Abstract
This study reviews carbon stocks and carbon dynamics in different types of forest land in Italy: ordinary managed forests, forest plantations, old growth forests, and trees outside forests. Forest management, combined with global environmental changes, increases the capacity of carbon uptake of ordinary managed forests. Forest plantations, particularly the ones subject to short‐rotation forestry systems, potentially have high soil carbon accumulation, especially in agricultural lands. Old growth forests, recently discovered as a carbon sink, cover a significant surface area in Italy. Moreover, the trees outside forests may represent a sensible carbon stock, especially in the context of urban environments. Our study points out the management actions that can be implemented in Italy to increase the carbon stocks of different forest ecosystems, such as increasing the mean annual increment in managed forests, enhancement of the national network of old growth forests, and expansion of forest plantations in suitable areas. These aspects have important implications after the recent recognition of the Land Use, Land Use Change and Forestry sector in the EU target within the 2030 Climate and Energy Policy Framework.
Introduction
Forests, which are the main component of so‐called “land sinks,” play a vital role in the global carbon cycle through the absorption of 2.9 ± 0.8 Pg of carbon (C) per year (in the period 2004–2013), thus mitigating climate change related to the increase of anthropogenic carbon dioxide (CO2) in the atmosphere (Le Quéré et al. 2014). Human activities, however, negatively affect carbon sequestration through deforestation and forest degradation, resulting in emissions of ~1.2 Pg C/yr, corresponding to 12% of total CO2 anthropogenic emissions (Citationvan der Werf et al. 2009). The sustainable management of forest resources, therefore, was fully included in the negotiations for the second commitment period of the Kyoto Protocol (2013–2020). The Durban Climate Change conference, which ended on 11 December 2011, marked a turning point for the rules and procedures for the agro‐forestry sector for countries with emissions reduction targets, i.e., the sector known in the negotiating jargon as Land Use, Land Use Change, and Forestry (LULUCF). The rules defined in Durban (CitationUNFCCC 2011) introduced substantial changes with respect to the rules established for the first commitment period (2008–2012) within the Marrakech Accords (CitationUNFCCC 2001). These include the identification of new activities, the mandatory accounting of forest management with a new forest carbon accounting method, the recognition of carbon stored in woody products, and the possibility of excluding part of the emissions arising from natural disturbances, such as exceptional fires. The accounting of emissions/removals resulting from activities of reforestation and deforestation (Article 3.3) remains unchanged, as does the voluntary accounting of activities such as cropland management, rangeland management, and revegetation (Article 3.4), with the addition of a new activity, i.e., wetland drainage and rewetting.
The new accounting method of credits/debts generated from forest management is based on the difference between the net balance of CO2 per year occurring in managed forests in the second commitment period (2013–2020) and a reference level defined for each country. Debts are generated if the absorption decreases compared to the reference level and credits are generated if there is a rise. For the EU countries, the reference level is the amount of sequestration expressed as Tg CO2/yr projected for the period of commitment, referring to a “business as usual” scenario. This calls for forest management policies capable of increasing forest carbon sinks in comparison with the condition expected in the absence of changes in current policies. To comply with the commitments proposed by Italy under the Climate‐Energy Package of the EU, however, there is also a need for the development of a national policy to support renewable energy production from forest biomass. While negotiations for the post‐2020 agreement under the UNFCCC are still ongoing, the EU has defined its own target within its Climate Policy Framework 2030 (2021–2030) to reduce greenhouse gas (GHG) emissions to 40% below 1990 levels by 2030.
Although the potential contribution of the LULUCF sector to achieving GHG reduction pledges is expected to be relatively modest for the EU (up to 2% of 1990 emissions in 1990), compared to other Annex I parties (CitationGrassi et al. 2012), the inclusion of the sector in European climate policies represents a first step for the recognition of the importance of land resources as mitigation option (CitationFares et al. 2015). In this context, forest management policies can effectively promote carbon storage, acting at different levels (CitationCorona and Barbati 2010) by: (1) saving carbon stock by reducing losses due to harvesting or disturbances such as fire; (2) increasing carbon stock through the implementation of longer rotation cycles or the creation of forest plantations that favor the sequestration and persistence of carbon in plants and soils (sinks); and (3) using biomass as a substitute energy source to reduce CO2 emissions from fossil fuels by implementing sustainable management that does not compromise the sink potential of Italian forests.
In the view of this composite scenario, this study examines the role that different types of forest carbon sinks may play in the dynamics of carbon in Italy, starting from a background that considers the processes of C mobilization in forest reservoirs and related pools (Accounting for carbon sequestered in the soil–plant‐atmosphere system); accordingly, the role of forest management strategies in the process of carbon sequestration is discussed, with the aim of assessing the mitigation potential and most appropriate policy options applicable in different operational contexts: managed forests (Carbon sequestration in managed forests: Ordinary managed forests) and forest plantations (Carbon sequestration in managed forests: Forest plantations); old growth forests (Old growth forests); and trees outside forests (Trees outside forests). The study finally discusses the implications related to global changes (Effects of global environmental changes) and future needs to optimize the carbon credits accounting system in Italy (Implications and perspective post–2020).
Accounting for Carbon Sequestered in the Soil–Plant–Atmosphere System
Carbon pools are the reservoirs of this element in a terrestrial ecosystem. The forest carbon pools considered in the accounting system of the IPCC are the above‐ and belowground biomass, litter, dead wood, and soil organic carbon (Penman 2003).
Among these pools, soil is the most important. Soil organic carbon makes up around two‐thirds of the terrestrial ecosystem's carbon, two times higher than the atmospheric carbon content (CitationSchlesinger 1995, CitationScharlemann et al. 2014). In the soil, we find both inorganic and organic carbon. The main component is organic carbon that is stored in soil organic matter (SOM). This is a dynamic entity and a function of residence time, that is, the time required by photosynthesized carbon to be cycled back to the atmosphere through respiratory processes (CitationLuo et al. 2001). Three different carbon fractions can be identified depending on their residence time (CitationBrady and Weil 1999): (1) the active fraction, composed of material with high ratios between carbon and nitrogen (N), such as polysaccharides and fulvic acids. It is the substrate preferred by soil microorganisms and comprises 10–20% of SOM. (2) The passive fraction, made up by humic colloids, which can stay in the soil for thousands of years. It is the majority of SOM (60–90%). (3) The low fraction, which has intermediate properties, and substrates high in lignin content and other recalcitrant compounds.
Residence time is also variable in other forest carbon pools (CitationGaudinski et al. 2000). Pools with short and long residence times can be identified. The former includes litter and fine roots, which are the main inputs of nutrients into the soil. The latter includes dead wood, with a residence time that can vary depending on the microbial community, climatic condition, forest type (sensu CitationBarbati et al. 2007), and dead wood size. Dead wood is classified as snags (standing dead trees), dead stumps, coarse woody debris (CWD), and fine woody debris. Dead wood is strongly influenced by forest management: managed forests usually have a relatively low dead wood content due to snag and CWD removal.
The relevance of soil in the carbon balance depends on the capacity to store carbon in pools with long residence time. Nevertheless, the soil carbon pool is not included in the Italian accounting system for carbon credits/debts of the commitment period 2013–2020. In fact, following the main finding of the 2011 review process of the national GHG inventory report, Italy has decided not to account for soil carbon stock changes from activities under Article 3.4, and has provided the requested information to demonstrate that the soils pool is not a source (CitationRomano et al. 2014).
The amount of carbon sequestered by forest ecosystems is equivalent to the net ecosystem productivity (NEP), that is, the biomass increment in the various pools over a certain period. NEP is a mass balance, the result of inputs and outputs of the processes controlling carbon movement in the soil–plant–atmosphere system. The net ecosystem carbon balance (NECB) can be estimated (CitationChapin et al. 2006) as
where NPP is net primary productivity. Subtracted from NPP are heterotrophic respiration (Rh), carbon losses due to forest management and harvest (H), carbon losses due to wildfire (F), leaching of inorganic and organic carbon dissolved in water (DIC and DOC, respectively), carbon losses as methane (CH4), and carbon losses in the form of organic volatile compounds (VOC).
Commonly, undisturbed forest ecosystems show higher NECB values and are considered carbon sinks (CitationHyvönen et al. 2007, CitationMagnani et al. 2007). In European forests, the annual increment of the carbon stock in woody biomass for the period 2005–2010 is estimated to be 0.53% and becomes 1.42% when the Russian Federation, where forest management practice typically removes more wood, is excluded (Forest Europe, UNECE, and CitationFAO 2011).
Forest management strategies have a substantial influence on NPP, Rh, H, and, indirectly, F components (CitationKolström et al. 2011). The NECB is, therefore, the result of complex interactions between environmental factors (water availability, nitrogen deposition, and climatic variability and extremes) and forest ecosystems. Harvests and disturbances represent confounding factors for predicting changes of the carbon sink potential in a global change scenario (CitationHeimann and Reichstein 2008, CitationLindner et al. 2010).
Carbon Sequestration in Managed Forests
Managed forests form the largest fraction of forests in the Northern Hemisphere and play a significant role in the global carbon cycle (CitationSchimel et al. 2001, CitationFares et al. 2015). In recent years, the productivity of managed forests has increased both at European (CitationSpiecker 2002) and global scales (CitationBoisvenue and Running 2006). Forest management is considered one of the possible drivers of rising levels of forests productivity in temperate forests (CitationCiais et al. 2005). In a more local study, for instance, forest management explained 50% of the increase in carbon accumulation in coniferous forests in Thuringia, Germany during the last century, while indirect human effects (increasing of CO2 and temperature, nitrogen deposition) explained 33% (CitationVetter et al. 2005).
Ordinary managed forests
The land‐use inventory (Inventario dell'Uso delle Terre; IUTI), the key instrument of the National Registry for forest carbon sinks in Italy, allows us to estimate, with a high level of statistical accuracy, the area covered by forest land in Italy that is eligible for forest management activities under the Kyoto protocol. Forest land amounted to 9-653 216 ha (standard error [SE] = 0.1%) at the beginning of the first commitment period (2008), showing an increase of 5.6% when compared to 1990 values (CitationCorona et al. 2012, CitationBarbati and Corona 2015). Most forest land in Italy can be regarded as “ordinary managed,” meaning that timber harvesting is regulated, at the very least, by regional forestry laws setting, e.g., the minimum rotation length and the size of harvest blocks.
The annual variation in aboveground C in forest land in Italy is estimated to range from +5.9 to +8.7 Tg according to the default CitationIPCC approach (2003) and from +7.0 to +8.6 Tg according to the stock change procedure (CitationTabacchi et al. 2010). Using a modeling approach, CitationNolè et al. (2015) estimated that most of total NPP of Italian forests belongs to the deciduous mixed oak woods (~8 Tg C/yr) followed by the Mediterranean shrub land (~6.5 Tg C/yr), while the minor contribution is related to the hygrophilous forests (~0.2 Tg C/yr; ).
Table 1. Total net primary production (all carbon pools considered) of Italian forest ecosystem (modified from CitationNolè et al. 2015).
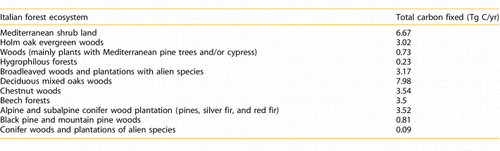
In Italy, key issues to promote forest carbon storage are the recovery of the ecological efficiency of forests, which in many cases have been overexploited for thousands of years, and the prevention of wildfires that dramatically offset GHG gains in forest areas (CitationChiriacò et al. 2013). In this perspective, forest management policies should aim at: (1) the restoration of forest stands degraded by past intensive logging; at least 1.3 Tg/yr of annual increase in carbon sequestration might be achieved through this measure (CitationCorona et al. 1997); (2) promoting a gradual increase of forest growing stock and, possibly, the adoption of longer rotation cycles in old/healthy forests that are at low risk from pests or environmental disturbances (CitationFares et al. 2015), including fires; (3) the conversion of coppice forest into high forest stands, where technically and economically viable; this action would bring positive effects on above‐ and belowground biomass accumulation (CitationCiancio et al. 2006); (4) reducing vulnerability to forest damage by wildfires by implementing proper forest fuel management techniques (CitationCorona et al. 2015) in forest lands covered by highly flammable forest types (CitationCorona et al. 2014).
In order to reduce the impact of forest harvesting operations on the Rh term and to raise NPP levels in the long term (see Accounting for carbon sequestered in the soil–plant‐atmosphere system), distinctive operational guidelines can be suggested, via: reducing the maximum size of clear‐cut harvest blocks; treatments favoring continuous tree cover; supporting natural regeneration that increases potential adaptation to climate change (e.g., drought) by favoring a mixture of forest species and local genotypes; and applying low‐impact harvesting methods to provide minimum disturbance to the soil, remaining vegetation, and extracted trees. This practice positively influences carbon stock change from trees left in the forest after harvest and the growth (and corresponding carbon storage) of new trees and vegetation.
Forest plantations
Forest plantations are intensively managed forest ecosystems, established artificially on croplands by planting or seeding. Forest plantations cover a relatively small surface in Italy, estimated as high as 144-376 ha (SE = 1877 ha) in 2008 (CitationCorona et al. 2012). Yet, plantations have a high carbon uptake potential, especially concerning their contribution to soil carbon accumulation. shows the estimated total NPP for Italian forest ecosystems: the major role for forest plantations is played by alpine silver and red fir plantations (~3.5 Tg C/yr) followed by broadleaved and alien species plantations (~3 Tg C/yr) and Mediterranean coniferous plantation (~0.8 Tg C/yr).
In the case of short‐rotation forestry (SRF), which is characterized by very short rotations (< 5 yr), land‐use conversion from farmland may bring an increase in the soil organic carbon content (SOC) of 0.3–3 Mg·ha−1·yr−1 (CitationPost and Kwon 2000). Even though an initial decline of SOC after the establishment of the SRF is possible (CitationHansen 1993), after 5 yr, there is a clear tendency toward an increase in SOC (CitationGrigal and Berguson 1998). For instance, a case study carried out in Italy (CitationScarascia‐Mugnozza et al. 2000) on poplar SRF shows that the increase in soil carbon content for the first 18 yr following the conversion of a maize cropland to SRF is in the order of 3 Mg CO2‐equivalents·ha−1·yr−1 and 8 Mg·ha−1·yr−1 in the aboveground biomass (CitationLiberloo et al. 2010).
The potential for carbon sequestration in European soils of forest plantations is confirmed by scenarios provided by CitationSmith et al. (1997), who estimated that afforestation of 30% of the European Union arable lands would increase soil carbon stocks by ~8% over a century. While the aboveground biomass from the SRF plantations is always used as a carbon‐neutral substitute fuel, and thus returns quickly to the atmosphere, the roundwood from other kinds of forest plantations (such as e.g., ordinary poplar plantations, characterized by rotation length longer than 9 yr) and ordinary managed high forests is mainly exploited for long‐lasting timber products (CitationBarbati et al. 2014). In recent years, the use of wood for construction purposes has replaced traditional material with higher energy costs, thus increasing the carbon sequestration (CitationMarchetti et al. 2015). Indeed, it should be also noticed that the potential supply of woody biomass for energy purposes from ordinary managed forests in Italy would largely satisfy the demand deriving from household consumption (CitationMaesano et al. 2014).
Model simulations carried out to compare the benefits for carbon sequestration of afforestation with a multifunctional oak–beech forest vs. a poplar SRF indicate that SRF reduces emissions by 24.3–29.3 Mg CO2·ha−1·yr−1, while the mixed forest reduces only 6.2–7.1 Mg CO2·ha−1·yr−1 (CitationDeckmyn et al. 2004). Even though SRF has high potential for carbon sequestration, a number of issues need to be addressed before SRF could be established widely on a national scale. Expansion of SRF plantations on arable land primarily depends on the economic viability for farmers, i.e., the costs/benefits associated with SRF compared with traditional cropland. Further, the high water use by some species (e.g., poplar, eucalypts) may significantly limit the area of land suitable for the establishment of SRF, e.g., in regions vulnerable to drought. In this respect, the activities of tree genetic improvement are also becoming more and more important in Italy, with the aim to select varieties that are able to survive in harsh environments and/or to maximize biomass production and thus carbon sequestration (CitationHarfouche et al. 2011).
A nationwide assessment of land suitability for the establishment of new forest plantations in Italy (reforestation or SRF) has been carried out in the framework of the FISR‐CARBOITALY project (CitationPapale 2006). The assessment was performed for a selection of target species suitable for reforestation (Pinus halepensis, Pinus pinaster, Quercus ilex, Q. cerris, Pseudotsuga menziesii, Alnus cordata) or SRF plantations (Salix alba, Populus alba, Populus × euroamericana). The environmental optima of each target species was modeled based on data in the literature. Farmland areas where the target species find their respective optimal ecological conditions (i.e., areas suitable for the establishment of the SRF or reforestation) were mapped by GIS techniques, on the basis of four environmental factors for which geodata sets were homogeneously available at national scale: mean annual precipitation, mean annual temperature, drought indices, soil depth, and soil texture. Carbon sequestration potential in suitable lands () was modeled using simple equations based on national average values for each species of mean annual increment (CitationGasparini et al. 2005), biomass expansion factor (CitationIPCC 2003), and basal density (CitationISPRA 2011).
Table 2. Suitable farmland area in Italy for the potential establishment of forest plantations and related carbon sequestration (above‐ and belowground biomass) potential.
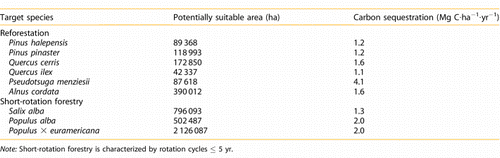
The optimum areas of target species overlap in some regions. From the perspective of optimizing carbon sequestration, establishing a mix of different species in these areas, possibly with different light requirements and/or growth rates (e.g., fast‐growing species improving the site conditions toward the optimum of slow‐growing species) is highly recommended. Mixed plantations contribute to improving the quality of SOC, and are more resistant to pests and diseases, besides being more aesthetically appealing (CitationBravo‐Oviedo et al. 2014). Moreover the suitable area selection should also take in consideration the main factors responsible for the SOC increase (CitationLaganiere et al. 2009): the tree species used (broadleaf tree species accumulate more SOC than conifers), the previous land use (there is more SOC accumulated when the afforestation is carried on over cropland than pasture), and the clay soil content (clay‐rich soils accumulate more SOC).
Old Growth Forests
Old growth forests are the products of structures and processes associated with the maturation and senescence of populations of trees under very low levels of anthropogenic disturbance (see Accounting for carbon sequestered in the soil–plant–atmosphere system, H term) for an extended period of time. This allows the development of a relatively high degree of structural complexity compared with ordinary managed forests.
The role of old growth forests as carbon sinks was underestimated in the past (CitationMotta 2008). Recent research based on a wide variety of case studies highlights, instead, a very active role of old growth forests in carbon sequestration, even in late phases of the biological cycle (CitationZhou et al. 2006, CitationLuyssaert et al. 2008). According to CitationLuyssaert et al. (2008) old growth forests represent about one‐third of global forests and ~50% of temperate and boreal forests and store 1.3 ± 0.5 Pg carbon per year, ~10% of global ecosystem net production (GENP).
In Italy, forest stands with old growth features amount to 93-100 ha (CitationBarbati et al. 2012). CitationPiovesan et al. (2010) quantified carbon stocks in different ecosystem carbon pools on a subset of Italian old growth beech forest sites. Preliminary carbon stock results indicate that these old growth forests are important carbon sinks, with 192–268 Mg C/ha of total biomass (67–73% aboveground; 27–33% belowground) and 7–21 Mg C/ha of dead wood. In these stands, forest floors (excluding dead wood) and soils also store an important amount of carbon (5–9 Mg C/ha litter layer; 168–420 Mg C/ha mineral soil). In general, carbon stocks of these forests are higher than in other managed stands, both in Italy and in Europe. In a recent analysis of biomass data coming from the forests of the national parks of Italy, mainly designed for nature conservation, CitationMarchetti et al. (2012) found that those can be considered a relevant C sink and confirmed the influence of both ecological conditions and management on C sequestration.
Trees Outside Forests
The category trees outside forests (TOF) usually includes woodlands located in rural and urban areas not strictly included within the category forests (as reported by CitationForest Europe et al. 2001): small woodlands, linear forest plantations (tree lined roads, windbreak trees), and scattered forest trees (CitationDe Foresta et al. 2013).
A study carried out by CitationCorona et al. (2009) provides an estimate of the sink capacity of TOF in nonurban areas in Italy: the carbon stored in the dendromass of TOF in plots across the Italian territory (sample representing ~1% of the total national area) is ~121 Mg/km2. Scaling this estimate up results in ~30 Tg/yr carbon stored by TOF plus ~1 Tg C present in the aboveground dendromass, nationally. These values are not negligible considering that the estimate does not include the carbon stored in the litter and in the soil.
The role of TOF on the carbon balance increases when we consider urban forests, i.e., TOF situated in urban areas. Within this definition we can include historical parks, newly established parks, or woodlands in peri‐urban areas. In particular, the establishment of new forests in urban environments seems to be more independent of the traditional system of public funding and more and more driven by the Voluntary Agreements Market for CO2 compensation (CitationGiulietti 2010). In Italy, 43-000 ha of urban forests have been estimated, with a mean area of 2.2 ha each (CitationCorona et al. 2011). Therefore, the potential contribution of these forests to reductions in CO2, as well as to reductions in atmospheric pollutants like oxides, hydrocarbons, and particulate matter, cannot be ignored.
The role for urban forests in CO2 control is not only directly related to CO2 absorption for photosynthesis and consequent carbon storage in the woody tissues of the plant, but also, indirectly, to the reduction in CO2 emissions resulting from energy conservation. This aspect is very important for microclimate regulation in the urban environment where urban trees reduce the heat island effect during warm seasons and provide a windbreak effect in cold seasons. The amount of CO2 emissions saved is more relevant in the hinterlands than in coastal areas since a continental climate induces bigger power consumption both for house heating and cooling.
A number of studies carried out, especially in the United States, estimate the CO2 control potential of urban forests. One of these studies in Tucson (Arizona, USA) estimated that 300 trees of different species in the residential area contributed 6000 Mg of CO2 saved over 40 years, with one‐fifth due to CO2 uptake and the remainder to energy saving, mostly to air cooling systems, considering the high temperatures recorded in this city (CitationCrema 2008). Assessments generated by models in several cities in the United States suggest that CO2 uptake by urban forests is in the order of hundreds of kg C·ha−1·yr−1 (CitationNowak et al. 2008) notwithstanding urban forest loss, on average, of 15% of stored carbon due to pruning activities and consequent decomposition (CitationJo and McPherson 1995).
In Europe, and particularly in Italy, there are few available studies of potential carbon storage by urban forests. In Liverpool (UK), a carbon uptake ranging between 17 Mg/ha in areas with higher tree density and 1 Mg/ha in areas with poor tree cover has been estimated in several residential areas, considering the entire life of those trees (CitationWhitford et al. 2001). In Italy assessments generated by different methodologies report values of 160 Mg CO2/yr (7.3 Mg·ha−1·yr−1) sequestered by the Parco Ducale's trees in Parma (R. Baraldi, personal communication), and of 54 Mg C/yr (0.7 Mg·ha−1·yr−1) in the Villa Borghese Park in Rome (C. Calfapietra and A. Morani, unpublished data).
Effects of Global Environmental Changes
Global environmental changes can have contrasting effects on forest carbon sequestration: although future changes in precipitation regimes are still uncertain at both local and global scales (CitationTrenberth et al. 2003), several models show a likely decrease of precipitation during the growing season in the Mediterranean area (CitationGiorgi and Lionello 2008). Reduced water input can strongly reduce NPP, especially in natural forest ecosystems (CitationCiais et al. 2005), while forest plantations and trees outside forest might be less sensitive to the water shortage through proper irrigation practices (CitationHeilman and Norby 1998, CitationMorani et al. 2014). Future atmospheric CO2 concentration coupled with rising temperature is expected to stimulate plant growth and carbon sequestration in natural ecosystems (CitationDufresne et al. 2002) as well in SRF plantations (CitationCalfapietra et al. 2003, CitationLiberloo et al. 2009). To sustain this increase in carbon sequestration and plant growth under future environmental conditions requires an increase of both N uptake from the soil and/or N‐use efficiency (CitationCalfapietra et al. 2007, CitationFinzi et al. 2007). It is widely accepted that N deposition overcomes the limitation in nitrogen availability (CitationJanssens and Luyssaert 2009) that can limit photosynthetic rates and consequently primary production. However, often the data used as input in the forest ecosystem models are coming from single‐factor responses from short‐term experiments and must be treated carefully when projected to the long term (CitationHyvönen et al. 2007).
Implications and Perspective Post‐2020
The different contexts presented suggest some possible directions to be considered in Italy to improve carbon absorption capacity in both the short and medium term through the correct management and planning of the main forest sinks. The direction undertaken by the EU with its decision on accounting rules for LULUCF (Decision 529/2013/EU) paves the way for a more comprehensive accounting of the land use sector, having also included in the reporting for the period 2013 and 2020 “cropland management” and “grassland management” as obligatory activities. The revision of such decision within the 2030 EU policy framework will have to follow the direction that will lead to a full accounting of the land sector.
Possible actions linked to the land sector could be developed using the following guideline recommendations: increase the mean stock units in coppices and mature forests; expand the old growth forests national network, through a specific conservation policy; increase forest areas through permanent forestation/reforestation in appropriate agricultural or abandoned lands or active conservation of the areas under natural recolonization; create integrated land and energy policy promoting SRF that represents a fast carbon sequestration option both in the soil and in the above‐ and belowground biomass while contributing to the production of biomass for energy uses. Moreover, the SRF culture's flexibility offers the possibility of adapting rotations and density to maximize productivity depending on climate change factors (CitationCalfapietra et al. 2010). Another guideline is a possible future increase of areas classified as TOF as an effect of the first (through greening measures) and second pillars of the European Common Agricultural Policy (2013–2020) through the measures listed in the Plans for Rural Development, for the establishment of woods, buffer zones, and riparian areas.
Finally, it is important to stress that, although in the first period of commitment no value was attributed to wood and its derivatives, the current accounting rules for LULUCF allow inclusion of this category in the calculations for the commitment period 2013–2020 and their accounting will be most likely retained in the post‐2020 set of LULUCF rules. Actually, the carbon stored in wood cannot be considered an emission yet, but an amount of frozen carbon that will be released into the atmosphere depending on the life cycle of the woody product (credit of the so‐called biological CO2). The possibility to account for the carbon credit connected with the “carbon pool” of woody products could represent an incentive for the production of long‐lasting woody products, with the indirect benefit of decreasing emissions from other materials with high emissions factors like cement, by substitution. Nevertheless, the accountability of this aspect requires the creation of accurate database of different types of woods supplied by our forests and their eventual exports.
Acknowledgments
We acknowledge Penny Tricker, University of Adelaide (Australia), for language revision of the manuscript as well as Ettore d'Andrea for useful information regarding unpublished data for old growth forests.
Literature Cited
- Barbati, A., and P. Corona. 2015. Chapter 4. The role of managed forest ecosystem: an inventory approach. Pages 61–70 in R. Valentini, and F. Miglietta, editors. The greenhouse gas balance of Italy. Springer-Verlag, Berlin, Germany.
- Barbati, A., P. Corona, and M. Marchetti. 2007. A forest typology for monitoring sustainable forest management: the case of European Forest Types. Plant Biosystems 1: 93–103.
- Barbati, A., B. Ferrari, A. Alivernini, A. Quatrini, P. Merlini, N. Puletti, and P. Corona. 2014. Sistemi forestali e sequestro del carbonio in Italia. L'Italia Forestale e Montana 69: 205–212.
- Barbati, A., R. Salvati, B. Ferrari, D. Di santo, A. Quatrini, L. Portoghesi, D. Travaglini, F. Iovino, and S. Nocentini. 2012. Assessing and promoting old-growthness of forest stands: lessons from research in Italy. Plant Biosystems 146: 167–174.
- Boisvenue, C., and S. W. Running. 2006. Impacts of climate change on natural forest productivity—evidence since the middle of the 20th century. Global Change Biology 12: 862–882.
- Brady, N., and R. Weil. 1999. The nature and properties of soils. Prentice-Hall, Upper Saddle River, New Jersey, USA.
- Bravo-oviedo, A., et al. 2014. European mixed forests: definition and perspectives. Forest Systems 3: 518–533.
- Calfapietra, C., et al. 2007. Nitrogen use efficiency of a short-rotation poplar plantation is increased under elevated CO2. Tree Physiology 27: 1153–1163.
- Calfapietra, C., B. Gielen, A. Galema, M. Lukac, P. De angelis, M.C. Moscatelli, R. Ceulemans, and G. Scarascia-mugnozza. 2003. Free-air CO2 enrichment (FACE) enhances biomass production in a short-rotation poplar plantation. Tree Physiology 23: 805–814.
- Calfapietra, C., B. Gielen, D. Karnosky, G. E. Scarascia-mugnozza, and R. Ceulemans. 2010. Response and potential of agroforestry crops under global change. Environmental Pollution 158: 1095–1104.
- Chapin, F., et al. 2006. Reconciling carbon-cycle concepts, terminology, and methods. Ecosystems 9: 1041–1050.
- Chiriacò, M. V., L. Perugini, D. Cimini, E. D'amato, R. Valentini, G. Bovio, P. Corona, and A. Barbati. 2013. Comparison of approaches for reporting forest fire-related biomass loss and greenhouse gas emissions in southern Europe. International Journal of Wildland Fire 22: 730–738.
- Ciais, P., et al. 2005. Europe-wide reduction in primary productivity caused by the heat and drought in 2003. Nature 437: 529–533.
- Ciancio, O., P. Corona, A. Lamonaca, L. Portoghesi, and D. Travaglini. 2006. Conversion of clearcut beech coppices into high forests with continuous cover: a case study in central Italy. Forest Ecology and Management 3: 235–240.
- Corona, P., M. Agrimi, F. Baffetta, A. Barbati, M. Chiriaco, L. Fattorini, E. Pompei, and W. Mattioli. 2011. Extending large-scale forest inventories to assess urban forests. Environmental Monitoring and Assessment 184: 1409–1422.
- Corona, P., and A. Barbati. 2010. Orizzonti operativi della pianificazione e gestione forestale a supporto delle politiche sui cambiamenti climatici. Pages 147–161 in G. Sanesi and P. Mairota, editors. Foreste e ciclo del carbonio in Italia: come mitigare il cambiamento climatico. Fondazione Gas Natural, Barcelona, Spain.
- Corona, P., A. Barbati. A. Tomao, R. Bertani. R. Valentini. M. Marchetti., L. Fattorini, and L. Perugini. 2012. Land use inventory as framework for environmental accounting: an application in Italy. iForest 5: 204–209.
- Corona, P., M. V. Chiriacò, R. Salvati, M. Marchetti, B. Lasserre, and B. Ferrari. 2009. Proposta metodologica per l'inventario su vasta scala degli alberi fuori foresta. L'Italia Forestale e Montana 6: 367–380.
- Corona, P., et al. 2015. Integrated forest management to prevent wildfires under Mediterranean environments. Annals of Silvicultural Research 39: 1–22.
- Corona, P., A. Ferrara, and O. La marca. 1997. Sustainable management of forests for atmospheric CO2 depletion: the Italian case. Journal of Sustainable Forestry 3/4: 81–91.
- Corona, P., B. Ferrari, R. Cartisano, and A. Barbati. 2014. Calibration assessment of forest flammability potential in Italy. iForest 7: 300–305.
- Crema, S. 2008. Urban forestry e stima del carbonio: analisi di linee guida e calcolo in zona urbana come applicazione ed opportunità per l'università di Padova. Thesis. Padova University, Padova, Italy.
- De foresta, H., E. Somarriba, A. Temu, D. Boulanger, H. Feuilly, and M. Gauthier. 2013. Towards the assessment of trees outside forests. Resources Assessment Working Paper 183. FAO, Rome, Italy.
- Deckmyn, G., B. Muys, J. Garcia quijano, and R. Ceulemans. 2004. Carbon sequestration following afforestation of agricultural soils: comparing oak/beech forest to short-rotation poplar coppice combining a process and a carbon accounting model. Global Change Biology 10: 1482–1491.
- Dufresne, J., P. Friedlingstein, M. Berthelot, L. Bopp, P. Ciais, L. Fairhead, H. Le treut, and P. Monfray. 2002. On the magnitude of positive feedback between future climate change and the carbon cycle. Geophysical Research Letters 29: 1405.
- FAO. 2001. Global forest resources assessment 2000. Food and Agriculture Organization of the United Nations. Rome, Italy.
- Fares, S., G. Scarascia mugnozza, P. Corona, and M. Palahi. 2015. Five steps for managing Europe's forests. Nature 519: 407–409.
- Finzi, A., et al. 2007. Increases in nitrogen uptake rather than nitrogen-use efficiency support higher rates of temperate forest productivity under elevated CO2. Proceedings of the National Academy of Sciences USA 104: 14014–14019.
- Forest Europe, UNECE, and FAO. 2011. State of Europe's forests 2011. Status and trends in sustainable forest management in Europe. Forest Europe, Oslo, Norway.
- Gasparini, P., F. De natale, L. Di cosmo, C. Gagliano, G. Salvadori, G. Tabacchi, and V. Tosi. 2005. I caratteri quantitativi - Parte 1, versione 2. Inventario Nazionale delle Foreste e dei Serbatoi Forestali di Carbonio (INFC). Ispettorato Generale Corpo Forestale dello Stato, CRA-MPF, Trento, Italy.
- Gaudinski, J. B., S. E. Trumbore, E. A. Davidson, and S. Zheng. 2000. Soil carbon cycling in a temperate forest: radiocarbon-based estimates of residence times, sequestration rates and partitioning of fluxes. Biogeochemistry 51: 33–69.
- Giorgi, F., and P. Lionello. 2008. Climate change projections for the Mediterranean region. Global and Planetary Change 63: 90–104.
- Giulietti, V. 2010. Gli accordi volontari per la compensazione della CO2 indagine conoscitiva per il settore forestale in Italia. Istituto Nazionale Economia Agraria, Roma, Italy.
- Grassi, G., M. G. Den elzen, A. F. Hof, R. Pilli, and S. Federici. 2012. The role of the land use, land use change and forestry sector in achieving Annex I reduction pledges. Climatic Change 115 (3–4): 873–881.
- Grigal, D. F., and W. E. Berguson. 1998. Soil carbon changes associated with short-rotation systems. Biomass and Bioenergy 14: 371–377.
- Hansen, E. A. 1993. Soil carbon sequestration beneath hybrid poplar plantations in the North Central United States. Biomass and Bioenergy 5: 431–436.
- Harfouche, A., M. Richard, and A. Altman. 2011. Tree genetic engineering and applications to sustainable forestry and biomass production. Trends in Biotechnology 29: 9–17.
- Heilman, P., and R. Norby. 1998. Nutrient cycling and fertility management in temperate short rotation forest systems. Biomass and Bioenergy 14: 361–370.
- Heimann, M., and M. Reichstein. 2008. Terrestrial ecosystem carbon dynamics and climate feedbacks. Nature 451: 289–292.
- Hyvönen, R., et al. 2007. The likely impact of elevated [CO2], nitrogen deposition, increased temperature and management on carbon sequestration in temperate and boreal forest ecosystems: a literature review. New Phytologist 173: 463–480.
- IPCC (Intergovernmental Panel on Climate Change). 2003. Good practice guidance for land use, land-use change and forestry. Institute for Global Environmental Strategies, Kanagawa, Japan.
- ISPRA. 2011. Italian Greenhouse Gas Inventory 1990-2009. Institute for Environmental Protection and Research, Rome, Italy.
- Janssens, I. A., and S. Luyssaert. 2009. Nitrogen's carbon bonus. Nature Geoscience 2: 318–319.
- Jo, H., and E. Mcpherson. 1995. Carbon storage and flux in urban residential greenspace. Journal of Environmental Management 45: 109–133.
- Kolström, M., et al. 2011. Reviewing the science and implementation of climate change adaptation measures in European forestry. Forests 2: 961–982.
- Laganiere, J., D. Angers, and D. Pare. 2009. Carbon accumulation in agricultural soils after afforestation: a meta-analysis. Global Change Biology 16: 439–453.
- Le quéré, C., et al. 2009. Trends in the sources and sinks of carbon dioxide. Nature Geoscience 2: 831–836.
- Liberloo, M., et al. 2010. Bio-energy retains its mitigation potential under elevated CO2. PLoS ONE 5: e11648.
- Liberloo, M., M. Lukac, C. Calfapietra, M. R. Hoosbeek, B. Gielen, F. Miglietta, G. E. Scarascia-mugnozza, and R. Ceulemans. 2009. Coppicing shifts CO2 stimulation of poplar productivity to above-ground pools: a synthesis of leaf to stand level results from the POP/EUROFACE experiment. New Phytologist 182: 331–346.
- Lindner, M., et al. 2010. Climate change impacts, adaptive capacity, and vulnerability of European forest ecosystems. Forest Ecology and Management 259: 698–709.
- Luo, Y., L. Wu, J. A. Andrews, L. White, R. Matamala, V. R. Schafer, and W. H. Schlesinger. 2001. Elevated CO2 differentiates ecosystem carbon processes: deconvolution analysis of Duke Forest FACE data. Ecological Monographs 71: 357–376.
- Luyssaert, S., E. D. Schulze, A. Borner, A. Knohl, D. Hessenmoller, B. E. Law, P. Ciais, and J. Grace. 2008. Old-growth forests as global carbon sinks. Nature 455: 213–215.
- Maesano, M., R. Drigo, B. Lasserre, G. Chirici, and M. Marchetti. 2014. Forest biomass for bioenergy: opportunities and constraints for good governance. A case study from Italy. Drewno 57 (192): 63–76.
- Magnani, F., et al. 2007. The human footprint in the carbon cycle of temperate and boreal forests. Nature 447: 849–851.
- Marchetti, M., G. Chierici, and B. Lasserre. 2015. Chapter 8. Carbon losses due to wood harvesting and the role of wood products. Pages 103–115 in R. Valentini, and F. Miglietta, editors. The greenhouse gas balance of Italy. Springer-Verlag, Berlin, Germany.
- Marchetti, M., L. Sallustio, M. Ottaviano, A. Barbati, P. Corona, R. Tognetti, L. Zayatter, and G. Capotorti. 2012. Carbon sequestration by forest in the National Parks of Italy. Plant Biosystems 146: 1001–1011.
- Morani, A., D. Nowak, S. Hirabayashi, G. Guidolotti, M. Medori, V. Muzzini, S. Fares, G. Scarascia mugnozza, and C. Calfapietra. 2014. Comparing i-Tree modeled ozone deposition with field measurements in a periurban Mediterranean forest. Environmental Pollution 195C: 202–209.
- Motta, R. 2008. Il ciclo del carbonio nelle foreste vetuste. iForest 5: 302–305.
- Nolè, A., et al. 2015. Chapter 5. The role of managed forest ecosystem: a modelling based approach. Pages 71–85 in R. Valentini, and F. Miglietta, editors. The greenhouse gas balance of Italy. Springer-Verlag, Berlin, Germany.
- Nowak, D., E. Crane, J. Stevens, R. Hoehn, J. Walton, and J. Bond. 2008. A ground-based method of assessing urban forest structure and ecosystem services. Arboriculture & Urban Forestry 34: 347–358.
- Papale, D. 2006. Il Progetto CARBOITALY: una rete nazionale per la misura dei sink forestali e agricoli italiani e lo sviluppo di un sistema di previsione dell'assorbimento dei gas serra. iForest 3: 165–167.
- Penman, J., et al. 2003. Good practice guidance for land use, land-use change and forestry. IPCC National Greenhouse Gas Inventories Programme and Institute for Global Environmental Strategies, Kanagawa, Japan.
- Piovesan, G., et al. 2010. Structural patterns, growth processes, carbon stocks in an Italian network of old-growth beech forests. L'Italia Forestale e Montana 65: 557–590.
- Post, W. M., and K. C. Kwon. 2000. Soil carbon sequestration and land-use change: processes and potential. Global Change Biology 6: 317–327.
- Romano, D., et al. 2014. Italian greenhouse gas inventory 1990–2012 national inventory report. ISPRA, Rapporti, Italy.
- Scarascia-mugnozza, G., P. De angelis, M. Sabatti, C. Calfapietra, R. Ceulemans, A. Peressotti, and F. Miglietta. 2000. A FACE experiment on short rotation, intensive poplar plantation: objective and experimental set up of POPFACE. Pages in 136–140 M. A. Sutton, J. M. Moreno, W. Van der putten, and S. Struwe, editors. Terrestrial ecosystem research in Europe: successes, challenges and policy. Final Conference of the Terrestrial Ecosystem Research Initiative– Concerted Action (TERICA). Ecosystem Research Report, European Commission, Luxembourg.
- Scharlemann, J. P. W., E. V. J. Tanner, R. Hiederer, and V. Kapos. 2014. Global soil carbon: understanding and managing the largest terrestrial carbon pool. Carbon Management 5: 81–91.
- Schimel, D. S., et al. 2001. Recent patterns and mechanisms of carbon exchange by terrestrial ecosystems. Nature 414: 169–172.
- Schlesinger, W. 1995. Soil respiration and changes in soil carbon stocks. Pages 159–168 in G. Woodwell, and F. T. Mackenzie, editors. Biotic feedbacks in the global climate system. Oxford University Press, Oxford, UK.
- Smith, P., D. Powlson, M. J. Glendining, and J. J. Smith. 1997. Potential for carbon sequestration in European soils: preliminary estimates for five scenarios using results from long-term experiments. Global Change Biology 3: 67–79.
- Spiecker, H. 2002. Tree rings and forest management in Europe. Dendrochronologia 20: 191–202.
- Tabacchi, G., F. De natale, and P. Gasparini. 2010. Coerenza ed entità delle statistiche forestali. Stime degli assorbimenti netti di carbonio. Sherwood 165: 11–19.
- Trenberth, K., A. Dai, R. M. Rasmussen, and D. B. Parsons. 2003. The changing character of precipitation. Bulletin of the American Meteorological Society 84: 1205–1217.
- UNFCCC. 2001. Land use, land-use change and forestry. Decision 16/CMP.1. United Nations Framework Convention on Climate Change, Bonn, Germany.
- UNFCCC. 2011. Working Group on further commitments for Annex I Parties under the Kyoto Protocol. United Nations Framework Convention on Climate Change, Bonn, Germany.
- Van der werf, G. R., D. C. Morton, R. S. Defries, J. G. Olivier, P. S. Kasibhatla, R. B. Jackson, G. Collatz, and J. T. Randerson. 2009. CO2 emissions from forest loss. Nature Geoscience 2: 737–738.
- Vetter, M., C. Wirth, H. Böttcher, G. Churkina, E. D. Schulze, T. Wutzler, and G. Weber. 2005. Partitioning direct and indirect human-induced effects on carbon sequestration of managed coniferous forests using model simulations and forest inventories. Global Change Biology 11: 810–827.
- Whitford, V., A. Ennos, and J. Handley. 2001. City form and natural process—indicators for the ecological performance on urban areas and their application to Merseyside, UK. Landscape and Urban Planning 57: 91–103.
- Zhou, G., S. Liu, Z. Li, D. Zhang, X. Tang, C. Zhou, J. Yan, and J. Mo. 2006. Old-growth forests can accumulate carbon in soils. Science 314: 1417.