Big Things in Narrow Channels
Microfluidics, the movement of liquids through channels in the 10–100 µm range, encompasses “essentially two big things,” according to Bart Romanowicz, Executive Director of Microtechnology, Nano Science and Technology Institute (NSTI), Cambridge, MA. One is the ability to take advantage of “very cool effects” of fluid motion through these narrow channels, allowing unique experiments that can't be done on a “regular” scale. The second is that many reactions can be run simultaneously in a very repeatable manner, including separation, amplification, labeling, and detection; these are being applied to the analysis of DNA, RNA, proteins, and intact cells. Another advantage, notes Romanowicz, is that only small volumes are needed, permitting analysis of limited samples and the use of only tiny quantities of reagents.
Many microfluidic-based experiments are performed in tiny cartridges or chips, often inexpensive and disposable, shrinking a bench top of experiments into a so-called lab-on-a-chip, in which reagents and samples are mixed and driven through the system from reaction to detection sites, perhaps with wash or separation steps in between. Detection methods include optical detection of the binding of fluorescent markers or electronic detection of molecular binding to electrodes. Use of lab-on-a-chip technology therefore requires the chips per se as well as design services to customize them and manufacturing services to fabricate them, such as: (i) some means of effecting fluid flow, such as electrokinetics or pneumatics; (ii) often separation steps (e.g., electrophoresis or binding to beads or other surfaces); (iii) a way to detect, quantify, and collect the reaction or final product; and (iv) software to control the processes and analyze the results. There are companies that specialize in each of the many aspects of microfluidics (e.g., producing surface treatments for the channels that affect their hydrophobicity). Hydrophilic areas allow the flow of water-based fluids, while hydrophobic areas stop the flow and function as valves.
Dr. Klaus Stefan Drese, IMM, Mainz, Germany
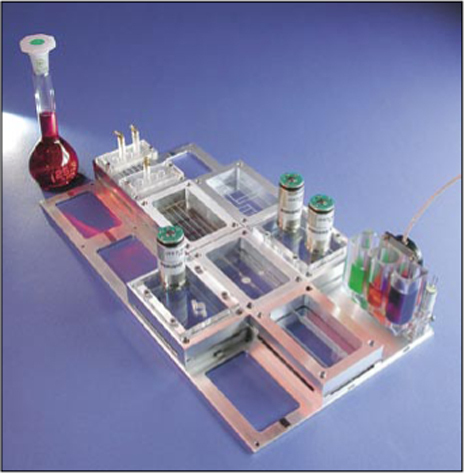
Microfluidic systems are manufactured by several methods. One, derived from more industrial applications, uses photolithography to form a pattern on a silicon, glass, or plastic wafer, which is then etched to create channels and reservoirs. Microfluidic systems can also be fabricated from glass or plastics using molds. Although custom-designed chips can be prohibitively expensive for many researchers, small, more generic, affordable chips are commercially available. At Purdue University, West Lafayette, IN, Michael Ladisch and his graduate student Tom Huang have developed a method of assembling microfluidic chips in a laboratory setting without reliance on current industrial processes. Channels are created by draping a piece of polydimethylsiloxane polymer over fine fibers on a glass slide. They anticipate that this method will allow researchers to experiment with the technology and adapt it to their own needs.
Nucleic acid applications of microfluidics include the preparation, detection, and automation of PCR. Microfluidics handily lends itself to analysis, sorting, counting, and imaging of intact cells and particles and to the separation and detection of proteins. The uses of lab-on-a-chip technology are limited only by the imagination, although in practice, the discipline has limits to overcome before it achieves its full potential.
Nucleic Acid Analysis
Microfluidics systems for isolating nucleic acids and amplifying DNA by PCR allow the analysis of trace samples from a variety of sources. In addition to commercially available systems, several laboratories are working on creating fully automated systems that will integrate all steps from the initial crude sample preparation through the analysis of results. These systems may reach commercialization in the next few years. Stephen Quake's group at the California Institute of Technology, Pasadena, CA, is developing integrated nanoliter systems capable of isolating nucleic acids from small numbers of bacterial and mammalian cells. This system seems amenable to scale up for parallel processing of multiple samples simultaneously.
Other laboratories have concentrated their efforts on microfluidic devices for PCR. Two such systems rely on rapid and accurate movement of the PCR reactants from one controlled temperature zone to another. Steffen Hardt, now at Darmstadt University of Technology, Darmstadt, Germany, and Klaus Drese from the Institute of Microtechnology Mainz (IMM), Mainz, Germany, have developed a slug-flow PCR system with their team at IMM. The IMM team is developing a sample preparation unit to complement the PCR chip. Another chip-based microfluidic PCR system, which also moves samples among different temperature zones, is being developed by P.-A. Auroux, Philip Day, and A. Manz, at Imperial College, London and University of Manchester, UK. These systems appear to offer more flexible programming options and greater speeds of reactions when compared with conventional thermal cyclers.
Cell Sorting
Fluorescence-activated cell sorting (FACS) is a standard method of separating cells and other particles. FACS, which by definition relies on the natural fluorescence of or labeling on the cells or particles of choice, is a target of downsizing to microfluidic proportions. Several groups are developing microFACS, including Quake's, and that of Hyunwoo Bang and Jun Keun Chang at Seoul National University, Seoul, Korea. Sorting in the former group's device relies on electroosmotic flow, and in the latter group's, like conventional flow cell sorters, sorting relies on hydrodynamic flow manipulation.
An alternative to microFACS is being developed in the laboratories of Michael MacDonald and Kishan Dholakia at the University of St. Andrews, Fife, UK, and Gabe Spalding, Illinois Wesleyan University, Bloomington, IL. Their technology uses an optical lattice formed by passing a single 1.07-µm laser beam through a diffractive beam splitter, thereby creating four additional beams that are combined with the original beam to make an interference pattern. A sample containing particles of varying sizes and/or properties can be passed through a projection of this pattern into a fractionation chamber. The particle size of choice is collected in an outflow stream, while the other particles in the sample are deflected away. The advantages of this system include the ability to sort particles ranging in size from macromolecules to cells, to process samples continuously, to reconfigure the sorting parameters in real time, as well as its low cost and lack of reliance on fluorescence. “We expect to have a portable system for our bio and medical collaborators within the year and a prototype of a bench top sorter working within 2 years,” MacDonald predicts. Their focus will probably be on enriching populations of cells rather than creating absolutely pure separations. “We are currently interested in volumes up to about 5 µL as an upper limit,” MacDonald said. “That should be able to give a statistically significant set of data for rare cell types, in blood, for example.”
Protein Analysis and Sample Preparation
“Protein analysis is the area where I see the biggest impact of microfluidics,” says Srinivas Iyer of the Los Alamos National Laboratory, Los Alamos, NM, thanks to major advances in separation and detection technology. Although micro liquid chromatography (LC) and capillary electrophoresis (CE) methods are improving, and chips exploiting the latter technology are commercially available, more efficient separation at a detectable level remains a goal. Optical detection is well-established, notes Iyer; the challenge is to develop a “user-friendly interface with the various mass spectrophotometers out there.” This requires the development of software that will enable joint control of the separations and mass spectrometry (MS) operations.
Ruedi Aebersold, University of Basel, Basel, Switzerland, thinks that at least portions of his group's method of rapid identification and quantification of cellular proteins for the development of “protein profiles” will be amenable to the lab-on-a-chip format. Their method uses isotope-coded affinity tags (ICAT) and MS to obtain a picture of proteins expressed in cells under varying conditions. “Certainly, analyzing ICAT-labeled peptides would be very straightforward, as long as the chip would be connected to a mass spectrometer. Doing labeling reaction on the chip as well would be a little more complex, but certainly not impossible.” This would require moving both the labeling reagent and the sample to a reaction area, and then removing the excess label before further processing. “I think these steps would be performed easier off-line,” Aebersold concludes.
The necessity for sample preparation outside the current microfluidics systems is a barrier to full automation that, at least for some if not most applications, will eventually be overcome. Romanowicz believes that since DNA chemistry is so well understood, detection is approaching single molecule sensitivity, and since DNA is relatively insensitive to other cellular contaminants, it is a likely target of a fully automated system with the ability to collect and process crude samples. Iyer would consider it a major advance if protein separation, digestion, and purification followed by direct MS analysis could be fully automated. Although he could envision a robotic system performing some of the tasks, at this time the robots capable of reproducibly spotting microfluidic-sized volumes are probably too expensive and large, annihilating the microfluidic advantage. The development of an efficient continuous capillary spotting method could solve this problem. Aebersold, on the other hand, feels that in the proteomics field, it is not possible to avoid a minimal number of preparatory steps before automated analysis.
Future Prospects
The reliance on very small sample sizes, the potential to detect a single infectious microorganism, and the feasibility of automation and remote operation of microfluidics-based systems make them attractive candidates for bedside diagnosis, environmental monitoring, biodefense applications, and drug discovery. In addition to the need for increased integration and automation of sample preparation and handling, as well as analysis of results into self-contained systems, there is clearly a need for standardization. Agilent Technologies, Santa Clara, CA, has recently entered that arena. In June they announced the availability of software for their bioanalyzer to measure RNA quality in their microfluidic chip system on a scale of 1 (totally degraded) to 10 (intact and pure).