starts before the vegetation change induced by human impact. This can suggest a nonsynchronous change of pollen and diatoms to human impact, where the diatoms responded to an early local influence of grazing in the catchment, while the pollen change was caused by a more regional human influence. The other possibility is that the change in the diatom flora was due to other factors than human disturbance such as climate change. The diatom flora had been relatively stable since ca. 7000 cal. yr BP but at ca. 2000 cal BP it could have reached an environmental threshold in connection with the climate change.
The disappearance of the aquatic plant Isoëtes lacustris most probably resulted in the disappearance of true aquatic oribatid mite taxa after 1700 cal yr BP. Oribatid mites such as Limnozetes ciliatus, Limnozetes rugosus, and Hydrozetes do not appear again. While the absence of Hydrozetes could be due entirely to the lack of aquatic and shore vegetation, the absence of Limnozetes ciliatus and L. rugosus could also be the result of a decrease in the pH of the lake. However, both L. ciliatus and L. rugosus are usually found in rather acid lakes (CitationBehan-Pelletier, 1989; CitationBehan-Pelletier and Bisset, 1994). Although there is a decrease in the diatom-inferred pH at the time these mite taxa disappear from the sediment, given their affinity to acid lakes, pH changes cannot be the sole reason why they disappeared. On the other hand, we should bear in mind that the pH reconstructions may be uncertain because of the effects of dissolution.
Phase 6: Human Impact 1555 Cal Yr Bp–ad 2000 (50.5–0 cm)
After 1550 cal yr BP a further decrease is seen in the tree and shrub pollen, while an increase occurs in Juniperus communis and herbs such as Rumex acetosa-type, Urtica dioica, Poaceae, Compositae (Cichorioideae), Epilobium, and Geranium sylvaticum-type. This suggests a more intensive use of the landscape through grazing, probably by sheep and cattle, and summer farming in the area. This activity would also result in a further lowering of the local treeline. Through trampling and use of the landscape more erosion occurred leading to increases in the input of material into the lake, as shown by the change in bulk density and the water content of the sediment. Human impact is also evident from the increase in microscopic charcoal. The large peak at 4 cm probably represents the increased settlement at Upsete during the construction of the Bergen–Oslo railway during AD 1894–1909. The reconstructed annual precipitation is about 500 mm off the present values known from meteorological observations. This is due to problems in the reconstructions due to poor coverage and good data of present day precipitation in Norway as a basis for the pollen-climate calibration data set. During this last phase there are no true aquatic oribatid mites, except for Malaconothrus, which is considered to be eurytopic and is therefore able to live in a wide range of environmental conditions (CitationPopp, 1962). Only a few taxa are found and they indicate both dry grassland and wetland, namely Trichoribates and Oppia translamellata, respectively (CitationI. W. Solhøy and T. Solhøy, 2000).
Characteristic diatom taxa in this phase are Diatoma mesodon, Fragilaria capucina agg., Navicula laevissima, and Pinnularia biceps. Fragilaria capucina varieties have been found by CitationGregory-Eaves et al. (1999) to increase in lakes with higher nutrient levels and can in Trettetjørn reflect the human settlement, such as nutrification through animal deposits in the lake catchment. Between the two sampled levels at 11 cm and 16 cm there is a significant shift in the diatom assemblages where Amphora libyca, Eunotia cf. sudetica, and Navicula laevissima increase both seen in the zonation and the DCA scores along axis one. Spheroidal carbonaceous particles produced from the burning of fossil fuels are present in the diatom slides from ca. 8 cm upwards. The change in the diatom flora reflects an altering of the lake environment that could have been caused by the increased settlement at Upsete. Interestingly, F. nanana reappears in the sediments, possibly reflecting a change in the lake's summer stratification.
Impacts of Future Climate Change
Our study demonstrates the sensitivity of lake and terrestrial biota to climatic shifts during the Holocene. The flora and fauna have both responded to changes in precipitation and summer temperature but have also changed due to human impact. Future climate scenarios for Norway indicate that the annual temperature will increase by 0.2 to 0.5°C per decade with the largest increase in the interior part of Norway especially during winter, and annual precipitation will increase in the western part of the country especially during autumn (http://regclim.met.no). These conditions are possibly favorable for an increase in snow cover during winter and an increase in erosion both during autumn rain and spring snow melt in the area surrounding Trettetjørn. These effects are already evident in western mountains in a study by CitationKlanderud and H. J. B. Birks (2003). High-altitude plants have disappeared from their lower elevation sites and increased in abundance at the highest altitudes on 23 mountains in central Norway that had been surveyed in AD 1930–31 and were subsequently resurveyed in AD 1998. This pattern was less evident in the west and was explained by increased snow-lie duration in the west, and hence a higher probability of acidification, in combination with a higher probability of erosion events. This is also demonstrated in our study where snow cover possibly controlled the pine treeline and in the future might suppress the expected treeline advance, at least for pine. The lake-water pH in Trettetjørn has been sensitive to climatic shifts during Holocene. The future climatic change will possibly also have impact on the lake-water pH such as the expected increase in snow lie duration during spring. The time period of lowest lake-water pH observed today during spring snowmelt will probably expand.
Summary
The lake and catchment history at Trettetjørn have been reconstructed by using pollen, plant macrofossil, diatoms, and oribatid mites for the last 8500 yr. The different proxies have similarities in the timing of compositional change in response to long-term climatic and catchment changes; but there are also unsimilarities in the timing of significant compositional shifts between proxies showing different sensitivities and thresholds to changes in the system. During the relatively cool and dry early Holocene phase, pioneer plant species in the catchment were characteristic of open vegetation on newly exposed soil. Relatively, high pH occurred in the lake related to the input of base cations from the immature soil. Woodland became established around the lake around 8270 cal yr BP, first with birch and later pine. Maximum Tjul reconstructed from pollen assemblages occurred from 7760 to 5200 cal yr BP, and declined from 5200 cal yr BP towards the present-day. More oceanic conditions are reflected in the vegetation and mite assemblages as Tjul decreased and Pann increased. During the last 5000 yr dissolution of diatom valves is evident affecting both the diatom diversity and inferred pH until present. During the last 2000 yr human impact increasingly affected the catchment. The construction of the Bergen–Oslo railway during AD 1894–1909 is reflected by a peak in microscopic charcoal and the presence of spheroidal carbonaceous particles, coinciding with a change in the diatom assemblages.
Acknowledgments
We are grateful to the following people for help during our work: John Birks and Sylvia Peglar provided the pollen-climate training set. Jostein Bakke, Øyvind Lie, and Gaute Velle helped obtain the cores. Gina Clarke, Wenche Eide, Lene Halvorsen, Marianne Heggen, Beate Ingvartsen, Tor Egil Larsen, and Dorte Köster assisted during water sampling. Øyvind Paasche provided preliminary magnetic susceptibility results. Lene Halvorsen did the LOI analysis, Einar Heegaard made the age-depth model, Arvid Odland provided the modern climate data, and John Birks and Hilary Birks read drafts of the manuscript. We also acknowledge the valuable comments from two anonymous reviewers. This work has been supported by the Norwegian Research Council grant through NORPEC, a NFR funded Strategic University Programme (SUP) at the University of Bergen, to Anne Bjune and Jorunn Larsen, and by the Grolle Olsen fund to Jorunn Larsen. This is publication Nr A 135 from the Bjerknes Centre for Climate Research.
References Cited
- Aas, B. and T. Faarlund . 1988. Postglasiale skoggrenser i sentrale sørnorske fjelltrakter. 14C-datering av subfossile furu- og bjørkerester. (Postglacial forest limits in central south Norwegian mountains. Radiocarbon datings of subfossil pine and birch specimens). Norsk Geografisk Tidsskrift 42:2–61.
- Battarbee, R. W. 1986. Diatom analysis. In Berglund, B. E. , editor. eds. Handbook of Holocene Palaeoecology and Palaeohydrology Chichester Wiley. 527–570.
- Battarbee, R. W. and M. J. Kneen . 1982. The use of electronically counted microshperes in absolute diatom analysis. Limnology and Oceanography 27:187–188.
- Behan-Pelletier, V. 1989. Limnozetes (Acari: Limnozetidae) of northeastern North America. The Canadian Entomologist 121:453–506.
- Behan-Pelletier, V. and B. Bisset . 1994. Oribatida of Canadian Peatlands. Memoirs of the Entomological Society of Canada 169:73–88.
- Bennett, K. D. 1996. Determination of the number of zones in a biostratigraphical sequence. New Phytologist 132:155–170.
- Birks, H. H. 1993. The importance of plant macrofossils in Late-Glacial climatic reconstructions: An example from western Norway. Quaternary Science Reviews 12:719–726.
- Birks, H. H. 2000. Aquatic macrophyte vegetation development in Kråkenes Lake, western Norway, during the late-glacial and early-Holocene. Journal of Paleolimnology 23:7–19.
- Birks, H. H. 2001. Plant macrofossils. In Smol, J. P. , H. J. B. Birks , and W. M. Last , editors. eds. Tracking Environmental Change Using Lake Sediments. Volume 3: Terrestrial, Algal, and Siliceous Indictors Dordrecht Kluwer Academic Publishers. 49–74.
- Birks, H. H. and H. J. B. Birks . 2000. Future uses of pollen analysis must include plant macrofossils. Journal of Biogeography 27:31–35.
- Birks, H. H. and H. J. B. Birks . 2003. Reconstructing Holocene climates from pollen and plant macrofossils. In Mackay, A. , R. W. Battarbee , H. J. B. Birks , and F. Oldfield , editors. Global Change in the Holocene London Arnold. 342–357.
- Birks, H. H. , R. W. Battarbee , H. J. B. Birks , E. G. with contributions by Bradshaw , S. J. Brooks , C. A. Duigan , V. J. Jones , G. Lemdahl , S. M. Peglar , J. O. Solem , I. W. Solhøy , T. Solhøy , and M. K. Stalsberg . 2000. The development of the aquatic ecosystem at Kråkenes Lake, western Norway, during the late-glacial and early-Holocene—a synthesis. Journal of Paleolimnology 23:91–114.
- Birks, H. J. B. 1976. Late-Wisconsinan vegetational history at Wolf Creek, central Minnesota. Ecological Monographs 46:395–429.
- Birks, H. J. B. 1995. Quantitative palaeoenvironmental reconstructions. In Maddy, D. and J. S. Brew , editors. eds. Statistical Modelling of Quaternary Science Data Technical Guide, 5. Cambridge Quaternary Research Association. 161–254.
- Birks, H. J. B. and A. D. Gordon . 1985. Numerical Methods in Quaternary Pollen Analysis London Academic Press. 317.
- Bjune, A. E. 2005. Holocene vegetation history and tree-line changes on a north–south transect crossing major climate gradients in southern Norway—evidence from pollen and plant macrofossils in lake sediments. Review of Palaeobotany and Palynology 133:249–275.
- Bjune, A. E. , H. J. B. Birks , and H. Seppä . 2004. Holocene vegetation and climate history on a continental–oceanic transect in northern Fennoscandia based on pollen and plant macrofossils from lakes situated at or near the present tree-line. Boreas 33:211–223.
- Bjune, A. E. , J. Bakke , A. Nesje , and H. J. B. Birks . 2005. Holocene mean July temperature and winter precipitation in western Norway inferred from lake sediment proxies. The Holocene 15:177–189.
- Camburn, K. E. and D. F. Charles . 2000. Diatoms of the Low-alkalinity Lakes in the Northeastern United States Philadelphia The Academy of Natural Sciences of Philadelphia. 152.
- Cameron, N. G. , H. J. B. Birks , V. J. Jones , F. Berge , J. Catalan , R. J. Flower , J. Garcia , B. Kawecka , K. A. Koinig , A. Marchetto , P. Sanchez-Castillo , R. Schmidt , M. Sisko , N. Solovieva , E. Stefkova , and M. Toro . 1999. Surface-sediment and epilithic diatom pH calibration sets for remote European mountain lakes (AL ∶ PE Project) and their comparison with the Surface Waters Acidification Programme (SWAP) calibration set. Journal of Paleolimnology 22:291–317.
- Cleveland, W. S. 1979. Robust locally-weighted regression and smoothing scatter plots. Journal of the American Statistical Association 74:829–836.
- Dahl, S. O. and A. Nesje . 1994. Holocene glacier fluctuations at Hardangerjøkulen, central-southern Norway: a high-resolution composite chronology from lacustrine and terrestrial deposits. The Holocene 4:269–277.
- Dahl, S. O. and A. Nesje . 1996. A new approach to calculating Holocene winter precipitation by combining glacier equilibrium-line altitudes and pine-tree limits: a case study from Hardangerjøkulen, central southern Norway. The Holocene 6:4381–398.
- Dalenius, P. 1962. Studies on the Oribatei (Acari) of the Torneträsk territory in Swedish Lapland III. The vertical distribution of the moss mites. Kungliga Fysiografiska Sällskapets i Lund Förhandlingar 32:105–129.
- Douglas, M. S. V. and J. P. Smol . 1999. Freshwater diatoms as indicators of environmental change in High Artic. In Stoermer, E. F. and J. P. Smol , editors. eds. The Diatoms: Applications for the Environmental and Earth Sciences Cambridge Cambridge University Press. 227–244.
- Enell, M. and P. Larsson . 1986. Chemical analyses. In Berglund, B. E. , editor. eds. Handbook of Holocene Palaeoecology and Palaeohydrology Chichester Wiley. 423–452.
- Florin, M-B. 1977. Late-glacial and Preboreal vegetation in southern Central Sweden. II. Pollen, spore and diatom analyses. Striae 5:1–60.
- Florin, M-B. 1980. The taxonomy of some Melosira species a comparative morphological study II. 6th Diatom Symposium Uppsala, Sweden University of Uppsala, Institute of Quaternary Geology. 43–74.
- Ford, M. S. 1990. A 10 000-yr history of natural ecosystem acidification. Ecological Monographs 60:57–89.
- Fægri, K. and J. Iversen . 1989. Textbook of Pollen Analysis. 4th rev. ed. Fægri, K. , P. E. Kaland , and K. Krzywinski , editors. eds. Chichester Wiley. 328.
- Grandjean, F. 1950. Sur Deux Espéces du Genre Dometorina N. G. et les Mæurs de D. Plantivaga (Berl.) (Acariens, Oribates). Bulletin de la Société Zoologique de France 75:5–6 224–242.
- Gregory-Eaves, I. , J. P. Smol , B. P. Finney , and M. E. Edwards . 1999. Diatom-based transfer functions for inferring past climatic and environmental change in Alaska, U.S.A. Arctic, Antarctic, and Alpine Research 31:353–365.
- Grimm, E. C. 1990. TILIA and TILIA.GRAPH. PC spreadsheet and graphics software for pollen data. INQUA Working Group on Data-Handling Methods, Newsletter 4:5–7.
- Heegaard, E. 2003. CagedepthR.txt - R function for age-depth relationship estimation. http://www.bio.uu.nl/˜palaeo/Congressen/Holivar/Literature_Holivar2003.htm .
- Heegaard, E. , H. J. B. Birks , and R. J. Telford . 2005. Relationships between calibrated ages and depth in stratigraphical sequences: an estimation procedure by mixed-effect regression. The Holocene 15:621–618.
- Hill, M. O. 1973. Diversity and evenness: a unifying notation and its consequences. Ecology 54:427–432.
- Hjelle, K. L. 1999. Modern pollen assemblages from mown and grazed vegetation types in western Norway. Review of Palaeobotany and Palynology 107:55–81.
- Jansen, E. , T. Dokken , A. Nesje , S. O. Dahl , H. Linge , U. S. Ninnemann , and O. H. Otteraa . 2005. Bjerknes Centre for Climate Research—combining past, present and future climate change. Norwegian Journal of Geology 85:33–44.
- Jones, V. J. , A. C. Stevenson , and R. W. Battarbee . 1989. Acidification of lakes in Galloway, south west Scotland: A diatom and pollen study of the post-glacial history of the Round Loch of Glenhead. Journal of Ecology 77:1–23.
- Jongman, R. H. G. , C. J. F. ter Braak , and O. F. R. van Tongeren . 1987. Data Analysis in Community and Landscape Ecology Wageningen Pudoc. 299.
- Jonsell, B. , editor. 2000. Flora Nordica 1 Stockholm Bergius Foundation.
- Jonsgard, B. and H. H. Birks . 1995. Late-glacial mosses and environmental reconstructions at Kråkenes, western Norway. Lindbergia 20:64–82.
- Juggins, S. 2003. C2. A program for analysing and visualising palaeoenvironmental data. Version 1.3. U.K University of Newcastle.
- Karppinen, E. 1955. Ecological and transect survey studies on Finnish Camisiids Acar., Oribatei. Annales Zoologici Societatis Vanamo, 17:3–80.
- Kauppila, T. and V. P. Salonen . 1997. The effect of Holocene treeline fluctuations on the sediment chemistry of Lake Kilpisjärvi, Finland. Journal of Paleolimnology 18:145–163.
- Klanderud, K. and H. J. B. Birks . 2003. Recent increases in species richness and shifts in altitudinal distributions of Norwegian mountain plants. The Holocene 13:1–6.
- Körner, C. 1998. A re-assessment of high elevation treeline positions and their explanations. Oecologia 115:445–459.
- Krammer, K. and H. Lange-Bertalot . 1986–1991. Süsswasserflora von Mitteleuropa: Bacillariophyceae 1–4 Stuttgart Gustav Fisher.
- Kullman, L. 1992. Orbital forcing and tree-limit history: hypothesis and preliminary interpretation of evidence from Swedish Lappland. The Holocene 2:131–137.
- Laing, T. E. , R. Pienitz , and J. P. Smol . 1999. Freshwater diatom assemblages from 23 lakes located near Norilsk, Siberia: A comparison with assemblages from other circumpolar treeline regions. Diatom Research 14:285–305.
- Larsen, J. , Ø Paasche , D. O. Andersen , A. E. Bjune , and A. de la Riva Caballero . 2006. Sedimentary diatom assemblages in a small alpine west Norwegian lake: evidence for metal release and precipitation?. In Witkowski, A. , editor. ed. Eighteenth International Diatom Symposium 2004, Miedzyzdroje, Poland Bristol Biopress Ltd. 221–234.
- Lie, Ø , S. O. Dahl , A. Nesje , J. A. Matthews , and S. Sandvold . 2004. Holocene fluctuations of a polythermal glacier in high-alpine eastern Jotunheimen, central-southern Norway: a multi-site, multi-parameter approach on lacustrine sediments. Quaternary Science Reviews 23:1925–1945.
- Maraun, M. and S. Scheu . 2000. The structure of oribatid mite communities (Acari, Oribatida): patterns, mechanisms, and implications for future research. Ecography 23:374–383.
- Moe, D. 1979. Tregrense-fluktuasjoner på Hardangervidda etter siste istid. In Nydal, R. , S. Westin , U. Hafsten , and S. Gulliksen , editors. eds. Fortiden i søkelyset Datering med 14C metoden gjennom 25 år. Trondheim NTH. 199–208.
- Moen, A. 1998. Vegetasjonsatlas for Norge: Vegetasjon Hønefoss Norwegian Mapping Authority.
- Moore, P. D. , J. A. Webb , and M. E. Collinson . 1991. Pollen Analysis Oxford Blackwell Scientific. 216.
- Nesje, A. 1992. A piston corer for lacustrine and marine sediments. Arctic and Alpine Research 24:257–259.
- Nesje, A. , S. O. Dahl , C. Andersson , and J. A. Matthews . 2000. The lacustrine sedimentary sequence in Sygneskardvatnet, western Norway: a continuous, high-resolution record of the Jostedalsbreen ice cap during the Holocene. Quaternary Science Reviews 19:1047–1065.
- Nesje, A. , J. A. Matthews , S. O. Dahl , M. S. Berrisford , and C. Andersson . 2001. Holocene glacier fluctuation of Flatebreen and winter-precipitation changes in the Jostedalsbreen region, western Norway, based on glaciolacustrine sediment records. The Holocene 11:267–280.
- Odland, A. 1996. Differences in the vertical distribution pattern of Betula pubescens in Norway and its ecological significance. Paläoklimaforschung 20:43–59.
- Pienitz, R. , J. P. Smol , and G. M. MacDonald . 1999. Paleolimnological reconstruction of Holocene climatic trends from two boreal treeline lakes, Northwest Territories, Canada. Arctic, Antarctic, and Alpine Research 31:82–93.
- Popp, E. 1962. Semiaqautile Lebensräume (Bülten) in hoch-und Niedermooren. International Review der gesamten Hydrobiologie 47:533–579.
- Punt, W. , et al , editor. 1976–95. The Northwest European Pollen Flora, vols. I–VII Amsterdam Elsevier.
- Renberg, I. 1991. The HON–Kajak sediment corer. Journal of Paleolimnology 6:167–170.
- Seppä, H. and J. Weckström . 1999. Holocene vegetational and limnological changes in the Fennoscandian tree-line area as documented by pollen and diatom records from Lake Tsuolbmajavri, Finland. Ecoscience 6:621–635.
- Seppä, H. and H. J. B. Birks . 2001. July mean temperature and annual precipitation trends during the Holocene in the Fennoscandian tree-line area: pollen-based reconstructions. The Holocene 11:527–539.
- Simonsen, A. 1980. Vertikale variasjoner i Holocen pollensedimentasjon i Ulvik, Hardanger. AmS Varia 8:1–65.
- Solhøy, I. W. and T. Solhøy . 2000. The fossil oribatid mite fauna (Acari: Oribatida) in late-glacial and early-Holocene sediments in Kråkenes Lake, western Norway. Journal of Paleolimnology 23:35–47.
- Solhøy, T. 1976. Species composition of the Oribatei (Acari) on oceanic mountain ground in western Norway. Norwegian Journal of Entomology 23:17–22.
- Solhøy, T. 1979. Oribatids (Acari) from an oligotrophic bog in western Norway. Fauna Norvegica Serie B 26:91–94.
- Solhøy, T. 2001. Oribatid mites. In Smol, J. P. , H. J. B. Birks , and W. M. Last , editors. eds. Tracking Environmental Change Using Lake Sediments. Volume 4. Zoological Indicators Dordrecht Kluwer Academic Publishers. 81–104.
- Stevenson, A. C. , S. Juggins , H. J. B. Birks , D. S. Anderson , N. J. Anderson , R. W. Battarbee , F. Berge , R. B. Davis , R. J. Flower , E. Y. Haworth , V. J. Jones , J. C. Kingston , A. M. Kreiser , J. M. Line , M. A. R. Munro , and I. Renberg . 1991. The Surface Water Acidification Project Palaeolimnology Programme: Modern diatom/lake-water chemistry data-set London ENSIS Publishing. 86.
- Stuiver, M. and P. J. Reimer . 1993. Extended 14C data base and revised CALIB 3.0 14C calibration program. Radiocarbon 35:215–230.
- Subías, L. S. and A. Arillo . 2001. Acari, Oribatei, Gymnonota II. In Ramos, M. A. , et al , editor. eds. Fauna Iberica, vol 15 Madrid Museo Nacional de Ciencias Naturales. CSIC.
- Tarras-Wahlberg, N. 1961. The Oribatei of a central Swedish bog and their environment. Oikos Supplement 4:1–56.
- ter Braak, C. J. F. and S. Juggins . 1993. Weighted averaging partial least squares regression (WA-PLS): an improved method for reconstructing environmental variables from species assemblages. Hydrobiologia 269/270:485–502.
- ter Braak, C. J. F. and P. Šmilauer . 1998. CANOCO reference manual and user's guide to Canoco for Windows: Software for canonical community ordination (version 4). Ithaca, NY, U.S.A Microcomputer Power.
- Travé, J. 1963. Écologie et biologie des Oribates (Acariens) saxicoles et arboricoles Paris Hermann.
- Troels-Smith, J. 1955. Karakterisering av løse jordarter. Danmarks Geologiske Undersøkelser Series IV 3:1–73.
- Velle, G. , J. Larsen , W. Eide , S. M. Peglar , and H. J. B. Birks . 2005. Holocene environmental history and climate of Råtåsjøen, a low-alpine lake in central Norway. Journal of Paleolimnology 33:129–153.
- Weckström, J. and A. Korhola . 2001. Patterns in the distribution, composition and diversity of diatom assemblages in relation to ecoclimatic factors in Arctic Lapland. Journal of Biogeography 28:31–45.