Abstract
Biodegradable polymeric materials are ideal carrier systems for biomedical applications. Features like controlled and sustained delivery, improved drug pharmacokinetics, reduced side effects and safe degradation make the use of these materials very attractive in a lot of medical fields, with dermatology included. A number of studies have shown that particle-based formulations can improve the skin penetration of topically applied drugs. However, for a successful translation of these promising results into a clinical application, a more rational approach is needed to take into account the different properties of diseased skin and the fate of these polymeric materials after topical application. In fact, each pathological skin condition poses different challenges and the way diseased skin interacts with polymeric carriers might be markedly different to that of healthy skin. In most inflammatory skin conditions, the skin’s barrier is impaired and the local immune system is activated. A better understanding of such mechanisms has the potential to improve the efficacy of carrier-based dermatotherapy. Such knowledge would allow the informed choice of the type of polymeric carrier depending on the skin condition to be treated, the type of drug to be loaded, and the desired release kinetics. Furthermore, a better control of polymer degradation and release properties in accordance with the skin environment would improve the safety and the selectivity of drug release. This review aims at summarizing the current knowledge on how polymeric delivery systems interact with healthy and diseased skin, giving an overview of the challenges that different pathological skin conditions pose to the development of safer and more specific dermatotherapies.
Introduction
Nanotechnology offers a vast possibility to improve delivery, bioavailability, and targeting of drugs and cosmetics to skin. Considering the good accessibility of skin, the possibility to treat skin disorders by topical therapies is very appealing. This method of administration increases patient compliance, reduces side effects, avoids the hepatic first pass metabolism, hereby enhancing the amount of drug at the site of action, and could help to reduce the dose required to obtain therapeutic effects.Citation1 Supramolecular drug delivery systems (DDS) applied topically to diseased skin should accumulate and release the drug at the site of action. In diseased skin, because of impaired stratum corneum (SC) and/or activated immune system, the penetration of macromolecules and particulate materials is often altered with respect to healthy skin. Thus, to avoid possible side effects, the use and development of biodegradable and biocompatible nanomaterials is necessary. In medicine, the term biodegradable describes materials which breakdown into molecules that are nontoxic and can be metabolized or eliminated by the body. The term biocompatible is often used to describe nondegradable materials that are eliminated by renal clearance and have been shown to be safe in in vivo studies. In this review, focus is given to biodegradable polymer materials that have been developed and tested as delivery agents for dermatotherapies. Some of the most investigated biocompatible polymeric materials will be also discussed. We aim to offer an overview of the different types of DDS and the key processes occurring after their topical administration. In particular, the different pathophysiological conditions occurring in skin diseases have been outlined in order to emphasize that each different pathological condition brings specific challenges that have to be taken into account when developing and investigating new carrier systems.
Types of biodegradable polymeric DDS
The first delivery systems that were investigated and marketed for dermatological and cosmetic applications were liposomes.Citation2 Thereafter, solid lipid nanoparticles (SLN) and nanostructured lipid carriers (NLC) were developed, which are more stable and have better skin penetration.Citation3 In the past few years, an increasing number of polymeric carriers have been investigated for topical drug delivery.Citation4 Biodegradable polymers offer certain advantages over lipid-based carriers. They can be stored over months, both hydrophobic and hydrophilic drugs can be encapsulated or adsorbed, they can be functionalized, and most importantly their physicochemical properties can be adjusted in order to achieve a controlled release of the encapsulated drug. Several different types of polymer-based DDS have been tested for drug delivery. Polymeric fibers are used in tissue engineering as scaffolds for tissue reconstruction and wound healing.Citation5,Citation6 Poly(ɛ-caprolactone) (PCL), collagen, and hyaluronic acid are some of the polymers used to prepare biological matrixes. They can be loaded with growth or angiogenic factors to favor tissue regrowth or antimicrobials to avoid wound infection.Citation7,Citation8 Hydrogels consist of hydrophilic molecules, called gelling agents, which are chemically or physically cross-linked and form a three-dimensional network with the ability to absorb high amounts of water.Citation9 Hydrogels can be made of natural polymers, like alginate, chitosan, dextran, or of synthetic material like poly(ethylene glycol) (PEG), poly(lactic-co-glycolic acid) (PLGA), or a combination of natural and synthetic polymers.Citation10 They can be loaded with a variety of drugs, mainly hydrophilic ones, and have been largely investigated for drug delivery in medicine. Organogels are gelling agent networks able to incorporate oil or organic solvents. Polymers like poly(ethylene) and PEG, as well as copolymers of methacrylic acidCitation11 and methyl methacrylate (MMA) are commonly used. These polymers are nonbiodegradable but are considered biocompatible;Citation12 because of their lipophilic nature they can incorporate hydrophobic drugs and have a better skin penetration.Citation13 Hydrogels have also been used to disperse nanocarriers enhancing their distribution on the skin surface and were applied as a delivery system for wound healing.Citation14,Citation15 Nanogels are nanoscaled organogels and hydrogels. Using appropriate linkers it is possible to make nanogels stimulus-responsive (eg, temperature, pH, light).Citation16,Citation17 Thus, the release of encapsulated drugs can be controlled by exploiting the unique parameters of diseased skin or even using an external trigger. The small size and the deformability of nanogels favor their accumulation in the SC and in the hair follicle canal. Polymeric spheres are made of several polymer chains which in water spontaneously “roll-up” to form spherical particles. Both natural and synthetic polymers have been used. Natural polymers are derived from animals as well as plants and include polysaccharides, such as cellulose, chitin, starch, alginate, galactan, hyaluronic acid, dextran, and gellan,Citation18 or protein-based carriers like albumin, gelatine, collagen, milk and silk proteins, soy proteins, and lectins.Citation19,Citation20 Polydepsipeptides are synthetic peptide polymers which are degraded into biocompatible amino acids.Citation21 Their composition can be adjusted to achieve an optimal complexation of the loaded drug and to control their physicochemical properties such as hydrophobicity/hydrophilicity. The most studied synthetic biodegradable polymers used to prepare micro and submicron spheres for drug delivery are poly(lactic acid) (PLA),Citation22 PLGA and PCL.Citation23,Citation24 PLA particles are prepared from renewable sources. They allow for an easy-scalable and low cost production and are degraded to lactic acid, a resorbable and non-toxic monomer.Citation25 Polymeric micelles are auto-assembled micelles made of amphiphilic macromolecules such as di- or tri-block copolymers. Examples of biodegradable micelles are those based on PEG, PLA, PCL, or chitosan, whereas poly(amidoamine) (PAMAM) micelles are considered biocompatible. The advantages of such particles are their small size, the solubilization of hydrophobic drugs, and the possibility to functionalize their surface with targeting units.Citation26 Dendrimers and dendritic polymers consist as macromolecules with a branched structure in which different types of drugs can be intercalated or covalently bound. Dendritic core-multishell nanocarriers have amphiphilic architecture consisting of a hydrophobic inner shell and a hydrophilic outer shell allowing the encapsulation of lipophilic drugs and the control of surface polarity. Polyester dendrimers made of glycerol, succinic acid, phenylalanine, and lactic acid are promising biodegradable carriers.Citation27
Polymeric carriers are available in different sizes ranging from nanometer to submicron and micrometer dimension. In general, the organic nature of the polymer and the three-dimensional conformation confer them flexibility and deformability, which are important requisites for penetration across tissue barriers.
Key processes after topical application
Interaction with the stratum corneum
The SC is made of protein-rich cells embedded in a lipid extracellular matrix. This arrangement has been referred to as the bricks and mortar model.Citation28 In addition, corneocytes are anchored together by means of intercellular proteic structures called corneodesmosomes.Citation29 Depending on their composition, size, and shape, macromolecules and supramolecular carriers interact differently with this very complex barrier. It has been postulated that lipid-based particles have the tendency to merge with the skin lipid layers, whereas polymeric particles, being more hydrophilic, might have different interactions with the extracellular lipids of the SC.Citation30 However, even if the components of polymeric carriers do not fuse with the lipids of the SC, the interaction with the SC may change their three-dimensional conformation leading to adsorption, destabilization, or agglomeration. Furthermore, the SC is covered with sebum, rich in lipids and proteins, which can interact with the applied polymeric materials destabilizing them or changing the way they interact with other skin components. A number of different commensal bacteria strains reside on the skin surface, in skin furrows and appendages and might interact with applied materials. The pH on the surface of the SC is 4.2–5.6.Citation31 Such an acidic pH can influence the colloidal stability of particles. The acidic pH increases the rate of hydrolysis and the activity of pH-sensitive enzymes, which in turn might accelerate the degradation of polymeric particles.Citation32 Finally, the SC is a dynamically evolving structure and, near to a more compact region (stratum compactum), a looser part can be distinguished due to desquamation processes (stratum disjunctum; ). Thus, substances accumulated in the stratum disjunctum are rapidly eliminated by the shedding of the outermost layers, whereas the SC can function as a reservoir for those particulate materials penetrating deeper. Once released, the drugs can diffuse across the SC to the viable skin layers. Three penetration pathways have been described for topically applied substances: the intercellular, the transcellular, and the transfollicular route.Citation33–Citation35 In general, the penetration pathway and rate of diffusion of released drugs depend on their lipophilicity/hydrophilicity, their molecular weight, and the carrier type.
Figure 1 Human terminal hair follicle. (A) Light microscopy image of a longitudinal section showing the hair follicle infundibulum and the hair shaft. The infundibulum is filled with cell debris and sebum. Insets (b–d) are regions of the hair follicle that were observed by means of transmission electron microscopy (B, C and D respectively), using an ultrafine section prepared from the same sample block. (B) The SC in the apical part of the hair follicle orifice consists of several corneocytes layers. Bacteria colonies are visible as well. (C) The SC in the hair follicle infundibulum is thinner than the SC in the upper part of the hair follicle orifice and in the inter-follicular epidermis. (D) Image of the hair shaft and the inner root sheath.
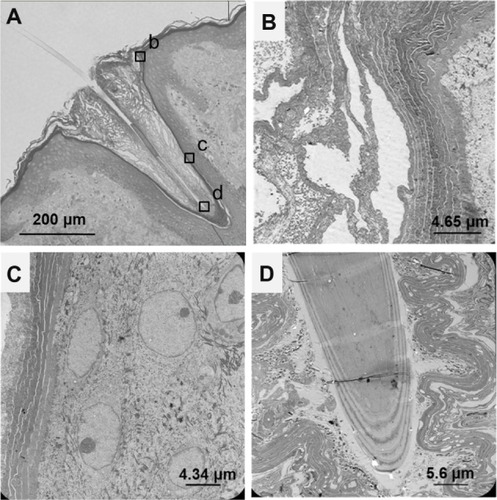
Hair follicle pathway
The hair follicle canal of the pilosebaceous unit represents a reservoir where micro-, submicron-, and nano-sized material can penetrate and accumulate ().
It took a while before the hair follicle was taken into consideration as a possible portal to skin penetration. In fact, hair follicle orifices represent only 0.1% of the skin surfaceCitation36 and it was assumed that the sebum impedes the penetration of material in the hair follicle canal. Only in the last decade a number of studies demonstrated the importance of the transfollicular pathway, especially for the preferential penetration of particulate materials. Particles that accumulate in the hair follicle are protected from SC turnover and persist for days before they are cleared.Citation37 The size of the hair follicle orifices ranges between 172±70 μm in terminal hair follicles and 86±37 μm in vellus hair follicles,Citation38 and the interaction surface of hair follicle infundibulum was calculated to be approximately 0.68 and 0.068 cm2 for empty or full follicles, respectively.Citation39 Consequently, the hair follicle infundibulum represents not only a reservoir but also a considerable surface area where interaction between accumulated material and skin structures can occur. The hair follicle is a shunt for a more rapid penetration of substances. When the hair follicle orifices were selectively closed, the applied substances reached the same blood values in volunteers with open hair follicles.Citation40,Citation41 This might be due to the fact that the SC barrier in the deep infundibulum is thinner () and molecules can diffuse more rapidly here than on the skin surface where the SC consists of several more layers (). In addition, those carriers accumulated in the hair follicle canal release the loaded drug, thereby generating a high local concentration of the drug which is a driving force for its diffusion to the viable skin. The removal of the hair follicle content (sebum, cells debris, and eventually bacteria) favors the penetration of topically applied particulate materials.Citation42
Translocation across the stratum corneum
Even if the debate on the translocation of particulate material across the SC is not yet closed, in general, it is believed that only lipophilic molecules with a molecular weight below 500 Dalton can pass the skin barrier, whereas the penetration of hydrophilic molecules, macromolecules, and nanoparticles is significantly impaired.Citation43–Citation45 Liposomes, solid lipid nanoparticles, and polymeric DDS were shown to enhance the skin penetration of loaded substances but there is no evidence for the translocation of entire particles across the intact SC of healthy skin. On the contrary, it seems that components of the lipid-based particles dissolve into the lipids of the SC enhancing its permeability.Citation46,Citation47 In the case of polymer-based carriers, data on the penetration across the SC are poor due to the fact that most of the studies cited in this article detected the released fluorochrome (or drug), but only a few studies attempted to find out the fate of the carrier after topical application. Interestingly, there is one report claiming that 200–250 nm poly(N-isopropylacrylamide) (NIPAM) and poly(N-isopropylacrylamide-co-acrylic acid) (NIPAM-co-AAc) nanogels penetrated across epidermal sheets of pig ears. Indeed, these biocompatible carriers were detectable by means of transmission electron microscopy (TEM) in the acceptor medium of the Franz diffusion cell.Citation48 However, these results apply to epidermal sheets isolated by heating at 60°C (which might damage the lipid matrix and corneodesmosomes) and not to full thickness skin. This underlines how challenging it is to visualize and elucidate the interaction between carriers and the skin barrier at the subcellular level.
Carriers penetration across disrupted skin barriers
When developing DDS for skin disorders, the different features of diseased skin have to be considered. Whereas in healthy skin particulate carriers penetrate in the SC and in the hair follicle canals releasing there the loaded drugs (), in unhealthy skin, where the integrity of the SC is altered, carrier systems might cross the SC barrier and enter the epidermis and dermis (). Several methods have been developed to bypass the SC, for example by disrupting it with adhesive tapes and abrasion,Citation49 altering it with permeabilizing agents,Citation50,Citation51 or using microneedles.Citation52 Most of these procedures were tested for transdermal drug delivery or transcutaneous vaccination strategies. Only a small number of studies investigated the penetration of particles across diseased animal or human skin.Citation53,Citation54 In fact, most animal studies report the diffusion of the released drugs and their efficacy but not on the fate of the used carrier systems.Citation54–Citation56 Most authors found significantly higher drug permeability in inflamed skin than in healthy skin.Citation55,Citation56 These results are explained with the swelling of the tissue and the increased intercellular fluid in the epidermis which might alter the structure of tight junctions. However no direct evidence for nanoparticle translocation was provided.
Figure 2 Skin penetration of polymeric carriers and drug release in (A) healthy skin and (B) hypothesis of polymer particle behavior in diseased skin.
Abbreviation: SC, stratum corneum.
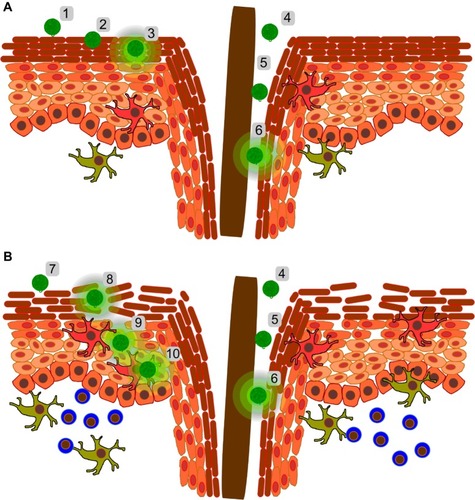
In general, those studies using ultraviolet Citation57 irradiation or mechanical methods to disrupt the SC and activate the immune system showed that topically applied nano-materials can be detected in the viable skin layers.Citation58–Citation60 Once particles cross the SC, they might also reach the subcutaneous lymph nodes after being taken up by skin antigen presenting cells or by passive transport following the lymph drainage.Citation61 All these findings underline the need for further knowledge on the interactions and fate of the carrier system after topical application on diseased skin.
Most skin diseases are associated with different degrees of SC disruption.Citation29 Disease-specific differences, however, can have a tremendous impact on how carrier systems interact with skin. In atopic dermatitis and contact eczema, defects in expression of tight junction components and filaggrin are responsible for the dysfunction of the SC resulting in a higher susceptibility to external allergens and opportunistic infections.Citation62 Thus, it is reasonable to think that particulate materials have easy access. Nanoparticles which can penetrate the epidermis and dermis can interact with immune system cells and be taken up by phagocytic cells. Because atopic and contact dermatitis are associated with altered immune responses, certain nanoparticles might exacerbate the symptoms of the pathology, as was found for polystyrene nanoparticles.Citation63 In psoriasis, keratinocyte hyper-proliferation and abnormal SC stacking leads to epidermal thickening and the formation of scales. The cytokine milieu induces vasodilatation and cell infiltration. Even if the SC is thicker, tight junction proteins are altered leading to increased skin permeability to topically applied drugs.Citation64 Nevertheless, this does not apply to all types of molecules and nanoparticles. In fact, Prow et al found that penetration of ZnO2 nanoparticles was not increased in psoriatic skin compared to a non-lesional skin region of the same patient.Citation65 However, no data are available with regard to the penetration of more flexible particles like polymer-based nanocarriers. Skin barrier properties are also altered during skin infection. For example, dermatophytes secrete proteolytic enzymes which degrade the SC allowing them to invade and disseminate through itCitation66 and at the same time altering skin permeability. In general, during bacterial and fungal infections, the SC is more permeable and the skin immune system is activated with the presence of cell infiltrates and vasodilatation similar to the situation found in eczema. Nevertheless, depending on the type of pathogen and the stage of disease, different degrees of inflammation, skin dryness, and desquamation are present. The skin barrier is compromised in aged skin as well.Citation67 It has been shown that elderly skin is dry and scaly. The reason for these defects is related to lower lipid levels in lamellar bodiesCitation68 and a decrease in epidermal filaggrin.Citation69 In addition, aged skin manifests epidermal and dermal atrophy, flattening of the epidermal rete ridges, as well as reduced numbers of fibroblasts and mast cells.Citation67
On the other hand, there are other skin conditions that are characterized by a less permeable skin barrier. In hypertrophic scars, for example, even if the amount of hydrophobic ceramide is decreased, the SC is irregular and thicker. Another example is acne vulgaris, which is characterized by a hyper-proliferation of keratinocytes in the hair follicle at the level of the sebaceous gland duct and an increased production of sebum which result in a strong barrier to the penetration of hydrophilic molecules and nanoparticles.
Cellular uptake
Once a carrier system or a drug has successfully crossed the skin barrier and has reached the viable epidermis layers, it can diffuse across the extracellular space and/or be taken up by epidermis and dermis cells (). Whereas small amphiphilic drugs can diffuse across the cell membrane, macromolecules like proteins, nucleic acids, as well as nano-and submicron-material can be internalized by cells only via the energy-dependent mechanism of endocytosis.Citation70,Citation71 In general, only certain types of cells can internalize particulate material with size above 200 nm.Citation70 For example, when dendritic cells were co-cultured with T-cells and incubated with fluorescently labeled bacteria and polystyrene particles (200 nm), we found that only dendritic cells internalized both particles (unpublished data, ).
Figure 3 Confocal fluorescence images showing the uptake of Escherichia coli particles and polystyrene particles (200 nm) by dendritic cells (unpublished data). Dendritic cells derived from peripheral blood mononuclear cells were co-cultured with T-cells and incubated with inactivated E. coli particles labeled with a pH sensitive dye that becomes fluorescent in an acidic environment (A; red) and FITC-loaded 200 nm polystyrene particles (B, green). The overlay image (C) shows that the bigger cells (ie, dendritic cells), but not T-cells, had internalized particles. Both E. coli and polystyrene particles were co-localized in intracellular acidic compartments.
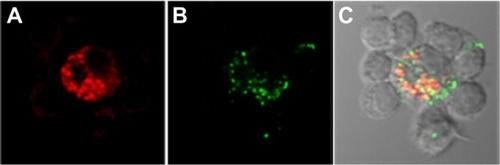
Langerhans cells in the epidermis are cells specialized in taking up particulate materials penetrating across a disrupted skin barrier. Also, keratinocytes, which are the most represented cells in the epidermis (90%), can internalize penetrated materials. Nevertheless, in vitro experiments with freshly isolated epidermis cells have shown that, depending on the particle size, keratinocytes are less prone than Langerhans cells to take up particles.Citation59 In the dermis, penetrated material comes in contact with cells like fibroblasts, mast cells, and T-cells. However, macrophages and dendritic cells are most likely to internalize them. Once internalized, the carrier systems can undergo degradation in the acid and hydrolytic environment of lysosomes and release the loaded drug.Citation72 Hydrolysis and enzymatic degradation may be exploited as a mechanism for drug release, though the same reactions may be deleterious for certain loaded molecules such as proteins or nucleic acids.Citation23 To avoid degradation, endosomal escape has to be achieved.Citation73 On the other hand, for vaccination purposes, the uptake of antigen-loaded carriers by antigen presenting cells and their intracellular degradation is advantageous, being the steps necessary for major histocompatibility complex (MHC)-I and MHC-II presentation.Citation22
Polymer degradation and drug release
Once applied on the skin surface, polymeric biomaterials may be degraded by chemical and/or enzymatic reactions depending on the nature of the polymer. Hydrolytic enzymes, including proteases, esterases, glycosidases, and phosphatases, can catalyze the water mediated cleavage of different chemical bonds. The degradation of polymer particles is often a complex process and the release of the loaded drug exhibits often non-linear kinetics. The degradation of the polymer may interfere with the release of the drug, inhibiting or accelerating it, or might degrade the loaded drugs.Citation74 Ideally, a biodegradable polymer should decompose into non-toxic products and should degrade in a controlled manner. In this way, not only the safety but also the selectivity and efficacy of the drug are enhanced. For example, hydrolysis of the drug-carrier bond can be controlled by introducing linkers cleavable by enzymes that are localized predominantly in the diseased tissue or target cell.Citation75 These processes are influenced by skin parameters like temperature, pH, and protein concentration.Citation76,Citation77 Also, it is possible to include degrading enzymes in the polymer matrix.Citation74 The type of polymer, the nature of interactions between drug and polymer, and the affinity of the drug to skin components determine the time and the place where the carrier is degraded and the drug released. Polymers can be readily hydrolyzed after penetration in the SC, upon penetration in the epidermis and dermis, or only after cellular uptake ().
The release of a loaded drug may also take place by other processes like conformational changes of the particles (destabilization, swelling, or shrinkage). Upon topical application, drugs adsorbed on the carrier surface may be released by affinity-driven diffusion or by exchange with skin components like proteins. The physicochemical properties of the carriers seem to play an important role in drug release and diffusion.Citation78,Citation79
Challenges for carrier-based dermatotherapies
Whereas transdermal delivery implies the transport of actives across the whole healthy skin to reach the systemic compartment or regions beneath the skin (eg, skeletal or muscular tissues), the treatment of skin pathological conditions implies the local delivery of actives to skin regions with altered parameters. The knowledge on the pathophysiology and mechanisms underlying a number of skin conditions has enormously increased in the past years, showing that diseased skin is morphologically, physiologically, and biologically different from healthy skin.Citation80 Thus, different strategies can be developed taking into consideration the special conditions which characterize affected skin and exploiting them to achieve a more selective delivery of the drug.
Skin inflammatory conditions
The treatment of atopic dermatitis, contact eczema, and psoriasis is achieved using anti-inflammatory drugs (steroidal and non-steroidal) or immunomodulatory and immunosuppressive drugs (eg, tacrolimus). These actives are administered topically or, in severe cases, orally or intravenously. One of the main problems of topical treatment with glucocorticoids is to reach effective concentrations of the anti-inflammatory drug in the epidermis avoiding drug localization in the dermis or its rapid diffusion to the systemic compartment. Glucocorticoid localization in the dermis leads to induction of metalloproteases and degradation of connective tissue, resulting in thinning of skin and skin atrophy. On the other hand, the rapid clearance of the drug from skin to the systemic compartment is responsible for reduction of drug efficacy. Nanocarriers offer the possibility to improve the efficacy and safety of corticosteroids by creating depots in the SC, hair follicle canal, and epidermis as well as local release of the drug in a sustained manner.Citation81,Citation82 In the case of macrolide drugs, like tacrolimus, there is a need for a better penetration across the SC especially in the case of psoriasis where keratinocytes over-proliferation results in plaque formation. In fact, the high molecular weight and the hydrophilic nature of the molecule impede its diffusion into the skin. The use of carrier systems that can increase its penetration across the SC and thereby protect it from degradation would increase the efficacy of this topical treatment. Furthermore, a better and more selective delivery of the drug to the dendritic and T-cell infiltrates in inflamed skin would reduce the side effects and increase the therapeutic activity of the drug. It is now recognized that skin dendritic cell subsets are involved in chronic inflammatory skin conditions and in the initiation of skin allergic reaction. Therefore, particle-based targeting of dendritic cells and their modulation seems to be a promising strategy against such skin conditions where an overreaction of skin immune cells is involved.Citation83
The skin penetration profile of small hydrophobic molecules, like non-steroidal anti-inflammatory drugs, can also be improved by encapsulation in particles. The delivery of flufenamic acid by PLGA particles resulted in a more prolonged and localized drug release in the epidermis, whereas surface modified PLGA and chitosan nanoparticles formulated in a gelling agent were successfully used to deliver spantide II and ketoprofen to allergic contact dermatitis and psoriatic plaque-like skin models.Citation24,Citation54 Polymer particles, because of their ability to protect encapsulated drugs, also have the potential to optimize the topical delivery of nucleic acid molecules, such as small interfering ribonucleic acid (siRNA) for the regulation of cytokines’ expression and control of inflammatory skin diseases.Citation84
Skin infections
The therapy of skin infections can also profit from a more selective and effective delivery of the antimicrobial drug. Especially in the case of pathogens colonizing the lower infundibulum, the use of nanocarriers that accumulate in the hair follicle canal and thus the achievement of high concentrations of antimicrobials can decisively improve the outcome of a topical therapy. Another challenge is the reduction of unwanted toxicity of antimicrobials toward skin cells. In the case of antibiotic-resistant strains, besides the development of new generations of antibiotics, new therapeutic approaches include the application of DDS for the slow but sustained release of toxic substances such as metal ions or nitric oxide, thus limiting their side effects. For example, hydrogel (polyethylene glycol, chitosan)-glass composites have been tested for the sustained release of nitric oxide, whereas silver nanoparticles are used as a source of silver ions.Citation85,Citation86 Biodegradable gelatin fibers loaded with antimicrobial silver nanoparticles were also tested as dressing materials in the treatment of wounds and chronic wounds.Citation87
Hair follicle associated disorders
The targeting of drugs to the pilosebaceous unit by means of nano- and submicron carriers can be exploited to treat hair follicle-associated inflammation, hair growth, and sebaceous gland disorders like alopecia and acne, respectively. For example, to treat alopecia androgenetica the drug should be targeted to the hair bulb where the hair shaft is generated. For this purpose, minoxidil was formulated on 40 nm poly(ɛ-caprolactone)-block-poly(ethyleneglycol) carriers with positive results.Citation88 For the treatment of acne vulgaris, the drug should be targeted to the infundibulum at the level of the sebaceous gland. Thus, microspheres accumulating preferentially in the hair follicle infundibulum would be the carrier of choice.Citation89 The release of the loaded drug can take place due to diffusion gradients or can be triggered by the special environment within the hair follicle canal.Citation90 We have shown that 228 nm PLA particles accumulated in the hair follicle infundibulum realizing a sustained release of the loaded lipophilic dye nile red for up to 24 hours.Citation91 Later, we found that the type of loaded dye also played an important role with regard to release kinetics and stability of the PLA particles in the hydrophobic environment of the hair follicle infundibulum.Citation90 Carrier systems can also improve the tolerability of retinoids for the treatment of acne. Despite their promising anti-aging and anti-comedolitic properties, these drugs can cause irritation and pain and their encapsulation in carrier systems can help to reduce this side effect. Most studies used lipid-based carriers but polymers have also been used in combination with emulsions or lipid carrier systems. For example, Cho et al used PEG-PCL-PEO triblock copolymers to stabilize retinol emulsions, whereas Ridolfi et al used chitosan-solid lipid nanoparticles to delivery tretinoin.Citation92,Citation93
The targeting of drugs to perifollicular immune system cells by means of polymer particles might be useful for the treatment of alopecia areata. This autoimmune disease is characterized by infiltrating cluster of differentiation (CD) 4 and CD8 T-cells directed against auto-antigens and results in the destruction of the hair shaft. The targeted inhibition of overreacting cells by means of particulate carriers might be a possible therapy. For example, 75 µm cationized gelatine particles were used to deliver siRNA as a new therapeutic approach, targeting the T-box21 gene (Tbx21) to repress the expression of interferon-γ gene.Citation94 Another possible treatment is the topical application of diphenylcyclopropenone (DCP), which is an irritant and diverts T-cells from the hair shaft. However, the treatment is painful (irritation) and can be done only by trained personnel. The use of particles might allow a slow but sustained release of the substance minimizing the irritating effect.
Skin cancer
Polymeric carrier systems like dendrimers, microspheres, and nanogels, besides lipid-based carriers, are ideal systems for the topical delivery of chemotherapeutic agents and siRNAs to treat skin malignancies.Citation95 In fact, the topical delivery of anti-proliferative agents and photosensitizing agents has the advantage of reducing side effects and improving the compliance of the patients. Such carrier systems, eg, PLA or PAMAM based particles, have been tested for the topical delivery of drugs to different types of skin tumors.Citation96–Citation99
Targeting the skin immune system
Skin possesses a large number of immune active cells, from keratinocytes to different sub-types of skin resident T-cells, macrophages, dendritic cells, and mast cells. The fact that mainly dendritic cells are prone to take up particulate material suggested using submicron carriers to target this cell population.Citation100 Dendritic cells are potent antigen presenting cells and represent the bridge between innate and adaptive immune response. It has been shown that targeting antigens to the skin by means of virus particles (MVA), but also using PLA particles, induces potent cellular responses as well as skin and mucosal humoral immunity.Citation101,Citation102 Thus, different types of nanoparticles are actually under investigation as vaccine delivery systems against cancer and infectious diseases.Citation103 In order to deliver a sufficient amount of antigen to skin dendritic cells, it is important that particles are delivered across the skin barrier and that they are taken up by the target cells. For this reason, different physical methods (eg, stripping, microneedles, electroporation, or iontophoresis) have been developed to overcome the skin barrier.Citation104 Thus, for vaccination purposes it is mandatory to use biodegradable and compatible material to avoid intolerance responses or accumulation of particles in secondary lymphatic organs.
Gene therapy
Another special challenge for drug delivery is the targeting of therapeutic genes to skin cells. Very promising is also the delivery of siRNA molecules in order to control the post-transcriptional pathways of gene expression. To achieve this goal several obstacles have to be overcome. The deoxyribonucleic acid (DNA) or RNA moieties has to be transported across the skin barrier, be protected from degrading nucleases, be taken up by cells, and reach its target in the cytoplasm or in the cell nucleus. Polymer materials are intensively investigated as delivery agents because of the possibility to protect the nucleic acid and to achieve a sustained release and expression of the therapeutic gene. Despite all obstacles, there are encouraging reports describing the successful in vivo expression of genes after topical delivery of DNA loaded on cationic or PEGylated liposome as well as chitosan nanoparticles.Citation105–Citation107 Tissue growth, vaccination, anticancer treatment, and anti-inflammatory therapy can be achieved by gene transfer or regulation of gene expression.Citation108,Citation109 Principally, all skin diseases with a genetic basis could be treated by delivery of the missing or dysfunctional gene.
Conclusion
Several studies showed the efficacy and advantages of biodegradable polymeric materials as carriers or delivery systems in dermatology. These materials are safe and offer tools to develop carrier systems tailored for each specific dermatological disease. For this purpose, future work should focus on a more detailed knowledge of the interactions occurring between polymeric carrier systems and skin environment. Drug permeation and efficacy measurements should be complemented by detailed investigation on carrier skin penetration, uptake by skin cells, mechanism of degradation and drug-release, as well as toxicological properties. Also, it is important that such investigations are conducted using skin models of the specific pathological conditions to be treated. Investigating the interaction of delivery systems with disrupted skin barrier or activated immune system will allow tailoring polymeric materials in order to enhance the selectivity and specificity of drug delivery. The fact that new polymer-based carrier systems are being developed and that the number of their dermatological applications is constantly increasing demonstrates the huge potential of these biodegradable and versatile materials.
Acknowledgments
We thank P Schrade and Prof Dr S Bachmann (Department of Electron Microscopy, Charité – Universitätsmedizin Berlin, Germany) for the electron microscopy imaging of the human hair follicle. This study was supported by the Deutsche Forschung Gesellschaft, SFB1112 - Nanocarrier: Architektur, Transport und zielgerichtete Applikation von Wirkstoffen für therapeutische Anwendungen.
Disclosure
The authors report no conflicts of interest in this work.
References
- PapakostasDRancanFSterryWBlume-PeytaviUVogtANanoparticles in dermatologyArch Dermatol Res2011303853355021837474
- EgbariaKWeinerNLiposomes as a topical drug delivery systemAdv Drug Deliver Rev199053287300
- MüllerRHRadtkeMWissingSASolid lipid nanoparticles (SLN) and nanostructured lipid carriers (NLC) in cosmetic and dermatological preparationsAdv Drug Deliv Rev200254Suppl 1S131S15512460720
- GuterresSSAlvesMPPohlmannARPolymeric nanoparticles, nanospheres and nanocapsules, for cutaneous applicationsDrug Target Insights2007214715721901071
- ZhongSPZhangYZLimCTTissue scaffolds for skin wound healing and dermal reconstructionWiley Interdiscip Rev Nanomed Nanobiotechnol20102551052520607703
- VenugopalJRamakrishnaSBiocompatible nanofiber matrices for the engineering of a dermal substitute for skin regenerationTissue Eng2005115–684785415998224
- EkaputraAKPrestwichGDCoolSMHutmacherDWThe three-dimensional vascularization of growth factor-releasing hybrid scaffold of poly (epsilon-caprolactone)/collagen fibers and hyaluronic acid hydrogelBiomaterials201132328108811721807407
- HadjizadehADoillonCJDirectional migration of endothelial cells towards angiogenesis using polymer fibres in a 3D co-culture systemJ Tissue Eng Regen Med20104752453120872739
- WichterleOLimDHydrophilic gels for biological useNature1960185117118
- HoffmanASHydrogels for biomedical applicationsAdv Drug Deliv Rev200254131211755703
- ArtsJHMuijserHDuistermaatEJunkerKKuperCFFive-day inhalation toxicity study of three types of synthetic amorphous silicas in Wistar rats and post-exposure evaluations for up to 3 monthsFood Chem Toxicol200745101856186717524541
- BettencourtAAlmeidaAJPoly(methyl methacrylate) particulate carriers in drug deliveryJ Microencapsul201229435336722251239
- RehmanKZulfakarMHRecent advances in gel technologies for topical and transdermal drug deliveryDrug Dev Ind Pharm2013
- ChenXPengLHShanYHAstragaloside IV-loaded nanoparticle-enriched hydrogel induces wound healing and anti-scar activity through topical deliveryInt J Pharm20134471–217118123500766
- Moya-OrtegaMDAlvarez-LorenzoCConcheiroALoftssonTCyclodextrin-based nanogels for pharmaceutical and biomedical applicationsInt J Pharm20124281–215216322388054
- Asadian-BirjandMSousa-HervesASteinhilberDCugginoJCCalderonMFunctional nanogels for biomedical applicationsCurr Med Chem201219295029504322963637
- SasakiYAkiyoshiKNanogel engineering for new nanobiomaterials: from chaperoning engineering to biomedical applicationsChem Rec201010636637620836092
- DangJMLeongKWNatural polymers for gene delivery and tissue engineeringAdv Drug Deliv Rev200658448749916762443
- ElzoghbyAOSamyWMElgindyNAProtein-based nanocarriers as promising drug and gene delivery systemsJ Control Release20121611384922564368
- ChoKWangXNieSChenZGShinDMTherapeutic nanoparticles for drug delivery in cancerClin Cancer Res20081451310131618316549
- FengYLuJBehlMLendleinAProgress in depsipeptide-based biomaterialsMacromol Biosci20101091008102120602421
- FahmyTMDementoSLCaplanMJMellmanISaltzmanWMDesign opportunities for actively targeted nanoparticle vaccinesNanomedicine (Lond)20083334335518510429
- PanyamJLabhasetwarVBiodegradable nanoparticles for drug and gene delivery to cells and tissueAdv Drug Deliv Rev200355332934712628320
- LuengoJWeissBSchneiderMInfluence of nanoencapsulation on human skin transport of flufenamic acidSkin Pharmacol Physiol200619419019716679821
- Brannon-PeppasLRecent advances on the use of biodegradable microparticles and nanoparticles in controlled drug deliveryInt J Pharm1995116119
- RöslerAVandermeulenGWKlokHAAdvanced drug delivery devices via self-assembly of amphiphilic block copolymersAdv Drug Deliv Rev20015319510811733119
- StiribaSEFreyHHaagRDendritic polymers in biomedical applications: from potential to clinical use in diagnostics and therapyAngew Chem Int Ed Engl20024181329133419750755
- MichaelsASChandrasekaranSKShawJEDrug permeation through human skin: theory and in vitro experimental measurementAICHE J1975215985996
- ProkschEBrandnerJMJensenJMThe skin: an indispensable barrierExp Dermatol200817121063107219043850
- BouwstraJAHoneywell-NguyenPLSkin structure and mode of action of vesiclesAdv Drug Deliv Rev200254 Suppl 1S41S5512460715
- Schmid-WendtnerMHKortingHCThe pH of the skin surface and its impact on the barrier functionSkin Pharmacol Physiol200619629630216864974
- RawlingsAVVoegeliRStratum corneum proteases and dry skin conditionsCell Tissue Res2013351221723523053051
- SimonettiOHoogstraateAJBialikWVisualization of diffusion pathways across the stratum corneum of native and in-vitro-reconstructed epidermis by confocal laser scanning microscopyArch Dermatol Res199528754654737625858
- WangTFKastingGBNitscheJMA multiphase microscopic diffusion model for stratum corneum permeability. II. Estimation of physicochemical parameters, and application to a large permeability databaseJ Pharm Sci200796113024305117876780
- LademannJKnorrFRichterHHair follicles – an efficient storage and penetration pathway for topically applied substances. Summary of recent results obtained at the Center of Experimental and Applied Cutaneous Physiology, Charité-Universitätsmedizin Berlin, GermanySkin Pharmacol Physiol200821315015518523412
- SzaboGThe number of eccrine sweat glands in human skinAdv Biol Skin1962315
- LademannJRichterHTeichmannANanoparticles – an efficient carrier for drug delivery into the hair folliclesEur J Pharm Biopharm200766215916417169540
- VogtAHadamSHeiderhoffMMorphometry of human terminal and vellus hair folliclesExp Dermatol2007161194695017927578
- SchwartzJRShahRKrigbaumHSachaJVogtABlume-PeytaviUNew insights on dandruff/seborrhoeic dermatitis: the role of the scalp follicular infundibulum in effective treatment strategiesBr J Dermatol2011165 Suppl 2182321919899
- Blume-PeytaviUMassoudyLPatzeltAFollicular and percutaneous penetration pathways of topically applied minoxidil foamEur J Pharm Biopharm201076345045320600888
- TrauerSLademannJKnorrFDevelopment of an in vitro modified skin absorption test for the investigation of the follicular penetration pathway of caffeineSkin Pharmacol Physiol201023632032720588083
- TollRJacobiURichterHLademannJSchaeferHBlume-PeytaviUPenetration profile of microspheres in follicular targeting of terminal hair folliclesJ Invest Dermatol2004123116817615191557
- WatkinsonACBungeALHadgraftJLaneMENanoparticles do not penetrate human skin – a theoretical perspectivePharm Res20133081943194623722409
- BaroliBPenetration of nanoparticles and nanomaterials in the skin: fiction or reality?J Pharm Sci2010991215019670463
- NohynekGJLademannJRibaudCRobertsMSGrey goo on the skin? Nanotechnology, cosmetic and sunscreen safetyCrit Rev Toxicol200737325127717453934
- SchreierHBouwstraJLiposomes and niosomes as topical drug carriers: dermal and transdermal drug deliveryJ Control Rel1994301115
- FangJYHongCTChiuWTWangYYEffect of liposomes and niosomes on skin permeation of enoxacinInt J Pharm20012191–2617211337166
- SamahNAWilliamsNHeardCMNanogel particulates located within diffusion cell receptor phases following topical application demonstrates uptake into and migration across skinInt J Pharm20104011–2727820817080
- ZhangLWMonteiro-RiviereNAAssessment of quantum dot penetration into intact, tape-stripped, abraded and flexed rat skinSkin Pharmacol Physiol200821316618018523414
- ProwTWMonteiro-RiviereNAInmanAOQuantum dot penetration into viable human skinNanotoxicology20126217318521456897
- LaneMESkin penetration enhancersInt J Pharm20134471–2122123462366
- PrausnitzMRMicroneedles for transdermal drug deliveryAdv Drug Deliv Rev200456558158715019747
- LinLLGriceJEButlerMKTime-correlated single photon counting for simultaneous monitoring of zinc oxide nanoparticles and NAD(P)H in intact and barrier-disrupted volunteer skinPharm Res201128112920293021717255
- ShahPPDesaiPRPatelARSinghMSSkin permeating nanogel for the cutaneous co-delivery of two anti-inflammatory drugsBiomaterials20123351607161722118820
- KangMJEumJYJeongMSFacilitated skin permeation of oregonin by elastic liposomal formulations and suppression of atopic dermatitis in NC/Nga miceBiol Pharm Bull201033110010620045944
- Abdel-MottalebMMMoulariBBeduneauAPellequerYLamprechtANanoparticles enhance therapeutic outcome in inflamed skin therapyEur J Pharm Biopharm201282115115722728016
- RouvièrePELiJBrillDJBionanotechnology application of polypeptides in a hair color product: self-assembly enables expression, processing, and functionalityBiotechnol J20138224725622777899
- VogtACombadiereBHadamS40 nm, but not 750 or 1,500 nm, nanoparticles enter epidermal CD1a+ cells after transcutaneous application on human skinJ Invest Dermatol200612661316132216614727
- RancanFGaoQGrafCSkin penetration and cellular uptake of amorphous silica nanoparticles with variable size, surface functionalization, and colloidal stabilityACS Nano2012686829684222797484
- MortensenLJOberdörsterGPentlandAPDelouiseLAIn vivo skin penetration of quantum dot nanoparticles in the murine model: the effect of UVRNano Lett2008892779278718687009
- MaheBVogtALiardCNanoparticle-based targeting of vaccine compounds to skin antigen-presenting cells by hair follicles and their transport in miceJ Invest Dermatol200912951156116419052565
- O’ReganGMSandilandsAMcLeanWHIrvineADFilaggrin in atopic dermatitisJ Allergy Clin Immunol20091243 Suppl 2R2R619720209
- YanagisawaRTakanoHInoueKIKoikeESadakaneKIchinoseTSize effects of polystyrene nanoparticles on atopic dermatitislike skin lesions in NC/NGA miceInt J Immunopathol Pharmacol201023113114120378001
- KirschnerNPoetzlCvon den DrieschPAlteration of tight junction proteins is an early event in psoriasis: putative involvement of proinflammatory cytokinesAm J Pathol200917531095110619661441
- ProwTWGriceJELinLLNanoparticles and microparticles for skin drug deliveryAdv Drug Deliv Rev201163647049121315122
- BaldoAMonodMMathyAMechanisms of skin adherence and invasion by dermatophytesMycoses201255321822321831104
- BaumannLSkin ageing and its treatmentJ Pathol2007211224125117200942
- GhadiallyRBrownBESequeira-MartinSMFeingoldKREliasPMThe aged epidermal permeability barrier. Structural, functional, and lipid biochemical abnormalities in humans and a senescent murine modelJ Clin Invest1995955228122907738193
- TezukaTQingJSahekiMKusudaSTakahashiMTerminal differentiation of facial epidermis of the aged: immunohistochemical studiesDermatology1994188121248305750
- ConnerSDSchmidSLRegulated portals of entry into the cellNature20034226927374412621426
- ZhangLWMonteiro-RiviereNAMechanisms of quantum dot nanoparticle cellular uptakeToxicol Sci2009110113815519414515
- KettigerHSchipanskiAWickPHuwylerJEngineered nanomaterial uptake and tissue distribution: from cell to organismInt J Nanomedicine201383255326924023514
- LutenJvan NostrumCFDe SmedtSCHenninkWEBiodegradable polymers as non-viral carriers for plasmid DNA deliveryJ Control Release200812629711018201788
- AzevedoHSReisRLUnderstanding the Enzymatic Degradation of Biodegradable Polymers and Strategies to Control their Degradation Rate Biodegradable Systems in Tissue Engineering and Regenerative MedicineBoca RatonCRC Press2005177201
- MansourAMDrevsJEsserNA new approach for the treatment of malignant melanoma: enhanced antitumor efficacy of an albumin-binding doxorubicin prodrug that is cleaved by matrix metalloproteinase 2Cancer Res200363144062406612874007
- YooHSLeeEAParkTGDoxorubicin-conjugated biodegradable polymeric micelles having acid-cleavable linkagesJ Control Release2002821172712106973
- WengHZhouJTangLHuZTissue responses to thermally-responsive hydrogel nanoparticlesJ Biomater Sci Polym Ed20041591167118015503633
- KüchlerSRadowskiMRBlaschkeTNanoparticles for skin penetration enhancement – a comparison of a dendritic core-multishell-nanotransporter and solid lipid nanoparticlesEur J Pharm Biopharm200971224325018796329
- KüchlerSAbdel-MottalebMLamprechtARadowskiMRHaagRSchäfer-KortingMInfluence of nanocarrier type and size on skin delivery of hydrophilic agentsInt J Pharm20093771–216917219439166
- ChiangATudelaEMaibachHIPercutaneous absorption in diseased skin: an overviewJ Appl Toxicol201232853756322912973
- HussainZKatasHMohd AminMCKumolosasiEBuangFSahudinSSelf-assembled polymeric nanoparticles for percutaneous co-delivery of hydrocortisone/hydroxytyrosol: an ex vivo and in vivo study using an NC/Nga mouse modelInt J Pharm20134441–210911923337632
- JaquesJARezerJFRuchelJBAn experimental model of contact dermatitis: evaluation of the oxidative profile of Wistar rats treated with free and nanoencapsulated clobetasolRedox Rep201217520621323068967
- ChuCCDi MeglioPNestleFOHarnessing dendritic cells in inflammatory skin diseasesSemin Immunol2011231284121295490
- BakROMikkelsenJGRegulation of cytokines by small RNAs during skin inflammationJ Biomed Sci2010175320594301
- FriedmanAJHanGNavatiMSSustained release nitric oxide releasing nanoparticles: characterization of a novel delivery platform based on nitrite containing hydrogel/glass compositesNitric Oxide2008191122018457680
- LansdownABSilver. I: Its antibacterial properties and mechanism of actionJ Wound Care200211412513011998592
- RujitanarojPOPimphaNSupapholPWound-dressing materials with antibacterial activity from electrospun gelatin fiber mats containing silver nanoparticlesPolymer2008492147234732
- ShimJSeok KangHParkWSHanSHKimJChangISTransdermal delivery of mixnoxidil with block copolymer nanoparticlesJ Control Release200497347748415212879
- KnorrFLademannJPatzeltASterryWBlume-PeytaviUVogtAFollicular transport route – research progress and future perspectivesEur J Pharm Biopharm200971217318019041720
- RancanFTodorovaAHadamSStability of polylactic acid particles and release of fluorochromes upon topical application on human skin explantsEur J Pharm Biopharm2012801768421945270
- RancanFPapakostasDHadamSInvestigation of polylactic acid (PLA) nanoparticles as drug delivery systems for local dermatotherapyPharm Res20092682027203619533305
- ChoHKChoJHChoiSWCheongIWTopical delivery of retinol emulsions co-stabilised by PEO-PCL-PEO triblock copolymers: effect of PCL block lengthJ Microencapsul201229873974622583129
- RidolfiDMMarcatoPDJustoGZCordiLMachadoDDuránNChitosan-solid lipid nanoparticles as carriers for topical delivery of tretinoinColloids Surf B Biointerfaces201293364022244299
- NakamuraMJoJTabataYIshikawaOControlled delivery of T-box21 small interfering RNA ameliorates autoimmune alopecia (Alopecia Areata) in a C3H/HeJ mouse modelAm J Pathol2008172365065818245811
- RomeroELMorillaMJHighly deformable and highly fluid vesicles as potential drug delivery systems: theoretical and practical considerationsInt J Nanomedicine201383171318623986634
- ZampieriALFerreiraFSResendeECBiodegradable polymeric nanocapsules based on poly(DL-lactide) for genistein topical delivery: obtention, characterization and skin permeation studiesJ Biomed Nanotechnol20139352753423621010
- VenugantiVVPerumalOPEffect of poly(amidoamine) (PAMAM) dendrimer on skin permeation of 5-fluorouracilInt J Pharm20083611–223023818582550
- ZhaoQHZhangYLiuYAnticancer effect of realgar nanoparticles on mouse melanoma skin cancer in vivo via transdermal drug deliveryMed Oncol201027220321219280372
- CasasABattahSDi VenosaGSustained and efficient porphyrin generation in vivo using dendrimer conjugates of 5-ALA for photodynamic therapyJ Control Release2009135213614319168101
- ReddySTSwartzMAHubbellJATargeting dendritic cells with biomaterials: developing the next generation of vaccinesTrends Immunol2006271257357917049307
- DuffyDPerrinHAbadieVNeutrophils transport antigen from the dermis to the bone marrow, initiating a source of memory CD8+ T cellsImmunity201237591792923142782
- LiardCMunierSAriasMTargeting of HIV-p24 particle-based vaccine into differential skin layers induces distinct arms of the immune responsesVaccine201129376379639121554912
- HansenSLehrCMNanoparticles for transcutaneous vaccinationMicrob Biotechnol20125215616721854553
- MatsuoKHirobeSOkadaNNakagawaSFrontiers of transcutaneous vaccination systems: novel technologies and devices for vaccine deliveryVaccine201331192403241523523401
- LiJLiXZhangYGene therapy for psoriasis in the K14-VEGF transgenic mouse model by topical transdermal delivery of interleukin-4 using ultradeformable cationic liposomeJ Gene Med201012648149020527041
- Özbaş-TuranSAkbuğaJPlasmid DNA-loaded chitosan/TPP nanoparticles for topical gene deliveryDrug Deliv201118321522221226549
- AissaouiAChamiMHusseinMMillerADEfficient topical delivery of plasmid DNA to lung in vivo mediated by putative triggered, PEGylated pDNA nanoparticlesJ Control Release2011154327528421699935
- ZhengDGiljohannDAChenDLTopical delivery of siRNA-based spherical nucleic acid nanoparticle conjugates for gene regulationProc Natl Acad Sci U S A201210930119751198022773805
- SeguraTFormulations and Delivery Limitations of Nucleic-Acid-Based TherapiesHandbook of Pharmaceutical Biotechnology201010131059