Abstract
In recent years, more and more researches on cell death mode in breast cancer, including apoptosis, ferroptosis, etc. Ferroptosisis a regulated form of cell death characterized by iron-dependent accumulation of lipid peroxidation to lethal levels, and numerous studies have shown that ferroptosis is closely associated with tumor cells. Breast cancer is one of the malignant tumors with the highest incidence in women, and TNBC accounts for about 15–20% of all types of breast cancer. Due to the poor prognosis, strong aggressiveness, high drug resistance and lack of molecular targeting characteristics of TNBC, the treatment of TNBC faces many difficulties and great challenges. A large number of studies have shown that ferroptosis plays an important role in the occurrence and development of TNBC, tumor diagnosis, treatment and prognosis, among which the main mechanisms inducing ferroptosis include oxidative stress pathway, iron metabolism pathway and lipid metabolism pathway. Since TNBC is highly sensitive to oxidative stress pathways, intracellular GSH reduces reactive oxygen species under the action of GSH peroxidase (GPX), and when intracellular lipid peroxidase (LPO) accumulates to a certain level, ferroptosis will be induced, thus achieving the purpose of killing TNBC cells. In addition, lipid metabolism is highly consistent with the high lipid level of TNBC tumor cells. As a new therapeutic method, nanotechnology has added security to the treatment of cancer with its high safety and excellent biocompatibility. Therefore, the combination of nanotechnology with iron-based radiotherapy, chemotherapy, targeting and immunization has great research value for the treatment of TNBC In addition, the novel idea of treating TNBC with ethnopharmacology combined with ferroptosis is also involved. This article reviews the mechanism of ferroptosis and the recent research on the treatment prospects of TNBC based on ferroptosis and nanotechnology, hoping to provide references for the treatment of diseases based on ferroptosis.
Background Introduction
Breast cancer is a heterogeneous disease and the most common malignant tumor among women in the world. According to US breast cancer statistics, 1 in 8 women will develop invasive breast cancer during their lifetime.Citation1,Citation2 In China, with the intensification of population aging and diversification of lifestyles, especially changes in reproductive habits, the incidence and death toll of female breast cancer in China are expected to continue to rise, increasing by 36.27 and 54.01% respectively by 2030.Citation3 Triple-negative breast cancer (TNBC) is a subtype of breast cancer that lacks the expression of estrogen receptor (ER), progesterone receptor (PR), and human epidermal growth factor receptor-2 (HER-2), it accounting for approximately 15 and 20% of all types of breast cancer.Citation4 Currently, the treatment of triple-negative breast cancer has many difficulties and faces huge challenges due to the disease’s poor prognosis, strong invasiveness, resistance to chemotherapy, and lack of molecular targeting. In recent years, many reports have suggested that ferroptosis, a form of regulated cell death characterized by the iron-dependent accumulation of lipid peroxidation to lethal levels, is inexorably linked to cancer. It is different from apoptosis, and the mechanism of apoptosis is mainly as follows: 1. Oxygen free radicals activate P53 gene; 2. Activated poly (ADP-ribose) transferase; 3, attack the unsaturated fatty acids on the cell membrane, directly causing damage to the cell membrane; 4. Activation of Ca2+/Mg2+ -dependent endonucleases; 5, cause the destruction of cell membrane structure, increase Ca2+ permeability; 6. Activate nuclear transcription factors such as NF-κB and AP-1, accelerate the expression of apoptosis related genes. It has been reported that TNBC cells are highly sensitive to oxidative stress and ferroptosis,Citation5 and the expression of TNBC glutathione (GSH) synthase is relatively low and more dependent on cystine/glutamate antitransport protein, it is more dependent on cystine/glutamate antitransporter protein (SLC7A11) -mediated amino acid metabolism. The combination of nanotechnology and traditional treatment methods has made the treatment of TNBC more diversified, and the curative effect has been significantly improved. In addition, the ultra-high safety and excellent biocompatibility of nanotechnology add a guarantee for the treatment of cancer with new nanotechnology. Therefore, this article reviews the research progress and treatment of ferroptosis and nanotechnology in triple negative breast cancer.
Overview and Mechanism of Ferroptosis
Ferroptosis is an iron-dependent programmed cell death that is different from apoptosis, necrosis, and autophagy. It is caused by the reaction of cytotoxic lipid free radicals ferrous iron with lipid peroxides. It was first introduced in It was first proposed by Dixon in 2012.Citation6,Citation7 According to current research progress, the mechanisms of ferroptosis mainly include oxidative stress pathways, iron metabolism pathways, etc.Citation8
Intracellular LPO Accumulation
Reduced glutathione (GSH) is an essential intracellular antioxidant produced from glutamate, cysteine, and glycine via the ATP-dependent cytosolic enzyme glutamate-cysteine ligase (GCL) It is synthesized in two steps with glutathione synthetase (GSS).Citation9 Some studies believe that the synthesis rate of glutathione is limited by the availability of cysteine.Citation10 The cystine-glutamate antiporter (System-Xc) is an intracellular antioxidant system composed of the transmembrane transport protein SLC7A11 and the transmembrane regulatory protein SLC3A2, which can reduce Cys (cystine) to cysteine acid, and participates in the synthesis of glutathione (GSH). GSH can reduce reactive oxygen species and reactive nitrogen species under the action of GSH peroxidase (GPX), and has antioxidant effects. When intracellular lipid peroxide (LPO) Accumulation to a certain level will induce ferroptosis. Therefore, when the activity or quantity of the cystine-glutamate antiporter (System-Xc) is affected, intracellular reactive oxygen species or lipid peroxides accumulate to a certain level, which will trigger ferroptosis.Citation8 TNBC is more dependent on amino acid metabolism mediated by the cystine/glutamate antiporter (SLC7A11), and the expression of glutathione (GSH) synthase is relatively low.
There are many members of the GPXs family, among which GPX4 is a key antioxidant enzyme in cells. It can reduce toxic LPO to non-toxic lipid alcohols and avoid oxidative damage to cells. Erastin is an inducer of ferroptosis, it can be divided into two categories: the first category of inducers acts by acting on the cystine- glutamate antiporter (system-Xc), such as: Erastin, etc.; the second category acts directly Inhibit the activity of glutathione peroxidase 4 (GPX4), such as RSL3, DP17, etc.Citation1,Citation11 Yang et alCitation12 found that erastin inhibited the import of cystine, leading to glutathione depletion and glutathione peroxidase 4 (GPX4) inactivation. Erastin inactivated cellular GPXs, resulting in cytoplasmic and lipid The production of mass ROS. Other antioxidant inhibitors will not consume GSH, which is an important cofactor for GPX enzyme activity, so they will not inhibit GPX activity or cause the accumulation of peroxide. Depleting GSH by using erastin or directly using the GPX4 inhibitor (1S,3R)-RSL3 inactivates GPX4, ultimately leading to overwhelming lipid peroxidationCitation9 ie, massive accumulation of LPO, leading to cell death. Therefore, the oxidative stress pathway is an important step in the ferroptosis process.
Iron Metabolism is Involved in Ferroptosis
Iron is an essential trace element for humans. It mainly exists in the body in the form of divalent or trivalent iron ions. Of course, iron metabolism also plays an important role in ferroptosis. Studies have foundCitation13 that when the amount of iron ions in the body is overloaded, excess ferrous iron ions generate hydroxyl radicals through the Fenton reaction to cause oxidative stress, producing a large amount of reactive oxygen species (ROS) to induce iron die. Feng et alCitation14 by immunizing mice with lymphoma cell membranes treated with ferroptosis inducer erastin, selective ferroptosis cells were detected. One of the ferroptosis antibody (3F3-FMA) was found to be an effective selective ferroptosis staining reagent. Antibodies to 3F3-FMA were tested in several cancer cells and the 3F3-FMA antigen was identified as the membrane protein transferrin receptor 1 (TFR1). TFR1 imports ferrous iron ions from the extracellular environment into cells via ferritin light chain (FTL) and ferritin heavy chain 1(ferritin heavy chain 1, FTH1) regulates the storage of iron ionsCitation15 and induces ferroptosis under the iron metabolism pathway. Some studies have foundCitation16 that HSPB1 can change the concentration of iron ions in the body by regulating TFR1, thereby affecting the process of ferroptosis. In summary, the iron metabolism pathway is different from the oxidative stress pathway, but they are interrelated and jointly regulate ferroptosis.
Other Related Ways
As a tumor suppressor gene, P53 is crucial for tumor suppression.Citation17 It mainly exerts its tumor suppressor function by inducing cell senescence, apoptosis, cell cycle arrest, DNA damage repair, and autophagy.Citation18 In addition, P53 is an important regulator of multiple pathways, including metabolism and ferroptosis, which is critical for tumor development and progression. Studies have shownCitation19 that p53 can inhibit the expression of SLC7A11 through a transcription-dependent mechanism and reduce the uptake of cystine, thus reducing the synthesis of GSH, causing the accumulation of intracellular LPO synthesis and inducing ferroptosis of cancer cells.
It has been reported that long-chain polyunsaturated fatty acids (PUFAs) in lipid molecules are more easily oxidized through free radicals or non-enzymatic reactions of enzymatic lipoxygenase, promoting the generation of LPO in the presence of iron ions, which is harmful to Tumor cells produce significant cytotoxicity.Citation20,Citation21 Recent studies have also found that other phospholipid peroxides can also induce ferroptosis in cells, such as phosphatidylethanolamine (PE) and phosphatidylcholine (PC).Citation22 Therefore, lipid reactive oxygen species-mediated cell damage is required for ferroptosis.
Ferroptosis suppressor protein 1 (FSP1) produces ubiquinone and is an enzyme that captures antioxidants (RTA).Citation23,Citation24 A recent study foundCitation25 that the reduced form of vitamin K is another potent anti-ferroptosis RTA, and FSP1 mediates the reduction of vitamin K to inhibit ferroptosis. Reduction of vitamin K is typically mediated by vitamin K epoxide reductase complex subunit 1 (VKORC1) and VKORC1-like 1 (VKORC1L1). Yang et alCitation26 not only verified several known ferroptosis regulators (such as FSP1), but also identified VKORC1L1 as a new ferroptosis suppressor gene. Yang et al also revealed a new connection between VKORC1L1 and the tumor suppressor protein p53, confirming that p53 directly regulates the expression of VKORC1L1 by binding to its promoter region, establishing VKORC1L1 as a direct transcription target of p53. Activation of p53 results in VKORC1L1 transcriptional repression, and p53-deficient cancer cells exhibit elevated VKORC1L1 levels. This study further highlights the critical role of VKORC1L1 in mediating ferroptosis resistance in p53-deficient cells, as its absence sensitizes these cells to ferroptosis. These findings provide valuable mechanistic insights into the regulation of ferroptosis by p53.Citation27 In addition, another report pointed out that Voltage-dependent anion channels (VDACs) are transmembrane channels that transport ions and metabolites. Yagoda et alCitation28 believe that erastin acts on VDACs, causing mitochondrial dysfunction, release of oxidative substances, and ultimately causing ferroptosis.
Research Progress of Ferroptosis in the Treatment of TNBC
Due to its heterogeneity, poor prognosis and lack of clear molecular targets for triple-negative breast cancer, in addition to surgical treatment and radiotherapy, chemotherapy such as anthracyclines and paclitaxel are currently the main clinical methods for the treatment of TNBC.Citation29 Furthermore, immunotherapy, targeted therapy, and combined nanotechnology also play an important role in the treatment of TNBC.
TNBC can accumulate acyl-coenzyme A (CoA) synthetase long-chain family member 4 (ACSL4) of polyunsaturated fatty acids (PUFAs) in the cancer cell membrane and transferrin receptor protein 1 (TfR1) responsible for iron import,Citation14,Citation30 which is closely related to iron death. One of the most important amino acids in TNBC cell development is Cys. Studies have shown that cystine deficiency can cause necrosis and ptosis of TNBC cells and ferroptosis.Citation31 Some researchers have found that treatment with the double tyrosine kinase inhibitor lapatinib and the solopromoter syracine can increase the expression of transferrin receptor (TFRC), reduce the expression of Ferroportin (FPN), promote intracellular iron accumulation and ROS levels, and ultimately induce ferroptosis in TNBC.Citation32
Chemotherapy and Ferroptosis Combined with Nanotechnology to Treat TBNC
The current clinical chemotherapy regimen for triple-negative breast cancer may show a certain degree of drug resistance during treatment, and is accompanied by a series of adverse reactions, making the treatment effect unsatisfactory. Therefore, there is a need to find a new treatment option to address the challenge of chemoresistance. There are reports that artemisinin (ART) and its derivatives, as first-line anti-malarial drugs widely used in clinical practice, can produce H2O2 in cells and can be used to supplement the lack of H2O2 in cells, thereby inducing ferroptosis.Citation33 Zhang et alCitation34 loaded chemotherapy drugs (DOX) and Dihydroartemisinin (DHA), one of the semi-synthetic derivatives of ART, into polyglutamic acid-stabilized Fe3O4 magnetic nanoparticles (Fe3O4-PGA) respectively. Fe3O4-PGA-DHA nanoparticles were obtained, and Fe3O4-PASPX-DOX nanoparticles were obtained by using Fe3O4 magnetic nanoparticles stabilized by loading polyaspartic acid. Under the conditions of the tumor microenvironment (TME), Fe2+ is released, catalyzes the Fenton reaction, and accelerates the accumulation of ROS and LPO. Transcriptomic analysis showed that Fe3O4-PGA-DHA + Fe3o4 - PASPX - DOX induced ferroptosis by inhibiting the phosphatidyl muscle 3-kinase (PL3K)/AKT/mammalian target of rapamycin (mTOR)/GPX4 pathway, and enhance the enrichment of apoptotic pathways (). The researchers randomly divided MDA-MB-231 tumor-bearing female mice into seven groups to evaluate the antitumor effects of the nanoparticles. The results showed that the Fe3O4-PGA-DHA group significantly inhibited tumor growth by 66%, indicating that Fe3O4-PGA was highly enriched at the tumor site and enhanced the anti-tumor effect of free DHA. Fe3O4-PASP-DOX group inhibited tumor growth by 48%. Most impressively, the Fe3O4-PGA-DHA + Fe3O4-PASP-DOX group had the most significant inhibitory effect on tumor growth (88%). Notably, there was no significant weight loss in any of these groups, suggesting that the nanoparticles had little cytotoxicity in the mice. Biological information analysis showed that inducing ferroptosis by inhibiting the expression of GPX4 can enhance the therapeutic effect of DOX on TNBC. This new type of nanoparticle not only has good biocompatibility and safety, but also has obvious effects on the treatment of TNBC. It also provides new ideas and methods for future research.
Figure 1 Fe3o4-pga-dha nanoparticles were obtained by loading dihydroartemisinin (DHA) onto polyglutamate-stabilized Fe3O4 magnetic nanoparticles (Fe3O4-PGA). Fe3o4-paspx-dox was obtained by combining DOX with Fe3O4-paspx magnetic nanoparticles (Fe3O4-paspx) loaded with polyaspartic acid. Two kinds of nano-drugs were injected into TME to release Fe2+ and DHA, Fe2+ and DHA induced ferroptosis through PL3K/AKT/mTOR/GPX4 pathway, and DOX promoted apoptosis of tumor cells, thereby jointly treating TNBC.
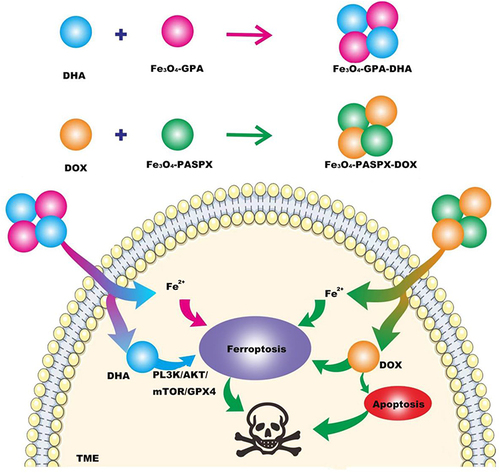
Radiotherapy and Ferroptosis Combined with Nanotechnology to Treat TNBC
Radiotherapy (RT) is one of the important clinical treatments for local control of triple-negative breast cancer (TNBC), such as X-ray, but the problem of radiotherapy resistance has always existed, seriously affecting the therapeutic effect of TNBC.Citation35 Therefore, improving radiotherapy resistance is urgent. Studies have foundCitation36 that RT upregulates the expression of acyl-CoA synthetase long chain family member 4 (ACSL4), and RT also induces the expression of solute carrier family 7 member 11 (SLC7A11) and GPX4. L-Buthionine-sulfoximine (BSO) is an inhibitor of γ glutamylcysteine synthetase (γ-GCS) and has been found to induce GSH depletion, thereby indirectly inactivating GPX4.Citation37 Since both radiotherapy and ferroptosis depend on ROS, Zeng et alCitation38 developed a multifunctional nanomedicine (BZAMH) based on metal-organic frameworks (MOFs). BSO is encapsulated in a ZIF-8 matrix, and then radiation-sensitive gold nanoparticles (Au NPs) are loaded in situ on the surface to give it a radiation-sensitizing effect. After loading, it is used as a template to form an iron-based metal polyphenol network (MPN), and finally modified with hyaluronic acid (HA) to obtain BSO/ZIF-8@Au@MPN@HA (BZAMH) nanohybrid. BZAMH nanohybrid combines ferroptosis induction and Sensitivity to radiotherapy in the TME and is used synergistically for RT treatment of TNBC (). The researchers gave X-ray irradiation to 4T1 tumour-bearing mice 6 h after the injection of the drug. It can be seen from the tumor volume curve of each mouse in each group that BZAMH+ X-ray treatment can effectively delay the growth of the tumor. During the treatment, the weight of mice in all groups showed the same trend of increase, indicating that this nanomide has good biocompatibility and no obvious toxicity to mice. The combination of nanomaterials and radiotherapy not only solves the problem of radiotherapy resistance, but also significantly improves the therapeutic effect of TNBC, opening up a new way for TNBC treatment.
Figure 2 BSO is an inhibitor of gamma-glutamylcysteine synthetase (gamma-GCs), which can induce GSH depletion and thus inactivate GPX4 to promote ferroptosis. Gold nanoparticles (Au NPs) have the effect of sensitizing radiation. BSO/ZIF-8@Au@MPN@HA (BZAMH) nanomaterials were obtained by modifying BSO, Au NPs and ZIF-8 matrix with hyaluronic acid (HA) and injected into tumor microenvironment to decompose and release BSO to promote ferroptosis of tumor cells. Au NPs enhances the sensitivity of radiotherapy, thereby combining TNBC treatment.
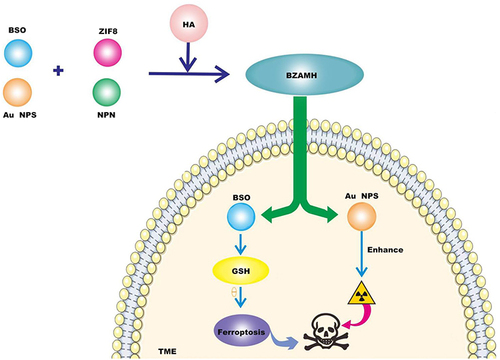
Targeted Ferroptosis Combined with Nanotechnology to Treat TNBC
Sorafenib (SRF), as a ferroptosis inducer, can limit the synthesis of GSH by inhibiting the cystine/glutamate antiporter (System Xc¶), thereby inhibiting the expression of GPX4 and inducing ferroptosis.Citation39 Atovaquone (ATO) is the only clinical drug targeting mitochondria.Citation40 It can hinder cellular respiration by targeting and inhibiting the mitochondrial oxidative phosphorylation (OXPHOS) process and preserve the production of reactive oxygen species (ROS). Studies have reportedCitation41 that ATO can inhibit the reduction of CoQ to CoQH2 catalyzed by DHODH. This process is crucial for mitochondria to resist the generation of lipid peroxides. Due to SRF’s selective killing effect on TNBC cells and its good self-assembly ability and the importance of ATO in ferroptosis, as well as the hydrophilicity and high binding of BSA, Zhou et alCitation42 used Bovine serum albumin (BSA) self-assembled to co-load ATO and SRF to prepare nanomedicine, named ATO/SRF@BSA. The drug releases SRF in the TME to consume GSH and induce ferroptosis. ATO targets mitochondria by occupying the DHODH catalytic site, inhibits the production of CoQH2, and downregulates the detoxification effect of CoQH2 produced in mitochondria on lipid peroxides, thereby increasing lipid peroxides. The accumulation of oxides more efficiently plays the role of ferroptosis in the treatment of TNBC (). We evaluated the antitumor effect of ATO/SRF@BSA on 4T1 tumors after 24 hours of administration in tumor-bearing mice, where ATO and SRF accumulated in tumor tissues. When the tumor volume reached about 100 mm3, the tumor-bearing mice were randomly divided into 4 groups and given ATO@BSA, SRF@BSA, ATO/ SRF@BSA every 3 days for 5 times in total. Serum CREA, BUN, ALT, ALP and AST were all within the normal range, which verified that all albumin nanoparticles had no obvious cytotoxicity and adverse effects on liver and kidney function. Compared with the untreated group, SRF@BSA inhibited the growth of tumor volume, while ATO@BSA had no significant anticancer effect. ATO/SRF@BSA can significantly slow down tumor proliferation, showing good anti-cancer effects. Bsa-based nanomedical drugs hold great promise in terms of their simplicity and substantial enhancement in the anti-cancer system, producing remarkable anti-cancer effects and providing a new perspective for the treatment of TNBC.
Figure 3 Sorafenib (SRF) and atovaquone (ATO) were loaded into bovine serum albumin (BSA) to prepare nanomaterials (ATO/SRF@BSA). ATO/SRF@BSA was injected into TME, and the ferroptosis inducer SRF was released to induce iron death of tumor cells. The release of targeted mitochondrial drug ATO down-regulates the defense mechanism of DHODH-Coenzyme Q (CoQH2), promotes the accumulation of lipid peroxides in mitochondria, triggers the explosion of lipid peroxides, and thus promotes ferroptosis of tumor cells.
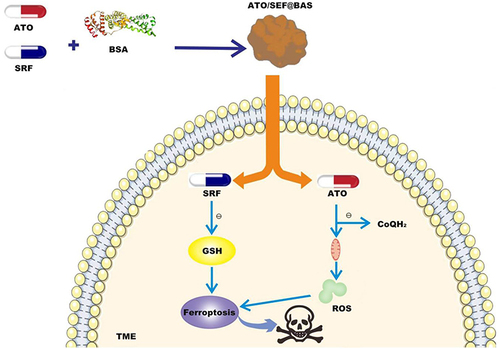
Immunotherapy and Ferroptosis Combined with Nanotechnology to Treat TNBC
Immunotherapy relies on the body’s own immune function to kill cancer cells or tumor tissue by activating the human immune system. However, due to the low tumor immunogenicity and limitations of the immunosuppressive tumor microenvironment (TME) in TNBC, the response to immunotherapy has been poor so far.Citation43 Therefore, a dual immunomodulatory strategy that effectively induces immunogenic death of tumor cells and reverses the immunosuppressive TME is needed to provide new treatment options for TNBC patients. Based on manganese (Mn)’s powerful ability to disrupt redox homeostasis and Ca2+’s excellent ability to induce ferroptosis through the Fenton reaction,Citation44,Citation45 and Mn2+’s enhanced sensitivity of the STING pathway to stimulate interferon (IFN-I) and the secretion of other pro-inflammatory cytokines enhances the function of immune cells.Citation46 Deng et alCitation47 constructed a Ca & Mn Dual ionic hybrid nanostimulants (CMS) that simultaneously acts as a ferroptosis agent for TNBC. Inducers and immune adjuvants. CMS catalyzes the decomposition of H2O2 to generate O2, Relief of intra-tumoral hypoxia, awakens the innate immune system, further reduces the expression of HIF-1α, and promotes the polarization of anti-inflammatory M2 tumor-associated macrophages (TAMs) from the M2 phenotype to the M1 phenotype. Mn2+-induced activation of the STING signaling pathway facilitates the efficient activation of dendritic cells (DCs) for antigen presentation and subsequent cytotoxic T lymphocytes (CTL) infiltration into tumor tissues (). The researchers established a tumor model. When the primary tumor size reached about 80 mm3, the 4t1 tumor-bearing mice were randomly divided into four groups. During 14 days of treatment, the weight of tumor-bearing mice in each group did not show abnormal changes. Histological observation of the main organs (heart, liver, spleen, lung, kidney) during treatment in the control group and the treatment group showed that the acute pathological toxicity and adverse reactions were negligible, indicating that there were no obvious side effects of treatment in each group. The tumor growth in CMS group was significantly slowed down. Among them, the tumor weight of the distal tumor in the CMS (20 mg/kg) group was also significantly lower than that in the other groups. These results suggest that CMS can also inhibit the growth of distant tumors, which may be related to the activation of anti-tumor immune responses. Nanomaterials can induce iron death and awaken innate immunity, enhance tumor immunogenicity, reverse immunosuppressive TME, and thus enhance anti-tumor immunity, so as to achieve the purpose of effective tumor immunotherapy by TNBC.
Figure 4 A kind of Ca & Mn dual-ion mixed nanomaterial (CMS) was constructed. In TME, the mixed valence of Mn in CMS leads to the consumption of GSH and the production of ROS to induce iron death, and Ca2+ induces iron death through Fenton reaction. CMS awakens innate immunity by alleviating intratumor hypoxia and activation of STING signaling pathway induced by Mn2+, promotes the polarization of macrophages (tam) from M2 phenotype to M1 phenotype, and effectively activates dendritic cells (dc) for antigen presentation and tumor cytotoxic T lymphocytes (ctl) infiltration into tumor tissues. Thus combined treatment of TNBC.
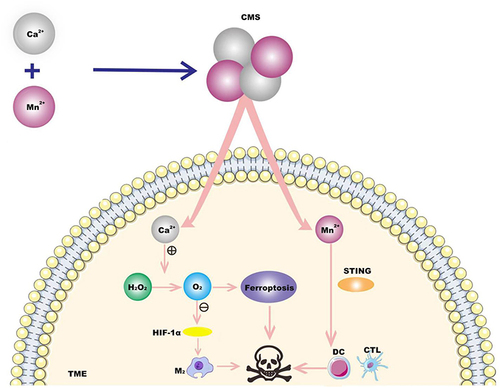
Chemical Kinetics and Ferroptosis Combined with Nanotechnology to Treat TNBC
Chemodynamic therapy (CDT) was first proposed by Bu et alCitation48 in 2016. CDT uses the weak acidity of the tumor microenvironment as the reaction condition, excess H2O2 as the reaction substance, and divalent Fe ions as the catalyst to trigger the Fenton reaction in tumor cells, catalyze H2O2 to generate •OH, and induce ferroptosis of tumor cells, thereby achieving The purpose of treating cancer.Citation49 Wang et alCitation50 prepared CT/CDT nanoparticles using functional materials and drugs such as hyaluronic acid (HA), maleimidocaproic acid (Mal), Ferrocene carboxylic acid (Fc), and DOX. Targeted drug delivery of nanoparticles is achieved based on HA, Mal consumes GSH and improves ferroptosis efficiency, and Fc can generate ROS under high H2O2 conditions and induce ferroptosis and apoptosis after loading DOX. The researchers established a 4 T1 tumor-bearing mouse model. When the tumor volume reached about 85 mm3, the tumor-bearing mice were randomly divided into 4 groups and given IV injection once every 2 days for a total of 4 times. The experiment lasted for 20 days. (DOX·HCl) group and CDT group (HCM) had moderate tumor inhibition. However, tumor volume and weight in the HCM@DOX group were significantly lower than those in the DOX·HCl and HCM groups. The tumor inhibition rate of HCM@DOX group was 81.87%, indicating that HCM@DOX has excellent anti-tumor efficiency. After 20 days of treatment, the tumor was resected and systematic pathologic analysis was performed by H&E, TUNEL, Ki67, GPX4 staining. The degree of apoptosis detected by H&E and TUNEL in HCM@DO group was higher than that in other groups. The mean body weight of mice in the DOX⋅HCl group began to decrease after the first dose and began to increase after withdrawal. In contrast, the average body weight of mice in the HCM@DOX group was relatively stable during treatment, suggesting that HCM@DOX was least toxic in vivo. The levels of ALT, AST, BUN and CRE in HCM@DOX group were all within the normal range after treatment, and there was no obvious damage in HE staining. Compared with the PBS group, the small animal ultrasound imager showed no cardiotoxicity HCM@DOX, confirming the safety of nanomaterials ().
Figure 5 CT/CDT nanoparticles were prepared using functional materials and drugs such as hyaluronic acid (HA), maleimide caproic acid (Mal), ferrocene carboxylic acid (Fc) and DOX. They were released in TME, Mal consumed GSH and improved the efficiency of ferroptosis. Fc is a stable organometallic complex, and the oxidation state of iron atom in the center of Fc is Fe2+. Under high H2O2 conditions, ROS can induce ferroptosis of tumor cells, and DOX can induce ferroptosis and apoptosis, so as to combine TNBC treatment.
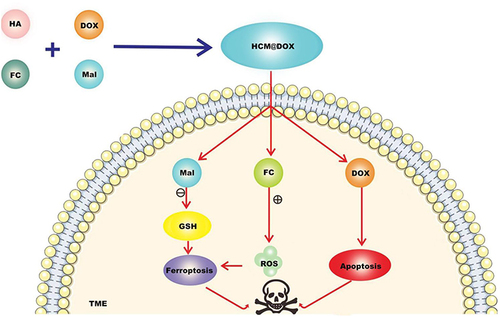
Combined Ethnopharmacology and Ferroptosis in the Treatment of TNBC
After introducing the treatment of TNBC by iron death combined with nanotechnology, the ethnopharmacology should attract our attention。Danggui Buxue Decoction (DBT) has been used in traditional Chinese medicine to nourish blood, enhance the immune system, and improve overall health.Citation51 The nuclear factor erythroid-related factor 2 (Nrf2) pathway, like heme oxygenase 1 (HO-1), plays a crucial role in regulating cellular antioxidant responses and protecting cells from oxidative stress.Citation52,Citation53 Inhibiting the activation of antioxidant pathways such as Nrf2/HO-1 leads to the accumulation of intracellular iron and induces ferroptosis.Citation54 Gong et alCitation55 found that DBT alone or combined with DOX could inhibit TNBC cell proliferation, and combined administration of DBT further enhanced the cell death and ROS production of TNBC cells. In vitro experiments also demonstrated that DOX and DBT combined to treat TNBC by inducing ferroptosis and destroying the Nrf2/HO-1/GPX4 axis. Polygala saponin is a traditional Chinese medicine, from which Polygala saponin can be extracted, which has anti-inflammatory and analgesic effects,Citation56 and some studies have foundCitation57 that Polygala saponin is also of some significance in the treatment of cancer. Li et alCitation58 found that Polygala saponin may promote the expression of p53, inhibit the expression of SLC7A11, which leads to the occurrence of cellular ferroptosis. Through this mechanism, Polygala saponin can inhibit the proliferation of MDA-MB-231 cells in triple-negative breast cancer, thus achieving the effect of TNBC treatment.
Conclusion and Outlook
In recent years, research on ferroptosis in TNBC has shown that ferroptosis plays an increasingly important role in the development, treatment, and prognosis of TNBC. Ferroptosis-inducing agents and classic anti-TNBC drugs are combined into nanoparticles, providing a promising approach for the treatment of TNBC. However, the current research on ferroptosis in TNBC is still in the basic research stage. In the future, we will further integrate ferroptosis and TNBC treatment more efficiently and closely, find specific drugs to target TNBC cells, or combine classic TNBC drugs to accurately promote Ferroptosis of TNBC cells is expected to provide new treatment strategies for TNBC. Inducing ferroptosis is a promising anti-cancer strategy, and we need more research to explore the role of ferroptosis-related factors in TNBC and provide new therapeutic targets for TNBC. In addition, the study of ethnopharmacology in conjunction with ferroptosis has opened new avenues in the treatment of TNBC and laid the foundation for solving the difficult problem of cancer.
Abbreviations
TNBC, Three negative breast cancer; GSH, Glutathione; GPX, Glutathione peroxidase; LPO, Lipid peroxides; SLC7A11, Cystine/glutamate antitransporter; System-Xc, Cystine - reverse glutamate transporter; SLC3A2, Across the membrane regulatory proteins; HSPB1, Heat shock proteins; PUFAs, Unsaturated fatty acids; PE(18:1_22:6), Phosphatidylethanolamine; VKORC1L1, Vitamin K epoxide reductase complex subtype-like 1; RTA, Radical trapping antioxidants; VDACs, Voltage dependent anion channel; Fe3O4- PGA, Polyglutamic acid stabilized Fe3O4 magnetic nanoparticles; TME, Tumor microenvironment; ACSL4, Member 4 of the long chain family of acyl-coa synthetases; γ-GCS, γ-glutamylcysteine synthetase; Au NPs, Au nanoparticles; BZAMH, BSO/ZIF-8@Au@MPN@HA; SRF, Ferroptosis inducers; ATO, Atovaquone; OXPHOS, Mitochondrial oxidative phosphorylation; BSA, Bovine serum albumin; DBT, Danggui Buxue Decoction; MDA-MB-231, Three negative breast cancer cells; Cys, cystine; Erastin, Ferroptosis inducers; ROS, Reactive oxygen species; RSL3, DP17, Glutathione peroxidase 4 inhibitors; 3F3- FMA, 3F3 ferric membrane antibodies; TFR1, Membrane protein transferrin receptor 1; FTL, Ferritin light chain; FTH1, Ferritin heavy chain 1; DOX, Doxorubicin; DHA, Dihydroartemisinin; VKORC1, Vitamin K epoxide reductase complex subunit 1; PC(18:0_22:6), Phosphatidylcholine; ART, Artemisia annua tiger ester; Fe3O4-PASPX, Aspartic acid stabilized Fe3O4 magnetic nanoparticles; RT, Radiation therapy; BSO, 1-Buthionine sulfoximine; MOFs, Metal-organic framework; HA, Hyaluronic acid; ZIF-8, Zeolite imidazole ester skeleton material; DHODH, Dihydrogen orotic acid dehydrogenase; CDT, Chemodynamic therapy; CMS, Calcium manganese double ion mixed nano-stimulator; MAL, Maleimidocaproic acid; FC, Ferrocene carboxylic acid; Polygala saponin, A kind of traditional Chinese medicine.
Ethical Approval
This study did not involve human or animal subjects, and thus, no ethical approval was required.
Author Contributions
All authors made a significant contribution to the work reported, whether that is in the conception, study design, execution, acquisition of data, analysis and interpretation, or in all these areas; All authors contributed to data analysis, drafting or revising the article, have agreed on the journal to which the article will be submitted, gave final approval of the version to be published, and agree to be accountable for all aspects of the work.
Disclosure
The authors report no conflicts of interest in this work.
Data Sharing Statement
Data availability is not applicable to this article as no new data were created or analyzed in this study.
Additional information
Funding
References
- Gautam N, Palte MJ, Deik AA, et al. Reviewing the significance of dendritic cell vaccines in interrupting breast cancer development. Mol Aspects Med. 2023;95(1):1617. doi:10.1016/j.mam.2023.101239
- Siegel RL, Miller KD, Wagle NS, et al. Cancer statistics. Cancer J Clinicians. 2023;73(1):17–48. doi:10.3322/caac.21763
- Huang Z, Wen W, Zheng Y, et al. Breast cancer incidence and mortality: trends over 40 years among women in Shanghai, China. Ann Oncol. 2016;27(6):1129–1134. doi:10.1093/annonc/mdw069
- Giaquinto AN, Sung H, Miller KD, et al. Breast cancer statistics, 2022. A Cancer J Clinicians. 2022;72(6):524–541. doi:10.3322/caac.21754
- Li P, Lin Q, Sun S, et al. Inhibition of cannabinoid receptor type 1 sensitizes triple-negative breast cancer cells to ferroptosis via regulating fatty acid metabolism. Cell Death Dis. 2022;13(9):808. doi:10.1038/s41419-022-05242-5
- Dixon SJ, Lemberg K, Lamprecht M, et al. Ferroptosis: an iron-dependent form of nonapoptotic cell death. Cell. 2012;149(5):1060–1072. doi:10.1016/j.cell.2012.03.042
- Rennekamp AJ. The Ferrous Awakens. Cell. 2017;171(6):1225–1227. doi:10.1016/j.cell.2017.11.029
- Guo Q, Chen G. Research on regulatory mechanism of ferroptosis and progress in tumorigenesis and therapy. Prog in Physiol Sci. 2019;50(2):88–93.
- Stockwell BR, Friedmann Angeli JP, Bayir H, et al. Ferroptosis: A regulated cell death nexus linking metabolism, redox biology, and disease. Cell. 2017;171(2):273–285. doi:10.1016/j.cell.2017.09.021
- Hirayama T, Nagasawa H. Chemical tools for detecting fe ions. J Clin Biochem Nutr. 2017;60(1):39–48. doi:10.3164/jcbn.16-70
- Liang C, Zhang X, Yang M, et al. Recent progress in ferroptosis inducers for cancer therapy. Adv Mater. 2019;31(51):e1904197. doi:10.1002/adma.201904197
- Yang WS, SriRamaratnam R, Welsch M, et al. Regulation of ferroptotic cancer cell death by GPX4. Cell. 2014;156(1–2):317–331. doi:10.1016/j.cell.2013.12.010
- Manz DH, Blanchette NL, Paul BT, et al. Iron and cancer: recent insights. Ann N Y Acad Sci. 2016;1368(1):149–161. doi:10.1111/nyas.13008
- Feng H, Schorpp K, Jin J, et al. Transferrin receptor is a specific ferroptosis marker. Cell Rep. 2020;30(10):3411–3423.e7. doi:10.1016/j.celrep.2020.02.049
- Zeng J, Chen Y, Xu X. Research progress of ferroptosis-related mechanisms, regulation and diseases. Chin Pharm J. 2017;52(4):253–257.
- Sun X, Ou Z, Xie M, et al. HSPB1 as a novel regulator of ferroptotic cancer cell death. Oncogene. 2015;34(45):5617–5625. doi:10.1038/onc.2015.32
- Kastenhuber ER, Lowe SW. Putting p53 in context. Cell. 2017;170(6):1062–1078. doi:10.1016/j.cell.2017.08.028
- Mello SS, Attardi LD. Deciphering p53 signaling in tumor suppression. Curr Opin Cell Biol. 2018;51:65–72. doi:10.1016/j.ceb.2017.11.005
- Jiang L, Kon N, Li T, et al. Ferroptosis as a p53-mediated activity during tumour suppression. Nature. 2015;520(7545):57–62. doi:10.1038/nature14344
- Doll S, Proneth B, Tyurina YY, et al. ACSL4 dictates ferroptosis sensitivity by shaping cellular lipid composition. Nat Chem Biol. 2017;13(1):91–98. doi:10.1038/nchembio.2239
- Yang WS, Kim KJ, Gaschler MM, et al. Peroxidation of polyunsaturated fatty acids by lipoxygenases drives ferroptosis. Proc Natl Acad Sci USA. 2016;113(34):E4966–75. doi:10.1073/pnas.1603244113
- Zou Y, Henry WS, Ricq EL, et al. Plasticity of ether lipids promotes ferroptosis susceptibility and evasion. Nature. 2020;585(7826):603–608. doi:10.1038/s41586-020-2732-8
- Bersuker K, Hendricks JM, Li Z, et al. The CoQ oxidoreductase FSP1 acts parallel to GPX4 to inhibit ferroptosis. Nature. 2019;575(7784):688–692. doi:10.1038/s41586-019-1705-2
- Doll S, Freitas FP, Shah R, et al. FSP1 is a glutathione-independent ferroptosis suppressor. Nature. 2019;575(7784):693–698. doi:10.1038/s41586-019-1707-0
- Mishima E, Ito J, Wu Z, et al. A non-canonical vitamin K cycle is a potent ferroptosis suppressor. Nature. 2022;608(7924):778–783. doi:10.1038/s41586-022-05022-3
- Yang X, Wang Z, Zandkarimi F, et al. Regulation of VKORC1L1 is critical for p53-mediated tumor suppression through vitamin K metabolism. Cell Metab. 2023;35(8):1474–1490.e8. doi:10.1016/j.cmet.2023.06.014
- Liu X, Zhuang L, Gan B. Unleashing ferroptosis for cancer therapy with warfarin. Trends Endocrinol Metab. 2023;34(11):683–684. doi:10.1016/j.tem.2023.08.008
- Yagoda N, Von Rechenberg M, Zaganjor E, et al. RAS-RAF-MEK-dependent oxidative cell death involving voltage-dependent anion channels. Nature. 2007;447(7146):864–868. doi:10.1038/nature05859
- Dent R, Trudeau M, Pritchard KI, et al. Triple-negative breast cancer: Clinical features and patterns of recurrence. Clin Cancer Res. 2007;13(15 Pt 1):4429–4434. doi:10.1158/1078-0432.CCR-06-3045
- Verma N, Vinik Y, Saroha A, et al. Synthetic lethal combination targeting BET uncovered intrinsic susceptibility of TNBC to ferroptosis. Sci Adv. 2020;6(34). doi:10.1126/sciadv.aba8968.
- Chen MS, Wang S-F, Hsu C-Y, et al. CHAC1 degradation of glutathione enhances cystine-starvation-induced necroptosis and ferroptosis in human triple negative breast cancer cells via the GCN2-eIF2α-ATF4 pathway. Oncotarget. 2017;8(70):114588–114602. doi:10.18632/oncotarget.23055
- Wang Y, Sun Y, Wang F, et al. Ferroptosis induction via targeting metabolic alterations in triple-negative breast cancer. Biomed Pharmacother. 2023;169:115866. doi:10.1016/j.biopha.2023.115866
- Tu Y. The discovery of artemisinin (qinghaosu) and gifts from Chinese medicine. Nat Med. 2011;17(10):1217–1220. doi:10.1038/nm.2471
- Zhang J, Zhou K, Lin J,et al. Ferroptosis-enhanced chemotherapy for triple-negative breast cancer with magnetic composite nanoparticles. Biomaterials. 2023;303:122395.
- Bai X, Ni J, Beretov J, et al. THOC2 and THOC5 regulate stemness and radioresistance in triple-negative breast cancer. Adv Sci. 2021;8(24):e2102658. doi:10.1002/advs.202102658
- Lang X, Green MD, Wang W, et al. Radiotherapy and immunotherapy promote tumoral lipid oxidation and ferroptosis via synergistic repression of SLC7A11. Cancer Discov. 2019;9(12):1673–1685. doi:10.1158/2159-8290.CD-19-0338
- Gao Y, Li Y, Cao H, et al. Hypertoxic self-assembled peptide with dual functions of glutathione depletion and biosynthesis inhibition for selective tumor ferroptosis and pyroptosis. J Nanobiotechnol. 2022;20(1):390. doi:10.1186/s12951-022-01604-5
- Zeng L, Ding S, Cao Y, et al. A MOF-based potent ferroptosis inducer for enhanced radiotherapy of triple negative breast cancer. ACS Nano. 2023;17(14):13195–13210. doi:10.1021/acsnano.3c00048
- Chen X, Kang R, Kroemer G, et al. Broadening horizons: the role of ferroptosis in cancer. Nat Rev Clin Oncol. 2021;18(5):280–296. doi:10.1038/s41571-020-00462-0
- Burnell ES. Drugs targeting mitochondrial functions, in Antimalarial Agents; 2020:375–402.
- Ashton TM, Fokas E, Kunz-Schughart LA, et al. The anti-malarial atovaquone increases radiosensitivity by alleviating tumour hypoxia. Nat Commun. 2016;7(1):12308. doi:10.1038/ncomms12308
- Zhou TJ. Glutathione depletion and dihydroorotate dehydrogenase inhibition actuated ferroptosis-augment to surmount triple-negative breast cancer. Biomaterials. 2024;2:305.
- Adams S, Gatti-Mays ME, Kalinsky K, et al. Current landscape of immunotherapy in breast cancer: A review. JAMA Oncol. 2019;5(8):1205–1214. doi:10.1001/jamaoncol.2018.7147
- Fu F, Wang W, Wu L, et al. Inhalable biomineralized liposomes for cyclic Ca(2+)-burst-centered endoplasmic reticulum stress enhanced lung cancer ferroptosis therapy. ACS Nano. 2023;17(6):5486–5502. doi:10.1021/acsnano.2c10830
- Zhu P, Pu Y, Wang M, et al. Correction to “MnOOH-Catalyzed autoxidation of glutathione for reactive oxygen species production and nanocatalytic tumor innate immunotherapy”. J Am Chem Soc. 2023;145(18):10441–10442. doi:10.1021/jacs.3c03717
- Sun X, Zhang Y, Li J, et al. Amplifying STING activation by cyclic dinucleotide-manganese particles for local and systemic cancer metalloimmunotherapy. Nat Nanotechnol. 2021;16(11):1260–1270. doi:10.1038/s41565-021-00962-9
- Deng X, Liu T, Zhu Y, et al. Ca & Mn dual-ion hybrid nanostimulator boosting anti-tumor immunity via ferroptosis and innate immunity awakening. Bioact Mater. 2024;33:483–496. doi:10.1016/j.bioactmat.2023.11.017
- Zhang C, Bu W, Ni D, et al. Synthesis of iron nanometallic glasses and their application in cancer therapy by a localized Fenton reaction. Angew Chem Int Ed Engl. 2016;55(6):2101–2106. doi:10.1002/anie.201510031
- Wang C, Xue F, Wang M, et al. 2D Cu-Bipyridine MOF nanosheet as an agent for colon cancer therapy: A three-in-one approach for enhancing chemodynamic therapy. ACS Appl Mater Interfaces. 2022;14(34):38604–38616. doi:10.1021/acsami.2c11999
- Wang N. A chemo/chemodynamic nanoparticle based on hyaluronic acid induces ferroptosis and apoptosis for triple-negative breast cancer therapy. Carbohydr Polym. 2024;2:329.
- Cao C. Ferulic acid orchestrates anti-oxidative properties of danggui buxue tang, an ancient herbal decoction: Elucidation by chemical knock-out approach. PLoS One. 2016;11(11):1.
- Ganesan K, Ramkumar KM, Xu B. Vitexin restores pancreatic β-cell function and insulin signaling through Nrf2 and NF-κB signaling pathways. Eur J Pharmacol. 2020;888:173606. doi:10.1016/j.ejphar.2020.173606
- Yang J, Mo J, Dai J, et al. Cetuximab promotes RSL3-induced ferroptosis by suppressing the Nrf2/HO-1 signalling pathway in KRAS mutant colorectal cancer. Cell Death Dis. 2021;12(11):1079. doi:10.1038/s41419-021-04367-3
- Cheng Y, Gao Y, Li J, et al. TrkB agonist N-acetyl serotonin promotes functional recovery after traumatic brain injury by suppressing ferroptosis via the PI3K/Akt/Nrf2/Ferritin H pathway. Free Radic Biol Med. 2023;194:184–198. doi:10.1016/j.freeradbiomed.2022.12.002
- Gong G. Danggui buxue tang improves therapeutic efficacy of doxorubicin in triple negative breast cancer via ferroptosis. J Ethnopharmacol. 2024;3:323.
- Son SR, Yoon Y-S, Hong J-P, et al. Chemical constituents of the roots of polygala tenuifolia and their anti-inflammatory effects. Plants. 2022;11(23):3307. doi:10.3390/plants11233307
- Hao K-X, Shen C-Y, Jiang J-G. Sedative and hypnotic effects of polygala tenuifolia willd. Saponins on insomnia mice and their targets. J Ethnopharmacol. 2024;323:117618. doi:10.1016/j.jep.2023.117618
- Li X, et al. Studies on Paris saponin promoting iron death in triple negative breast cancer cells through p53/SLC7A11 signal axis. Med J Chin People Liber. 2023;48(1):58–63.