Abstract
Slick and Slack high-conductance potassium channels have been recently discovered, and are found in the central nervous system and in the heart. Both channels are activated by Na+ and Cl−, and Slick channels are also inhibited by adenosine triphospate (ATP). An important role of setting the resting membrane potential and controlling the basal excitability of neurons has been suggested for these channels. In addition, no specific blockers for these channels are known up to the present. With the purpose of studying the pharmacological characteristics of Slick and Slack channels, the effects of exposure to the antiarrhythmic compound clofilium were evaluated. Clofilium was able to modulate the activity of Slick and Slack channels effectively, with a stronger effect on Slack than Slick channels. In order to evaluate the pharmacological behavior of Slick and Slack channels further, 38 commonly used potassium channel blockers were tested. Screening of these compounds did not reveal any modulators of Slick and Slack channels, except for clofilium. The present study provides a first approach towards elucidating the pharmacological characteristics of Slick and Slack channels and could be the basis for future studies aimed at developing potent and specific blockers and activators for these channels.
Introduction
Potassium channels are essential for numerous physiological processes, and are involved in a number of disorders, including cardiac arrhythmias, neuronal diseases (episodic ataxia, neurodegeneration), and renal disease (Bartter’s syndrome).Citation1 A number of small molecules with the ability either to block or to open specific types of potassium channels have been identified, and may be potential drugs for treatment of a number of diseases.Citation2 In addition, a number of peptides derived from venom of the scorpion (see below), snakes (dendrotoxin), spiders (hanatoxin, SGTx), snails (conotoxins), and the honey bee (apamin, tertiapin) have been identified as being highly selective blockers of a number of ion channels. Scorpion toxins with the ability to block potassium channels include: charybdotoxin and iberiotoxin, affecting Slo1 channels; margatoxin and agitoxin, modulating Kv1.3 channels; and scyllatoxin and tamapin, affecting Slo1 channels.Citation3 In general, peptide toxins (about 20–60 amino acids in length) have high selectivity and bind to relatively large external motifs of potassium channels in contrast with small molecules (molecular weight 200–500), which normally occlude the inner pore.Citation2 However, due to differential expression of the numerous potassium channels in distinct cell types and even in specific regions inside cells, development of modulators constitutes a challenge for both academia and pharmaceutical companies.
Slick (Slo2.1) and Slack (Slo2.2) are potassium channels encoded by the Slo gene family of high-conductance potassium channels. In contrast with Slo1 channels, Slick and Slack channels are not activated by Ca2+, but by Na+ and Cl−.Citation4 Furthermore, Slick channels are also inhibited by adenosine triphosphate (ATP).Citation5 Both channels have been found to be widely distributed in the central nervous system and apparently in cardiac myocytes.Citation6,Citation7 Given these characteristics, together with their low voltage sensitivity, Slick and Slack channels most likely play an important role in tuning of the resting potential and basal excitability in neurons. The importance of channels setting the resting membrane potential seems to increase during episodes of hypoxia, when the intracellular Na+ concentration rises due to failure of Na+/K+-ATPase. The protective role these channels may have during hypoxia is supported by the fact that mutations of the Slack ortholog in Caenorhabditis elegans result in animals that are hypersensitive to hypoxia.Citation4 In addition, it has been shown that Slack channels are activated by estradiol, a fact that could account for estradiol-dependent neuroprotection in ischemia.Citation8 Together, these observations raise the possibility that Slick and Slack channels could be used therapeutically as drug targets. Additionally, identification of pharmacological agents targeting these channels will result in important tools for the study of the physiological significance of Slick and Slack channels in different tissues and organs. However, at present, knowledge concerning the pharmacology of Slick and Slack channels is limited, and no specific pharmacological agents targeting Slick and Slack potassium channels have been identified. For Slack channels, antiarrhythmic drugs like quinidine and bepridil have been found to act as inhibitors, and bithionol, an antiparasitic compound, is an activator of Slack channels as well as Slo1 channels.Citation9 In addition, it was recently found that niflumic acid and loxapine, an antipsychotic drug, activate Slick and Slack channels, respectively.Citation10,Citation11 The present work constitutes a first approach towards analyzing the effect of clofilium, a potent antiarrhythmic compound, on Slick and Slack channels. A number of other well known ion channel modulators were also screened for a potential effect.
Materials and methods
Heterologous expression of Slick and Slack channels in Xenopus laevis oocytes
Preparation of Xenopus laevis oocytes was performed as previously described by Grunnet et al.Citation12 hSlick and rSlack cDNA cloned into a pOX vector (kindly provided by L Salkoff) was linearized with NotI (New England Biolabs, Ipswich, MA). mRNA was obtained by in vitro transcription using a mMessage mMachine kit (Ambion, Carlsbad, CA) according to the manufacturer’s instructions. RNA was extracted using a MEGAclear kit (Ambion). Next, 50 nL containing approximately 10 ng of Slick or Slack mRNA was injected into the oocytes, which were cultured in Kulori medium (90 nM NaCl, 1 mM KCl, 1 mM MgCl2, 1 mM CaCl2, 5 mM HEPES, pH 7.4) at 19°C for 4–5 days prior to our experiments.
Electrophysiological measurements and screening for Slick and Slack blockers
Expression of Slick and Slack channels was verified by measuring current flow through the channels using a two-electrode voltage clamp amplifier (Warner Instruments, Novarto, CA). Oocytes were impaled with electrodes pulled from a micropipette puller (P-97, Sutter Instruments, Novato, CA) and filled with 1 M KCl (resistance about 0.5–1.5 mΩ). Currents were measured either by a stepwise protocol, ie, 500 msec depolarizations from −100 to +80 mV (holding potential −80 mV for 4 seconds) or by a pulse protocol, ie, 500 msec depolarizations from −80 to +80 mV (holding potential −80 mV for 3 seconds).
In order to screen for compounds able to modulate the activity of Slick and Slack channels, 38 potassium channel blockers (Alomone, Jerusalem, Israel) were dissolved in bath solution (Kulori) immediately before the experiments were conducted. Currents were measured before and after treatment. The compounds tested were E-4031 (10 μM), recombinant margatoxin (300 nM), mast cell degranulating peptide (10 μM), δ-dendrotoxin (1 μM), dendrotoxin K (1 μM), dendrotoxin-I (1 μM), recombinant BeKm-1 (500 nM), recombinant Aa1 (1.3 μM), BDS-I (1.06 μM), BDS-II (1.05 μM), recombinant argitoxin-1 (500 nM), recombinant argitoxin-2 (500 nM), recombinant argitoxin-3 (500 nM), sApamin (10 μM), γ-dendrotoxin (1 μM), α-dendrotoxin (2 μM), recombinant charybdotoxin (500 nM), recombinant tityustoxin Kα (300 nM), β-dendrotoxin (2 μM), recombinant ergotoxin (500 nM), recombinant hongotoxin-1 (955 nM), recombinant OsK (500 nM), recombinant kaliotoxin-1 (500 nM), Lq2 (300 nM), recombinant maurotoxin (600 nM), recombinant noxiustoxin (1 μM), phrixotoxin-2 (1 μM), recombinant scyllatoxin (500 nM), recombinant stichodactyla toxin (500 nM), recombinant stromatoxin (1.32 μM), recombinant tertiapin Q (1 μM), recombinant tapamin (1 μM), recombinant tertiapin (1 μM), recombinant Heteropodatoxin-2 (1 μM), recombinant iberiotoxin (500 nM), paxiline (5 μM), penitrem A (5 μM), and verruculogen (5 μM). Paxiline, penitrem A, and verruculogen were dissolved in dimethyl sulfoxide to prepare stocks and then further diluted to final concentrations in Kulori medium. Dimethyl sulfoxide did not exceed 0.01% in the final solutions used. The solvents used in the present study did not affect currents through Slick or Slack channels. All the blockers were tested at the highest reasonable concentration. The tested concentrations were at least one order of magnitude higher than the effective concentration previously described for each blocker on specific potassium channels.
The effect of the antiarrhythmic compound clofilium was tested at 0.01, 0.1, 10, and 100 μM. Clofilium was directly dissolved in Kulori medium prior to application to oocytes expressing Slick or Slack channels. Currents were measured before and after application of treatment. Current values from endogenous channels of noninjected oocytes were subtracted from currents measured for oocytes expressing Slick and Slack channels. The expression of normalized current as a function of drug concentration allowed fitting the dose-response curves with a Hill equation, using a nonlinear regression analysis on GraphPad Prism 5® (Windows GraphPad software, San Diego, CA). The data are shown as the mean and the standard error. A higher concentration of clofilium was also tested, but was not included in the dose-response curves due to the possibility of nonspecific effects on the targeted channels.
Data acquisition and analysis were performed using the following packages: pClamp® (Molecular Devices, San Jose, CA), GraphPad Prism 5®, and Microsoft Excel®. Sequences of Slick, Slack, and hERG channels were analyzed using the CLC Main Workbench 6.5 (Cambridge, MA). Sequences were aligned at the pore region and S6 transmembrane segment in Slick, Slack, and hERG channels. Conservation of residues was evidenced by sequence logos.
Results
Effect of clofilium on Slick and Slack channels
The effect of clofilium on Slick and Slack potassium channels expressed in X. laevis oocytes was analyzed using a two-electrode voltage clamp. shows Slick and Slack currents measured before (black) and after (red) application of 100 μM clofilium. As evident from the current traces, clofilium was able to reduce the activity of Slack channels with a stronger potency compared with its effect on Slick channels. The blocking effect of clofilium on Slick and Slack channels was readily reversible upon washing with the culture medium (data not shown). In the experiments shown in , we examined the effect of clofilium on channel kinetics, and it can be seen that clofilium slows the activation kinetics of both channels.
Figure 1 Effect of clofilium on Slick and Slack potassium channels. Upper panels show current traces of representative Xenopus laevis oocytes expressing either Slick (A) or Slack (B) channels before (black) and after (red) treatment with clofilium 100 μM. Currents were stimulated by a pulse protocol with 500 msec depolarizations from −80 to +80 mV from a holding potential of −80 mV for 3 seconds. Activation kinetics of Slick (C) and Slack (D) in representative oocytes are shown in the lower panels.
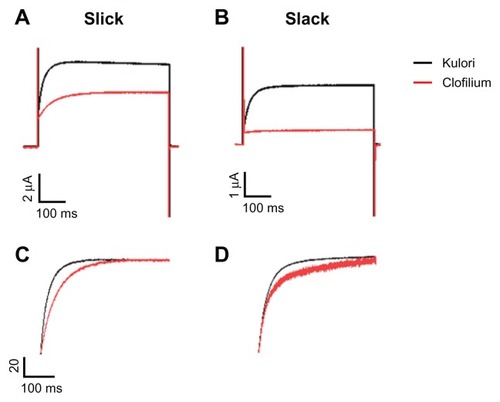
To determine the affinity of clofilium, we plotted currents through Slick and Slack channels upon application of increasing concentrations of clofilium (). Concentrations of clofilium up to 10 μM did not exert any significant blocking effect in either Slick or Slack channels. However, at a concentration of 100 μM, clofilium was able to inhibit currents through Slick and Slack channels to 75% ± 1% and 51% ± 3% of control values, respectively. As a consequence, the effect of this compound is more potent on Slack channels than on Slick channels,indicating that Slack channels are probably significantly more sensitive than Slick channels to clofilium.
Figure 2 Dose response of Slack and Slick channels upon treatment with clofilium. Oocytes expressing Slick (A) or Slack (B) channels were exposed to different concentrations of clofilium (0.01–100 μM). K+ currents were measured at the end of depolarizing steps of a pulse protocol (500 msec depolarizations from −80 to +80 mV, holding potential −80 mV for 3 seconds). Inhibition curves upon application of increasing doses of clofilium for Slick (A) and Slack (B) expressing oocytes have IC50 values shown together.
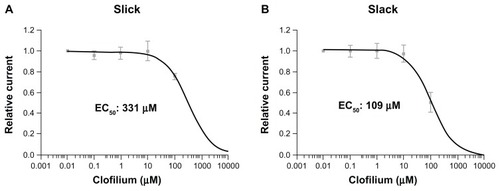
Screening of potassium channel blockers for Slick and Slack
Since knowledge of the pharmacology of Slick and Slack channels is sparse, a set of well known blockers, covering most potassium channels in the genome, was tested to determine their effect on Slick and Slack channels. A total of 38 compounds, including toxins and small peptides, were used for this purpose. Some of the potassium channel blockers tested have been previously shown to have a potent effect on other related potassium channels, eg, iberitoxin and charybdotoxin in Slo1 channels,Citation13,Citation14 and apamin and scyllatoxin in SK channels. Furthermore, blockers of Kir channels and Kv channels, such as tertiapin, agitoxins, dendrotoxins, and margatoxin, which are known to block these channels at nanomolar doses, were also tested. None of the tested compounds was able to significantly modulate the activity of Slick or Slack channels to a significant extent ().
Figure 3 Effect of different K+ channel blockers on Slick and Slack channels.
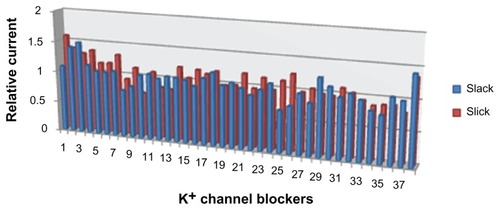
Discussion
The use of channel blockers or openers are important tools in order to be able to understand better the roles Slick and Slack channels may play under physiological and pathophysiological conditions. The present study provides evidence for the effect of clofilium on Slick and Slack potassium channels. We observed that both channels are inhibited after treatment with clofilium, and the effect of this compound seems to be more potent on Slack than Slick channels. Our experiments also show that clofilium affects channel kinetics, resulting in slower activation of both Slick and Slack channels as compared with controls. The slower gating behavior probably underlies the progressive blocking action of clofilium of open channels and a slower onset of channel inhibition, as has been suggested for hERG and hEAG channels.Citation15
Clofilium has been shown to block a number of potassium channels with different potency, including hERG (IC50 2.5 nM), hEAG (IC50 255 nM),Citation15 Kv1.5 (IC50 840 nM),Citation16 and KCNQ1-KCNE1 (IC50 about 100 μM),Citation17,Citation18 and recently another member of the high-conductance potassium channel family, ie, the Slo3 channel.Citation19 Within the Slo gene family, clofilium blocks Slo3 channels in micromolar concentrations (IC50 50 μM),Citation19 whereas it does not seem to affect Slo1 channels.Citation20 In our hands, clofilium 100 μM blocked 50% of currents through Slack channels, whereas the same concentration blocked Slick channels to 70% of control levels. Consequently, these members of the Slo2 gene family, despite being highly related in structure and localization, have different sensitivity for this potent antiarrhythmic agent. Given that relatively high doses of clofilium were needed to block these channels, clofilium should not be regarded as a specific blocker of Slick and Slack channels. However, it should be borne in mind that during treatment with this compound, not only hERG and hEAG channels are potently affected, but other channels such as KCNQ1/KCNE1, Slick, and Slack could also be affected. It is noteworthy that clofilium blockade in X. laevis oocytes needs doses at least 10 times higher to have a visible effect on hERG channels compared with mammalian cells.Citation15 In addition, Gessner and HeinemannCitation15 have suggested that the effective concentration of clofilium in cytoplasm is several orders of magnitude lower than that outside the cell. Furthermore, Malayev et alCitation16 have reported that clofilium block on Kv1.5 channels is more than two-fold stronger when applied from the intracellular side. Consequently, it is possible that lower doses of clofilium could exert a stronger effect on Slick and Slack channels expressed in mammalian cell lines and/or when applied as inside-out patches.
It has been shown that certain residues in the pore helix and the S6 transmembrane segment of hERG channels are involved in the interaction with clofilium.Citation21 In an attempt to suggest possible interaction sites of clofilium with Slick and Slack channels, we performed a sequence alignment between these channels and hERG. The alignment revealed residues which could be involved in the interaction of clofilium with Slick and Slack channels. The alignment showed a highly conserved valine at the selectivity filter, together with a residue in the S6 segment, namely phenylalanine, at position F274 for Slick and F327 for Slack, which could probably be involved in the interaction of clofilium with these channels, given that these residues have been shown to bind clofilium in hERG channels (). Other residues of hERG channels have also been related to clofilium interaction, ie, threonine T623, serine S624, and tyrosine Y652. However, these residues are not highly conserved between Slick, Slack, and hERG channels (), and it could be speculated that this is the reason for the difference in clofilium sensitivity.
Figure 4 Potential residues involved in the interaction of clofilium with Slick and Slack channels and sequence alignment for the pore helix through the S6 transmembrane segment of Slick, Slack, and hERG channels.
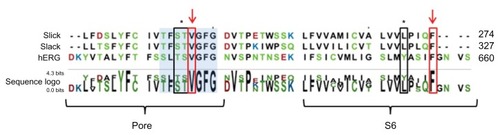
In a search for pharmacological agents affecting Slick and Slack channels, a screen of a series of commonly used potassium channel blockers was performed. Among the 38 compounds used, between toxins and small peptides, none of them was able to modulate the activity of Slick or Slack channels to a significant extent. This is the first report that provides evidence from a basic screen of different potassium channel blockers on Slick and Slack channels. Like every first screen, it constitutes a starting point towards unveiling the pharmacological characteristics of these channels. Further research is needed to identify potent and, in the best case, specific blockers of Slick and Slack channels.
Conclusion
The present study aimed to elucidate the pharmacological characteristics of Slick and Slack high-conductance potassium channels. We were able to identify clofilium as a modulator of the activities of these channels. We suggest that the potent effect of clofilium as an antiarrhythmic could be explained by its action on different potassium channels, in which Slick and Slack may be implicated. We have also presented evidence of other potassium channel blockers not having a significant effect on Slick or Slack channels. It will be interesting in future experiments to tailor small peptides according to the structure of the channels to act as specific blockers for either of these channels.
Acknowledgments
Ms Z Rasmussen is thanked for her expert technical assistance. This work was supported by grants from The Danish Medical Research Council, The Carlsberg Foundation, The Novo Nordic Foundation, The Lundbeck Foundation (Lucens), and The Fougner-Hartmann Foundation.
Disclosure
The authors declare no conflicts of interest, financial or otherwise, in this work.
References
- ShiehCCCoghlanMSullivanJPPotassium channels: molecular defects, diseases, and therapeutic opportunitiesPharmacol Rev200052455759411121510
- WulffHCastleNAPardoLAVoltage-gated potassium channels as therapeutic targetsNat Rev Drug Discov2009812982100119949402
- JenkinsonDHPotassium channels – multiplicity and challengesBr J Pharmacol2006147Suppl 1S63S7116402122
- YuanASantiCMWeiAThe sodium-activated potassium channel is encoded by a member of the Slo gene familyNeuron20033776577312628167
- BhattacharjeeAJoinerWJWuMSlick (Slo2.1), a rapidly-gating sodium-activated potassium channel inhibited by ATPJ Neurosci20032337116811169114684870
- BhattacharjeeAGanLKaczmarekLKLocalization of the Slack potassium channel in the rat central nervous systemJ Comp Neurol2002454324125412442315
- BhattacharjeeAvon HehnCAMeiXLocalization of the Na+-activated K+ channel Slick in the rat central nervous systemJ Comp Neurol20054841809215717307
- ZhangLSukharevaMBarkerJLDirect binding of estradiol enhances Slack (sequence like a calcium-activated potassium channel) channels’ activityNeuroscience2005131227528215708472
- YangBGribkoffVKPanJPharmacological activation and inhibition of Slack (Slo2.2) channelsNeuropharmacology200651489690616876206
- BitonBSethuramanujamSPicchioneKThe antipsychotic drug loxapine is an opener of the Na+-activated potassium channel Slack (Slo2.2)J Pharmacol Exp Ther2011340370671522171093
- DaiLGargVSanguinettiMCActivation of Slo2.1 channels by niflumic acidJ Gen Physiol2010135327529520176855
- GrunnetMMacAulayNJorgensenNKRegulation of cloned, Ca2+-activated K+ channels by cell volume changesPflugers Arch20024441–216717711976929
- Gimenez-GallegoGNaviaMAReubenJPPurification, sequence, and model structure of charybdotoxin, a potent selective inhibitor of calcium-activated potassium channelsProc Natl Acad Sci U S A19888510332933332453055
- GalvezAGimenez-GallegoGReubenJPPurification and characterization of a unique, potent, peptidyl probe for the high conductance calcium-activated potassium channel from venom of the scorpion Buthus tamulusJ Biol Chem19902651911083110901694175
- GessnerGHeinemannSHInhibition of hEAG1 and hERG1 potassium channels by clofilium and its tertiary analogue LY97241Br J Pharmacol2003138116117112522086
- MalayevAANelsonDJPhilipsonLHMechanism of clofilium block of the human Kv1.5 delayed rectifier potassium channelMol Pharmacol19954711982057838129
- HonoreEAttaliBRomeyGCloning, expression, pharmacology and regulation of a delayed rectifier K+ channel in mouse heartEMBO J19911010280528111655403
- YangWPLevesquePCLittleWAKvLQT1, a voltage-gated potassium channel responsible for human cardiac arrhythmiasProc Natl Acad Sci U S A1997948401740219108097
- ZengXHYangCKimSTDeletion of the Slo3 gene abolishes alkalization-activated K+ current in mouse spermatozoaProc Natl Acad Sci U S A2011108145879588421427226
- Fernández-FernándezJMNoblesMCurridAMaxi K+ channel mediates regulatory volume decrease response in a human bronchial epithelial cell lineAm J Physiol Cell Physiol20022836C1705C171412388065
- PerryMde GrootMJHelliwellRStructural determinants of HERG channel block by clofilium and ibutilideMol Pharmacol200466224024915266014