Abstract
Background
Factors limiting exercise in patients with COPD are complex. With evidence for accelerated pulmonary vascular aging, destruction of alveolar–capillary bed, and hypoxic pulmonary vasoconstriction, the ability to functionally expand surface area during exercise may become a primary limitation.
Purpose
To quantify measures of alveolar–capillary recruitment during exercise and the relationship to exercise capacity in a cohort of COPD patients.
Methods
Thirty-two subjects gave consent (53% male, with mean ± standard deviation age 66±9 years, smoking 35±29 pack-years, and Global Initiative for Chronic Obstructive Lung Disease (GOLD) classification of 0–4: 2.3±0.8), filled out the St George’s Respiratory Questionnaire (SGRQ) to measure quality of life, had a complete blood count drawn, and underwent spirometry. The intrabreath (IB) technique for lung diffusing capacity for carbon monoxide (IBDLCO) and pulmonary blood flow (IBQc, at rest) was also performed. Subsequently, they completed a cycle ergometry test to exhaustion with measures of oxygen saturation and expired gases.
Results
Baseline average measures were 44±21 for SGRQ score and 58±11 for FEV1/FVC. Peak oxygen consumption (VO2) was 11.4±3.1 mL/kg/min (49% predicted). The mean resting IBDLCO was 9.7±5.4 mL/min/mmHg and IBQc was 4.7±0.9 L/min. At the first workload, heart rate (HR) increased to 92±11 bpm, VO2 was 8.3±1.4 mL/kg/min, and IBDLCO and IBQc increased by 46% and 43%, respectively, compared to resting values (p,0.01). The IBDLCO/Qc ratio averaged 2.0±1.1 at rest and remained constant during exercise with marked variation across subjects (range: 0.8–4.8). Ventilatory efficiency plateaued at 37±5 during exercise, partial pressure of mix expired CO2/partial pressure of end tidal CO2 ratio ranged from 0.63 to 0.67, while a noninvasive index of pulmonary capacitance, O2 pulse × PetCO2 (GxCap) rose to 138%. The exercise IBDLCO/Qc ratio was related to O2 pulse (VO2/HR, r=0.58, p<0.01), and subjects with the highest exercise IBDLCO/Qc ratio or the greatest rise from rest had the highest peak VO2 values (r=0.65 and 0.51, respectively, p<0.05). Of the noninvasive gas exchange measures of pulmonary vascular function, GxCap was most closely associated with DLCO, DLCO/Qc, and VO2 peak.
Conclusion
COPD patients who can expand gas exchange surface area as assessed with DLCO during exercise relative to pulmonary blood flow have a more preserved exercise capacity.
Introduction
Exercise stresses our physiological reserve by increasing muscular contraction and the demand for blood flow and ventilation to maintain gas exchange.Citation1 As cardiac output rises, the typically compliant pulmonary vascular bed expands to increase lung surface area while at the same time retaining a low pressure system for the efficient forward blood flow. In health, this expansion of the alveolar–capillary layer results in a nearly linear rise in lung diffusing capacity for carbon monoxide (DLCO) with cardiac output (Q) as exercise intensity increases.Citation2
In patients with COPD, as destruction of the alveolar–capillary bed occurs, areas of ventilation and perfusion mismatch create hypoxic constriction of pulmonary vessels. Furthermore, airway obstruction increases intrathoracic pressure influencing venous return to the right side of the heart.Citation3 Remodeling of the pulmonary capillaries may also occur resulting in stiffer vessels. Thus, as the disease pathophysiology progresses, the process limits the ability of the alveolar–capillary bed to expand and potentially not only limits the rise in gas exchange surface area but also creates an impediment to forward blood flow or cardiac output, which could ultimately contribute to exercise intolerance in this population.
Physiology of exercise in COPD has been a topic of great interest. Previous work by PotterCitation3 suggested that the degree of airway obstruction during exercise may result in a blunted rise in cardiac output presumably due to the large rise in expiratory intrathoracic pressures, while Montes de OcaCitation4 demonstrated an association of oxygen pulse, a surrogate for stroke volume, with exercise capacity in COPD patients. Similarly, FujiCitation5 suggested that a higher slope of change in pulmonary arterial pressure relative to the change in Q was associated with a reduced exercise capacity. We and others have previously demonstrated that resting DLCO is predictive of exercise capacity in a COPD population and more significantly so when accounting for the resting pulmonary blood flow (Qc), or essentially the functional lung surface area for gas exchange relative to cardiac output.Citation6 The DLCO is highly dependent on Q, and to date, most studies suggest a strong linear relationship between the two with no evidence of a DLCO plateau during heavy exercise even in highly fit individuals.Citation7 Since a rise in cardiac output is the main determinant of pulmonary capillary recruitment, the DLCO relationship relative to Q gives a good overall estimate of the ability to expand this vascular bed.
Therefore, the focus of this study was to quantify DLCO at rest and during exercise using the intrabreath method in a moderate-to-severe COPD population (functional alveolar–capillary recruitment). We hypothesized that the subjects who could increase DLCO in proportion to the rise in Qc (maintenance of the relationship) would have a more preserved exercise capacity. We preferred the intra-breath over the single-breath DLCO (SBDLCO) technique because the latter is difficult to perform with a 10-s breath hold in dyspneic COPD patients at rest and more so during exercise. The intrabreath technique has been compared to other techniques such as the rebreathe or open circuit and has been validated.Citation8–Citation10
An additional goal of our study was to analyze the relationship of DLCO and Qc to other noninvasive measures of respiratory gas exchange that have been associated with pulmonary vascular function. These included ventilatory efficiency, partial pressure of mix expired CO2 (PeCO2)/partial pressure of end tidal CO2 (PetCO2) ratio, as well as a more novel measure previously associated with pulmonary vascular capacitance in the heart failure population, O2 pulse × PetCO2 (GxCap).Citation11–Citation13
Methods
Ethics and consent
The study, ethics, and consent forms were reviewed and approved by the Western Institutional Review Board (WIRB, study number 1153374).
Subjects
Patients with a history of COPD who were sent for clinical pulmonary function testing (PFT) and/or exercise testing were offered enrollment. Inclusion criteria was a history of moderate-to-severe COPD using the Global Initative for Chronic Obstructive Lung Disease (GOLD) criteria. Exclusion criteria included an inability to exercise, dependence on home oxygen therapy, or a body mass index (BMI) >42. Prior to participation, the study goals and requirements were reviewed with the patients. If willing to participate, subjects signed informed consent.
Overview of study
After reporting to the outpatient clinic, study participants filled out the St George’s Respiratory Questionnaire (SGRQ) to measure quality of life (QOL) and performed PFTs which included resting measures of maximal lung volumes and flow rates using classical spirometry.Citation14,Citation15 In addition, the assessment of DLCO was obtained using the classical SBDLCO technique and was also obtained using the intrabreath DLCO method (IBDLCO) which also included a measure of Qc.Citation16 A small blood sample was obtained prior to testing for assessment of hemoglobin in order to correct the measures of DLCO. Subjects subsequently performed cardiopulmonary exercise testing (CPET) using the CareFusion metabolic cart (San Diego, CA, USA) on a semi-recumbent cycle ergometer (Lode, Groningen, the Netherlands). The test protocol started with 20 W for both men and women and increased by 10 W every 2 min. Prior to exercise testing, subjects were instrumented with a 12-lead ECG and a forehead pulse oximeter for peripheral arterial oxygen saturation (SaO2) for continuous monitoring. Subjects wore a nose clip and breathed on a mouthpiece for measurement of gas exchange during the exercise test. During the last 30 s of each workload, a 12-lead ECG was recorded, blood pressure (BP) was assessed, and an average of the heart rate (HR) and SaO2 over this period was determined. We assessed the patient dyspnea by the Borg scale (on the 0–10 scale) and the total body effort by the rated perceived exertion (RPE) score (on the Borg 6–20 scale). Subjects were encouraged to exercise to near exhaustion by achieving an RPE of 17–18 or a dyspnea score $7. Upon reaching peak exercise, subjects performed active recovery where they continued to pedal with no resistance and remained on the mouthpiece for 1 min. After this, the subjects stopped pedaling and were given time for HR and BP to return to baseline before being dismissed.
Pulmonary function and single-breath DLCO
Spirometry was performed using pneumotachograph-based pulmonary function equipment by CareFusion that had passed evaluation using 24 waveforms recommended by the American Thoracic Society (ATS). Classic resting SBDLCO was determined using CareFusion instrument following the recommendations of the ATS/ERS.Citation17,Citation18
Intrabreath lung diffusing capacity and Qc
Qc and DLCO were measured using inert and soluble gases on the CareFusion Vmax system using the intrabreath method.Citation16,Citation19 For this method, subjects were asked to breathe on a mouthpiece while wearing a nose clip. Subjects were instructed to exhale to near residual volume (RV) and then were switched into an inspiratory reservoir and took a full inhalation of a test gas mixture containing 0.3% carbon monoxide (CO), 0.3% methane, 0.3% acetylene, 21% O2, and balance N2. Subjects were trained to exhale at a steady rate until they were back near RV. From the rate of disappearance of CO and acetylene in comparison with the inert gas methane, the rate of disappearance of CO and acetylene was determined. This rate of disappearance of CO was used to calculate IBDLCO. Since acetylene does not bind to hemoglobin, the rate of its disappearance is limited primarily by the flow of blood through the lungs, thereby providing a measure of Qc.Citation16,Citation20 The measure of IBDLCO and IBQc was practiced several times at rest in each subject until reproducible values were obtained and performed near the end of the first workload in triplicate. If necessary, the first workload was extended before incrementing the cycle ergometer resistance in order to obtain reproducible measures.
QOL Questionnaires – SGRQ
The SGRQ is a 50-item questionnaire developed to measure health status (QOL) in patients with diseases causing airway obstruction. Scores are calculated for three domains: symptoms, activity, and impacts (psychosocial) as well as a total score. Psychometric testing has demonstrated its repeatability, reliability, and validity. Sensitivity has been demonstrated in clinical trials.Citation14 Each patient was asked to fill out the SGRQ questionnaire prior to CPET.
Gas exchange, ventilation, and lung mechanics
During exercise testing, oxygen consumption (VO2), carbon dioxide production (VCO2), breathing frequency (fb), tidal volume (Vt), minute ventilation (VE), and derived variables were measured continuously and/or calculated using a low-resistance open circuit automated metabolic system (CareFusion).
In addition, measures associated with ventilation and perfusion matching or pulmonary vascular health were determined. One of the measures was PeCO2 relative to PetCO2 which is associated with ventilation and perfusion matching in the lungs and reported to be reduced in COPD patients having pulmonary hypertension with a negative slope during exercise. The other gas exchange measure was GxCap which is a noninvasive estimate of pulmonary vascular capacitance calculated as oxygen pulse multiplied by PetCO2. Oxygen pulse tracks stroke volume and PetCO2 has been shown to reasonably track pulmonary arterial pressure. The last of the measures was ventilatory efficiency or VE/VCO2.Citation11,Citation13
Statistics
We were interested in exercise limitation in the COPD population and the potential role of the alveolar–capillary bed, the lung surface area for gas exchange, or the ability to expand this bed as a mediating factor. Thus, our primary measures were the change in IBDLCO and the change in IBDLCO in proportion to IBQc across subjects and the relationship to exercise or aerobic capacity – primarily peak VO2. Thus, we used multiple regression and correlational analysis to asses these relationships. In addition, general descriptive statistics were used to define our group and paired t-tests were used to further analyze changes with exercise.
Results
Subject characteristics and resting pulmonary function
Thirty-two subjects completed all aspects of the study (age 66±9 years, 53% male, smoking history 35±29 pack-years, BMI 30±6 kg/m2; mean ± SD). The study cohort consisted of primarily moderate COPD patients based on the GOLD classification (2.3±0.8), but with some subjects mild and others more severe (GOLD classification range: 1–4). This degree of disease was reflected in the QOL scores (SGRQ averaged 44±21) and medication use with the majority of patients on multiple inhalers and with 20% on oral steroids. None of the subjects were on continuous oxygen at the time of the study. Hemoglobin values were essentially within normal limits and averaged 13.5±1.7 g/dL.
The forced vital capacity (FVC) averaged 2.48±0.69 L or 75% of age and gender predicted, while the forced expiratory volume in 1 s (FEV1) averaged 56%±16% of predicted with an FEV1/FVC ratio of 58%±11%, range 33%–78%. The FEF25–75 averaged 0.75±0.38 L or 26%±13% of predicted. The SBDLCO averaged 13.2±5.5 mL/min/mmHg and 58%±23% of age and gender predicted. Both the SGRQ score and the GOLD classification were somewhat associated with resting DLCO (r values ranging from 0.33 to 0.38).
Cardiopulmonary exercise responses
Cardiopulmonary responses to exercise are shown in . The most common symptoms for stopping exercise were dyspnea, general fatigue, and leg fatigue. Peak work achieved was 43 W with a VO2 peak of 11.4 mL/kg/min or 49% of age and gender predicted. Peak HR was 106 bpm or 70% of age predicted. Tidal volume increased early in exercise but then plateaued as ventilation was primarily rate dependent beyond the first workload. Peak minute ventilation reached 70% of the estimated breathing capacity and inspiratory capacity (IC) fell throughout exercise suggesting expiratory flow limitation and some hyperinflation as exercise progressed. Oxygen pulse rose to 7.8 mL per beat by the first exercise load, but then rose slowly thereafter.
Table 1 Cardiopulmonary responses to exercise in COPD patients (N=32)
Ventilatory efficiency assessed as the VE/VCO2 ratio was elevated at rest and fell with exercise but stayed elevated without a clear exercise nadir. The PeCO2/PetCO2 ratio started at 0.54 at rest and stayed below a ratio of 0.70 throughout exercise. The gas exchange estimate of pulmonary capacitance, GxCap, on average increased 138% from rest to peak exercise but plateaued or declined in approximately half (47%) of the subjects over the last two workloads.
Measures of DLCO and pulmonary blood flow
The changes in DLCO, Qc, stroke volume, and DLCO relative to Qc are shown in . Individual changes in DLCO and DLCO/Qc from rest to the first workload are shown in and . On average, DLCO increased 45% from rest to the first workload. However, there was large variation in responses with three subjects demonstrating >10% fall in DLCO and another four subjects demonstrating minimal change with exercise. Pulmonary blood flow increased on average 40% during the first workload, while stroke volume increased 18%. The mean IBDLCO relative to Qc (DLCO/Qc) stayed relatively constant with exercise increasing on average 14% from rest to the first workload while the majority of subjects demonstrated a rise in this ratio, 12 of the subjects had no change or had a fall, suggesting Qc was increasing out of proportion to lung surface area for gas exchange in these subjects.
Table 2 Intrabreath lung diffusing capacity and pulmonary blood flow at rest and during exercise in COPD patients (N=32)
Respiratory measures associated with lung diffusion
We were interested in the relationship of other noninvasive indices associated with pulmonary vascular function and lung diffusion during exercise. Ventilatory efficiency for carbon dioxide, (VE/VCO2) (r=−0.44), the mixed expired-to-end tidal CO2 ratio, (PeCO2/PetCO2) (r=0.40), and GxCap were all associated with the exercise IBDLCO measure (p<0.05). However, the GxCap was the gas exchange measure mostly associated with exercise IBDLCO () (r=0.71).
Relationship of lung diffusion, pulmonary blood flow, and indices of pulmonary vascular function to exercise capacity
The exercise IBDLCO and change from rest in the IBDLCO were only marginally associated with VO2 peak (r=0.43 and 0.39, p<0.05), while the relationship of exercise DLCO/Qc or the change in DLCO/Qc to VO2 peak was more strongly related (r=0.63 and 0.54, respectively, p<0.01). shows the relationship of VO2 peak and VCO2 peak with DLCO/Q. The modest correlation with these measures suggests that subjects with the greatest recruitment in lung gas exchange surface area relative to pulmonary blood flow were the subjects with the best exercise tolerance. With multiple regression including IBDLCO, the change in IBDLCO and DLCO/Qc, the DLCO/Qc from rest to exercise was the measure most significantly associated with VO2 peak. DLCO/Qc was also associated with O2pulse (r=0.63). Furthermore, exercise capacity was associated with the PeCO2/PetCO2 ratio with those with higher ratios having better exercise tolerance (r=0.57 between PeCO2/PetCO2 vs VO2 peak), while both the absolute measure of GxCap during exercise and the change from rest to peak exercise being associated with VO2 peak (r=0.53 and 0.66, respectively, p<0.01, ). The lung function measure most associated with DLCO or DLCO/Qc during exercise was the exercise IC (r=0.58 and 0.63, respectively, p<0.01, ).
Figure 4 Relationship of VO2 peak (A) and VCO2 peak (B) with DLCO/Qc.
Abbreviations: DLCO, diffusing capacity for carbon monoxide; Qc, pulmonary blood flow; VCO2, carbon dioxide production; VO2, oxygen consumption.
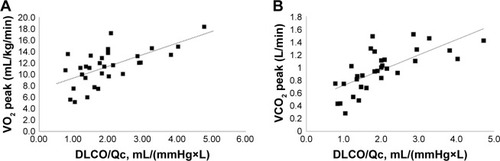
Discussion
Primary findings
Our primary finding was that COPD patients who can increase respiratory gas exchange surface area (ie, alveolar–capillary reserve – as assessed by DLCO) relative to the rise in Qc have the most preserved exercise capacity. In addition, the ability to expand the pulmonary vascular bed is associated with noninvasive indices of pulmonary capacitance as well as with IC assessed during exercise. While these data are correlative, this implies that a contributing factor to exercise intolerance in this population may be the inability to recruit pulmonary capillaries.
Pulmonary vasculature in COPD
The majority of our patients had a history of smoking as the likely primary etiology of their airway disease. Years of exposure to inhalants causes destruction and remodeling of the alveolar–capillary bed, accelerated aging, inflammation, and other chronic metabolites that circulate to cause a general vasculopathy and stiffening of vessels.Citation21–Citation23 There are often regions of inhomogeneous pulmonary vasoconstriction due to the ventilation and perfusion abnormalities. All of these factors contribute to a blunted ability to increase or expand the alveolar–capillary bed. Previous work has suggested thatCitation5 an abnormal rise in pulmonary arterial pressure relative to the rise in cardiac output was associated with reduced exercise performance in COPD patients and presumably a resistance to forward flow through the lungs – thus a form of cardiac output limitation.Citation5 However, VO2 peak was not related to the degree of airflow obstruction in this study which is an interesting finding. Other studies have also suggested a strong relationship between exercise capacity and oxygen pulse, a surrogate for stroke volume.Citation4 In addition, airway obstruction itself causes an increase in intrathoracic pressure that may act as an impediment to venous return – potentially further influencing cardiac output.Citation3 While we did not directly measure hemodynamics, there is typically a strong linear relationship between DLCO and Q in healthy individuals showing a steady and proportional increase with moderate-to-heavy exercise. Presumably, this represents recruitment and distension of the pulmonary capillaries. Since DLCO is flow independent, a rise in DLCO is primarily influenced by the expansion of this bed. Thus, our measure of DLCO/Qc would primarily represent a rise in pulmonary capillary blood volume that is in contact with functional alveoli. While, on average, DLCO did rise with exercise and DLCO relative to Qc (DLCO/Qc) stayed relatively constant, there were a large number of subjects (38%) where this ratio plateaued or fell with exercise, suggesting that as pulmonary blood flow increased, there was a non-proportional increase in gas exchange surface area.Citation24
DLCO relative to other respiratory gas exchange measures in COPD
We also assessed several other reported respiratory gas exchange measures associated with ventilation and perfusion matching and pulmonary vascular function. Ventilatory efficiency (VE/VCO2), the partial pressure of CO2 relative to end tidal CO2 (PeCO2/PetCO2), and an index of pulmonary vascular capacitance (GxCap) are all associated with lung diffusing capacity.Citation13 Ventilatory efficiency is commonly reported elevated in patients with pulmonary vascular disease and is associated with high dead space ventilation, hyperventilation, and a rapid shallow breathing pattern.Citation25 The PeCO2/PetCO2 ratio and the slope of change from rest to exercise have been suggested to differ between primarily pulmonary vascular disease versus conditions of more ventilation and perfusion mismatch, with lower ratios suggesting worsening disease. Furthermore, GxCap has previously been shown to correlate well with invasive measures of pulmonary vascular capacitance.Citation13 It is interesting that while all these measures were associated with DLCO, the GxCap measure was the most significantly linked both to DLCO and to peak exercise performance. The premise is that oxygen pulse tends to track stroke volume changes, while PetCO2 tends to fall with greater pulmonary vascular pressures in conditions like pulmonary hypertension – thus a surrogate for pressure. Thus, since the DLCO is essentially dependent on the recruitment and distension of the pulmonary capillaries, it essentially should increase with greater pulmonary vascular capacitance. While both increased with exercise, there were a significant number of subjects where these rose minimally or failed to increase.
Previous studies looking at exercise intolerance in COPD
A number of studies have demonstrated a clear impact of airway obstruction on exercise performance.Citation26–Citation28 However, as the disease progresses, it clearly becomes a systemic disease impacting many organ systems. Previous work by O’Donnell has demonstrated an association with the degree of hyperinflation and that improvement of obstruction with bronchodila-tors clearly enhances exercise capacity.Citation29 In addition, muscle dysfunction, muscle wasting, and deconditioning also begin to develop.Citation30,Citation31 It is clear that COPD is not a homogeneous disease, and thus, it is likely that multiple issues contribute to the loss of exercise capacity. Our study highlights the importance of the pulmonary vasculature in determining exercise tolerance in this chronic disease state.
Limitations and conclusion
There are a number of potential study limitations. First, the population was small, and thus, general conclusions are limited. Second, we only assessed DLCO during the first exercise load. While we attempted to measure this at other work levels and got success in some cases, we felt confident only in our data at the lower work level. Subjects were trained to perform the maneuver reproducibly at rest and typically performed three reproducible maneuvers at the lower workloads, but struggled to perform them reproducibly at the higher work intensities. Thus, we were examining their ability to recruit lung alveolar–capillary surface area with relatively modest workloads and comparing these data to their peak aerobic capacity. However, the DLCO response to exercise should be relatively linear with workload, VO2, and cardiac output, and thus, the ability to expand the pulmonary circulation early in exercise is likely representative of their reserve with heavier activity. The first workload also represented on average nearly 75% of peak VO2 and thus would be considered a moderately heavy load for this population. Third, we did not have direct measures of pulmonary vascular pressures to better understand the relationships between pulmonary blood flow, pulmonary pressure, and the rise in DLCO which would have given better insight into what was limiting the alveolar–capillary expansion. Finally, there is a baseline degree of deterioration in the alveolar–capillary bed in the COPD population. While DLCO increase is predictive of the ability to exercise more, it does not fully explain the exercise response. Therefore, additional studies are needed to better understand regulating factors in exercise that might improve functional alveolar–capillary surface area.
Acknowledgments
The authors thank David Sinks and Beth Anke from CareFusion for their technical support and also Salome Ilkhan, Angie Holland, and other staff in the Cardiopulmonary Exercise lab in Augusta for their assistance in testing and scheduling the patients.
This work was presented as a poster at the CHEST 2016: American College of Chest Physicians Annual Meeting, October 22–26, Los Angeles, CA, USA.
Disclosure
The authors report no conflicts of interest in this work.
References
- DempseyJAJ.B. Wolffe memorial lecture. Is the lung built for exercise?Med Sci Sports Exerc19861821431553517547
- RampullaCMarconiCBeulckeGAmaducciSCorrelations between lung-transfer factor, ventilation, and cardiac output during exerciseRespiration19763364054151005935
- PotterWAOlafssonSHyattREVentilatory mechanics and expiratory flow limitation during exercise in patients with obstructive lung diseaseJ Clin Invest19715049109195547281
- Montes de OcaMRassuloJCelliBRRespiratory muscle and cardiopulmonary function during exercise in very severe COPDAm J Respir Crit Care Med19961545128412898912737
- FujiiTKuriharaNFujimotoSHirataKYoshikawaJRole of pulmonary vascular disorder in determining exercise capacity in patients with severe chronic obstructive pulmonary diseaseClin Physiol19961655215338889315
- BehniaMWheatleyCAvolioAJohnsonBInfluence of resting lung diffusion on exercise capacity in patients with COPDBMC Pulm Med201717111728841877
- TamhaneRMJohnsonRLJrHsiaCCPulmonary membrane diffusing capacity and capillary blood volume measured during exercise from nitric oxide uptakeChest200112061850185611742912
- CottonDJPrabhuMBMinkJTGrahamBLEffect of ventilation inhomogeneity on “intrabreath” measurements of diffusing capacity in normal subjectsJ Appl Physiol (1985)19937529279328226498
- HuangYCO’BrienSRVredenburghJFolzRJMacintyreNRIntrabreath analysis of carbon monoxide uptake during exercise in patients at risk for lung injuryRespir Med200610071226123316314083
- SnyderEMJohnsonBDBeckKCAn open-circuit method for determining lung diffusing capacity during exercise: comparison to rebreatheJ Appl Physiol (1985)20059951985199116024523
- HansenJEUlubayGChowBFSunXGWassermanKMixed-expired and end-tidal CO2 distinguish between ventilation and perfusion defects during exercise testing in patients with lung and heart diseasesChest2007132397798317573506
- TaylorBJJohnsonBDThe pulmonary circulation and exercise responses in the elderlySemin Respir Crit Care Med201031552853820941654
- TaylorBJOlsonTPChul HoKMaccarterDJohnsonBDUse of noninvasive gas exchange to track pulmonary vascular responses to exercise in heart failureClin Med Insights Circ Respir Pulm Med20137536024093002
- MeguroMBarleyEASpencerSJonesPWDevelopment and Validation of an Improved, COPD-Specific Version of the St. George Respiratory QuestionnaireChest2007132245646317646240
- RosenbergEThe 1995 update of recommendations for a standard technique for measuring the single-breath carbon monoxide diffusing capacity (transfer factor)Am J Respir Crit Care Med199615412652668680694
- CharlouxAEnacheIRichardRDiffusing capacity of the lung for CO and pulmonary blood flow during incremental and intermittent exerciseScand J Med Sci Sports2010201e121e12919422637
- BrusascoVCrapoRViegiGAmerican Thoracic Society, European Respiratory SocietyComing together: the ATS/ERS consensus on clinical pulmonary function testingEur Respir J20052611215994380
- MacintyreNCrapoROViegiGStandardisation of the single-breath determination of carbon monoxide uptake in the lungEur Respir J200526472073516204605
- HuangYCO’BrienSRMacIntyreNRIntrabreath diffusing capacity of the lung in healthy individuals at rest and during exerciseChest2002122117718512114355
- MartonenTBWilsonAFTheoretical basis of single breath gas absorption testsJ Math Biol19821422032207119583
- SeegerWAdirYBarberaJAPulmonary hypertension in chronic lung diseasesJ Am Coll Cardiol20136225 SupplD109D11624355635
- SiafakasNMAntoniouKMTzortzakiEGRole of angiogenesis and vascular remodeling in chronic obstructive pulmonary diseaseInt J Chron Obstruct Pulmon Dis20072445346218268919
- VonbankKFunkGCMarzlufBAbnormal pulmonary arterial pressure limits exercise capacity in patients with COPDWien Klin Wochenschr200812023–2474975519122986
- Borghi-SilvaAOliveiraCCCarrascosaCRespiratory muscle unloading improves leg muscle oxygenation during exercise in patients with COPDThorax2008631091091518492743
- SunXGHansenJEOudizRJWassermanKExercise pathophysiology in patients with primary pulmonary hypertensionCirculation2001104442943511468205
- GallagherCGExercise limitation and clinical exercise testing in chronic obstructive pulmonary diseaseClin Chest Med19941523053268088095
- JohnsonBDBadrMSDempseyJAImpact of the aging pulmonary system on the response to exerciseClin Chest Med19941522292468088090
- PepinVSaeyDLavioletteLMaltaisFExercise capacity in chronic obstructive pulmonary disease: mechanisms of limitationCOPD20074319520417729063
- O’DonnellDEWebbKAExertional breathlessness in patients with chronic airflow limitation. The role of lung hyperinflationAm Rev Respir Dis19931485135113578239175
- AlivertiAMacklemPTThe major limitation to exercise performance in COPD is inadequate energy supply to the respiratory and locomotor musclesJ Appl Physiol (1985)20081052749751 Discussion 755–74718678622
- RodriguesFEstudo dos factores limitativos do exercicio fisico em doentes com doenca pulmonar obstrutiva cronica [Limiting factors of exercise capacity in patients with COPD]Rev Port Pneumol2004101961 Portuguese15190427