Abstract
Purpose
The compound traditional Chinese medicine Xihuang pill (XHP) has been adopted to treat breast cancer (BC) for centuries, but its specific mechanism of action is unclear.
Materials and Methods
The active ingredients and related targets of XHP were screened using the TCMSP and TCMID databases. GSE139038 was downloaded from the GEO database, and differentially expressed genes (DEGs) were analyzed. The intersection of targets and DEGs were chosen to build an ingredients–target genes network. Protein–protein interaction network construction and functional enrichment analysis of target genes were conducted.
Results
A PPI network of 37 targets was constructed, and seven core nodes (FOS, MYC, JUN, PPARG, MMP9, PTGS2, SERPINE1) were identified. Functional enrichment analysis revealed that the aforementioned targets were mainly enriched in the IL-17, toll-like receptor, and tumor necrosis factor signaling pathways, which are deeply involved in the inflammatory microenvironment of tumors.
Conclusion
This network pharmacology study indicated that XHP can inhibit the development of BC by targeting a variety of proteins and signaling pathways involved in the inflammatory microenvironment.
Introduction
Breast cancer (BC) is the most commonly diagnosed cancer among women worldwide.Citation1 Thanks to early detection, the mortality rate of BC has decreased even though its incidence has actually increased.Citation2 It is estimated that more than 90% of cancer-related deaths are caused by metastasis.Citation3–Citation5 Metastasis involves both cancer cells and other cells (eg, stromal cells, immune cells) in the tumor microenvironment. These cells secrete various cytokines and/or growth factors, which in turn affect the progression of BC through various mechanisms.Citation6 Inflammation can influence the progression, metastasis, and outcome of BC by establishing favorable immune microenvironments.Citation7,Citation8 Therefore, targeting inflammatory pathways could be helpful in the development of novel prevention and therapeutic strategies.
Xihuang pill (XHP) is a well-known compound traditional Chinese medicine (TCM) with anti-cancer activity. It has been used to treat BC, furunculosis, and scrofula and to relieve swelling and pain.Citation9 A meta-analysis revealed that XHP combined with chemotherapy could significantly enhance the tumor response and alleviate toxicity induced by chemotherapy in patients with BC.Citation10 Studies illustrated that XHP can inhibit growth and induce apoptosis in BC cells (MCF-7, MDA-MB-231).Citation9 XHP induced Bcl-2–independent apoptosis and S phase arrest in Hs578T cells.Citation11 XHP induced apoptosis in estrogen receptor-negative BC cells, possibly by upregulating the mRNA expression of TP53, which induced the expression of the apoptotic protein Bax.Citation12 XHP contains Niuhuang (Calculus Bovis), Shexiang (Moschus), Ruxiang (Olibanum), and Moyao (Myrrh). Modern pharmacological studies illustrated that Ruxiang and Moyao have strong anti-inflammatory effects.Citation13 Network pharmacology analysis indicated that XHP might target the IL-17, Toll-like receptors (TLRs), and tumor necrosis factor (TNF) signaling pathways. These signaling pathways are closely related to immunity and inflammation. Exploration of the molecular mechanism of XHP is of great significance for promoting its clinical application.
Materials and Methods
Identification of the Differentially Expressed Genes (DEGs) of BC
The gene expression dataset GSE139038 was downloaded from the GEO database (https://www.ncbi.nlm.nih.gov/geo/ ). GSE139038 was based on the GPL27630 Print_1437 platform. GSE139038 contains 65 samples including 41 breast tumors (24 early stage, 17 locally advanced), 18 adjacent normal tissues (paired normal), and 6 apparently normal tissues from breasts in patients who underwent surgery for non-malignant conditions. R packages (“limma” and “pheatmap”) were used to analyze GSE139038. DEGs between normal tissue and breast tumors were detected on the basis of the criteria adjusted P-value < 0.05 and |logFC| > 1.
Screening the Chemical Ingredients and Related Targets of XHP
The ingredients of XHP and related targets were screened using the TCMSP (http://tcmspw.com/index.php ) and TCMID databases (http://119.3.41.228:8000/tcmid/ ). Ingredients meeting the criteria oral bioactivity (OB) ≥30% and drug-likeness (DL) ≥0.18 were selected to identify related targets.
Ingredients–Targets Network Construction
The common genes between ingredients-related targets and the DEGs of BC were collected and sorted. The ingredients–targets network was visualized using Cytoscape software (www.cytoscape.org/ ).Citation14
Protein–Protein Interaction (PPI) Network Construction and Analysis
The STRING database (http://string-db.org/ ) is designed to analyze PPI information. To evaluate potential PPIs, DEGs were uploaded to the STRING database. The PPI pairs were extracted with a combined score >0.4. Subsequently, the PPI network was visualized using Cytoscape software. Nodes with a higher degree of connectivity tend to be more essential in maintaining the stability of the entire network. CytoHubba, an app plugin in Cytoscape, was used to calculate the degree of each protein node.Citation15 In our study, degree, betweenness, and closeness were used to determine the degree of connectivity. The intersections of the top 10 genes in each method were regarded as core proteins.
Functional Enrichment Analysis
R packages (“clusterProfiler,” “org.Hs.eg.db,” “enrichplot,” and “ggplot2”) were used to conduct biological process (BP), cellular component (CC), molecular function (MF), and Kyoto Encyclopedia of Genes and Genomes (KEGG) analyses (p-value cutoff = 0.05, q-value cutoff = 0.05).
Results
Identification of the DEGs of BC
The GSE139038 samples were separated into normal and tumor group (24 and 41 samples, respectively). In total, 1464 DEGs were identified, including 990 downregulated and 474 upregulated genes, as presented in the volcano map () and heatmap ().
Screening of the Active Ingredients and Targets of XHP
In total, 46 ingredients and 209 targets (Table S1 ) of XHP were identified using the TCMSP and TCMID databases. Targets and DEGs were integrated to form an ingredients–targets network using Cytoscape software. The integrated network contained 37 molecules and 37 target proteins ().
PPI Network Construction
The aforementioned 37 targets were mapped to the STRING database to construct the PPI network (). The PPI pairs were extracted with a combined score >0.4, and disconnected nodes in the network were deleted. The network contained 36 nodes and 151 edges, and the PPI enrichment p-value was <1.0e−16. The CytoHubba plugin was used to located hub nodes according to degree, betweenness, and closeness (–). The intersections of the top 10 nodes in each method were identified as core targets (FOS, MYC, JUN, PPARG, MMP9, PTGS2, SERPINE1). The PPI network () of core targets has significantly more interactions than expected (enrichment p-value = 1.13e−07).
Table 1 Top 10 Genes in the Network Ranked by Degree
Table 2 Top 10 Genes in the Network Ranked by Betweenness
Table 3 Top 10 Genes in the Network Ranked by Closeness
Functional Enrichment Analysis
R packages were adopted to conduct functional enrichment analysis of the 37 targets. BP analysis indicated that targets were significantly enriched in response to lipopolysaccharide (GO: 0032496), response to molecule of bacterial origin (GO: 0002237), and response to purine-containing compound pathways (GO: 0014074; ). CC analysis revealed that the targets were mainly enriched in RNA polymerase II transcription factor complex (GO: 0090575), nuclear transcription factor complex (GO: 0044798), and apical plasma membrane pathways (GO: 0016324; ). MF analysis indicated that targets were significantly enriched in transcription factor activity, RNA polymerase II proximal promoter sequence-specific DNA binding (GO: 0000982), chemokine receptor binding (GO: 0042379), and activating transcription factor binding pathways (GO: 0033613; ). To further reveal the potential mechanism of action of XHP in the treatment of BC, KEGG pathway enrichment analysis was conducted. The IL-17 signaling pathway (hsa04657), TLR signaling pathway (hsa04620), TNF signaling pathway (hsa04668), and BC (hsa05224) were screened using q-value <0.05 (). The interaction network between the KEGG pathways and targets is presented in .
Figure 6 Biological process analysis of the target genes. The top 20 biological processes enriched in Gene Ontology analysis are presented. The bubble size indicates the gene count, and the color indicates the adjusted p-value.

Figure 7 Cellular component analysis of the target genes. The top 20 cellular components enriched in Gene Ontology analysis are presented. The bubble size indicates the gene count, and the color indicates the adjusted p-value.
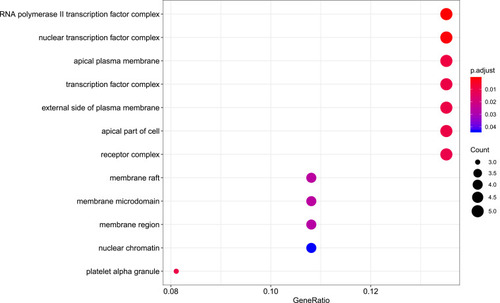
Figure 8 Molecular function analysis of the target genes. The top 20 molecular functions enriched in Gene Ontology analysis are presented. The bubble size indicates the gene count, and the color indicates the adjusted p-value.

Discussion
Compound TCMs have the characteristics of “multi-component, multi-pathway and multi-target” synergistic effects, and they have unique advantages in the treatment of a series of complex diseases such as cancer, diabetes, and stroke.Citation16–Citation18 Network pharmacology has integral and systematic characteristics, and thus, it can effectively explore the basis of medicinal substance and mechanism of action, which has great significance for improving the therapeutic application of compound Chinese medicines.
In recent years, the inhibitory effects of TCM on tumor recurrence and metastasis have attracted increasing attention.Citation19,Citation20 Relevant studies discovered that TCMs can improve the quality of life of patients with cancer, reduce the risks of tumor recurrence and metastasis, and prolong survival.Citation21–Citation23 Although a few reports revealed that XHP can inhibit proliferation and induce apoptosis in BC cells,Citation9,Citation11,Citation12 the mechanism of action is unclear.
We screened 37 chemical molecules in XHP and 37 target proteins. Some molecules targeted multiple proteins, among which seven proteins (FOS, MYC, JUN, PPARG, MMP9, PTGS2, SERPINE1) were identified as core nodes. FOS forms a tight complex (non-covalently) with the transcription factor JUN/AP-1, which plays important roles in the development of BC.Citation24,Citation25 A recent study also found XHP could regulate the MEKK1/SEK1/JNK1/AP-1 pathway, which confirmed the possible interaction between XHP and the FOS/JUN/AP-1 complex.Citation26 Chen et al discovered that the overexpression of MMP9 stimulated the invasiveness of BC cell-formed spheroids in vitro and DCIS in vivo, whereas depletion of MMP9 inhibited their invasiveness.Citation27 Upregulation of PTGS2 is also associated with increased cell adhesion, phenotypic changes, anti-apoptosis, and tumor angiogenesis.Citation28 In cancer cells, PTGS2 plays key roles in the production of prostaglandin E2 (PGE2) and development of tumors.Citation29 A study by Bos et al indicated that COX2 (also known as PTGS2) and other genes mediate BC metastasis to the brain.Citation30 Nandi et al found that PGE2 promotes lymphangiogenesis associated with BC by activating EP4 receptors on lymphatic endothelial cells.Citation31 Kochel et al discovered that multidrug resistance-related protein can export PGE2 and promote metastasis in basal/triple-negative BC.Citation32 SERPINE1 (also known as plasmin activator inhibitor-1) has been demonstrated to be highly expressed in various types of tumor biopsies.Citation33 More importantly, SERPINE1 and urokinase-type plasminogen activator have been identified as prognostic factors for disease progression and relapse in BC.Citation33,Citation34
Tumor cells have the ability to produce cytokines and chemokines by activating transcription factors such as nuclear factor-κB (NF-κB) and interferon regulatory factors (IRFs).Citation35 These cytokines and chemokines induce the mobilization and reprogramming of immune cells and activate stromal cells in the extracellular matrix adjacent to the tumor. These cells in turn produce more cytokines and chemokines, thereby amplifying the entire process. Cytokines and/or growth factors secreted by these immune or stromal cells further regulate the activity of recruited immune cells, leading to the sustenance of a tumor-friendly inflammatory microenvironment.Citation7,Citation36 Therefore, cytokine and chemokine pathways and the inflammatory tumor microenvironment could be therapeutic targets with great potential. Su et al found that XHP might promote Treg cell apoptosis in the tumor microenvironment and further inhibit the tumor growth of 4T1 mouse breast cancer cells.Citation26 Functional enrichment analyses indicated that target proteins were significantly enriched in the IL-17, TLR, and TNF signaling pathways. These pathways are well known to play significant roles in inflammation and tumor development.Citation35,Citation37–Citation43 It has been reported that IL-17 activates the Src/PI3K/Akt/NF-κB, MAPK, Stat3, and COX-2 pathways, which play significant roles in tumorigenesis, angiogenesis, and metastasis.Citation44,Citation45 IL-17 is overexpressed in the intratumoral stromal cells of triple-negative BC. Upon overexpression, IL-17 can activate tumor microangiogenesis through its signal transduction pathways, resulting in increased tumor secretion of VEGFA, thereby promoting tumor progression.Citation46 TLRs trigger multiple signaling pathways involving nuclear factor B, IRFs, and MAPKs to produce various cytokines with important roles in diseases such as cancer.Citation47 Inhibition of TLR signaling can suppress human BC cell viability, invasion, and migration.Citation48,Citation49 TNF-α is involved in all stages of BC progression, participating in cell proliferation, survival, and motility; inflammation maintenance; acquisition of stemness; and resistance to chemotherapy.Citation43,Citation50 TNF-α levels at the tumor site or in plasma/serum in patients with BC are correlated with the clinical status and outcome.Citation51
Conclusion
This network pharmacology study indicated that XHP can inhibit the development of BC by targeting a variety of proteins and signaling pathways involved in the inflammatory microenvironment. Additional research is needed to clarify the effects of XHP on different molecular subtypes of BC.
Acknowledgment
We thank Joe Barber Jr. PhD, from Liwen Bianji, Edanz Editing China (www.liwenbianji.cn/ac ), for editing the English text of a draft of this manuscript.
Disclosure
All authors declare no conflicts of interest.
References
- BrayF, FerlayJ, SoerjomataramI, SiegelRL, TorreLA, JemalA. Global cancer statistics 2018: GLOBOCAN estimates of incidence and mortality worldwide for 36 cancers in 185 countries. CA Cancer J Clin. 2018;68(6):394–424. doi:10.3322/caac.2149230207593
- NarodSA, IqbalJ, MillerAB. Why have breast cancer mortality rates declined?J Cancer Policy. 2015;5:8–17. doi:10.1016/j.jcpo.2015.03.002
- MehlenP, PuisieuxA. Metastasis: a question of life or death. Nat Rev Cancer. 2006;6(6):449–458.16723991
- MonteiroJ, FoddeR. Cancer stemness and metastasis: therapeutic consequences and perspectives. Eur J Cancer. 2010;46(7):1198–1203. doi:10.1016/j.ejca.2010.02.03020303259
- GuanX. Cancer metastases: challenges and opportunities. Acta Pharm Sin B. 2015;5(5):402–418.26579471
- DeshmukhSK, SrivastavaSK, TyagiN, et al. Emerging evidence for the role of differential tumor microenvironment in breast cancer racial disparity: a closer look at the surroundings. Carcinogenesis. 2017;38(8):757–765. doi:10.1093/carcin/bgx03728430867
- DeshmukhSK, SrivastavaSK, PoosarlaT, et al. Inflammation, immunosuppressive microenvironment and breast cancer: opportunities for cancer prevention and therapy. Ann Transl Med. 2019;7(20):593. doi:10.21037/atm.2019.09.6831807574
- ZahidH, SimpsonER, BrownKA. Inflammation, dysregulated metabolism and aromatase in obesity and breast cancer. Curr Opin Pharmacol. 2016;31:90–96. doi:10.1016/j.coph.2016.11.00327875786
- PanG, WangW, WangL, et al. Anti-breast cancer effects and mechanisms of Xihuang pill on human breast cancer cell lines. J Tradit Chin Med. 2013;33(6):770–778. doi:10.1016/S0254-6272(14)60011-X24660610
- MaoD, FengL, HuangS, ZhangS, PengW, ZhangS. Meta-analysis of Xihuang pill efficacy when combined with chemotherapy for treatment of breast cancer. Evid Based Complement Alternat Med. 2019;2019:3502460. doi:10.1155/2019/350246030992708
- ZhengW, HanS, JiangS, et al. Multiple effects of Xihuang pill aqueous extract on the Hs578T triple-negative breast cancer cell line. Biomed Rep. 2016;5(5):559–566. doi:10.3892/br.2016.76927882217
- HeLJ, LiJS, ChenX, et al. [Effect of serum containing Xihuang pill on proliferation of human breast cancer cell line MDA-MB-435 and MCF-7 cells]. Zhongguo Zhong Yao Za Zhi. 2018;43(13):2784–2788. Chinese.30111032
- GuoQ, LinJ, LiuR, et al. Review on the applications and molecular mechanisms of Xihuang pill in tumor treatment. Evid Based Complement Alternat Med. 2015;2015:854307. doi:10.1155/2015/85430726170886
- ShannonP, MarkielA, OzierO, et al. Cytoscape: a software environment for integrated models of biomolecular interaction networks. Genome Res. 2003;13(11):2498–2504. doi:10.1101/gr.123930314597658
- ChinCH, ChenSH, WuHH, HoCW, KoMT, LinCY. CytoHubba: identifying hub objects and sub-networks from complex interactome. BMC Syst Biol. 2014;8(Suppl 4):S11. doi:10.1186/1752-0509-8-S4-S1125521941
- LuoH, VongCT, ChenH, et al. Naturally occurring anti-cancer compounds: shining from Chinese herbal medicine. Chin Med. 2019;14:48.31719837
- XiaoE, LuoL. Alternative therapies for diabetes: a comparison of western and Traditional Chinese Medicine (TCM) approaches. Curr Diabetes Rev. 2018;14(6):487–496. doi:10.2174/157339981366617051910323028523995
- LiuT, DingY, WenA. Traditional Chinese medicine for ischaemic stroke. Lancet Neurol. 2018;17(9):745. doi:10.1016/S1474-4422(18)30290-4
- TangK-Y, DuS-L, WangQ-L, ZhangY-F, SongH-Y. Traditional Chinese medicine targeting cancer stem cells as an alternative treatment for hepatocellular carcinoma. J Integr Med. 2020;18:196–202. doi:10.1016/j.joim.2020.02.00232067923
- YanZ, LaiZ, LinJ. Anticancer properties of traditional Chinese medicine. Comb Chem High Throughput Screen. 2017;20(5):423–429. doi:10.2174/138620732066617011614181828093974
- QiFH, ZhaoL, ZhouAY, et al. The advantages of using traditional Chinese medicine as an adjunctive therapy in the whole course of cancer treatment instead of only terminal stage of cancer. Biosci Trends. 2015;9(1):16–34. doi:10.5582/bst.2015.0101925787906
- TaoWW, JiangH, TaoXM, JiangP, ShaLY, SunXC. Effects of Acupuncture, Tuina, Tai Chi, Qigong, and traditional Chinese medicine five-element music therapy on symptom management and quality of life for cancer patients: a meta-analysis. J Pain Symptom Manage. 2016;51(4):728–747. doi:10.1016/j.jpainsymman.2015.11.02726880252
- LiaoYH, LiCI, LinCC, LinJG, ChiangJH, LiTC. Traditional Chinese medicine as adjunctive therapy improves the long-term survival of lung cancer patients. J Cancer Res Clin Oncol. 2017;143(12):2425–2435. doi:10.1007/s00432-017-2491-628803328
- MinDY, JungE, KimJ, LeeYH, ShinSY. Leptin stimulates IGF-1 transcription by activating AP-1 in human breast cancer cells. BMB Rep. 2019;52(6):385–390. doi:10.5483/BMBRep.2019.52.6.18930293548
- XuJ, ChenY, OlopadeOI. MYC and breast cancer. Genes Cancer. 2010;1(6):629–640. doi:10.1177/194760191037869121779462
- SuL, JiangY, XuY, et al. Xihuang pill promotes apoptosis of Treg cells in the tumor microenvironment in 4T1 mouse breast cancer by upregulating MEKK1/SEK1/JNK1/AP-1 pathway. Biomed Pharmacother. 2018;102:1111–1119. doi:10.1016/j.biopha.2018.03.06329710529
- ChenG, DingXF, PressleyK, et al. Everolimus inhibits the progression of ductal carcinoma in situ to invasive breast cancer via downregulation of MMP9 expression. Clin Cancer Res. 2019.
- RodriguesS, BruyneelE, RodrigueCM, ShahinE, GespachC. [Cyclooxygenase 2 and carcinogenesis]. Bull Cancer. 2004;91(Suppl 2):S61–76. French.
- TongD, LiuQ, WangLA, et al. The roles of the COX2/PGE2/EP axis in therapeutic resistance. Cancer Metastasis Rev. 2018;37(2–3):355–368. doi:10.1007/s10555-018-9752-y30094570
- BosPD, ZhangXHF, NadalC, et al. Genes that mediate breast cancer metastasis to the brain. Nature. 2009;459(7249):1005–1009. doi:10.1038/nature0802119421193
- NandiP, GirishGV, MajumderM, XinX, Tutunea-FatanE, LalaPK. PGE2 promotes breast cancer-associated lymphangiogenesis by activation of EP4 receptor on lymphatic endothelial cells. BMC Cancer. 2017;17(1):11. doi:10.1186/s12885-016-3018-228056899
- KochelTJ, ReaderJC, MaX, KunduN, FultonAM. Multiple drug resistance-associated protein (MRP4) exports prostaglandin E2 (PGE2) and contributes to metastasis in basal/triple negative breast cancer. Oncotarget. 2017;8(4):6540–6554. doi:10.18632/oncotarget.1414528029661
- LiS, WeiX, HeJ, TianX, YuanS, SunL. Plasminogen activator inhibitor-1 in cancer research. Biomed Pharmacother. 2018;105:83–94. doi:10.1016/j.biopha.2018.05.11929852393
- DuffyMJ, McGowanPM, HarbeckN, ThomssenC, SchmittM. uPA and PAI-1 as biomarkers in breast cancer: validated for clinical use in level-of-evidence-1 studies. Breast Cancer Res. 2014;16(4):428. doi:10.1186/s13058-014-0428-425677449
- BhateliaK, SinghK, SinghR. TLRs: linking inflammation and breast cancer. Cell Signal. 2014;26(11):2350–2357. doi:10.1016/j.cellsig.2014.07.03525093807
- ChowMT, LusterAD. Chemokines in cancer. Cancer Immunol Res. 2014;2(12):1125–1131. doi:10.1158/2326-6066.CIR-14-016025480554
- ChangSH. T helper 17 (Th17) cells and interleukin-17 (IL-17) in cancer. Arch Pharm Res. 2019;42(7):549–559. doi:10.1007/s12272-019-01146-930941641
- SongY, YangJM. Role of interleukin (IL)-17 and T-helper (Th)17 cells in cancer. Biochem Biophys Res Commun. 2017;493(1):1–8. doi:10.1016/j.bbrc.2017.08.10928859982
- LimKH, StaudtLM. Toll-like receptor signaling. Cold Spring Harb Perspect Biol. 2013;5(1):a011247. doi:10.1101/cshperspect.a01124723284045
- EchizenK, HiroseO, MaedaY, OshimaM. Inflammation in gastric cancer: interplay of the COX-2/prostaglandin E2 and Toll-like receptor/MyD88 pathways. Cancer Sci. 2016;107(4):391–397. doi:10.1111/cas.1290127079437
- JavaidN, ChoiS. Toll-like receptors from the perspective of cancer treatment. Cancers (Basel). 2020;12(2):297. doi:10.3390/cancers12020297
- van HorssenR, Ten HagenTL, EggermontAM. TNF-alpha in cancer treatment: molecular insights, antitumor effects, and clinical utility. Oncologist. 2006;11(4):397–408.16614236
- CruceriuD, BaldasiciO, BalacescuO, Berindan-NeagoeI. The dual role of tumor necrosis factor-alpha (TNF-alpha) in breast cancer: molecular insights and therapeutic approaches. Cell Oncol (Dordr). 2020;43(1):1–18. doi:10.1007/s13402-019-00489-131900901
- PleC, FanY, Ait YahiaS, et al. Polycyclic aromatic hydrocarbons reciprocally regulate IL-22 and IL-17 cytokines in peripheral blood mononuclear cells from both healthy and asthmatic subjects. PLoS One. 2015;10(4):e0122372. doi:10.1371/journal.pone.012237225860963
- RyuH, ChungY. Regulation of IL-17 in atherosclerosis and related autoimmunity. Cytokine. 2015;74(2):219–227. doi:10.1016/j.cyto.2015.03.00925890878
- QianXL, XuP, ZhangYQ, et al. Increased number of intratumoral IL-17+ cells, a harbinger of the adverse prognosis of triple-negative breast cancer. Breast Cancer Res Treat. 2020;180:311–319. doi:10.1007/s10549-020-05540-631993861
- MogensenTH. Pathogen recognition and inflammatory signaling in innate immune defenses. Clin Microbiol Rev. 2009;22(2):240–273, Table of Contents.19366914
- SchwartzAL, DickersonE, DagiaN, MalgorR, McCallKD. TLR signaling inhibitor, phenylmethimazole, in combination with tamoxifen inhibits human breast cancer cell viability and migration. Oncotarget. 2017;8(69):113295–113302. doi:10.18632/oncotarget.1035829371911
- XuM, LiD, YangC, JiJS. MicroRNA-34a inhibition of the TLR signaling pathway via CXCL10 suppresses breast cancer cell invasion and migration. Cell Physiol Biochem. 2018;46(3):1286–1304. doi:10.1159/00048911129689563
- ZhangZ, LinG, YanY, et al. Transmembrane TNF-alpha promotes chemoresistance in breast cancer cells. Oncogene. 2018;37(25):3456–3470. doi:10.1038/s41388-018-0221-429559745
- MaY, RenY, DaiZJ, WuCJ, JiYH, XuJ. IL-6, IL-8 and TNF-alpha levels correlate with disease stage in breast cancer patients. Adv Clin Exp Med. 2017;26(3):421–426. doi:10.17219/acem/6212028791816