Abstract
Background
Previous studies have demonstrated the potential beneficial effect of N-acetylcysteine (NAC) in chronic obstructive pulmonary disease (COPD). However, the required dose and responder phenotype remain unclear. The current study investigated the effect of high-dose NAC on airway geometry, inflammation, and oxidative stress in COPD patients. Novel functional respiratory imaging methods combining multislice computed tomography images and computer-based flow simulations were used with high sensitivity for detecting changes induced by the therapy.
Methods
Twelve patients with Global Initiative for Chronic Obstructive Lung Disease stage II COPD were randomized to receive NAC 1800 mg or placebo daily for 3 months and were then crossed over to the alternative treatment for a further 3 months.
Results
Significant correlations were found between image-based resistance values and glutathione levels after treatment with NAC (P = 0.011) and glutathione peroxidase at baseline (P = 0.036). Image-based resistance values appeared to be a good predictor for glutathione peroxidase levels after NAC (P = 0.02), changes in glutathione peroxidase levels (P = 0.035), and reduction in lobar functional residual capacity levels (P = 0.00084). In the limited set of responders to NAC therapy, the changes in airway resistance were in the same order as changes induced by budesonide/formoterol.
Conclusion
A combination of glutathione, glutathione peroxidase, and imaging parameters could potentially be used to phenotype COPD patients who would benefit from addition of NAC to their current therapy. The findings of this small pilot study need to be confirmed in a larger pivotal trial.
Introduction
Chronic obstructive pulmonary disease (COPD) is a heterogeneous disorder characterized by dysfunction of the small and large airways, as well as destruction of the lung parenchyma and its vasculature in highly variable combinations. The hallmark of COPD is expiratory flow limitation, which is slowly progressive and irreversible.Citation1–Citation3 There is also increasing evidence that the inflammatory processes of COPD are closely associated with oxidative stress. Proinflammatory cytokines and growth factors stimulate production of reactive oxygen species. The resulting imbalance between oxidants and antioxidants triggers signaling cascades for a variety of transduction pathways and gene expression, leading in turn to altered expression of proinflammatory factors. Neutrophils are key cells in the inflammatory response in COPD. Excessive transmigration into the lung tissues and abnormal activation are largely responsible for overproduction of reactive oxygen species and release of proteolytic enzymes in the lungs.
N-acetylcysteine (NAC) is a glutathione precursor with beneficial properties. It is a source of sulfhydryl groups in cells and increases the level of reduced glutathione. Reduced glutathione serves as a reducing agent by forming intermolecular disulfide nonradical end product-oxidized glutathione. NAC is also a scavenger of free radicals as it interacts with reactive oxygen species, such as the hydroxyl radical (OH-) and hydrogen peroxide (H2O2).Citation4–Citation7
In COPD patients, NAC has been used as a mucolytic and for its antioxidant properties. In a large-scale study, it was not possible to demonstrate that NAC decreases the decline in forced expiratory volume in one second (FEV1) in COPD patients after a period of 3 years.Citation8 In the same study, however, there were some preliminary indications that NAC reduce hyperinflation, since a decrease in functional residual capacity was observed over time. However, the effects of NAC are certainly dose-dependent.Citation9 It might well be that the lack of efficacy is partially explained by use of dosages that are too low.Citation9
Considering all the above, it would be worthwhile to study the effect of higher dosages of NAC on the small airways, inflammation, and oxidative stress in COPD patients. In this randomized crossover study, patients with Global initiative for chronic Obstructive Lung Disease (GOLD) stage II COPD received placebo for 3 months and NAC at a dosage of 600 mg 3 times daily for 3 months in addition to their usual medication. For this trial, we used novel advanced imaging tools that have been demonstrated in the past to yield more sensitive outcome parameters compared with classical lung function tests.Citation10 This functional respiratory imaging method was validated in previous studies using gamma scintigraphy, Citation11,Citation12 single photon emission computed tomography (CT),Citation13 and hyperpolarized 3He magnetic resonance imaging.Citation14 Subsequently, this method was used to assess the effect of a long-acting β2-agonist,Citation15 a combination of a long-acting β2-agonist and inhaled corticosteroid,Citation10 and a short-acting anticholinergic and short-acting β2-agonist.Citation16 In the current study, we hypothesized that this method could be used to gain additional insight into the mode of action of NAC in COPD patients, particularly the relationship between upregulation of glutathione/glutathione peroxidase and changes in airways volume and resistance. Further, this pilot study aimed to provide a basis for identifying patient phenotypes that would benefit most from NAC therapy.
Materials and methods
The study was conducted according to all ethical principles. Approval was obtained from the ethics committee and all patients gave their informed consent (NCT0969904). The study started in August 2009 and ended in June 2012.
Patient population
Twelve COPD patients (nine men and three women) were included. To be eligible for the study, patients had to have documented COPD and meet the following inclusion criteria: a smoking history of at least 10 pack-years; compatible symptoms, including dyspnea, cough, and sputum production; a decreased Tiffeneau index (FEV1/forced vital capacity [FVC]) < 0.7; age ≥40 years; cessation of smoking for at least one month; moderate to severe COPD with an FEV1 30%–80% of predicted [GOLD stages II and III]; and treatment according to GOLD guidelines. The following exclusion criteria were applied: exacerbation during the last 8 weeks; allergy to acetylcysteine or to another element of the product; phenylketonuria; untreated active peptic ulcer; renal and/or cardiac insufficiency; previous treatment with NAC for more than 6 months or during the last 3 months; ongoing treatment with oral, intravenous, or intramuscular corticosteroids; pregnancy or breast-feeding; and treatment with an orally administered cephalosporin.
Study design
At all visits, patients received full lung function testing yielding the following parameters: FEV1, FEV1/FVC, peak expiratory flow from spirometry, and airway resistance (Raw) and specific airway resistance from body plethysmography. A low-dose multislice CT scan was taken at total lung capacity and functional residual capacity. From these images of the airways, lung and lobar volumes could be obtained as well as airway resistance values by means of computational fluid dynamics. In addition, a blood sample was taken to measure levels of glutathione, glutathione peroxidase, superoxide dismutase, and interleukin-8. Patients completed the Saint George’s Respiratory Questionnaire (SGRQ, ). At the first visit, patients were randomized according to a computer-generated randomization list to treatment with NAC (Fluimucil®, Zambon SpA, Bresso, Italy) at a dose of 600 mg three times daily or placebo in addition to their usual treatment. After 3 months of treatment, the patients returned to the hospital for further tests and were crossed over to NAC or placebo. After a further 3 months, all measurements were repeated.
Table 1 Flowchart describing visits and tests
To limit exposure to ionizing radiation, a dose reduction protocol was used for the CT scans. A VCT light speed scanner with 64 detector rows (General Electric, Fairfield, CT, USA) was used. The multislice CT settings were as follows: tube voltage, 120 kV; tube current,10–100 mA; noise factor, 28; collimation, 0.625 mm; rotation time, 0.6 seconds; and pitch factor, 1.375. The resulting radiation dose was in the order of 1–2 mSv per scan. Images were reconstructed to a slice thickness of 0.6 mm. Digital Imaging and Communications in Medicine (DICOM) images were assessed using a commercially available software package approved by the US Food and Drug Administration (Mimics, Materialise, Leuven, Belgium). The tracheobronchial tree was subsequently segmented using a semiautomatic approach. A total of three airway models were obtained per patient, ie, a baseline model, a model after 3 months of treatment with NAC or placebo, and a repeat model 3 months after crossover from NAC or placebo. After segmentation, all models for the same patient were superimposed using a least squares method to ensure that a comparison was possible between the different geometries. Segmentation yields the airway volumes at the central (iVawcent) and distal (iVawdist) levels. In addition to airway volumes, it was also possible to extract the lung and lobar volumes from the CT images at functional residual capacity and total lung capacity. Using computational fluid dynamics, it is possible to describe the flow patterns inside the airways based on computer simulation. Computational fluid dynamics numerically solves the Navier–Stokes equations inside the prescribed flow domain, in this case the airway tree structures at different measurement points. The main outcome parameter of computational fluid dynamic calculations is airway resistance in the central (iRawcent) and distal airways (iRawdist).
Statistical analysis
Statistical analysis was performed using Statistica 9.1 software (StatSoft Inc, Tulsa, OK, USA). Differences were assessed using the Wilcoxon matched-pairs test for equal sample sizes that are related or Mann–Whitney U test for independent samples. Correlations were assessed using the Spearman’s rank test. A P-value < 0.05 was considered to be statistically significant.
Results
Patients
All patients were categorized by GOLD guidelines as stage II with an average post-bronchodilation FEV1 of 65.38% ± 7.12% predicted. The average age of the patients was 65.00 ± 9.63 years, average height was 171.17 ± 8.27 cm, and the average smoking history was 56.16 ± 33.12 pack-years ().
Table 2 Patient characteristics at baseline
Image-based resistance and volume
shows image-based resistance (iRaw) and volume (iVaw) measurements for the individual patients. The left hand side depicts iRaw, while the right hand side shows iVaw. The left upper quadrant indicates an increase in iRaw, hence nonresponders. The left lower quadrant indicates a decline in iRaw, hence responders. For iVaw it is reversed, whereby the right upper quadrant indicates an increase in iVaw, therefore identifying responders, and the right lower quadrant indicates an decline in iVaw, hence nonresponders. From , it can be seen that there was a relatively even distribution between patients who responded in terms of iRaw and iVaw measurements. There were six patients with a reported reduction in iRawcent and four with a reported reduction in iRawdist. In total, five patients demonstrated an increase in iVawdist and three patients showed an increase in iVawcent. In general, the changes could be considered small. However, the differences are considerable for individual patients on a local scale. shows the changes in airway resistance after placebo and NAC treatment for a patient who could be considered an iRaw or NAC responder. It could be observed that the changes are not homogeneously distributed throughout the airway system with a general decline in airway resistance up to 50% after NAC treatment and an increase in airway resistance up to 20% after placebo. shows the results for a nonresponder in terms of iRaw after NAC treatment. It could be observed that local resistance tended to increase up to 20% both after placebo and after NAC treatment.
Figure 1 Changes in iRaw and iVaw for all patients.
Abbreviations: iRaw, image-based resistance; iVaw, image-based volume.
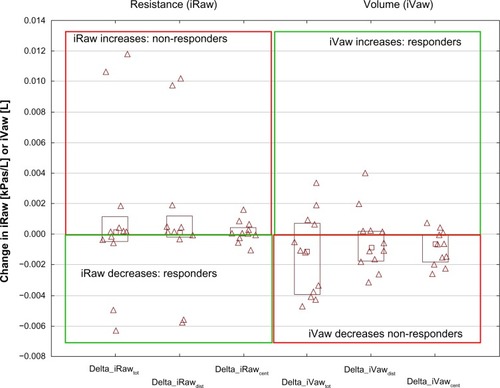
Glutathione peroxidase and glutathione
The study showed a significant correlation (R = −0.7, P = 0.011) between the level of glutathione after NAC treatment and the change in resistance of the central airways (iRawcent) measured using imaging and computational fluid dynamics (). No correlation was found between glutathione after placebo and iRawcent after placebo. The level of glutathione peroxidase at baseline (R = 0.61, P = 0.036) and after placebo treatment (R = 0.67, P = 0.017) correlated with the change in distal airway resistance (iRawdist) after NAC treatment (). No significant correlation was found between the baseline and placebo values for glutathione peroxidase and the change in iRawdist after placebo. Using the Mann–Whitney U test, it could be observed that the level of glutathione after treatment was significantly higher (P = 0.020) in patients who demonstrated a reduction in iRawcent compared with patients in whom iRawcent stayed constant or increased (). A similar result was found based on the segmented airway volume of the central airways (iVawcent). Glutathione after NAC treatment was significantly higher (P = 0.042) in patients with an increase in iVawcent. In patients who demonstrated a reduction in total (central + distal) image-based airway resistance (iRawtot), the change in glutathione peroxidase was significantly (P = 0.035) higher compared with patients in whom iRawtot did increase. On average, glutathione peroxidase levels increased in the group with reduced iRawtot and decreased in the group with elevated iRawtot after treatment. FEV1 also increased slightly in the group with reduced iRawtot and decreased in the group with higher iRawtot after NAC treatment, although the difference between the two groups was not significant ().
Figure 4 Significant correlation between change in computational fluid dynamics-based resistance of the central airways, iRawcent, and glutathione levels after NAC treatment.
Abbreviations: iRaw, image-based resistance; GSH, glutathione; NAC, N-acetylcysteine.
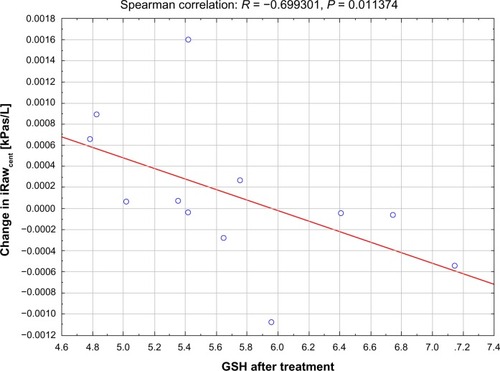
Figure 5 Significant correlation between change in computational fluid dynamics-based resistance of the distal airways iRawdist and level of GPx at baseline (top) and after placebo (bottom).
Abbreviations: iRaw, image-based resistance; GPx, glutathione peroxidase.
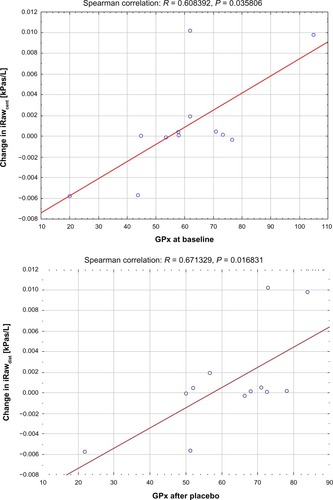
Figure 6 Glutathione after NAC treatment was significantly different for patients who experienced a decrease in iRawcent (top) and an increase in iVawcent (bottom).
Abbreviations: iRaw, image-based resistance; GSH, glutathione; iVaw, image-based volume.
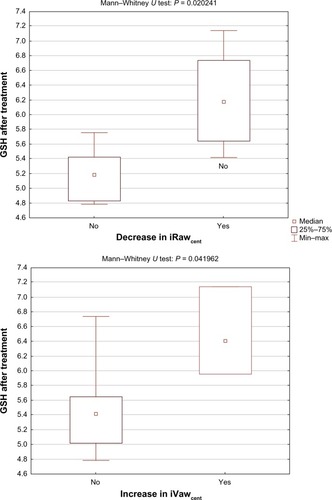
Figure 7 Significant difference in change in GPx between responders and nonresponders in terms of iRawtot (top) and no significant difference in change in GPx between responders and nonresponders in terms of FEV1 (bottom).
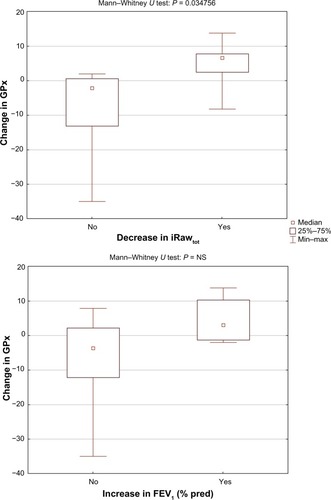
Hyperinflation
For patients in whom iRawdist decreased, hyperinflation in terms of lobar functional residual capacity level also decreased (). For patients in whom iRawdist did not decrease, the median lobar functional residual capacity level increased. The difference between the two groups was significant (P = 0.00084). Two patients experienced a reduction in iRawdist larger than 0.002 kPa/L. The Wilcoxon matched-pairs test showed a significant improvement in lobar inspiratory capacity (lobar total lung capacity – lobar functional residual capacity) in these patients compared with baseline (P = 0.028) and placebo (P = 0.011). A significant decline in lobar inspiratory capacity was observed between baseline and placebo (P = 0.011, ). The SGRQ symptom score also improved in these two patients.
Table 3 Lobar inspiratory capacity decreased significantly after placebo and increased after treatment with NAC in patients with a reduction in iRawdist < 0.002 kPa/L
Discussion
This study aimed to assess the effect of high-dose NAC on image-based airway and lung geometry, inflammation, and oxidative stress in patients with moderate COPD. Previous studiesCitation10,Citation16 have demonstrated that functional respiratory imaging can detect changes that are not reflected in conventional pulmonary function tests. Significant correlations were found between imaging parameters and markers related to oxidative stress. The initial results of this placebo-controlled, crossover trial could assist in gaining further insight into the effect of NAC and selection of potential responders.
The effect of NAC in COPD patients has been the subject of many clinical trials in the past. The capability of NAC to increase levels of reduced glutathione and hence to enhance protection against oxidative stress has been clearly demonstrated in preclinical studies.Citation17–Citation19 Nevertheless, it has been difficult to demonstrate consistently the added clinical value of NAC in COPD patients. Reasons often cited for this are the relatively small size of the clinical trials and variation in the dose of NAC used in the trials.Citation20 Positive effects of NAC demonstrated in previous trials include a reduction in air trapping and subsequent improvement in inspiratory capacity.Citation21 Also, a reduction in the hazard ratio for exacerbations was reported in BRONCUS (Bronchitis Randomized on N-acetylcysteine (NAC) Cost-Utility Study),Citation8 potentially due to enhanced protection against viral infections.Citation20 The use of lung function, and especially FEV1, to establish the effect of NAC, which is not a bronchodilator, has been challenging because FEV1 is a poor predictor of clinical symptoms, exercise tolerance, and response to bronchodilators in COPD.Citation21–Citation23
Our placebo-controlled, crossover study, for the first time, uses novel imaging tools with a higher sensitivity to detect changes in the respiratory system induced by high-dose NAC. Previous studies have validated and demonstrated the capabilities of these new methods to show changes induced by short-acting and long-acting bronchodilators and anti-inflammatory compounds. Interestingly, even despite the small sample size, the current study was able to show correlations between image-based parameters (ie, computational fluid dynamics-based resistance) and the enzyme glutathione peroxidase, which protects against oxidative stress, and the antioxidant glutathione. Both the enzyme and the antioxidant are known to be affected by NAC. To put these results into perspective, it is useful to compare the changes in central and distal airway resistance as measured by the CT/computational fluid dynamics combination with changes previously reported in our other imaging trials. It could be observed that for patients responding well to NAC, the magnitude of the reduction in airway resistance was in the same order as the effect induced by 320/90 μg budesonide/formoterol combinationCitation10 and just below the maximum effect of 400 μg of salbutamol and 80 μg of ipratropium bromide.Citation16 As in previous studies, the placebo group also demonstrated in general an increase in airway resistance which indicates that, despite the lack of clear evidence, COPD patients need to use their inhalation medication consistently to limit the decline in lung function.
The role of glutathione peroxidase appears to be interesting. The current study clearly shows a correlation between the baseline and placebo values for glutathione peroxidase and the change in resistance after treatment. It is known that the biochemical function of glutathione peroxidase is to reduce free H2O2 to water, thereby protecting the organism from oxidative stress. We could speculate that patients with low baseline glutathione peroxidase have additional reserves that could be activated by high doses of NAC, eventually resulting in a reduction in airway resistance. This hypothesis needs to be investigated further in a larger clinical trial in which the role of cytokines, not studied in the current trial, could also be explored further. If the hypothesis is confirmed, the level of glutathione peroxidase could be the basis for selecting patients who would benefit from treatment with NAC in addition to their current therapy.
Further, the current pilot study demonstrated that FEV1 was not a good predictor for changes in glutathione peroxidase induced by NAC therapy. The iRawdist on the other hand was able to group patients according to the difference in glutathione peroxidase with statistical significance. The latter can be explained by the enhanced signal-to-noise ratio of imaging. By focusing on a specific clinically relevant region, ie, the central and distal airways, it is possible to eliminate “noise” from the upper airway and the patient’s effort. However, the spirometer’s inherent black box approach does not allow this. In line with previous studies, it was demonstrated that NAC could have a beneficial effect on hyperinflation in a subset of patients. The functional residual capacity level of the lobes was significantly reduced in patients showing a decrease in distal airway resistance. Inspiratory capacity at a lobar level was improved in the best iRawdist responders. Even though this was only in two patients, the improvement in SGRQ symptom score potentially indicates a beneficial effect of reduction in hyperinflation on the patient’s quality of life. Recent large-scale studies also point in the same direction, whereby hyperinflation appears to be an important determinant of the patient’s clinical condition.Citation24,Citation25
The current pilot study demonstrates how new imaging tools can assist research into the pathophysiology of COPD and the subsequent effect of inhalation therapies. However, the sample size of the current study was very small and the results need to be confirmed in larger clinical trials. Nonetheless, there is increasing evidence that these novel imaging methods could complement existing pulmonary function tests and patient-reported outcome parameters to provide better phenotyping of COPD patients in order to enhance and expedite future development of respiratory drugs.
Author contributions
JDB, WV, SV, CVH, RC, PMP and WDB were responsible for the conception and design of the study, along with its analysis and interpretation. JDB, WV, SV, CVH, RC, PMP, and WDB drafted and revised the manuscript for important intellectual content.
Disclosure
JDB is a founder/shareholder of FluidDA NV, Kontich, Belgium. WV, SV, and CVH are employed by FluidDA NV, and WDB is the director of FluidDA NV. PMP and RC have no conflicts of interest to report. The study was supported by Zambon SpA, Bresso, Italy.
References
- HoggJCChronic obstructive pulmonary disease: an overview of pathology and pathogenesisNovartis Found Symp200123441911199102
- KuwanoKBoskenCHParéPDBaiTRWiggsBRHoggJCSmall airways dimensions in asthma and in chronic obstructive pulmonary diseaseAm Rev Respir Dis19931485122012258239157
- BoskenCHWiggsBRParéPDHoggJCSmall airway dimensions in smokers with obstruction to airflowAm Rev Respir Dis199014235635702389908
- SadowskaAMVan OverveldFJGóreckaDThe interrelationship between markers of inflammation and oxidative stress in chronic obstructive pulmonary disease: modulation by inhaled steroids and antioxidantRespir Med200599224124915715193
- SadowskaAMVerbraeckenJDarquennesKDe BackerWARole of N-acetylcysteine in the management of COPDInt J Chron Obstruct Pulmon Dis20061442543418044098
- SadowskaAMManuel-y-KeenoyBVertongenTEffect of N-acetylcysteine on neutrophil activation markers in healthy volunteers: in vivo and in vitro studyPharmacol Res200653321622516384711
- SadowskaAMLuytenCVintsA-MVerbraeckenJVan RanstDDe BackerWASystemic antioxidant defences during acute exacerbation of chronic obstructive pulmonary diseaseRespirology200611674174717052302
- DecramerMRutten-van MölkenMDekhuijzenPNREffects of N-acetylcysteine on outcomes in chronic obstructive pulmonary disease (Bronchitis Randomized on NAC Cost-Utility Study, BRONCUS): a randomised placebo-controlled trialLancet200536594701552156015866309
- SadowskaAMManuel-Y-KeenoyBDe BackerWAAntioxidant and anti-inflammatory efficacy of NAC in the treatment of COPD: discordant in vitro and in vivo dose-effects: a reviewPulm Pharmacol Ther200720192216458553
- De BackerLAVosWDe BackerJVan HolsbekeCVinchurkarSDe BackerWThe acute effect of budesonide/formoterol in COPD: a multi-slice computed tomography and lung function studyEur Respir J201240229830522183484
- De BackerJWVosWGGorléCDFlow analyses in the lower airways: patient-specific model and boundary conditionsMed Eng Phys200830787287918096425
- VinchurkarSBackerLDVosWHolsbekeCVBackerJDBackerWDA case series on lung deposition analysis of inhaled medication using functional imaging based computational fluid dynamics in asthmatic patients: effect of upper airway morphology and comparison with in vivo dataInhal Toxicol2012242818822260527
- De BackerJWVosWGVinchurkarSCValidation of computational fluid dynamics in CT-based airway models with SPECT/CTRadiology2010257385486221084417
- De RochefortLVialLFodilRIn vitro validation of computational fluid dynamic simulation in human proximal airways with hyperpolarized 3He magnetic resonance phase-contrast velocimetryJ Appl Physiol200710252012202317289906
- De BackerJWVosWGDevolderAComputational fluid dynamics can detect changes in airway resistance in asthmatics after acute bronchodilationJ Biomech200841110611317698073
- De BackerLVosWSalgadoRFunctional imaging using computer methods to compare the effect of salbutamol and ipratropium bromide in patient-specific airway models of COPDInt J Chron Obstruct Pulmon Dis 201120116637646
- LappasMPermezelMRiceGEN-Acetyl-cysteine inhibits phospholipid metabolism, proinflammatory cytokine release, protease activity, and nuclear factor-kappaB deoxyribonucleic acid-binding activity in human fetal membranes in vitroJ Clin Endocrinol Metabol200388417231729
- UngheriDPisaniCSansonGProtective effect of n-acetylcysteine in a model of influenza infection in miceInt J Immunopathol Pharmacol200013312312812657201
- CaiSChenPZhangCChenJ-BWuJOral N-acetylcysteine attenuates pulmonary emphysema and alveolar septal cell apoptosis in smoking-induced COPD in ratsRespirology200914335435919341424
- DekhuijzenPNVan BeurdenWJThe role for N-acetylcysteine in the management of COPDInt J Chron Obstruct Pulmon Dis2006129910618046886
- StavDRazMEffect of N-acetylcysteine on air trapping in COPD: a randomized placebo-controlled studyChest2009136238138619447919
- CelliBCOPD, inflammation and its modulation by phosphodiesterase 4 inhibitors: time to look beyond the FEV1Chest200612915616424403
- JonesPWHealth status measurement in chronic obstructive pulmonary diseaseThorax2001561188088711641515
- WedzichaJDecramerMSeemungalTThe role of bronchodilator treatment in the prevention of exacerbations of COPDEur Respir J20124061545155422835613
- ComeCEDivoMJSan José EstéparRLung deflation and oxygen pulse in COPD: results from the NETT randomized trialRespir Med2012106110911921843930