Abstract
Fieldwork within the framework of SEDAG (SEDiment cascades in Alpine Geosystems) has focused on hydrology, fluvial sediment transport dynamics, and hydrogeomorphological characteristics of the alpine river Partnach (Reintal Valley, Bavarian Alps, Germany). In August 2005 a dambreak flood occurred in the Reintal Valley. The contributing catchment area supplying material for bed load transport at the outlet of the catchment has been enlarged by more than 33% (4.3 km2 + 1.6 km2) due to lake infilling, and thus, reconnecting formerly disconnected parts of the catchment. Post-dambreak downstream variation of total and specific stream power, bed shear stress, and particle characteristics (size and shape) were determined and compared with pre-dambreak findings. As to our knowledge, results of pre- and post-dambreak fluvial-geomorphic investigations have not been published so far for an individual river. Results show high values for total and specific stream power in the post-dambreak regime. In addition, general trends for downstream decreasing in particle size, stream power, and bed shear stress show a degree of regularity after disturbance. Nevertheless, further adjustments of the Partnach channel system in response to the dambreak event are expected in the near future.
Introduction
In high-altitude fluvial systems large amounts of sediment are transported in the main stream. Also, in high mountain areas landslides very often occur. When they reach the valley bottom channel avulsions occur (CitationKorup, 2004) or even dams are created. If mountain valleys are affected by landslide dams, throughput of the fluvial sediment is interrupted, the valley is fragmented into subsystems, and the landscape is in a state of disturbance (CitationHewitt, 2006). In this context, CitationHewitt (2006) described a landslide interruption epicycle of mountain valleys, which consists of five phases:
Phase 1: the rock avalanche complex—landslide emplacement,
Phase 2: the impoundment complex—aggradation and constructional landforms upstream of barrier, possible downstream erosion and/or sedimentation,
Phase 3: the degrading interruption complex—trenching and removal of the impoundment complex, downstream sedimentation,
Phase 4: the superimposed interruption complex—exhumation of buried valley fill and incision into pre-landslide valley floor,
Phase 5: the “shadow” interruption complex—minor, persistent legacies of interruption, mainly bedrock forms.
The systems change from Phase 2 to 3 contains the dambreak event that drives new disturbance, if for example the dam was persistent for decades or centuries and the river system adjusted to it.
Also, moraines that dam proglacial lakes or earthen dams have a potential to fail (CitationCosta, 1988). The collapse of dams causes a very rapid conversion of potential to kinetic energy in a flood wave that may have hazardous as well as geomorphic consequences for the downstream channel reaches (CitationJarrett and Costa, 1986; CitationVuichard and Zimmermann, 1987; CitationCosta, 1988; CitationWalder and Costa, 1996; CitationHubbard et al., 2005; CitationMorche et al., 2007). Due to global warming and glacier retreat, the frequency of catastrophic drainage events of moraine-dammed lakes may increase (CitationDesloges and Church, 1992; CitationClague and Evans, 2000). So the importance of recent studies on the geomorphic impact of dambreak floods increases as well.
The main interesting geomorphic problems of the post-dambreak state are the behavior of fluvial sediment transport, particularly bed-load transport, along with the stream channel response to the new conditions in terms of channel morphology and bed sediment characteristics. Studies concerning these issues are rare due to low frequency of dambreak events. CitationPitlick (1993) and CitationBathurst and Ashiq (1998) presented results about the response of the Roaring River channel to the Lawn Lake dambreak flood of 1982. However, to our knowledge, no study has been published which combines results from pre- and post-dambreak investigations. One main problem of studies dealing with the geomorphic effects of large floods is “the quantitative assessment of the effects of the flood: a record of the pre-flood landscape is ideally required, in addition to the monitoring of changes during the passage of the floods and their subsequent modification” (CitationAnderson and Calver, 1977, p. 253). Fortunately, we are able to present such data, and this paper focuses on results of post-dambreak investigations on downstream variation of stream gradient and bed material grain size and shape characteristics of an alpine stream. These new data will be compared with published results from pre-dambreak investigations. Finally, we are able to demonstrate the effect of the dambreak event on the specific stream power at bank-full stage in each channel cross section.
Study Area
Reintal Valley
Previous investigations within the joint project “Sedimentary Cascades in Alpine Geosystems” have provided a detailed overview of the prominent geomorphic forms and dominant processes in the Reintal Valley, as well as details about the physiographic character (). Additionally, the Reintal valley is a key test site of the I.A.G. SEDIBUD Working Group and a selection of key data are stored in the database (http://www.geomorph.org/wg/wgsb.html).
Table 1 Reintal Valley publications with contributions by SEDAG project members.
The Reintal Valley is a formerly glaciated, U-shaped valley located in the Bavarian Alps approximately 80 km south of Munich (). The basin lithology is comprised of homogeneous limestone (Wettersteinkalk). The valley is fragmented into subsystems by large landslide deposits; upstream of the barriers areas were dominated by sedimentation (Phase 2 after CitationHewitt, 2006). The lowermost landslide dam at the lake Vordere Blaue Gumpe (VBG) failed in 2005. The interruption complex, the dam, was incised and partly removed by a high-magnitude flood event and by subsequent flow. Hence, the downstream channel reach is now in Phase 3 (after CitationHewitt, 2006). Preliminary results show an increasing bedload transport during the first post-dambreak field season (CitationMorche et al., 2008a). The most affected channel reach of the Partnach River in the Reintal Valley between the former lake VBG and the outlet of the catchment is the focus of our current investigations.
The Lake History and The Dambreak Flood Event
The dam of the VBG was created by a landslide that must have occurred around a.d. 1800. CitationMorche et al. (2006) dated the event relatively precisely by means of historical data (maps, sketches, paintings). During the 19th and 20th centuries the lake was subsequently filled with sediments. The growth of the delta was observed by interpretation of aerial photographs, and since 2000, by terrestrial geodetic surveying (CitationMorche et al., 2006). Delta sedimentation was also observed by CitationSass et al. (2007), who carried out ground-penetrating radar surveys. The different delta stages can be clearly seen as foreset bed structures in the radargrams (CitationSass et al., 2007).
Following a wet period, a thunderstorm hit the Reintal Valley on 22/23 August 2005, and the resultant flood caused the dambreak. The peak outflow was estimated according the methods of CitationCosta (1988), to be in a range between 26 and 50 m3 s−1 (CitationMorche et al., 2007), and more than 9500 m3 of water were released in a short time. The dam was lowered about 3 m and in the first downstream reach, more than 100,000 t of sediment were eroded. The sediments were deposited in the proximal downstream channel reaches (300 to 700 m downstream of the former dam), indicating a very high peak discharge. The flood wave attenuated rapidly further downstream. A similar pattern was recently reported by CitationKershaw et al. (2005) for a moraine dammed-lake outburst flood in British Columbia. Plan form changes of the Partnach River channel of up to 10 m were recognized, and only 25% of the eroded sediment mass was exported from the catchment (CitationMorche et al., 2007).
Methods
Flood Stage Mapping
Photographs were taken during the falling limb of the flood. The flood stage level was mapped during a field campaign in September 2005 using freshly deposited material on terraces, bed load impact structures on trees, and organic debris deposited in trees and on the banks. All flood marks could clearly be identified even the year after the flood, when the cross sections were surveyed and the particle counts were carried out. The area affected by the dambreak flood was determined by interpretation of official orthophotographs (©Landesamt für Geoinformation und Vermessung Bayern). The extent of the flood covered the whole valley floor of about 0.06 km2, which is three times larger than the area inundated by a previous flood in 2003 ().
Figure 2 Map of the Partnach River channel reach (investigated from the Vordere Blaue Gumpe dam to gauging station Bockhütte); background: orthophotograph from 18 July 2006 (©Landesamt für Vermessung und Geoinformation, permission for use and publication from 6 February 2007, reference number: VM3831B-oN/7-0138.). The situation round the Vordere Blaue Gumpe is shown in detail on a photograph in .
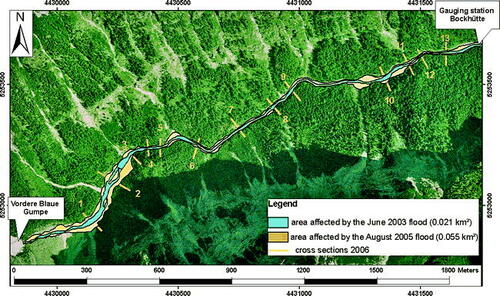
Geodetic Surveys
Geodetic surveys using an ELTA Rec ZEISS tachymeter were carried out to determine the longitudinal profile and the gradient of the channel. The survey follows the study design of the previous observation periods (CitationMorche et al., 2008b) with errors in vertical and horizontal dimensions below 1 cm. Several hundred points were measured on the channel bed along the line of maximum flow velocity. The longitudinal profile in 2003 had a mean point spacing of 2.3 m, 3.6 m in July and August 2005, and 6.3 m in September 2006 profiles. The data of the 2006 geodetic survey were then used for the calculation of the gradient (I in m m−1).
Cross Sections
During the field campaign, 13 cross sections were surveyed. Every cross section covers the post-dambreak bankfull level. At each cross section, the bankfull discharge (Q in m3 s−1) was calculated using the channel gradient (I), hydraulic radius (R in m), and the cross sectional area (A in m2) in Manning's flow equation. Manning's n was determined with particle size data following pre-dambreak investigations. The methodological procedures including a discussion of the crucial roughness parameter are described in detail in CitationMorche et al. (2008b). Further on, stream power (Ω in W m−1), specific stream power (ω in W m−2), and bed shear stress (τ0 in N m−2) were determined for each cross section.
The bench marks of the seven study sites measured in the previous observation periods were damaged during the dambreak flood. Where possible the bench marks were relocated at their previous position knowing the coordinates from former geodetic surveys. In the case of bank erosion or deposition, the cross section was expanded. An additional six new cross sections were installed in order to refine the spatial resolution. Coupling the data from the geodetic and cross section survey and the particle analysis (see below) we were able to document downstream trends in grain size and shape and stream power. Recently, the investigation of the downstream variation in e.g. stream power has gained growing importance in fluvial geomorphology. CitationKnighton (1999) showed the downstream variation of total and specific stream power in rivers of the Trent River catchment (U.K.). CitationReinfels et al. (2004) investigated downstream trends of channel gradient as well as total and specific stream power in the Bellinger River catchment (Australia).
Surface Particle Characteristics
At every measured cross section (except cross section 3) a modified Wolman particle count (100 particles) was carried out in the field. The grain size composition of the bed sediment was determined by sieving the samples in half ϕ-units (ϕ = −log2(D in mm)) electronically using an Excel spreadsheet. The size range of the intermediate axis of all 1200 measured particles is 3 to 1600 mm. It was possible to calculate the principle percentiles as well as the sediment sorting coefficient according to CitationFolk and Ward (1957) knowing the particle size distribution. Finally, the ϕ-values (except the sorting coefficient) were recalculated in metric units.
In addition to the intermediate axis of each particle, the shortest and longest axes were measured to compute the shape of each particle. Particle shape characteristics were determined by applying the widely used triangular shape diagram of CitationSneed and Folk (1958). We used a modified version of the Tri-Plot software developed by CitationGraham and Midgeley (2000) to calculate the particle shape classes.
Results
Longitudinal Profile
The changes of the longitudinal profile of the Partnach River are shown in . The river profile was surveyed in 2003, July/August 2005, and September 2006. After a first flood event in July 2005 detailed geodetic surveys of the channel bottom could only be carried out to a 1050 m downstream distance. Since the survey could not be finished due to the occurrence of the dambreak event, the 2005 profile is shorter. However, the July 2005 event eroded the channel bed significantly (). During the dambreak flood event a large amount of sediment (>105 t) was eroded in the upstream reach (0–300 m) and most of it deposited directly in the downstream reaches (CitationMorche et al., 2007). The channel bed of the Partnach River was aggraded several meters compared to the short July 2005 profile and the entire post-dambreak channel bed is elevated compared to the 2003 profile. The aggradation is about 9 m at the beginning of the profile immediately downstream of the steepest channel reach and decreases in the further river course (). Major plan form changes of the Partnach River channel occurred during the dambreak event when large parts of both banks were disturbed (CitationMorche et al., 2007). During the first post-dambreak field season no significant changes in the plan form were recognized. The lateral input of the main sediment sources in the same year was surveyed by CitationMorche et al. (2008a) and CitationHeckmann et al. (2008) using terrestrial laser scanning and electronic tachymeters.
Downstream Variation of Particle Characteristics
The particle size and shape distributions of the bed material sampled in 2006 are shown in and . The bed material of cross section 1 is very coarse with about 35% boulder size particles, 50% cobbles, and about 15% gravel (CS 1; ), and the upstream gradient is the highest (0.13). Here, the largest particle (1.6 m) moved by the dambreak flood was found (). Also, the D16 (56 mm), D50 (169 mm), and D84 (512 mm) sizes were the coarsest of all of the cross sections (). In general, the particle sizes decrease further downstream with an increasing amount of gravel and cobble particles (). Downstream of cross section 6 only a few or single boulders were found in the cross sections on the Partnach River bed.
Figure 4 Particle size and shape (Sneed and Folk triangular diagram) distributions at the different cross sections, C—compact, P—platy, B—bladed, and V—very.
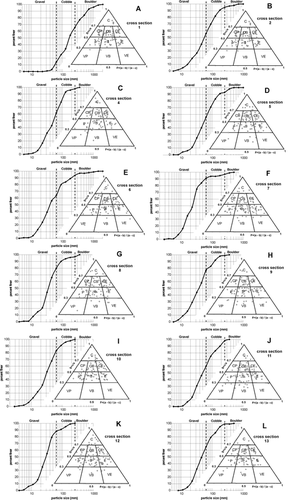
Table 2 Particle size and shape characteristics and hydraulic parameters of the surveyed cross sections at bankfull level. L—distance downstream the dam, D16, 50 ,84—particle size where 16, 50 or 84% are finer, Dmax—largest particle, C—compact, P—platy, B—bladed, E—elongated, VP—very platy, VB—very bladed, VE—very elongated, Ω—stream power, ω—specific stream power, τ0—bed shear stress, I—gradient (m m−1), Q—discharge, V—mean velocity, A—cross sectional area, W—width, R—hydraulic radius, n.d.—not determined. Note: no particles were measured at cross section 3. Therefore, particle size data of cross sections 2 and 4 were averaged and used for the calculation of the bankfull discharge at cross section 3.
Compact-bladed and bladed particles dominated the shape distribution (, ). On the further river course downstream the dominant particle shape is “bladed” immediately followed by “compact bladed” (, ). This shape class was determined to be also dominant for the large talus cone/debris flow complex (CitationMorche and Schmidt, 2005), which is recognized to be the most important sediment source during the dambreak event (CitationMorche et al., 2007) and in 2006, too (CitationHeckmann et al., 2008; CitationMorche et al., 2008a).
Pre- and post-dambreak particle size characteristics show a similar downstream variation. For both conditions, a general downstream fining trend exists (, ). However, while the post-dambreak D50 is coarser than the pre-dambreak one, the D84 is finer ().
Figure 5 Pre-and post- dambreak downstream trends of particle size (pre-dambreak data is taken from CitationMorche et al., 2008b).
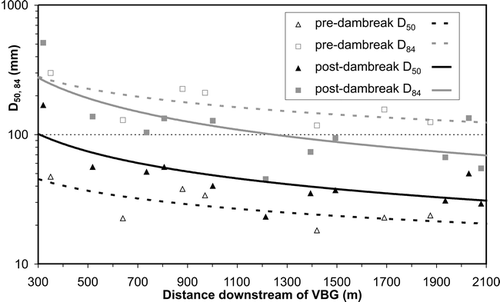
Table 3 Power law regression equations (Y = aXb) between downstream distance (X) and pre- and post-dambreak D50, D84, Ω, ω, and τ0 (all Y) and the levels of determination (R2).
The channel bed sediment consists of a smaller number of particle size classes. Due to the large flood deposits in and nearby the channel, abundant sediment supply can serve as an explanation, bearing in mind that the source of the flood-deposited sediments was one single large talus cone/debris flow complex right below the Vordere Blaue Gumpe (CitationHeckmann et al., 2008; CitationMorche et al., 2007).
Downstream Variation of Stream Power and Bed Shear Stress
Total and specific stream power as well as bed shear stress show clearly visible downstream decreases in the first post-dambreak observation period compared to the gradual declines and power law exponents (<1) of the pre-dambreak trends (, ). Total stream power measured at the beginning of a particular channel reach is one to two orders of magnitude greater after dambreak. Further downstream, a reduced difference between post- and pre-dambreak stream power is evident. In general the post-dambreak values reached at the beginning of the study reach are unusually high. The total stream power of the Partnach River is 8.8 × 105 W m−1 320 m below the former VBG dam and shows the large energy expenditure that could be reached if the bankfull level were reached. But, in fact, the bankfull level is a crucial parameter in this section where most of the eroded material from the first channel reach is deposited. The bankfull level is defined as the lowest value of the width-depth ratio (CitationKnighton, 1998, p. 163). Using this criterion for determining the cross sectional area at the first cross section a value of 80 m2 was determined and used in the continuity equation for calculation of bankfull discharge.
Figure 6 Pre-and post- dambreak downstream trends of stream power and bed shear stress (pre-dambreak data is taken from CitationMorche et al., 2008b).
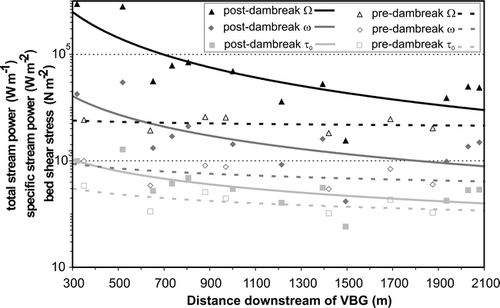
The dambreak outflow must have been like a burst, and the downstream traveling flood wave attenuated more and more with increasing distance. The highly unstable conditions of the event are still imprinted on the river morphology. For example, the bankfull level after the dambreak event is mainly determined by flood deposits which created new and in some cases much higher banks. Bankfull discharge, mean velocity, and cross sectional area generally decrease in the downstream direction (), and the general laws of downstream hydraulic geometry (CitationLeopold and Maddock, 1953) are not fulfilled. So the steep falling of the empirically derived bankfull discharge from cross section 1 to cross section 3 cannot be explained only by transmission losses but also by the large amounts of coarse sediments deposited in that reach on the banks and on the channel bed (CitationMorche et al., 2007).
The new banks consist of loose deposited gravel- to boulder-sized sediments, and are unstable and therefore vulnerable to fluvial erosion. Therefore, it can be expected that during the next flood(s) morphological adjustments will lower the bankfull level (to the pre-dambreak state?) and consequently the total and specific stream as well as bed shear stress power especially in the channel to near the first cross sections.
Discussion and Conclusions
Variation of downstream trends of bed particle characteristics (size and/or shape) can be explained by different underlying bedrock lithologies (CitationKnighton, 1982; CitationWerritty, 1992), sediment supply by tributaries (CitationRice, 1999), and the fluvial processes of selective sorting and abrasion (CitationPowell, 1998; CitationSurian, 2002). In the special case of a dambreak event in the Rubicon River, California, CitationScott (1967) and CitationScott and Gravlee (1968) observed a similar downstream fining of the flood deposited sediments. It is explained with conditions of continuously decreasing competency during the flood and progressive sorting (CitationScott and Gravlee, 1968). Due to the homogeneous lithology of the Reintal Valley, lateral sediment supply and selective sorting were the main controls of the bed material particle characteristics of the Partnach River in pre-dambreak times (CitationMorche and Witzsche, 2006; CitationMorche et al., 2008b). Previous investigations have shown an increase in boulder size immediately downstream of tributary slope channels (CitationMorche and Schmidt, 2005). Debris flows and avalanches mobilize sediment on the slopes and supply the main channel—the way a typical sediment cascade works (CitationBecht et al., 2005). Then the coarsest particles stay in the channel bed while the Partnach River is able to remove the finer ones. After the dambreak flood large amounts of sediment are available to fluvial transport processes. High sediment input from the main sediment sources (e.g. truncated talus cone and Vordere Blaue Gumpe Basin in ) lead to transport conditions of equal mobility even during lower flows. All particle sizes are in motion when sediment is supplied to the channel—a finding that was evaluated, not quantified, during field visits in summer 2007, when a kinematic wave–like sediment slug moving down the disturbed channel reach () was recognized by the first author (see two of the sediment slug), and by bed load measurements (CitationMorche et al., 2008a).
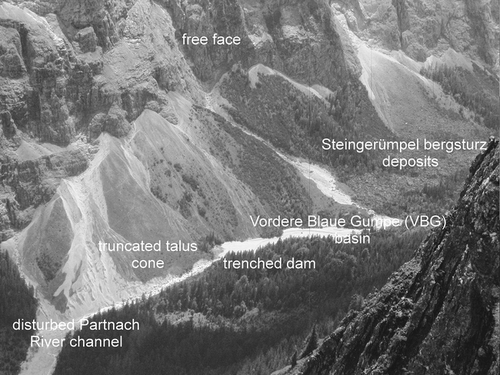
During the first post-dambreak observation period, large amounts of sediment were supplied by different sediment stores along the river course. Once supplied to the Partnach River the sediment was transported during different bed load periods. In total, more sediment was exported from the observed channel reach than supplied from lateral and upstream sources (CitationMorche et al., 2008a). Hence, the sediment balance was negative. The net total annual sediment export from the catchment was 2900 t and about two magnitudes higher than in pre-dambreak times (CitationMorche et al., 2008a). This net export indicates channel bed degradation since the dambreak event in August 2005. But, even in September 2006, one year after the main flood period, the channel bed was higher than before the dambreak (). Consequently, the channel bed immediately after the dambreak flood must have been even much higher.
Although channel bed degradation and sediment export obviously dominated after the dambreak event (annual time scale), the Partnach River system can be placed in Hewitt's phase 3 with trenching of the barrier and downstream sedimentation. The start of phase 4 will be reached when the Partnach River channel bed is below the longitudinal profile of the pre-dambreak conditions (see ).
The first two cross sections are located in the most affected area ( and ) and have very high values of bankfull discharge, stream power, and bed shear stress. The most important factor in their calculation is the bankfull cross sectional area, which was determined strictly according to the CitationKnighton (1998) criterion of the lowest width-depth ratio. Within the most affected channel reaches, which are represented by the first two cross sections, about 63,000 t were deposited during the dambreak flood (CitationMorche et al., 2007). We expect that the channel will adjust to a lower bankfull level in the near future, when the proximal flood deposits, now determining the bankfull level, will be exhausted.
Applying Hewitt's phase model (see above) to the Vordere Blaue Gumpe dam in the Reintal Valley, the following timetable of the different phases can be provided:
Phase 1: around 1800 (landslide),
Phase 2: around 1800 to 2005 (dambreak event),
Phase 3: since the dambreak event 2005 (after incision of the dam),
Phase 4: soon, after the next flood(s),
Phase 5: centuries or even millennia, when sediment stores (0.07 km3 after CitationSchrott et al., 2003) are mainly removed and bed rock contacts surface.
Wherever dambreak events occur, massive destruction and landscape disturbance follow. The degree to which the channel will adjust to the new equilibrium will depend on future discharges, riparian vegetation growth, comminution rates of sediment clasts, sediment transport capacity, and bed armoring processes. Studies of geomorphic impacts of outburst floods in British Columbia have shown that the recovery of a river system to a near pre-event state may take decades (Nostetuko River; CitationClague and Evans, 2000) or even centuries (Noeick River; CitationDesloges and Church, 1992). In order to observe the ongoing response of the Partnach River system to the failure of the Vordere Blaue Gumpe dam in August 2005 in terms of channel and bank adjustment as well as sediment transport dynamics, first approaches (terrestrial laser scanning, bed load measurements, hydrogeomorphological field work) presented by CitationMorche et al. (2008a) and in this study will be widened and intensified in an ongoing research project.
uaar_a_11957376_sm0001.zip
Download Zip (33.7 MB)Acknowledgments
The investigations were funded by the German Research Foundation (DFG, grants to Karl-Heinz Schmidt, SCHM 472/12-1-3 and SCHM 472/15-1). Cars were kindly made available by the Martin-Luther-University Halle-Wittenberg. Special thanks go to our students for assistance during the field work, to the SEDAG-partners (Bonn, Eichstätt, Salzburg) and to the chair of the I.A.G.-working group SEDIBUD Achim Beylich for their cooperation. Driving permissions were kindly provided by the Bayerische Staatsforsten AöR (Garmisch-Partenkirchen/Oberammergau). The comments of the two reviewers are greatly appreciated.
References Cited
- Anderson, M. G. and A. Calver . 1977. On the persistence of landscape features formed by a large flood. Transactions of the Institute of British Geographers New Series 2 2:243–254.
- Bathurst, J. C. and M. Ashiq . 1998. Dambreak flood impact on mountain stream bedload transport after 13 years. Earth Surface Processes and Landforms 23:643–649.
- Becht, M. , F. Haas , T. Heckmann , and V. Wichmann . 2005. Investigating sediment cascades using field measurements and spatial modelling. Wallingford IAHS Press, IAHS Publication 291. 206–213.
- Clague, J. J. and S. G. Evans . 2000. A review of catastrophic drainage of moraine-dammed lakes in British Columbia. Quaternary Science Reviews 19 17–18:1763–1783.
- Costa, J. E. In Baker, V. R. and P. C. Patton . 1988. Floods from dam failures. Flood Geomorphology. New York Wiley. 439–464.
- Desloges, J. R. and M. Church . 1992. Geomorphic implications of glacier outburst flooding: Noeick River valley, British Columbia. Canadian Journal of Earth Sciences 29:551–564.
- Folk, R. L. and W. C. Ward . 1957. Brazos River bar: a study in the significance of grain size parameters. Journal of Sedimentary Petrology 27 1:3–26.
- Götz, J. and L. Schrott . In Kellerer-Pirkelbauer, A. , M. Keiler , C. Embleton-Hamann , and J. Stötter . 2007. A comparison of recent and postglacial sediment fluxes in a paraglacial context. A scale based approach (Reintal, Bavarian Alps). 105–112. Geomorphology for the future, conference proceedings, Obergurgl, Austria, 2nd–7th September 2007.
- Graham, D. J. and N. G. Midgeley . 2000. Graphical representation of particle shape using triangular diagrams an EXCEL spreadsheet method. Earth Surface Processes and Landforms 25:1473–1477.
- Heckmann, T. 2006. Untersuchungen zum Sedimenttransport durch Grundlawinen in zwei Einzugsgebieten der Nördlichen Kalkalpen. 312. Quantifizierung, Analyse und Ansätze zur Modellierung der geomorphologischen Aktivität. Eichstädter Geographische Arbeiten 14.
- Heckmann, T. , V. Wichmann , and M. Becht . 2002. Quantifying sediment transport by avalanches in the Bavarian Alps—First results. Zeitschrift für Geomorphologie Suppl-Bd 127:137–152.
- Heckmann, T. , V. Wichmann , and M. Becht . 2005. Sediment transport by avalanches in the Bavarian Alps revisited—A perspective on modelling. Zeitschrift für Geomorphologie Suppl-Bd 138:11–25.
- Heckmann, T. , F. Haas , V. Wichmann , and D. Morche . 2008. Sediment budget and morphodynamics of an alpine talus cone on different timescales. Zeitschrift für Geomorphologie 52 Suppl I 1:103–121, doi: https://doi.org/10.1127/0372-8854/2008/0052S1-0103.
- Hewitt, K. 2006. Disturbance regime landscapes: mountain drainage systems interrupted by large rockslides. Progress in Physical Geography 30 3:365–393, doi: https://doi.org/10.1191/0309133306pp486ra.
- Hoffmann, T. and L. Schrott . 2002. Modelling sediment thickness and rockwall retreat in an Alpine valley using 2D-seismic refraction (Reintal, Bavarian Alps). Zeitschrift für Geomorphologie Suppl-Bd 127:153–173.
- Hubbard, B. , A. Heald , J. M. Reynolds , D. Quincey , S. D. Richardson , M. Z. Luyo , N. S. Portilla , and M. J. Hambrey . 2005. Impact of a rock avalanche on a moraine-dammed proglacial lake: Laguna Safuna Alta, Cordillera Blanca, Peru. Earth Surface Processes and Landforms 30:12–1264, doi:https://doi.org/10.1002/esp.1198.
- Jarrett, R. D. and J. E. Costa . 1986. Hydrology, geomorphology, and dam-break modelling of the July, 1982 Lawn Lake dam and Cascade Lake dam failures, Larimer County, Colorado. 78. U.S. Geological Survey Professional Paper 1369.
- Keller, D. and M. Moser . 2002. Assessments of field methods for rock fall and soil slip modelling. Zeitschrift für Geomorphologie Suppl-Bd 127:127–135.
- Kershaw, J. A. , J. J. Clague , and S. G. Evans . 2005. Geomorphic and sedimentological signature of a two-phase outburst flood moraine-dammed Queen Bess Lake, British Columbia, Canada. Earth Surface Processes and Landforms 30:1–25, doi:https://doi.org/10.1002/esp.1122.
- Knighton, A. D. 1982. Longitudinal changes in the size and shape of stream bed material: evidence of variable transport conditions. Catena 9:25–34.
- Knighton, A. D. 1998. Fluvial Forms and Processes. A New Perspective. London Arnold.
- Knighton, A. D. 1999. Downstream variation in stream power. Geomorphology 29:293–306.
- Korup, O. 2004. Landslide-induced river channel avulsions in mountain catchments of southwest New Zealand. Geomorphology 63:57–80, doi: https://doi.org/10.1016/j.geomorph.2004.03.005.
- Krautblatter, M. , M. Moser , L. Schrott , and J. Wolf . in press. A study on sediment yield and geomorphic work comprising all rockfall magnitudes in an Alpine Catchment (Reintal, German Alps). Geomorphology.
- Leopold, L. B. and T. Maddock . 1953. The hydraulic geometry of stream channels and some physiographic implications. U.S. Geological Survey Professional Paper 252.
- Morche, D. 2006. Aktuelle hydrologische Untersuchungen am Partnach-Ursprung (Wettersteingebirge). Wasserwirtschaft 96:53–58.
- Morche, D. and K. H. Schmidt . 2005. Particle size and particle shape analyses of unconsolidated material from sediment sources and sinks in a small Alpine catchment (Reintal, Bavarian Alps, Germany). Zeitschrift für Geomorphologie Suppl-Bd 138:67–80.
- Morche, D. and M. Witzsche . In Rowan, J. , R. W. Duck , and A. Werritty . 2006. Spatial and temporal variation in grain size distributions of alluvial deposits in an Alpine catchment. Sediment Dynamics and the Hydromorphology of Fluvial Systems 39–46. Poster Report Booklet International Symposium IAHS/ICCE, 3rd–7th July 2006, Dundee Scotland.
- Morche, D. , C. Katterfeld , S. Fuchs , and K-H. Schmidt . 2006. The life-span of a small high mountain lake, the Vordere Blaue Gumpe in Upper Bavaria, Germany. Wallingford IAHS Press, IAHS Publication 306. 72–81.
- Morche, D. , K-H. Schmidt , T. Heckmann , and F. Haas . 2007. Hydrology and geomorphic effects of a high magnitude flood in an Alpine river. Geografiska Annaler 89 A 1:5–19, doi: https://doi.org/10.1111/j.1468-0459.2007.00304.x.
- Morche, D. , K-H. Schmidt , I. Sahling , M. Herkommer , and J. Kutschera . 2008a. Volume changes of Alpine sediment stores in a state of post-event disequilibrium and the implications for downstream hydrology and bed load transport. Norsk Geografisk Tidsskrift 62:89–101, doi: https://doi.org/10.1080/00291950802095079.
- Morche, D. , M. Witzsche , and K-H. Schmidt . 2008b. Hydrogeomorphological characteristics and fluvial sediment transport of a high mountain river (Reintal Valley, Bavarian Alps, Germany). Zeitschrift für Geomorphologie 52 Suppl I 1:51–77, doi: https://doi.org/10.1127/0372-8854/2008/0052S1-0051.
- Pitlick, J. 1993. Response and recovery of a subalpine stream following a catastrophic flood. Geological Society of America Bulletin 105:657–670.
- Powell, D. M. 1998. Patterns and processes of sediment sorting in gravel-bed rivers. Progress in Physical Geography 22 1:1–32.
- Reinfels, I. , T. Cohen , P. Batten , and G. Brierley . 2004. Assessment of downstream trends in channel gradient, total and specific stream power: a GIS approach. Geomorphology 60:403–416, doi: https://doi.org/10.1016/j.geomorph.2003.10.003.
- Rice, S. 1999. The nature and controls on downstream fining within sedimentary links. Journal of Sedimentary Research 69 1:32–39.
- Sass, O. , M. Krautblatter , and D. Morche . 2007. Rapid lake infill following major rockfall (bergsturz) events revealed by ground-penetrating (GPR) measurements, Reintal, German Alps. The Holocene 17 7:965–976, doi: https://doi.org/10.1177/0959683607082412.
- Schmidt, K. H. and D. Morche . 2006. Sediment output and effective discharge in two small high mountain catchments in the Bavarian Alps, Germany. Geomorphology 80:131–145, doi: https://doi.org/10.1016/j.geomorph.2005.09.013.
- Schneevoigt, N. J. and L. Schrott . 2006. Linking geomorphic systems theory and remote sensing. A conceptual approach to Alpine landform detection (Reintal, Bavarian Alps, Germany). Geographica Helvetica 61:181–190.
- Schneevoigt, N. J. , S. van der Linden , H. P. Thamm , and L. Schrott . 2008. Detecting Alpine landforms from remotely sensed imagery. A pilot study in the Bavarian Alps. Geomorphology 93:104–119, doi: https://doi.org/10.1016/j.geomorph.2006.12.034.
- Schrott, L. , A. Niederheide , M. Hankammer , G. Hufschmidt , and R. Dikau . 2002. Sediment storage in a mountain catchment: geomorphic coupling and temporal variability (Reintal, Bavarian Alps, Germany). Zeitschrift für Geomorphologie Suppl-Bd 127:175–196.
- Schrott, L. , G. Hufschmidt , M. Hankammer , T. Hoffmann , and R. Dikau . 2003. Spatial distribution of sediment storage types and quantification of valley fill deposits in an alpine basin, Reintal, Bavarian Alps, Germany. Geomorphology 55:45–63.
- Schrott, L. , J. Götz , M. Geilhausen , and D. Morche . 2006. Spatial and temporal variability of sediment transfer and storage in an Alpine basin (Reintal valley, Bavarian Alps, Germany). Geographica Helvetica 61:191–200.
- Scott, K. M. 1967. Downstream changes in sedimentological parameters illustrated by particle distribution from a breached rockfill dam. Wallingford IAHS Press, IAHS Publication 75. 309–318.
- Scott, K. M. and G. C. Gravlee . 1968. Flood surge on the Rubicon River, California—Hydrology, hydraulics, and boulder Transport. 1–40. U.S. Geological Survey Professional Paper 422-M.
- Sneed, E. D. and R. L. Folk . 1958. Pebbles in the lower Colorado River, Texas—A study in particle morphogenesis. Journal of Geology 66:114–150.
- Surian, N. 2002. Downstream variation in grain size along an Alpine river: analyses of controls and processes. Geomorphology 43:137–149.
- Unbenannt, M. 2002. Fluvial sediment transport dynamics in small alpine rivers—First results from two Upper Bavarian catchments. Zeitschrift für Geomorphologie Suppl-Bd 127:197–212.
- Vuichard, D. and M. Zimmermann . 1987. The 1985 catastrophic drainage of a moraine dammed lake, Khumbu Himal, Nepal: cause and consequences. Mountain Research and Development 7:91–110.
- Walder, J. S. and J. E. Costa . 1996. Outburst floods from glacier-dammed lakes: the effect of mode of lake drainage on flood magnitude. Earth Surface Processes and Landforms 21:701–723.
- Werritty, A. In Billi, P. , R. D. Hey , and C. R. Thorne . 1992. Downstream fining in a gravel-bed river in southern Poland—Lithologic controls and the role of abrasion. Dynamics of Gravel-Bed Rivers. Wiley Chichester. 333–350.