Abstract
Background and Objectives
Parasternal intercostal ultrasound morphology reflects spirometric COPD severity. Whether this relates to the systemic nature of COPD or occurs in response to hyperinflation is unknown. We aimed to assess changes in ultrasound parasternal intercostal muscle quantity and quality (echogenicity) in response to relief of hyperinflation. We hypothesised that reduction in hyperinflation following endobronchial valve (EBV) insertion would increase ultrasound parasternal thickness and decrease echogenicity.
Methods
In this prospective cohort study, eight patients with severe COPD underwent evaluation of health-related quality of life, lung function, and sonographic thickness of 2nd parasternal intercostal muscles and diaphragm thickness, both before and after EBV insertion. Relationships between physiological and radiographic lung volumes, quality of life and ultrasound parameters were determined.
Results
Baseline FEV1 was 1.02L (SD 0.37) and residual volume (RV) was 202% predicted (SD 41%). Median SGRQ was 63.26 (range 20–70.6). Change in RV (−0.51 ± 0.9L) following EBV-insertion showed a strong negative correlation with change in parasternal thickness (r = −0.883) ipsilateral to EBV insertion, as did change in target lobe volume (−0.89 ± 0.6L) (r = −0.771). Parasternal muscle echogenicity, diaphragm thickness and diaphragm excursion did not significantly change.
Conclusions
Dynamic changes in intercostal muscle thickness on ultrasound measurement occur in response to relief of hyperinflation. We demonstrate linear relationships between intercostal thickness and change in hyperinflation following endobronchial valve insertion. This demonstrates the deleterious effect of hyperinflation on intrinsic inspiratory muscles and provides an additional mechanism for symptomatic response to EBVs.
Plain Language Summary
Parasternal intercostal thickness negatively correlates with COPD severity although contribution of hyperinflation is unclear. We demonstrate strong correlations between reduction in residual volume, reduction in CT-target lobe volume and increased parasternal thickness following endobronchial valve insertion, suggesting reduced parasternal thickness is related to hyperinflation. This provides a novel mechanism for symptomatic improvement post-EBV insertion.
Introduction
Chronic obstructive pulmonary disease (COPD) is characterised by non-reversible airflow limitation,Citation1 predisposing to lung hyperinflation with associated functional impairment. Hyperinflation has been shown to have significant impacts on dyspnoea, related to inability to appropriately increase tidal volumeCitation2–Citation4 and increased neural respiratory muscle drive with exercise.Citation5 Reduction in the degree of hyperinflation with helioxCitation6 and inhaled bronchodilatorsCitation7 during exercise has been shown to improve exercise capacity and dyspnoea. Emphysema and hyperinflation have additionally been shown to have significant impacts on cardiovascular function.Citation8
Additionally, systemic inflammation is prominent in COPD, leading to reductions in peripheral and respiratory (intercostal) muscle bulk and quality.Citation9–Citation11 Such loss of muscle mass in hospitalised patients with COPD independently predicts increased mortality and risk of hospital readmission.Citation12
The parasternal intercostal muscles are purely inspiratory in nature, with contraction resulting in cranial and outward rib displacement.Citation13 We have demonstrated that parasternal intercostal muscle thickness and quality in COPD have high intra- and inter-rater reliability when measured with ultrasound and that changes in these measures (decrease in thickness and increase in echogenicity) correlate with spirometric disease severity.Citation14 The clinical significance of intercostal muscle function is an area of ongoing interest. Augmentation of intercostal blood flow and oxygen delivery in COPD with heliox during exercise results in reduced dyspnoea and respiratory muscle work,Citation15,Citation16 independent of redistribution of blood flow.Citation17 Additionally, failure of EMG-parasternal intercostal muscle drive to improve following COPD exacerbation is associated with increased risk of hospital readmission,Citation18 with elevated EMG activity associated with increased mortality.Citation19 Finally, altered parasternal intercostal muscle morphology in critically ill patients undergoing mechanical ventilation with decreased intercostal and diaphragm thickness is associated with prolonged duration of ventilation and longer ICU stay.Citation20 Parasternal intercostal thickening is increased in patients with diaphragm dysfunction, and this thickening is independently associated with failure of spontaneous breathing trials.Citation21
A key finding in our previous study was that parasternal muscle thickness was reduced in more severe COPD; there are several potential reasons for this finding, which require further exploration. Firstly, there is an established relationship between muscle bulk and COPD severity which reflects the underlying inflammatory nature of COPD, along with general patient factors including reduced physical activity, comorbid medical conditions and medication use.Citation22 Therefore, the reduction in muscle thickness may indicate a more generally unwell patient.
Another potential explanation for diminished parasternal intercostal muscle thickness in COPD is from muscle stretch due to thoracic hyperinflation. This may impair muscle length–tension relationships, and ─ in association with changes in rib displacement during contraction ─ reduce the capacity of parasternal intercostal muscles to effectively generate pressure changes at the airway level.Citation23
In COPD, relief of hyperinflation can be achieved in selected patients via endobronchial valve (EBV) insertion. These one-way valves allow expiratory but prevent inspiratory flow, causing collapse of distal lung segments. These anatomical changes are usually accompanied by improvements in lung function, symptoms and exercise capacity.Citation24–Citation27
To establish ─ under clinical experimental conditions ─ whether hyperinflation contributes significantly to diminished parasternal muscle thickness in COPD, we performed ultrasound measurements before and after therapeutic unilateral EBV insertion to reverse hyperinflation. We aimed to assess whether there would be a demonstrable change in parasternal thickness in patients undergoing this procedure and to assess the relationship between physiological and radiological measures of lung volume and ultrasound indices.
We hypothesised that diminished parasternal intercostal muscle thickness on ultrasound would be reversed following EBV insertion, commensurate with the extent to which hyperinflation was successfully relieved.
Methods
Participants
Respiratory muscle ultrasound was performed on consecutive patients undergoing endobronchial valve placement at the Royal Melbourne Hospital, a university referral centre, prior to and following EBV insertion between 1 January 2017 and 30 June 2018. The baseline data of these patients have been included in a previously published work in a larger cohort.Citation14
The decision to insert EBV was made on the basis of consensus guidelines,Citation28 with patients demonstrating a residual volume (RV) of greater than 150%, with CT-measured fissural completeness, and Chartis® (Pulmonx, Redwood City, CA, USA) performed in the setting of equivocal scans to exclude collateral ventilation. Patients also underwent CT chest scanning post-procedure to assess changes in CT-measured total lung volume. No specific nutritional or physical interventions were performed between scans, of note pulmonary rehabilitation was not undertaken routinely for logistical reasons.
Baseline St George Respiratory Questionnaire (SGRQ),Citation29 short-form 7-day recall International Physical Activity Questionnaire (IPAQ)Citation30,Citation31 and Charlson Comorbidity IndexCitation32,Citation33 were obtained, along with routine physiological data (). Written informed consent was obtained from all patients prior to initial ultrasound with baseline data included in our previously published work,Citation14 and this project received institutional ethics approval (Melbourne Health Human Research Ethics Committee: HREC 2015.296) and was conducted in accordance with the Declaration of Helsinki.
Table 1 Baseline Demographics
Ultrasound Examination
Ultrasound was performed by a single operator (PW) with 8 years of ultrasound experience and qualifications in respiratory ultrasoundCitation14,Citation34 Briefly, B-mode images were obtained utilising a 6–14mHz linear array on a MindRay TE-5 machine (Shenzhen MindRay Bio-Medical Electronics Co. Ltd. Shenzen, China) with the participant at 45 degrees head-up in supine position on bed with standardised depth and gain.
Two still images were taken of the 2nd parasternal intercostal muscle bilaterally in the sagittal plane at end-tidal inspiration with a window visualising the 2nd/3rd ribs respectively () with the mean thickness of 2 measurements utilised for analysis; intercostal muscle echogenicity was analysed using histogram analysis. Additional musculoskeletal ultrasound measurements were taken, including right diaphragm thickness at end inspiration and expiration using B-mode and excursion measured with M-mode.Citation35 The second parasternal intercostal muscle was chosen as this was the most reliable site in our previous work.Citation14 Ultrasound was performed prior to EBV insertion, and approximately eight weeks following EBV insertion, at time of clinical review.
Figure 1 Parasternal intercostal muscle ultrasound.
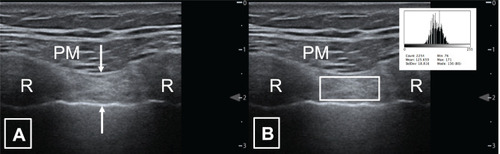
Measurements were performed on stored images using freely available NIH ImageJ software (https://imagej.nih.gov/ij/index.html NIH, Bethesda, MD, USA). Muscle thickness in millimetres was measured between the inner and outermost echogenic layer of the muscle fascial borders for both intercostal and diaphragm muscles. Muscle echogenicity was calculated utilising the square methodCitation36 to define the region of interest for analysis using ImageJ histogram function ().
Statistical Analysis
Data were analysed using GraphPad Prism 7 (GraphPad Software Inc., CA USA). Descriptive statistics were performed utilising parametric tests after testing for normality of distribution with D’Agostino and Pearson normality test. Correlation was measured using Pearson correlation coefficient. P-values were not reported for correlation data given the limited number of subjects. Data are described by mean and standard deviation unless otherwise specified, depending on normality of distribution.
Reporting is in keeping with STROBE guidelines.Citation37
Results
Eight consecutive patients who underwent EBV placement () were included in this study. A ninth also underwent EBV insertion during this period however valves were removed prior to follow-up scanning due to progressive dyspnoea with respiratory failure.
In keeping with published data, there was a range of physiological responsiveness to EBV insertion in our cohort, with regards to airflow obstruction (FEV1), hyperinflation (RV) and quality of life (SGRQ) ().
Table 2 Pre- and Post-Physiological and QoL Results
Overall there was a non-significant increase in FEV1 and fall in RV likely due to the small sample size, with a magnitude of effect similar to published results.Citation26 Unsurprisingly there was also no overall significant improvement in SGRQ (). There was a significant decrease in CT-measured volume of the treated lung (−611.6mL (95% CI −1145 to −78.73) p = 0.03), with a non-significant trend towards increased volume on the non-treated side (+181.6mL (95% CI −50.66 to 413.8mL) p = 0.107). Target lobe atelectasis was achieved in 3 patients, with significant decreases in target lobe volume (−0.887mL (95% CI −1370 to −404.2) p = 0.003).
Intercostal Muscle Changes
There was a strong negative correlation between change in residual volume and parasternal intercostal muscle thickness on the treated side in all participants (r = −0.883 p = 0.004). Additionally, there was a strong positive correlation between change in RV and 2nd parasternal thickness on the non-treated side (r = 0.840 p = 0.009) (). The strength of correlation appeared stronger in those where there was target lobe atelectasis (r = −0.955 and r = 0.945, respectively).
Figure 2 Relationship between change in residual volume, CT-target lobe volume and change in parasternal thickness following endobronchial valve insertion.
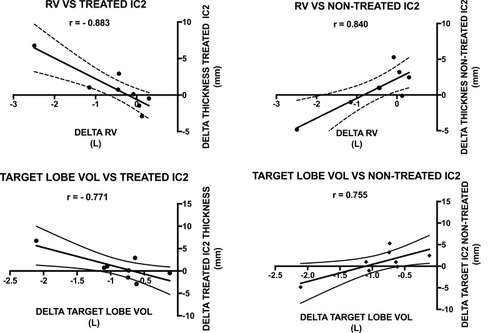
There was a strong negative correlation between change in target lobe volume and change in parasternal intercostal thickness on the treated (r = −0.771 p = 0.025) side, with a strong positive correlation on the non-treated side (r = 0.755 p = 0.03) ().
There was a non-significant correlation between change in overall CT-measured lung volume and parasternal intercostal thickness on the treated side (r = −0.517 p = 0.189) and between change in CT-volume and parasternal thickness on the non-treated side (r = 0.479 p 0.229), although the directionality of relationships was preserved. In those achieving target atelectasis, again there were strong correlation coefficients noted between change in CT-volume and parasternal thickness on the treated and non-treated sides (r = −0.999 and r = 0.975, respectively).
Overall, there was no significant correlation identified between change in parasternal thickness and change in FEV1 for either the treated or non-treated hemithorax (treated side r = 0.42 p = 0.295 and non-treated side r = −0.511 p = 0.195), and no significant relationship between change in CT volume and change in parasternal thickness for either side (treated side r = −0.599, p = 0.117 and non-treated side r = 0.398 p = 0.329).
There was no significant correlation identified between change in intercostal muscle echogenicity and change RV, FEV1, ipsilateral CT or total CT volume.
By participant physiological and ultrasound data are presented in Tables S1 and S2.
Diaphragm Muscle and Physical Activity Changes
There were non-significant moderate negative correlations between change in diaphragm thickness and excursion and change in RV (r = −0.662 p = 0.074 and −0.341 p = 0.409, respectively), with a moderate non-significant positive correlation between change in diaphragm thickness and change in FEV1 (r = 0.524 p = 0.183). There were similar results with regards to change in target lobe volume on CT for thickness (r = −0.659 p = 0.76), with no suggestion of a linear relationship between diaphragm excursion and change in target lobe volume (r = −0.067 p = 0.875).
There was no significant difference between pre-IPAQ raw scores or calculated metabolic equivalents per week and those following EBV insertion.
Discussion
Changes in Intercostal Muscle Thickness
We have demonstrated a significant linear relationship between changes in intercostal muscle thickness and residual volume in patients who have undergone EBV. In the hemithorax treated with EBV insertion, relief of hyperinflation was accompanied by an increase in parasternal muscle thickness, exactly as we hypothesised.
Overall, these experimental results support the hypothesis that diminished parasternal muscle thickness in COPD is at least in significant part due to hyperinflation. This does not exclude the possibility that systemic inflammation and general muscle factors may also play important roles. Patients however had low levels of comorbidity as reflected in age-adjusted Charlson Comorbidity Index scores between 4 and 5, which reflected age and COPD status with no other recorded significant comorbidity.
The mechanism of benefit of endobronchial lung volume reduction is thought to be predominantly due to improved FEV1 following relief of hyperinflation. Limited studies have suggested other factors, including improved cardiac function,Citation38 ventilation-perfusion matchingCitation39 and improved chest wall synchrony.Citation40 Our findings suggest for the first time that improved intercostal muscle function may also contribute to symptomatic improvements. Increased parasternal muscle thickness following EBV insertion is likely accompanied by a restoration of optimal length–tension relationships and improved rib conformation.
It has previously been demonstrated that hyperinflation reduces the ability of both the diaphragm and parasternal intercostal muscles to alter airway opening pressure in a volume-dependent fashionCitation23 with a relative increase in the contribution to negative pressure generation by the intercostal muscles with progressive hyperinflation.Citation41 Our results provide a second mechanistic explanation for the contribution of intercostal muscles toward clinical benefits seen in lung volume reduction.
In the contralateral hemithorax (ie not treated with EBV insertion), we found an unexpected reduction in intercostal muscle thickness, possibly suggesting a reciprocal relationship between changes in rib conformation in each hemithorax in response to increased volume of the contralateral lung. Interestingly, in the study by Zoumot et alCitation40 chest wall synchrony was only significantly improved on the treated hemithorax, which is also in keeping with our findings. This lends further support towards the benefit of optimising muscle length–tension relationships and alteration in the direction of rib displacement related to the interaction between rib displacement and lung volume to improve function.Citation23
The correlations identified between parasternal muscle thickness and changes in RV and target lobe volume were stronger than for changes in diaphragm indices; nevertheless, the relative contributions of each of these muscle groups require further evaluation.
The demonstration of a strong linear relationship between parasternal intercostal thickness and residual volume suggests benefit for ultrasound measurement as a simple, safe and repeatable bedside marker mirroring changes in hyperinflation and gas trapping. Additionally, the demonstration of strong relationships between target lobe volume change and parasternal thickness may allow rapid assessment of changes in response to EBV insertion if replicated in larger cohorts. The greater strength of correlation in those achieving target lobe atelectasis further underlines these results.
Changes in CT Lung Volume
We have demonstrated significant correlations between changes in parasternal thickness and changes in target lobe volume, reflecting the responsiveness of this measure to relief of hyperinflation.
CT-defined lung volume did not show the same strength of correlation with ultrasound indices as physiological measurement of residual volume or target lobe volume changes, although the direction of correlations was consistent. CT scans were performed at deep inspiration which has previously been shown to underestimate TLC by approximately 12%, with expiratory scans (not performed on this occasion) significantly overestimating RV.Citation42 We hypothesise that the non-significant correlation is related to the small sample size, as well as the inherent variability of CT volume measurements related to the lack of standardisation of inspiration. This is supported the fact there was no significant correlation between change in overall CT volume and change in either TLC (r = 0.344 p 0.404) or RV (r = 0.682 p = 0.062) in our dataset.
Changes in Intercostal Muscle Echogenicity
We did not observe changes in muscle echogenicity, though the study period is perhaps shorter than required to observed changes in the composition of these small muscles. It is worth noting that in the context of critical illness,Citation43 there are demonstrable changes in echogenicity within larger muscle groups within 10 days, although this is a highly catabolic state. Studies repeating respiratory muscle ultrasound assessment at more distant time points will be required to assess the impact of EBV on muscle quality.
Changes in Diaphragm Thickness and Excursion
There was no significant difference between measurements in either diaphragm thickness or excursion pre- and post-EBV insertion. There was a non-significant negative correlation between changes in RV, target lobe volume and diaphragm thickness. The direction of this relationship was as anticipated based on the relationship between the diaphragm and hyperinflation, with previous studies showing alterations in the diaphragm zone of apposition on US in hyperinflation.Citation44,Citation45 A larger cohort may have identified a significant relationship between these parameters.
Change in diaphragm excursion showed a non-significant correlation with change in RV and target lobe volumes, which is as would be anticipated given the improvements given previous studies using MRICitation46 and USCitation47 showing reduced excursion with hyperinflation with improvement following lung volume reduction surgery.Citation48
Limitations
There are limitations to our data. Firstly, this is a small study, with scans performed by a single operator. Whilst we have previously shown excellent intra-observer agreement including with these pre-EBV data in our previously,Citation14 the reproducibility of these findings by other sonographers is needed. Secondly, although endobronchial valve insertion affords an experimental opportunity to study parasternal muscles following a defined physiological intervention – relief of hyperinflation – it remains to be shown whether such changes occur following other therapeutic interventions. Finally, the addition of a control group of patients with COPD who have not undergone intervention would add further strength to these findings.
Implications
These data raise a possible mechanism by which EBV insertion may improve symptoms, via the alterations in intercostal muscles, likely due to restoration of optimal length–tension relationships. Measurements of intercostal thickness may provide clinicians with information regards to hyperinflation, particularly with regards to changes over time, with increasing thickness strongly related to reducing RV. This may provide clinicians with a non-invasive easily performed marker at the bedside.
The emerging ICU data with regards to intercostal muscle thickness/thickening and ventilation outcomes are of interest,Citation21 raising the possibility that adding intercostal measurements to diaphragm US may help identify those who are at risk of difficulties with ventilation weaning. Our data further underline the value of ultrasound imaging of the parasternal muscles.
Finally, a larger cohort study utilising controls who do not undergo mechanical relief of hyperinflation would provide further data with regards to the relationship between lung volume and intercostal muscle thickness. A longer duration of follow-up would allow further changes to be described, including changes in echogenicity which were likely not identified in our study due to the limited time between scans. These data would be further enriched by measurement of serum inflammatory markers, parasternal muscle EMG and ideally muscle biopsies to assess for remodelling, with previous research suggesting that in severe COPD parasternal muscles undergo fibre switching to fast to slow fibre and slow myosin heavy chain types.Citation49 This would also help further elucidate the relationship with diaphragm parameters, with our small sample size precluding any firm conclusions. Measurements of respiratory muscle strength, particularly with regards to inspiratory pressure generation would be of interest.
Conclusions
Dynamic changes in parasternal intercostal muscle thickness can be observed on respiratory muscle ultrasound. In patients with COPD undergoing EBV insertion, parasternal intercostal muscle thickness increased in proportion to the relief of hyperinflation, providing a mechanistic explanation for the diminished muscle thickness observed in COPD.
These findings also provide a further mechanism of symptomatic benefit following bronchoscopic lung volume reduction with EBV insertion.
Abbreviations
COPD, chronic obstructive pulmonary disease; CT, computed tomography; EBV, endobronchial valve; IPAQ, International Physical Activity Questionnaire; FEV1, forced expiratory volume in 1 second; RV, residual volume; SGRQ, St George Respiratory Questionnaire; TLC, total lung capacity.
Disclosure
Dr Selina M Parry reports grants from NHMRC Early Career Fellowship, outside the submitted work. The authors report no other conflicts of interest in this work.
Acknowledgments
We gratefully acknowledge the invaluable assistance of Michael Hofmann and Jason Callahan from the Department of Molecular Imaging, Peter McCallum Cancer Centre, Parkville Australia in the acquisition of lung volume data. The abstract of this paper was presented at the 2018 ERS International Conference as a poster presentation with interim findings. The poster’s abstract was published in “Poster Abstracts” in European Respiratory Journal 2018 52: PA5399; DOI: 10.1183/13993003.congress-2018.PA5399.
References
- VogelmeierCF, CrinerGJ, MartinezFJ, et al. Global strategy for the diagnosis, management and prevention of chronic obstructive lung disease 2017 report: GOLD executive summary. Respirology. 2017;22(3):575–601.28150362
- OfirD, LavenezianaP, WebbKA, LamYM, O’DonnellDE. Mechanisms of dyspnea during cycle exercise in symptomatic patients with GOLD stage I chronic obstructive pulmonary disease. Am J Respir Crit Care Med. 2008;177(6):622–629. doi:10.1164/rccm.200707-1064OC18006885
- GuenetteJA, WebbKA, O’DonnellDE. Does dynamic hyperinflation contribute to dyspnoea during exercise in patients with COPD? Eur Respir J. 2012;40(2):322–329. doi:10.1183/09031936.0015771122183485
- LavenezianaP, WebbKA, OraJ, WadellK, O’DonnellDE. Evolution of dyspnea during exercise in chronic obstructive pulmonary disease: impact of critical volume constraints. Am J Respir Crit Care Med. 2011;184(12):1367–1373. doi:10.1164/rccm.201106-1128OC21885624
- De TroyerA, LeeperJB, McKenzieDK, GandeviaSC. Neural drive to the diaphragm in patients with severe COPD. Am J Respir Crit Care Med. 1997;155(4):1335–1340. doi:10.1164/ajrccm.155.4.91050769105076
- PalangeP, ValliG, OnoratiP, et al. Effect of heliox on lung dynamic hyperinflation, dyspnea, and exercise endurance capacity in COPD patients. J Appl Physiol (1985). 2004;97(5):1637–1642. doi:10.1152/japplphysiol.01207.200315234959
- O’DonnellDE, WebbKA, NederJA. Lung hyperinflation in COPD: applying physiology to clinical practice. COPD Res Pract. 2015;1(1):4. doi:10.1186/s40749-015-0008-8
- BarrRG, BluemkeDA, AhmedFS, et al. Percent emphysema, airflow obstruction, and impaired left ventricular filling. N Engl J Med. 2010;362(3):217–227. doi:10.1056/NEJMoa080883620089972
- SeymourJM, SpruitMA, HopkinsonNS, et al. The prevalence of quadriceps weakness in COPD and the relationship with disease severity. Eur Respir J. 2010;36(1):81–88. doi:10.1183/09031936.0010490919897554
- GuerriR, GayeteA, BalcellsE, et al. Mass of intercostal muscles associates with risk of multiple exacerbations in COPD. Respir Med. 2010;104(3):378–388. doi:10.1016/j.rmed.2009.10.01519932014
- ParkMJ, ChoJM, JeonKN, et al. Mass and fat infiltration of intercostal muscles measured by CT histogram analysis and their correlations with COPD severity. Acad Radiol. 2014;21(6):711–717. doi:10.1016/j.acra.2014.02.00324809313
- GreeningNJ, Harvey-DunstanTC, ChaplinEJ, et al. Bedside assessment of quadriceps muscle by ultrasound after admission for acute exacerbations of chronic respiratory disease. Am J Respir Crit Care Med. 2015;192(7):810–816. doi:10.1164/rccm.201503-0535OC26068143
- De TroyerA, KirkwoodPA, WilsonTA. Respiratory action of the intercostal muscles. Physiol Rev. 2005;85(2):717–756. doi:10.1152/physrev.00007.200415788709
- WallbridgeP, ParrySM, DasS, et al. Parasternal intercostal muscle ultrasound in chronic obstructive pulmonary disease correlates with spirometric severity. Sci Rep. 2018;8(1):15274. doi:10.1038/s41598-018-33666-730323179
- VogiatzisI, HabazettlH, AlivertiA, et al. Effect of helium breathing on intercostal and quadriceps muscle blood flow during exercise in COPD patients. Am J Physiol Regul Integr Comp Physiol. 2011;300(6):R1549–59. doi:10.1152/ajpregu.00671.201021411767
- LouvarisZ, VogiatzisI. Contrasting the physiological effects of heliox and oxygen during exercise in a patient with advanced COPD. Breathe (Sheff). 2019;15(3):250–257. doi:10.1183/20734735.0197-201931508165
- LouvarisZ, VogiatzisI, AlivertiA, et al. Blood flow does not redistribute from respiratory to leg muscles during exercise breathing heliox or oxygen in COPD. J Appl Physiol (1985). 2014;117(3):267–276. doi:10.1152/japplphysiol.00490.201424903919
- SuhES, MandalS, HardingR, et al. Neural respiratory drive predicts clinical deterioration and safe discharge in exacerbations of COPD. Thorax. 2015;70(12):1123–1130. doi:10.1136/thoraxjnl-2015-20718826194996
- PatoutM, MeiraL, D’CruzR, et al. Neural respiratory drive predicts long-term outcome following admission for exacerbation of COPD: a post hoc analysis. Thorax. 2019;74(9):910–913. doi:10.1136/thoraxjnl-2018-21207431028235
- NakanishiN, OtoJ, UenoY, NakatakiE, ItagakiT, NishimuraM. Change in diaphragm and intercostal muscle thickness in mechanically ventilated patients: a prospective observational ultrasonography study. J Intensive Care. 2019;7:56. doi:10.1186/s40560-019-0410-431827804
- DresM, DubeBP, GoligherE, et al. Usefulness of parasternal intercostal muscle ultrasound during weaning from mechanical ventilation. Anesthesiology. 2020;132(5):1114–1125. doi:10.1097/ALN.000000000000319132084029
- MaltaisF, DecramerM, CasaburiR, et al. An official American Thoracic Society/European Respiratory Society statement: update on limb muscle dysfunction in chronic obstructive pulmonary disease. Am J Respir Crit Care Med. 2014;189(9):e15–62. doi:10.1164/rccm.201402-0373ST24787074
- De TroyerA, WilsonTA. Effect of acute inflation on the mechanics of the inspiratory muscles. J Appl Physiol (1985). 2009;107(1):315–323. doi:10.1152/japplphysiol.91472.200819265064
- KloosterK, SlebosDJ, ZoumotZ, DaveyC, ShahPL, HopkinsonNS. Endobronchial valves for emphysema: an individual patient-level reanalysis of randomised controlled trials. BMJ Open Respir Res. 2017;4(1):e000214. doi:10.1136/bmjresp-2017-000214
- CrinerGJ, SueR, WrightS, et al. A Multicenter RCT of Zephyr(R) endobronchial valve treatment in heterogeneous emphysema (LIBERATE). Am J Respir Crit Care Med. 2018;198(9):1151–1164. doi:10.1164/rccm.201803-0590OC29787288
- DaveyC, ZoumotZ, JordanS, et al. Bronchoscopic lung volume reduction with endobronchial valves for patients with heterogeneous emphysema and intact interlobar fissures (the BeLieVeR-HIFi trial): study design and rationale. Thorax. 2015;70(3):288–290. doi:10.1136/thoraxjnl-2014-20512724664535
- ParkTS, HongY, LeeJS, et al. Bronchoscopic lung volume reduction by endobronchial valve in advanced emphysema: the first Asian report. Int J Chron Obstruct Pulmon Dis. 2015;10:1501–1511.26251590
- SlebosDJ, ShahPL, HerthFJ, ValipourA. Endobronchial valves for endoscopic lung volume reduction: best practice recommendations from expert panel on endoscopic lung volume reduction. Respir Int Rev Thor Dis. 2017;93(2):138–150. doi:10.1159/000453588
- JonesPW, QuirkFH, BaveystockCM, LittlejohnsP. A self-complete measure of health status for chronic airflow limitation. The St George’s Respiratory Questionnaire. Am Rev Respir Dis. 1992;145(6):1321–1327. doi:10.1164/ajrccm/145.6.13211595997
- CraigCL, MarshallAL, SjostromM, et al. International physical activity questionnaire: 12-country reliability and validity. Med Sci Sports Exerc. 2003;35(8):1381–1395. doi:10.1249/01.MSS.0000078924.61453.FB12900694
- Hurtig-WennlofA, HagstromerM, OlssonLA. The International Physical Activity Questionnaire modified for the elderly: aspects of validity and feasibility. Public Health Nutr. 2010;13(11):1847–1854. doi:10.1017/S136898001000015720196910
- CharlsonM, SzatrowskiTP, PetersonJ, GoldJ. Validation of a combined comorbidity index. J Clin Epidemiol. 1994;47(11):1245–1251. doi:10.1016/0895-4356(94)90129-57722560
- HallWH, RamachandranR, NarayanS, JaniAB, VijayakumarS. An electronic application for rapidly calculating Charlson comorbidity score. BMC Cancer. 2004;4:94. doi:10.1186/1471-2407-4-9415610554
- WallbridgePD, JoostenSA, HannanLM, et al. A prospective cohort study of thoracic ultrasound in acute respiratory failure: the C3PO protocol. JRSM Open. 2017;8(5):2054270417695055. doi:10.1177/205427041769505528515954
- SarwalA, WalkerFO, CartwrightMS. Neuromuscular ultrasound for evaluation of the diaphragm. Muscle Nerve. 2013;47(3):319–329. doi:10.1002/mus.2367123382111
- SarwalA, ParrySM, BerryMJ, et al. Interobserver reliability of quantitative muscle sonographic analysis in the critically Ill population. J Ultrasound Med. 2015;34(7):1191–1200. doi:10.7863/ultra.34.7.119126112621
- von ElmE, AltmanDG, EggerM, et al. The Strengthening the Reporting of Observational Studies in Epidemiology (STROBE) statement: guidelines for reporting observational studies. PLoS Med. 2007;4(10):e296. doi:10.1371/journal.pmed.004029617941714
- PizarroC, SchuelerR, HammerstinglC, TuletaI, NickenigG, SkowaschD. Impact of endoscopic lung volume reduction on right ventricular myocardial function. PLoS One. 2015;10(4):e0121377. doi:10.1371/journal.pone.012137725856379
- PizarroC, AhmadzadehfarH, EsslerM, et al. Effect of endobronchial valve therapy on pulmonary perfusion and ventilation distribution. PLoS One. 2015;10(3):e0118976. doi:10.1371/journal.pone.011897625822624
- ZoumotZ, LoMauroA, AlivertiA, et al. Lung volume reduction in emphysema improves chest wall asynchrony. Chest. 2015;148(1):185–195. doi:10.1378/chest.14-238025654309
- MartinezFJ, CouserJI, CelliBR. Factors influencing ventilatory muscle recruitment in patients with chronic airflow obstruction. Am Rev Respir Dis. 1990;142(2):276–282. doi:10.1164/ajrccm/142.2.2762382890
- KauczorHU, HeusselCP, FischerB, KlammR, MildenbergerP, ThelenM. Assessment of lung volumes using helical CT at inspiration and expiration: comparison with pulmonary function tests. AJR Am J Roentgenol. 1998;171(4):1091–1095. doi:10.2214/ajr.171.4.97630039763003
- ParrySM, El-AnsaryD, CartwrightMS, et al. Ultrasonography in the intensive care setting can be used to detect changes in the quality and quantity of muscle and is related to muscle strength and function. J Crit Care. 2015;30(5):1151.e9–1151.e14. doi:10.1016/j.jcrc.2015.05.024
- GormanRB, McKenzieDK, PrideNB, TolmanJF, GandeviaSC. Diaphragm length during tidal breathing in patients with chronic obstructive pulmonary disease. American Journal of Respiratory & Critical Care Medicine. 2002;166(11):1461–1469. doi:10.1164/rccm.200111-087OC12406839
- GormanRB, McKenzieDK, ButlerJE, TolmanJF, GandeviaSC. Diaphragm length and neural drive after lung volume reduction surgery. American Journal of Respiratory & Critical Care Medicine. 2005;172(10):1259–1266. doi:10.1164/rccm.200412-1695OC16109977
- IwasawaT, KageiS, GotohT, et al. Magnetic resonance analysis of abnormal diaphragmatic motion in patients with emphysema. Eur Respir J. 2002;19(2):225–231. doi:10.1183/09031936.02.0004460211866002
- BariaMR, ShahgholiL, SorensonEJ, et al. B-mode ultrasound assessment of diaphragm structure and function in patients with COPD. Chest. 2014;146(3):680–685. doi:10.1378/chest.13-230624700122
- SugaK, TsukudaT, AwayaH, et al. Impaired respiratory mechanics in pulmonary emphysema: evaluation with dynamic breathing MRI. J Magn Resonance Imaging. 1999;10(4):510–520. doi:10.1002/(SICI)1522-2586(199910)10:4<510::AID-JMRI3>3.0.CO;2-G
- LevineS, NguyenT, FrisciaM, et al. Parasternal intercostal muscle remodeling in severe chronic obstructive pulmonary disease. J Appl Physiol (1985). 2006;101(5):1297–1302. doi:10.1152/japplphysiol.01607.200516777998