Abstract
Background/purpose
The aim of this study was to test the hypothesis that mechanical ventilation (MV) during cancer surgery induces lung stroma/tissue milieu changes, creating a favorable microenvironment for postoperative lung metastatic tumor establishment.
Materials and methods
In Protocol A, female BALB/c mice were divided into an MV group and a control (no MV) group, both of which were anesthetized and subjected to intravenous injection of green fluorescent protein (GFP)-labeled mouse mammary carcinoma cell line (4T1) cells. After 24 h, the lung tissue was removed and the number of GFP-labeled 4T1 cells was calculated. In Protocol B, the clinically relevant mouse model of spontaneous breast cancer lung metastasis was used with surgical resection of the primary tumor to investigate the MV event that dictates postoperative lung metastasis. Female BALB/c mice were inoculated in the mammary fat pad with 4T1 cells. After 14-d growth, mice were anesthetized and divided into an MV group and a control (no MV) group during surgical procedures (mastectomy). Metastatic tumor burden was assessed two weeks after mastectomy by both macroscopic metastatic nodule count, hematoxylin–eosin histology, immunohistochemistry for the macrophage marker (CD68), and epithelial cell adhesion molecule (EpCAM).
Results
MV was associated with a significant increase in the number of circulating breast tumor cells (GFP-labeled 4T1 cells) remaining in the microvasculature of the lung (P<0.01). Immunohistochemical results showed increased infiltration of CD68-positive macrophages within injured lung parenchyma and metastatic tumor as well as increased expression of EpCAM in metastatic nodules. Postoperative metastases were more prevalent in the mechanically ventilated mice group compared to the non-ventilated group (P<0.05).
Conclusion
MV-induced lung metastasis occurs by attracting circulating tumor cells to the site of the lung injury and by accelerating the proliferation of preexisting micro-metastases in the lung. These observations indicate that the metastasis-enhancing effect of MV should be considered in general anesthesia during cancer surgery.
Introduction
The main cause of high mortality rates due to breast cancer is the development of distant metastasis. Molecular mechanisms controlling metastatic growth and dormancy continue to provide many challenges, despite advances in the understanding of its pathogenesis.Citation1,Citation2 Although there are various treatment options for breast cancer, control of advanced disease often depends on surgical removal of the primary tumor. Unfortunately, both clinical and experimental studies have shown that cancer surgery may increase the risk of metastases and recurrence.Citation2,Citation3 Tumor manipulation during surgery may increase the metastatic process via tumor cell dissemination into the lymphatic network and bloodstream. Moreover, many patients shelter micro-metastases and scattered tumor cells at the time of surgery. Moreover, perioperative factors can inhibit important host defense and promote the development of metastases.Citation3–Citation5
It is well known that anesthesia plays an important role in cancer recurrence.Citation5,Citation6 Retrospective studies suggest that regional anesthesia reduces the risk of tumor metastasis and recurrence compared with general anesthesia.Citation6 It is increasingly recognized that anesthetic drugs administered during general anesthesia can inhibit the cellular immune system and affect cancer recurrence.Citation7–Citation9 MV is mandatory in patients undergoing general anesthesia. Although MV is considered a safe procedure, it can generate pulmonary stress, triggering local inflammation and local coagulation.Citation10–Citation12 We, therefore, hypothesize that MV used in general anesthesia during breast cancer surgical procedures may directly affect lung metastasis.
Metastasis is a complex, coordinated process that depends on the interaction between cancer cells and the tumor micro-environment, involving several cell types, cytokines, and cell-adhering molecular pathways.Citation13–Citation16 During metastasis, cells that are arrested in a capillary bed (intravasation) are able to invade the host-organ parenchyma (extravasation), resulting in the growth of secondary tumor cell colonies.Citation15–Citation18 Animal studies have shown that, following intravenous injection, the vast majority of circulating tumor cells are initially arrested in the lungs, although few cells remain in the lungs after 1–3 days.Citation19 The arrest of circulating cancer cells in the capillary beds of the lungs and their subsequent release have been associated with the anti-metastatic effect of healthy microvascular endothelial barriersCitation17,Citation18 and the host immune system.Citation20 Disruption of the endothelial barrier has been reported to directly facilitate cancer cell intravasation/ extravasation, which accelerates cancer metastasis.Citation21
The association between high-airway-pressure MV and pronounced morphological changes at both the epithelial and microvascular endothelial barriers is well defined in animal models.Citation12 There is evidence that subtle endothelial changes and inflammatory reaction within the lungs might be caused by MV at low lung volume.Citation22,Citation23 Macrophages have a central role in the maintenance of immunological homeostasis and host defense. Macrophages can be rapidly activated by MV and, thus, may play an important role as inflammatory mediators in the pathogenesis of lung injury induced by MV.Citation24,Citation25 A number of animal studies have shown that injurious ventilatory strategies are associated with the release of a variety of proinflammatory mediators, which is important in cell signaling. Proinflammatory cytokines such as IL-1β, IL-6, and TNF-α are produced predominantly by activated macrophages and are involved in the upregulation of inflammatory reactions.Citation11,Citation24,Citation25
EpCAM, a transmembrane glycoprotein, is highly expressed in rapidly proliferating carcinomas, including breast cancer.Citation26 Recent data suggest a more multipotent role of EpCAM, such as cell–cell adhesion, cell signaling, migration, inflammation, proliferation, and differentiation.Citation27–Citation29 In addition, it was shown that EpCAM plays a major role in lung metastatic processes.Citation26,Citation30
In the present study, we investigated the role MV plays in promoting lung metastasis. The study was designed to use two protocols (A and B) to assess: 1) the effect of MV on lung 4T1 tumor-cell retention in BALB/c mice, 24 h after 4T1 cell tail-vein injection (Protocol A); 2) the impact of MV on vascular permeability, alveolar damage, and inflammatory cell infiltrates (Protocol A); and 3) effects of MV during surgical resection of the primary tumor on lung metastasis development, macrophage infiltration, and EpCAM expression in lungs two weeks after the operation (Protocol B) using a clinically relevant animal model of spontaneous breast cancer lung metastasis.
Materials and methods
Experimental animals
Female BALB/c mice (6–8 weeks old) were purchased from Beijing HFK Bioscience Co. Ltd. (batch number SCXK 2014-004). Study animals were housed in the animal research facility at the China-Japan Friendships Clinic Medical Research Institute. The treatment and use of animals during the study were in accordance with the regulations of the Guide for the Care and Use of Laboratory Animal of the National Institutes of Health (GB14925-2010). The study protocols were approved by the Committee on the Ethics of Animal Experiments of the China-Japan Friendship Hospital.
Anesthesia
Mice were anaesthetized by intraperitoneal injection of a mixture of pentobarbital sodium (60 mg/mL), diazepam (5 mg/ mL), and PBS in the proportion 1:2:1 (0.4 mL/kg body weight).
Mechanical ventilation
MV was undertaken as described elsewhere.Citation13,Citation37 Briefly, after sodium pentobarbital anesthesia and insertion of a tracheotomy tube (1.3 mm outer diameter and 0.8 mm inner diameter), female BALB/c mice were connected to a ventilator (DHX-50; Chengdu Instrument Company, Chengdu, People’s Republic of China) and mechanically ventilated for 60 min (tidal volume, 8 mL/kg; positive end-expiratory pressure, 4 cm H2O; fraction of inspired oxygen, 0.40).Citation22,Citation23,Citation25
Cell culture
The highly metastatic 4T1 breast cancer cell line derived from a spontaneously arising BALB/c mammary tumor was supplied by the Cell Center of the Chinese Academy of Medical Sciences (Beijing, People’s Republic of China). These 4T1 cells (3×105 cells/mL) were plated on six-well plates at a concentration of 3×104 cells/well and transfected using the polybrene method with GFP-containing plasmid (plenti6-EGFP, 8 μg/mL; Invitrogen, NY, USA). The cells were maintained in Roswell Park Memorial Institute (RPMI) 1640 medium, supplemented with 10% FBS and 1% penicillin–streptomycin (Gibco-Invitrogen, Grand Island, NY, USA), at 37°C in a humidified incubator in 5% CO2.Citation31 The cell-culture medium was changed 16 h after seeding, and cells were split after 48 h.
Histology
Mice were euthanized, and the lungs were excised. The lung tissues were fixed in 4% paraformaldehyde at room temperature for 12 h, then dehydrated overnight in 30% sucrose at 4°C, and embedded in optimal cutting temperature compound (OCT compound). Serial 8-μm tissue sections were sliced throughout the entire lungs on a cryostat cryomicrotome (Leica 3050S) and mounted on positively charged microscope slides (Super Frost Plus; ThermoFisher Scientific, Waltham, MA, USA). The frozen lung sections were subjected to standard H&E and immunohistochemical staining. For H&E staining, sections were stained by following standard procedure. The Olympus IX71 Inverted Fluorescence Microscope, equipped with a blue excitation cube, was used to detect GFP signals in the frozen lung sections. The total number of 4T1 cells per lung section (n=8, 8–10 sections) was counted and averaged among the animals of each group.Citation32
Immunohistochemical procedures
For EpCAM staining, frozen tissue sections were washed in TBS (25 mM Tris, 150 mM NaCl, and 3mM KCl, adjusted to pH 8.0 with 1N NaOH) and incubated in 0.6% H2O2 and 0.06% NaN2 for 30 min to quench endogenous peroxidase activity. After 30-min incubation in 1% BSA-TBS, the slides were incubated with a rabbit polyclonal anti-EpCAM, 1:100 (c-terminus, ab71916; Abcam, Cambridge, UK) or with a rabbit polyclonal anti-CD68, 1:100 (Abcam, Shanghai, People’s Republic of China) primary antibody in 0.1% BSA-TTBS (TBS containing 0.1% Triton X-100) at 4°C overnight, followed by a second incubation with biotinylated goat anti-rabbit-IgG, 1:200 (DAKO, Denmark) for 60 min at room temperature. The sections were developed in GDN solution for 5–20 min and counterstained with hematoxylin,Citation30 before being dehydrated through a gradient of alcohol, cleared with xylene, and mounted with Mountex (Histolab, Gothenburg, Sweden). Images were taken with an Olympus microscope (BX51; Olympus, Japan).
Quantification of EpCAM immunostaining was undertaken by digital image analysis with IPP 6.0 (Media Cybernetics). A total of five fields selected from hotspot areas (metastatic nodules) in the digital image (×400) were acquired per slice, and five slices from separate animals within each group (four animals) were examined. The IOD of all positive staining in each field as well as the AOP were measured.
For macrophage quantitative analysis, the number of CD68-positive cells was manually counted in five random fields at ×20 magnification of view per section from four animals per group.
Animal experiment protocols
The summary of the experiments is depicted in . The number of mice studied in each experiment is described in each protocol.
Figure 1 Schematic summary of the experimental protocols. Mice were injected with 4T1-GFP cells via the tail vein under intraperitoneal (i.p.) anesthesia, with or without MV. Twenty-four hours later, the mice were sacrificed for analysis of lung 4T1 tumor cells retention (Protocol A). In Protocol B, 4T1 cells were inoculated into the mammary fat pad of 6-week-old female BALB/c mice. Fourteen days later, mastectomy was done under i.p. anesthesia with or without MV. The mice were sacrificed at Day 28 for the lung metastasis assay as described in the “Materials and methods” section.
Abbreviations: d, days; h, hours; MV, mechanical ventilation.
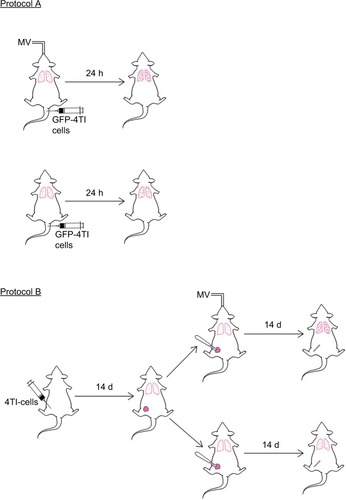
Protocol A
Mice were injected with 1×105 4T1-GFP cells dissolved in 100 μL sterile PBS via the median tail vein, followed by MV for 60 min under anesthesia. Control mice were only injected intravenously with 1×105 GFP-labeled 4T1 cells without MV after intraperitoneal administration of pentobarbital sodium. Mice were killed after 24 h, and the lungs were excised and fixed in 10% neutral buffered formalin overnight. The tissue samples were dehydrated, embedded, and cryosectioned for fluorescence imaging to visualize the surviving circulating tumor cells. Furthermore, adjacent frozen sections were stained with H&E and used for EpCAM immunohistochemistry staining (MV and no MV groups, n=8).Citation32
Protocol B
The effect of MV on breast cancer lung metastasis in a 4T1 orthotopic mouse model was assessed using BALB/c mice. Briefly, mice were lightly anesthetized, the fur over the lateral thorax was shaved, and a 5 mm-long incision was made to reveal the mammary fat pad. The mammary fat pad was injected with 5×105 4T1 breast tumor cells dissolved in 100 μL sterile PBS and the incision was closed with a wound clip. Tumor volume was measured once a week using an electronic caliper. Two weeks after 4T1 inoculation, the mice were randomized into two groups (six mice per group). In Group 1, a complete resection of the mammary primary tumor was carried out in combination with MV for 60 min (under anesthesia). In Group 2, only the surgical excision was done under anesthesia with pentobarbital for a period of 60 min without MV. Two weeks after operation (four weeks after 4T1 tumor cell implantation), the mice were euthanized and the lungs were excised and fixed in 10% Bouin’s solution for 24 h to count the number of lung tumor nodules under a dissecting microscope. Furthermore, the lungs were washed in PBS, placed in 4% formalin solution for 48 h, then dehydrated, embedded, and cryosectioned for H&E and EpCAM immunohistochemical staining, and subjected to double-confirmed quantitative analysis under a microscope (BX51; Olympus). Results are reported as the average number of nodules per group (MV and no MV groups, n=6).Citation33
Statistical analyses
Data are presented as the mean±SD. Statistical significance was calculated by Student’s t test using the Graph Pad Prism statistical program (version 6.0; Graph Pad Software, Inc., San Diego, CA, USA). P<0.05 was considered to be statistically significant.
Results
MV induces lung tissue abnormalities and inflammatory responses in the metastatic 4T1 breast cancer model
The initial objective was to identify a reasonable volume of MV that would have a measurable experimental effect in the 4T1 spontaneous lung metastasis model without a significant increase in mortality two weeks after surgical excision of the primary tumor (data not presented). Preliminary experiments confirmed previous data, in which MV using clinically relevant ventilation settings (tidal volume, 8 mL/ kg; positive end-expiratory pressure, 4 cm H2O; fraction of inspired oxygen, 0.21; 60 min) induced mild lung injury (local alveolar injury, increase in vascular permeability, and influx of inflammatory cells) in BALB/c mice (). The results suggested that MV induced morphological changes in pulmonary microvascular endothelial structures before the appearance of definite metastases.
Figure 2 (A–F) Representative histological view and images of immunohistochemical staining using anti-EpCAM body in the lung treated with MV or without MV. H&E stain of lung sections (A and B) shows micro-metastases formation and mild lung injury 24 h after intravenous 4T1 cells inoculation and MV treatment (Protocol A). Bar: 50 µm. (A) Arrow shows the presence of small-blood-vessel hemorrhaging and vascular congestion of tumor emboli. The majority of tumor cells were found lining the interior of blood vessels. However, a few tumor cells were found spreading into the surrounding tissue in the areas wherein blood vessel integrity was lost (indicated by an arrowhead). Note the presence of minimal interstitial edema and increase in intravascular and interstitial inflammatory cells in the same areas (indicated by an arrowhead). (B) Empty arrow shows the presence of a single tumor nodule within a lung parenchyma injury field (the “seeding” region) and empty arrowhead indicates normal lung parenchyma, whereas the lungs show intact blood vessels, bronchioles, and alveolar spaces. (C) Low power (×10) photomicrograph of anti-EpCAM immunohistochemical staining in lung tissue sections taken from BALB/c mice in the control group (28 d after 5×105 4T1 cells were injected into the mammary fat pad, untreated with MV during surgical procedures 14 d after inoculation [Protocol B]). The arrowhead indicates weak EpCAM immunohistochemical staining in 4T1 cells in the central part of a single tumor nodule. Intact surrounding lung parenchyma is negative for EpCAM. m, tumour nodule. (D) Treated MV during surgical procedures (Protocol B) shows that almost all 4T1 cells express EpCAM in a single tumor nodule. Weak-to-moderate EpCAM immunohistochemistry staining was observed in the surrounding lung parenchyma. H&E staining of lung sections (E) shows extensive tumor cell metastasis and lung architecture destruction. Arrows show diffuse increase of interstitial cellularity, with both mononuclear cells and neutrophilic infiltration; the arrowhead indicates blood vessel formation within the tumor nodule. Bar: 100 µm. (F) High-power (×40) photomicrograph of lung, 28 d after 5×105 4T1 cells were injected into the mammary fat pad, treated with MV during surgical procedures, and 14 d after inoculation (Protocol B), 4T1 tumor cells were observed with strong membrane or cytoplasmic staining of EpCAM within the tumor nodule (indicated by empty arrows) and a few epithelial cells with strong cytoplasmic EpCAM immunohistochemical staining were observed surrounding the tumor nodule (indicated by empty arrowhead). Bar: 50 µm. (G) Quantification of EpCAM with integrated optical density (IOD) in the lung metastatic tumor nodule of the MV exposure group and control group by immunohistochemical staining. Data presented as mean±SD, n=4; the ** indicates statistical significance, P<0.01. (H) Quantification of EpCAM with the area of positive staining (AOP) in lung metastatic tumor nodules in the MV exposure group and control group. Data presented as mean±SD, n=4; the ** indicates statistical significance, P<0.01.
Abbreviations: d, days; EpCAM, epithelial cell adhesion molecule; h, hours; m, tumor nodule; MV, mechanical ventilation; S, surrounding lung parenchyma.
![Figure 2 (A–F) Representative histological view and images of immunohistochemical staining using anti-EpCAM body in the lung treated with MV or without MV. H&E stain of lung sections (A and B) shows micro-metastases formation and mild lung injury 24 h after intravenous 4T1 cells inoculation and MV treatment (Protocol A). Bar: 50 µm. (A) Arrow shows the presence of small-blood-vessel hemorrhaging and vascular congestion of tumor emboli. The majority of tumor cells were found lining the interior of blood vessels. However, a few tumor cells were found spreading into the surrounding tissue in the areas wherein blood vessel integrity was lost (indicated by an arrowhead). Note the presence of minimal interstitial edema and increase in intravascular and interstitial inflammatory cells in the same areas (indicated by an arrowhead). (B) Empty arrow shows the presence of a single tumor nodule within a lung parenchyma injury field (the “seeding” region) and empty arrowhead indicates normal lung parenchyma, whereas the lungs show intact blood vessels, bronchioles, and alveolar spaces. (C) Low power (×10) photomicrograph of anti-EpCAM immunohistochemical staining in lung tissue sections taken from BALB/c mice in the control group (28 d after 5×105 4T1 cells were injected into the mammary fat pad, untreated with MV during surgical procedures 14 d after inoculation [Protocol B]). The arrowhead indicates weak EpCAM immunohistochemical staining in 4T1 cells in the central part of a single tumor nodule. Intact surrounding lung parenchyma is negative for EpCAM. m, tumour nodule. (D) Treated MV during surgical procedures (Protocol B) shows that almost all 4T1 cells express EpCAM in a single tumor nodule. Weak-to-moderate EpCAM immunohistochemistry staining was observed in the surrounding lung parenchyma. H&E staining of lung sections (E) shows extensive tumor cell metastasis and lung architecture destruction. Arrows show diffuse increase of interstitial cellularity, with both mononuclear cells and neutrophilic infiltration; the arrowhead indicates blood vessel formation within the tumor nodule. Bar: 100 µm. (F) High-power (×40) photomicrograph of lung, 28 d after 5×105 4T1 cells were injected into the mammary fat pad, treated with MV during surgical procedures, and 14 d after inoculation (Protocol B), 4T1 tumor cells were observed with strong membrane or cytoplasmic staining of EpCAM within the tumor nodule (indicated by empty arrows) and a few epithelial cells with strong cytoplasmic EpCAM immunohistochemical staining were observed surrounding the tumor nodule (indicated by empty arrowhead). Bar: 50 µm. (G) Quantification of EpCAM with integrated optical density (IOD) in the lung metastatic tumor nodule of the MV exposure group and control group by immunohistochemical staining. Data presented as mean±SD, n=4; the ** indicates statistical significance, P<0.01. (H) Quantification of EpCAM with the area of positive staining (AOP) in lung metastatic tumor nodules in the MV exposure group and control group. Data presented as mean±SD, n=4; the ** indicates statistical significance, P<0.01.Abbreviations: d, days; EpCAM, epithelial cell adhesion molecule; h, hours; m, tumor nodule; MV, mechanical ventilation; S, surrounding lung parenchyma.](/cms/asset/4248dd1c-19b5-493e-87c6-973973bf2f48/dcmr_a_12185308_f0002_c.jpg)
MV affects arrest and retention of 4T1 in pulmonary microvasculature
Localization of GFP-4T1 cells in the lungs was examined 24 h after MV treatment. A significantly larger number of intravenously injected GFP-4T1 tumor cells were retained in the lungs of MV-treated mice () than in the lungs of controls without MV (), indicating tumor cell survival in the lungs at an early stage after seeding from the blood system. The majority of 4T1 tumor cells were localized in the injured lung areas and formed microtumor emboli in the mice after MV treatment (). The integrity of the pulmonary vasculature was found to be disrupted, the microvasculature wall was no longer smooth and regular, and capillary dilatation was apparent (). Furthermore, the penetration of circulating tumor cells in the injured lung areas was more severe than in the normal lungs ().
Figure 3 (A) Average numbers of visible GFP-4T1 cells in individual mouse lung were counted and are presented as mean±SD, n=8. The * indicates statistical significance, P<0.05. (B) Average numbers of visible 4T1 metastatic nodules in individual mouse lung were counted and are presented as mean±SD, n=6. The * indicates statistical significance, P<0.05. (C) Low-power (×10) photomicrograph of lung frozen sections from BALB/c mouse in the control group (24 h after intravenously injection of 1×105 GFP-labeled 4T1 cells without MV, Protocol A) shows GFP-labeled 4T1 cells scattered within the lung parenchyma. (D) Photomicrograph (×10) of lung frozen sections from BALB/c mice (24 h later, treated with MV when the tail vein was injected with 1×105 GFP-labeled 4T1 cells, Protocol A). Note the minimal interstitial edema and GFP-labeled 4T1 cells diffusely exist within injured lung parenchyma.
Abbreviations: d, days; GFP, green fluorescent protein; h, hours; MV, mechanical ventilation.
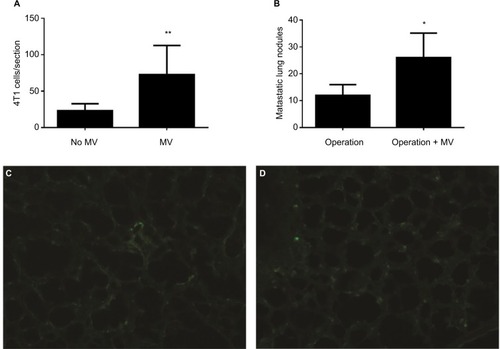
Furthermore, H&E staining revealed that the lung tissues of the MV-treated mice had inflammatory cellular infiltrates characterized by prominent lymphocytic cells (), suggesting that MV altered the microenvironment of the lung by triggering an inflammatory response. These results suggest that even low-volume MV can induce mild lung injury, while producing an attractive microenvironment for circulatory tumor cells to arrest and survive in the lung at an early stage after seeding from the bloodstream. In addition, these results show that MV modulated the pulmonary microenvironment by disrupting vascular integrity and altering vascular endocrine morphology (, and ).
MV affects postoperative lung metastases of 4T1 cells
To determine whether MV under surgery affects postoperative pulmonary metastases, we implanted 5×105 4T1 cells subcutaneously into the flanks of female BALB/c mice. After 14 d, the entire primary tumor (diameter, 8–15 mm, n=6) receded with or without MV (control) under mastectomy. Lung tissues were removed from the mice 28 d postoperatively and soaked in Bouin’s solution to count metastatic nodules. Tumor metastatic nodules were more prevalent in the MV-treated lungs than in the normal lungs (). We found that 4T1 tumor cell localization and metastatic growth in the lung were dramatically enhanced following surgical removal of the primary tumor with MV than without MV (control, n=6; ). In the MV-treated group, the number of pulmonary nodules was 2.22-fold that of the control group (P<0.05).
MV alters EpCAM expression patterns in the pulmonary in the metastatic 4T1 breast cancer model
In the MV group, immunohistochemical staining of EpCAM in the postoperative pulmonary metastases showed strong membrane staining and moderate-to-strong staining in the cytoplasm (), indicating tumor cell survival and growth. In the control group (without MV under mastectomy), lung metastasis nodules demonstrated weak immunohistochemical staining. In addition, low EpCAM expression was found at the periphery of the tumor, suggesting functional inactivation of EpCAM (). EpCAM staining was not only pronounced in metastatic foci, in which 4T1 tumor cells were observed with strong membrane or cytoplasmic staining (), but also labeled the surrounding lung parenchyma in the mechanically ventilated group (). In contrast, sections from lungs without MV treatment showed no changes in apparent EpCAM immunoreactivity in surrounding normal lung parenchyma of metastatic foci ().
Histologic examination of H&E staining of lung sections showed extensive destruction of lung architecture in the MV group (). Moreover, in conditions of the disordered repair of epithelium, the normal relationship of epithelial cells and basal membrane was altered. Surprisingly, a strong cytoplasmic linear EpCAM reaction was seen in the epithelial cells in injured lung tissues (proliferative phase) surrounding the metastatic nodules (), while alveolar epithelium exhibited normal weak basolateral membranous expression patterns in lung parenchyma in the group without MV ().
The IOD value, representing the intensity of expression of EpCAM, and the AOP value, presenting the area of positive EpCAM staining, were measured using IPP 6.0. The IOD increased markedly in the MV group compared with the no MV group (P<0.01). In addition, the AOP was larger in the MV group than in the no MV group (P<0.01; ).
MV increases macrophage infiltrates in lung parenchyma and lung metastatic nodules in the metastatic 4T1 breast cancer model
CD68 (macrophage marker) immunohistochemical staining of lung sections showed increased macrophage infiltration in the lung parenchyma of MV-treated mice when compared to the without MV-treated mice (), indicating that MV altered the microenvironment of the lung by triggering a host inflammatory response. In addition, we found that macrophages in the lung metastatic nodules were dramatically enhanced following surgical removal of the primary tumor with MV than without MV (), suggesting that macrophages also play an important role in this increased metastatic growth.
Figure 4 (A–D) Representative images of immunohistochemical staining of macrophages (CD68+) in lung tissue of BALB/c mice. In the control group (28 d after 5×105 4T1 cells were injected into the mammary fat pad, untreated with MV during surgical procedures, at 14 d after inoculation, Protocol B). CD68 expression was mainly detected in the cytoplasm. Positive staining is indicated by brown particles. (A) No CD68-positive macrophages were found in the lung parenchyma and (C) only scattered CD68-positive macrophages exist in the metastatic nodule. Bar: 50 µm. In the MV-treated group, 28 d after 5×105 4T1 cells were injected into the mammary fat pad, treated with MV during surgical procedures, and at 14 d after inoculation (Protocol B). (D) High infiltration of CD68-positive macrophages in metastatic nodules and (B) CD68-positive macrophages were also found in surrounding lung parenchyma. Bar: 50 µm. (E) Quantification of macrophages in lung tissue using CD68 antibody by immunohistochemical staining. MV exposure resulted in a significant increase in parenchymal cells that stained positive for CD68 when compared to controls. Data presented as mean±SD, n=4. **Statistical significance, P<0.01. Magnifications A-B 20x, and C-D 40x.
Abbreviations: d, days; h, hours; MV, mechanical ventilation.
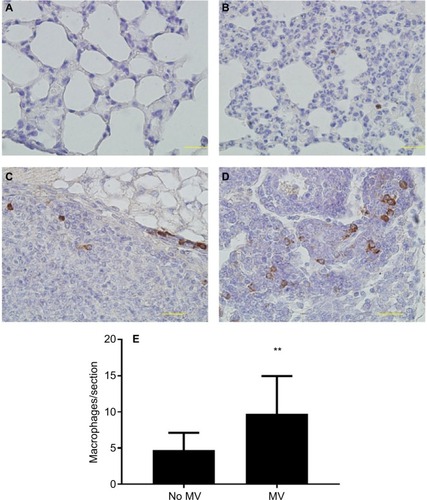
Discussion
The tumor-metastasis-enhancing effect of general anesthesia has been previously demonstratedCitation5–Citation7; however, the mechanisms by which general anesthesia increases tumor growth have remained unclear.Citation7–Citation9 Recent reports have shown that pulmonary inflammation and the peripheral immune system can be modified by MV.Citation10–Citation12 This led us to hypothesize that MV during general anesthesia may have a direct effect on lung metastasis. Results from this current study have demonstrated that MV promoted lung metastasis on three aspects. First, we demonstrated that MV promotes tumor establishment during the process of pulmonary metastasis by attracting the circulating 4T1 cells to the site of local inflammation and tissues with lung damage induced by MV. Second, the most important clinically relevant finding was that MV promotes postoperative lung metastases in the 4T1 primary tumor model of spontaneous breast cancer lung metastasis. Third, analysis of CD68 (macrophage marker) and EpCAM expression after MV demonstrated increased infiltration of macrophages within the metastatic tumor and increased EpCAM expression in lung metastases and surrounding lung tissues.
To our knowledge, this is the first report showing an association between MV and the development of lung metastases. Tumor cells require a favorable microenvironment to survive and proliferate at distant sites. Cell–cell interactions among tumor cells, platelets, leukocytes, and endothelial cells have been shown to contribute to enhanced tumor cell survival and to the facilitation of metastasis.Citation13–Citation16 This study demonstrates that MV modulated the pulmonary microenvironment by disrupting vascular integrity, altering vascular endocrine morphology, triggering an inflammatory response, and, then, this altered microenvironment dictates to extravasate, survive, and ultimately facilitate breast cancer cell proliferation in the lung. These findings support the concept that structural disruption of endothelial-capillary permeability and exposure of the basal membrane matrix increases cancer cell attachments after MV.Citation17,Citation18
We were interested in examining the modulation of EpCAM expression because it has been implicated in multiple cellular functions including cell adhesion, nuclear signaling, migration, proliferation, and regeneration in normal and inflammatory tissuesCitation26,Citation29 and has been shown to promote cancer progression, invasion, and metastasis.Citation26–Citation30 EpCAM, a cell–cell adhesion molecule, is typically overexpressed in a variety of epithelial cancers, for example, breast carcinomas.Citation30 Normal epithelial cells express EpCAM at variable, but generally lower, levels in situ at intercellular basolateral interfaces than in carcinoma cells. Active proliferation in a number of epithelial tissues is associated with elevated EpCAM expression levels.Citation29 Therefore, we suppose that EpCAM plays a crucial role in the breast cancer lung metastatic cascade after MV. In this study, we demonstrated that the increase in metastatic burden coincided with 4T1 tumor cell EpCAM overexpression within lung metastases, and increased EpCAM expression in epithelial cells has also been found in surrounding lung parenchyma in the MV group. Overexpression of EpCAM has been found to be associated with enhanced transcription and translation of the proto-oncogene c-myc.Citation34 Moreover, it is known that EpCAM stimulates the inflammatory process by activating and attracting macrophages, which can promote tumor invasion.Citation35 EpCAM seems to be involved in the increased metastatic growth after MV in this study.
EpCAM is a type I transmembrane glycoprotein with its N-terminus facing the outer cellular space, extracellular domain (EpEx), single-transmembrane domain, and with the C-terminus directed to the cytoplasm, intracellular domain (EpICD). EpCAM-mediated proliferative signaling has been linked to proteolytic cleavage, RIP. After RIP, EpICD is released in the cytoplasm and shuttles into the cell nucleus on forming a complex with β-catenin to participate in upregulation of EpCAM gene transcription.Citation26,Citation27 Furthermore, shedding of EpEx has been shown to contribute to cancer progression by acting as modulator of cell adhesion, through inducing proteolytic activity and facilitating cell signaling.Citation35
The TACE/ADAM17 is a metalloprotease that has been reported to initiate EpCAM cleavage at the extracellular site.Citation35 There is increasing evidence that mechanical stress affects the release and activation of metalloprotease.Citation12 The increased level of metalloprotease in injured tissue induced by MV appears to be involved in the regulation of EpCAM signaling of 4T1 and epithelial cells. Furthermore, it is interesting to note that we found increased infiltration of CD68-positive macrophages within the injured lung parenchyma and the metastatic tumor. A number of animal studies have shown that injurious ventilatory strategies are associated with the release of a variety of proinflammatory mediators,Citation24,Citation25 which are important in cell signaling. These proinflammatory mediators, including TNFα and CD44 which have been identified as substrates for RIP, are produced predominantly by activated macrophages and are involved in the upregulation of inflammatory reactions.Citation25,Citation36,Citation37
It has been identified that β-catenin activation induced the expression of EpCAM. The Wnt/β-catenin pathway is activated when a Wnt ligand binds to a seven-pass transmembrane Frizzled (Fz) receptor and its co-receptor and results in cytosolic accumulation of β-catenin, which then translocates to the nucleus and participates in upregulation of EPCAM gene transcription.Citation26,Citation27 Recently, it has been shown that MV increased protein levels of Wnt5a (Wnt ligands) and non-phosphorylated (threonine and serine) β-catenin in lungs. In addition, several studies have reported synthesis of Wnt ligands by macrophages.Citation37,Citation38 It may, therefore, be reasonable to hypothesize that Wnt/catenin signaling plays a crucial role in modulating EpCAM expression following MV.
Accordingly, lung-protective strategies should be carried out in breast cancer patients during surgery. Novel strategies per-operation may include approaches aimed not only at decreasing pressures or volumes during MV, but also directed at the development of interventions that are aimed directly at preventing the initiation and/or propagation of the inflammatory response. Perioperative usage of nonsteroidal anti-inflammatory drugs to inhibit matrix metalloproteinasesCitation33 and inhaled hydrogen can act as an antioxidant and may be useful to reduce MV-associated inflammatory responsesCitation39; prophylactic inhalation of anti-inflammatory cytokines and pretreatment with intratracheal anti-TNF-α or anti-EpCAM antibody may reduce postoperative cancer metastasis and improve survival.Citation26,Citation40
Conclusion
The present results are significant in at least two major aspects. First, the present findings strongly indicate that lung injury inflammation after MV can alter the micro-environmental conditions within the lung to provide a favorable environment for metastasis. Second, MV-induced lung metastasis occurs by attracting the circulating tumor cells to the site of the lung injury and by accelerating the proliferation of preexisting micro-metastases in the lung. These observations indicate that the metastasis-enhancing effect of MV should be considered, which may provide novel strategies per-operation to prevent postoperative cancer metastasis.
Abbreviations
4T1 cells | = | mouse mammary carcinoma cell line |
AOP | = | area of positive staining |
BSA | = | bovine serum albumin |
BSA-TTBS | = | TBS containing 0.1% Triton X-100 |
EpCAM | = | epithelial cell adhesion molecule |
EpEx | = | EpCAM extracellular domain |
EpICD | = | EpCAM intracellular domain |
FBS | = | fetal bovine serum |
GDN | = | glucose-diaminobenzidine-nickel |
GFP | = | green fluorescent protein |
H&E | = | hematoxylin and eosin |
IgG | = | immunoglobulin G |
IL | = | interleukin |
IOD | = | integrated optical density |
IPP | = | Image-Pro Plus |
MV | = | mechanical ventilation |
PBS | = | phosphate-buffered saline |
RIP | = | regulated intramembrane proteolysis |
SD | = | standard deviation |
TACE | = | TNFα converting enzyme |
TNFα | = | tumor necrosis factor alpha |
TBS | = | Tris-buffered saline |
Acknowledgments
This work was supported in part by an international research grant from the China-Japan Friendships Hospital and China-Japan Friendships Clinical Research Institute. Dr. Yinglai Huang is the recipient of an international research staff exchange foundation from the Swedish Society of Medicine. The authors are thankful to Professors Tiande Zhao and Zhihua Chen for their valuable assistance and advice during this project. The authors are also indebted to Weiliang Sun and Jing Gou for technical assistance and image analysis.
Disclosure
The authors report no conflicts of interest in this work.
References
- ChiangACMassaguéJMolecular basis of metastasisN Engl J Med20083592627522823
- PeachGKimCZacharakisEPurkayasthaSZiprinPPrognostic significance of circulating tumour cells following surgical resection of colorectal cancers: a systematic reviewBr J Cancer201010291327133420389297
- GoldfarbYBen-EliyahuSSurgery as a risk factor for breast cancer recurrence and metastasis: mediating mechanisms and clinical prophylactic approachesBreast Dis20062619911417473369
- AslaksonCJMillerFRSelective events in the metastatic process defined by analysis of the sequential dissemination of subpopulations of a mouse mammary tumorCancer Res1992526139914051540948
- SnyderGLGreenbergSEffect of anaesthetic technique and other perioperative factors on cancer recurrenceBr J Anaesth2010105210611520627881
- SesslerDIDoes regional analgesia reduce the risk of cancer recurrence? A hypothesisEur J Cancer Prev200817326927218414199
- SantamariaLBSchifillitiDLa TorreDFlodaleVDrugs of anaesthesia and cancerSurg Oncol2010192638119394815
- KurosawaSKatoMAnesthetics, immune cells, and immune responsesJ Anesth200822326327718685933
- TavareANPerryNJBensonanaLLTakataMMaDCancer recurrence after surgery: direct and indirect effects of anesthetic agentsInt J Cancer201213061237125021935924
- LionettiVRecchiaFARanieriVMOverview of ventilator-induced lung injury mechanismsCurr Opin Crit Care2005111828615659950
- HalbertsmaFJVanekerMSchefferGJVan der HoevenJGCytokines and biotrauma in ventilator-induced lung injury: a critical review of the literatureNeth J Med2005631038239216301759
- GattinoniLProttiACaironiPCarlessoEVentilator-induced lung injury: the anatomical and physiological frameworkCrit Care Med20103810 Suppl53954819851093
- HartIR“Seed and soil” revisited: mechanisms of site-specific metastasisCancer Metastasis Rev1982115166764375
- FidlerIJThe pathogenesis of cancer metastasis: the “seed and soil” hypothesis revisitedNat Rev Cancer20033645345812778135
- NguyenDXBosPDMassaquéJMetastasis: from dissemination to organ-specific colonizationNat Rev Cancer20099427428419308067
- MathotLStenningerJBehavior of seeds and soil in the mechanism of metastasis: a deeper understandingCancer Sci2012103462663122212856
- MierkeCTRole of the endothelium during tumor cell metastasis: is the endothelium a barrier or a promoter for cell invasion and metastasis?J Biophys2008200818351620107573
- Al-MehdiABTozawaKFisherABShientagLLeeAMuschelRJIntra-vascular origin of metastasis from proliferation of endothelium-attached tumor cells: a new model for metastasisNat Med200261100102
- WeissLWardPMArrest and retention of circulating cancer cells in the lungs of animals with defined metastatic statusCancer Res1982425189819037066901
- CoussensLMWerbZInflammation and cancerNature2002420691786086712490959
- OrrFWAdamsonIYYoungLPromotion of pulmonary metastasis in mice by bleomycin-induced endothelial injuryCancer Res19864628918972416435
- VanekerMHalbertsmaFJvan EgmondJMechanical ventilation in healthy mice induces reversible pulmonary and systemic cytokine elevation with preserved alveolar integrity: an in vivo model using clinical relevant ventilation settingsAnesthesiology2007107341942617721244
- VanekerMJoostenLAHeunksLMLow-tidal-volume mechanical ventilation induces a toll-like receptor 4-dependent inflammatory response in healthy miceAnesthesiology2008109346547218719444
- PuginJDunnIJollietPActivation of human macrophages by mechanical ventilation in vitroAm J Physiol19982756 Pt 1L1040L10509843840
- FrankJAWrayCMMcAuleyDFSchwendenerRMatthayMAAlveolar macrophages contribute to alveolar barrier dysfunction in ventilator-induced lung injuryAm J Physiol Lung Cell Mol Physiol20062916L1191L119816877636
- van der GunBTMelchersLJRuitersMHde LeijLFMcLaughlinPMRotsMGEpCAM in carcinogenesis: the good, the bad or the uglyCarcinogenesis201031111913192120837599
- MaetzelDDenzelSMackBNuclear signalling by tumour-associated antigen EpCAMNat Cell Biol200911216217119136966
- MunzMBaeuerlePAGiresOThe emerging role of EpCAM in cancer and stem cell signalingCancer Res200969145627562919584271
- MartowiczASeeberAUntergasserGThe role of EpCAM in physiology and pathology of the epitheliumHistol Histopathol201631423925526507835
- SpizzoGFongDWurmMEpCAM expression in primary tumour tissues and metastases: an immunohistochemical analysisJ Clin Pathol201164541542021415054
- MisteliTSpectorDLApplications of green fluorescent protein in cell biology and biotechnologyNat Biotechnol199715109619649335045
- DuPréSARedelmanDHunterKWJrThe mouse mammary carcinoma 4T1: characterization of the cellular landscape of primary tumours and metastatic tumour fociInt J Exp Pathol200788535136017877537
- GlasnerAAvrahamRRosenneEImproving survival rates in two models of spontaneous postoperative metastasis in mice by combined administration of a beta-adrenergic antagonist and a cyclooxygenase-2 inhibitorJ Immunol201018452449245720124103
- MünzMKieuCMackBSchmittBZeidlerRGiresOThe carcinoma-associated antigen EpCAM upregulates c-myc and induces cell proliferationOncogene200423345748575815195135
- van KilsdonkJWvan KempenLCvan MuijenGNRuiterDJSwartGWSoluble adhesion molecules in human cancers: sources and fatesEur J Cell Biol201089641542720227133
- ZhangYLiuGDullROSchwartzDEHuGAutophagy in pulmonary macrophages mediates lung inflammatory injury via NLRP3 inflammasome activation during mechanical ventilationAm J Physiol Lung Cell Mol Physiol20143072L173L18524838752
- Cosín-RogerJOrtiz-MasiáDCalatayudSM2 macrophages activate WNT signaling pathway in epithelial cells: relevance in ulcerative colitisPLoS One2013810e7812824167598
- VillarJCabreraNEValladaresFActivation of the Wnt/β-catenin signaling pathway by mechanical ventilation is associated with ventilator-induced pulmonary fibrosis in healthy lungsPLoS One201169e2391421935365
- HuangCSKawamuraTLeeSHydrogen inhalation ameliorates ventilator-induced lung injuryCrit Care2010146R23421184683
- FanEVillarJSlutskyASNovel approaches to minimize ventilator-induced lung injuryBMC Med2013118523536968