Abstract
Tuberculosis (TB) stands as the second most prevalent cause of global human mortality from infectious diseases. In 2022, the World Health Organization documented an estimated number of global TB cases reaching 7.5 million, which causes death for 1.13 million patients. The continuous growth of drug-resistant TB cases due to various mutations in the Mycobacterium tuberculosis (MTB) strain, raises the urgency of the exploration of novel anti-TB treatments. Ursolic acid (UA) is a natural pentacyclic triterpene found in various plants that has shown potential as a novel anti-TB agent. This review aims to provide an overview of the therapeutic prospects of UA against MTB, with a particular emphasis on in silico, in vitro, and in vivo studies. Various mechanisms of action of UA against MTB are briefly recapped from in silico studies, such as enoyl acyl carrier protein reductase inhibitors, FadA5 (Acetyl-CoA acetyltransferase) inhibitors, tuberculosinyl adenosine transferase inhibitors, and small heat shock protein 16.3 inhibitor. The potential of UA to overcome drug resistance and its synergistic effects with existing antituberculosis drugs are briefly explained from in vitro studies using a variety of methods, such as Microplate Alamar Blue Assay, Mycobacteria Growth Indicator Tube 960 and Resazurin Assays, morphological change evaluation using transmission electron microscopy, and in vivo studies using BALB/C infected with multi drug resistant clinical isolates. Besides its promising mechanism as an antituberculosis drug, its complex chemical composition, limited availability and supply, and lack of intellectual property are also reviewed as those are the most frequently occurring challenges that need to be addressed for the successful development of UA as novel anti-TB agent.
Keywords:
Introduction
Tuberculosis (TB), an infectious disease caused by Mycobacterium tuberculosis (MTB), remains a significant threat to global health. According to the World Health Organization, in 2022 there will be 10.6 million new cases of TB, resulting in 1.6 million fatal accidents. The combination of drug resistance and human immunodeficiency virus (HIV) coinfection contributes to the elevated mortality rate, with 6.7% of people living with HIV. Geographically, most TB cases were in Southeast Asia (45%), including Indonesia.Citation1
Multidrug-resistant tuberculosis (MDR-TB), which is a form of TB caused by bacteria that do not respond to at least isoniazid and rifampicin, the two most potent TB drugs, is a growing problem in TB treatment. Moreover, extensively drug-resistant tuberculosis (XDR-TB) is a more severe form of MDR-TB caused by bacteria that do not respond to the most effective second-line anti-TB medications, leaving patients frequently without further treatment options.Citation1 The need for developing novel chemical entities of TB medicines is highlighted by the rising prevalence of treatment resistance.
Ursolic acid (3-hydroxy-urs-12-ene-28-oic acid) is a pentacyclic triterpenoid with a variety of pharmacological effects. As a secondary metabolite, this substance is typically found in fruit peels and stem bark.Citation2 Since it is found in the leaves of rosemary, thyme, oregano, lavender, and hawthorn, ursolic acid (UA) has been used as an herb extract in traditional medicine for centuries. There is a growing interest in UA because of its beneficial effects including anti-inflammatory,Citation3 anti-cancer,Citation4 and antioxidant.Citation5–7 This pentacyclic triterpenoid has also been proven to have antibacterial effects,Citation8,Citation9 and it has been demonstrated that their action can make bacteria more susceptible to other chemicals, including antibiotics.Citation10
The antimycobacterial activity of UA isolated from Duroia macrophylla was assessed, and the results showed that UA significantly inhibited the growth of MTB with a minimum inhibitory concentration (MIC) of 25 µg/mL against sensitive and resistant strains of MTB.Citation11 The concentration of mycolic acid in the MTB H37Ra strain decreases when treated with UA, according to Jyoti et al. The transmission and scanning electron microscopy (SEM and TEM) images of a sensitive MTB strain treated with UA revealed morphological abnormalities and cell lysis.Citation12 In addition, investigations of the combination of UA and antituberculosis drugs (isoniazid, ethambutol, streptomycin, and rifampin) revealed a synergistic effect against MTB.Citation13 Jiménez-Arellanes et al also observed that the combination of UA and oleanolic acid (OA) has immunomodulatory effects.Citation14 The combination is also effective against intracellular MTB, resulting in substantially decreased lung bacillary loads.Citation14
This review aims to provide an overview of the therapeutic potential of UA against MTB, with a particular emphasis on in silico, in vitro, and in vivo studies. While acknowledging the challenges associated with UA isolation and purification, and the lack of property protection, this review discreetly identifies emerging prospects that may guide the future research and development of UA as an anti-TB drug.
Chemical Characteristic and Isolation of UA
UA has a molecular mass of 456.71 g/mol and the chemical formula C30H48O3 (). UA is soluble in alcoholic sodium hydroxide and heated glacial acetic acid but poorly soluble in water.Citation15 It is primarily biosynthesized from the dammarenyl cation via the folding and cyclization of squalene, which forms the fifth ring of UA via ring extension and the formation of an additional ring. In the compound, there are three oxygen atoms, which activate double or triple neutral ligands and the transfer of electron pairs to the transition metal atom.Citation15
Figure 1 Structure of UA (PubChem ID: 64945).Citation16
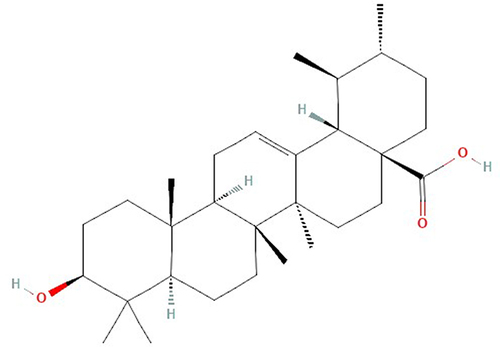
UA is a pentacyclic triterpene that is present widely in various medicinal plants, particularly in leaves and flowers, such as thyme and calendula.Citation17 Fruit skins, eg, apple and cambuca peels,Citation18,Citation19 are also known as an excellent source of UA since they are produced as protective coating against biotic and abiotic stresses, stomatal water-loss, and the leaching of organic and inorganic compounds.Citation20 Commercially dried fruit such as raisins and dried berries are also proven to contain UA.Citation21 Latest study conducted by Kalogeropoulos et al has also shown that several wild edible mushrooms contain UA although in a small concentration.Citation22
UA is a Biopharmaceutics Classification System (BCS) IV agent that has poor solubility and permeability. Its perceived permeability coefficient in human intestinal epithelial Caco-2 cell monolayers is 2.7×10−6 cm/s.Citation23 When UA was measured by LC-MS in rat plasma and tissue, it was discovered that the liver was the main location of UA metabolism and that it received significant pharmacokinetic properties, including a Tmax of 0.42 ± 0.11 h, a Cmax of 1.10 ± 0.31 μg/mL, an Area Under Curve (AUC) of 1.45 ± 0.21 μg·h/mL, and a Ka of 5.64 ± 1.89 /h.Citation24 After oral administration of a Sambucus chinensis L. extract containing UA at 80.32 mg/kg, LC-MS determined the pharmacokinetic parameters in rat plasma. The results demonstrated t1/2 at 4.3 h, elimination rate constant (Ke) at 0.16 h, Tmax at 1.0 h, Cmax at 294.8 ng/mL, AUC0-t at 1007.1 ng/mL, and AUC(0-t) at 1175.3 ng/mL.Citation25
Based on the results of earlier studies, the isolation of UA can be achieved using various extraction methods and a range of solvents.Citation26 Traditional extraction techniques such as maceration, soxhlet extraction, and heat reflux extraction have been predominantly utilized for extracting UA from fruits and medicinal plants.Citation26 Conventional solvents, including water, lower alcohols, alcohol-water mixtures, acetone, diethyl ether, chloroform, hexane, and ethyl acetate, have been compared for extracting UA from seeds and fruits.Citation27 Previous studies have also indicated that microwave-assisted extraction (MAE), ultrasonic-assisted extraction (USAE), and accelerated solvent extraction (ASE) can be employed to enhance extraction selectivity and reduce extraction times when obtaining UA from natural sources, resulting in favorable yields and recovery rates.Citation28
Despite being the current primary way to acquire UA on a large scale, extraction from medicinal plants is considered inefficient due to its high energy and time consumption, and results in low purity and yield percentage.Citation29–31 An alternative method available for producing UA is by using engineered microbes that express specific plant biosynthesis enzymes.Citation31,Citation32 Previous studies have conducted the manufacturing of UA using numerous microorganisms as biocatalyst, such as Saccharomyces cerevisiaeCitation30,Citation31 and Yarrowia lipolytica.Citation32
Yarrowia lipolytica has been found to produce the highest UA titer of 254.3 mg/L in 24-deep-well plates.Citation32 However, Saccharomyces cerevisiae is more convenient to work with and is considered an ideal host for producing natural products.Citation33 Initially, Saccharomyces cerevisiae only produced very low amounts of UA (0.1 mg/L) through the heterologous expression of CrAS and CrAO (CYP from Catharanthus roseus).Citation34 However, by using the right selection of CPRs and optimizing fermentation conditions, the UA titer in Saccharomyces cerevisiae reached 123.27 mg/L in a 5-L fermenter.Citation35 Latest study that used combinatorial metabolic engineering strategies by optimizing the CYP-CPR pair and redistributing the synthetic pathway has shown an increased result of UA titer (483.4 mg/L); furthermore, the separation of heterologous enzymes was eliminated through LD compartmentalization, and the NADPH regeneration system was strengthened, resulting in further increases in the titer of UA to 1132.9 mg/L in a 3-L fermenter.Citation30
UA as a New Treatment for Tuberculosis: Current Progress
In silico Study
Enoyl Acyl Carrier Protein Reductase Inhibitor
Enoyl acyl carrier protein reductase (InhA) is a fatty acid synthesis enzyme that belongs to the nicotinamide adenine dinucleotide (NADH) dependent acyl carrier protein reductase family. InhA is an essential enzyme involved in mycolic acid biosynthesis. In the extension phase of the fatty acid synthase (FAS) II pathway, this enzyme catalyzes the NADH-specific reduction of 2-trans-enoyl-ACP. InhA, a member of the NADH-dependent acyl carrier protein reductase family, is a critical enzyme in the synthesis of fatty acids, specifically mycolic acid.Citation4 The InhA enzyme is the target for the pro-drug of isoniazid (INH), which is catalyzed to become active by the enzyme catalase-peroxidase (KatG).Citation36
As a pentacyclic triterpenoid, UA also showed a good affinity to InhA with a binding energy of −9.2 kcal/mol. According to the dynamic simulation, the UA-inhA complex was relatively stable and established hydrogen bonds with Thr196 and Ile194 ().Citation37
Figure 2 Mechanisms of UA for anti-TB agent using computational methods (reproduced with permission from Ref. (Ramon-Luing et al, 2023),Citation38 ©2023 Pathogens; (Sundararajan and Muniyan, 2021),Citation39 ©2021 Molecular Biology Reports; (Jacobo-Delgado et al, 2023),Citation40 ©2023 Frontiers in Immunology. Created with Biorender.com. Retrieved from https://app.biorender.com/biorender-templates. Rv3377c, Diterpene cyclase; Rv3378, Tuberculosinyl adenosine transferase; FadA5, Acetyl-CoA acetyltransferase; InhA, Enoyl acyl carrier protein reductase.
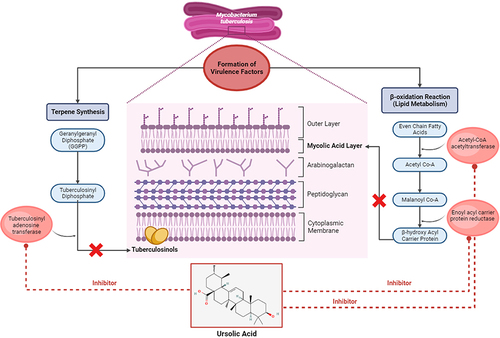
FadA5 (Acetyl-CoA Acetyltransferase) Inhibitor
During the chronic phase of TB infections, FadA5, a thiolase from MTB, catalyzes the final step of the β-oxidation reaction responsible for cholesterol side-chain degradation, thereby releasing essential metabolites. MTB eliminates cholesterol from the host to fulfil its metabolic needs, avoid host immunity, and facilitate macrophage infiltration. Bacteriostasis occurs as a consequence of the inhibition of the cholesterol catabolic pathways.Citation41,Citation42 Thus, it reveals that FadA5 might be a potential for drug development against TB.
UA was subjected to investigation for its interaction with FadA5 from MTB through the molecular dynamics and hybrid quantum mechanics approaches. Analysis of root mean square fluctuations (RMSF) demonstrated that the stability of the FadA5-UA complex exhibited greater fluctuations compared to FadA5 bound with OA and myristic acid (MA). The binding free energies followed a decreasing order as: FadA5-UA > FadA5-OA > FadA5-MA > FadA5-dBA. Furthermore, the key amino acids, namely Ser93, Leu128, Gln151, Val60, His347, and Val349 have optimal binding affinity at the active site of the FadA5 thiolase.Citation43
Tuberculosinyl Adenosine Transferase Inhibitor
Tuberculosinyl adenosine transferase, encoded by Rv3378c gene, is an MTB enzyme that is responsible for catalyzing the synthesis of tuberculosinyladenosine, a class of proteins that act as virulence factors in MTB infections and are also important for bacterial survival inside macrophages and bacterial wall biosynthesis. Inhibiting this enzyme could potentially lead to the depletion of MTB’s virulence factors, thereby hindering its pathogenicity.Citation44
Kataev et al investigated the activity of UA against Tuberculosinyl adenosine transferase. The structure of UA was found to be very well accommodated in the enzyme’s binding site. The analysis revealed that the compound interacts with a good binding energy (−9.8 kcal/mol) which indicates moderate antitubercular activities of UA.Citation45
Small Heat Shock Protein 16.3 Inhibitor
Using molecular docking and dynamics simulation, Jee et al demonstrated the ability of UA to inhibit MTB’s small heat shock protein 16.3 (sHSP16.3). This protein plays a role in MTB growth and development, pathogenicity, and thickening of the cell wall. It also serves as a molecular chaperone during stress and is critical for MTB survival in the host. Based on a molecular docking investigation, UA GLU56 and PRO58 formed two robust hydrogen bonds. The RMSD of the backbone plot and visual inspection of the bound structures revealed that the ligand-bound protein complex eventually converged to a stable structure on a time scale of 60 ns, indicating robust ligand binding without disrupting the native protein structure.Citation46
In vitro Study
Researchers have also conducted in vitro studies to investigate the potential anti-TB effect of UA. The UA has been reported to possess the potential for inhibiting mycobacterial cytochrome B subunit (QcrB) protein, outperforming lansoprazole sulphide and telacebec because of its high binding affinity to QcrB protein, established through the formation of hydrogen bonds and van der Waals forces with hydrophobic amino acids.Citation47 In addition, UA exhibited a significant antimycobacterial effect, with MIC value of up to 25 μg/mL against MTB strain H37Rv, including isoniazid-resistant (INH-R), rifampicin-resistant (RIF-R), and ethambutol-resistant (EMB-R) strains. This effect was attributed to the irregularities in the structure of the bacterial cell membrane.Citation47 Moreover, UA demonstrated a significant reduction in the number of viable bacilli present in the lungs and displayed diverse immunoregulatory activities (). UA also encoded and stimulated the production of cytokines such as IFN-γ, TNF-α, and iNOS.Citation14
Table 1 Several in vitro Essay of UA for Several Type of TB-Resistant
Mycobacterium avium, one of the closest relatives of MTB, offers a valuable model for researching MTB due to its tuberculosis-like effect on humans and immune tolerance of the host. The colony-forming unit analysis revealed that UA reduced the intracellular and extracellular presence of Mycobacterium avium in macrophages. During infection, UA downregulated the levels of TNF-α and IL-6 while upregulating the levels of IL-1 and nitrite. Additionally, it inhibited the phosphorylation of ERK1/2 but induced the phosphorylation in the C-Jun N-terminal kinase signaling pathway. This study provides experimental evidence on the potential application of UA and suggests that it may be a promising candidate for future TB treatment.Citation49
Pitaloka et al conducted susceptibility testing to determine the MIC of UA against various strains of MTB, including the H37Rv strain, streptomycin-rifampin resistant strain, and isoniazid-ethambutol resistant strain, all of which exhibited an MIC of 50 µg/mL. Interestingly, when UA was combined with conventional anti-TB medications, a synergistic interaction was observed, and no antagonistic effects were reported.Citation13
SEM micrographs of the MTB-sensitive H37Rv strain displayed distinguished signs of cell wall damage, characterized by deep craters, burst cells, lysis, and substantial wall disruption, leading to a distorted shape. Outer membrane thickening indicated severe damage in the MTB-sensitive H37Rv.Citation37 Moreover, TEM showed that UA severely affected the cell wall peptidoglycan of the H37Ra-sensitive strain of MTB. The entire PG layer detach from the cell body due to an osmotic imbalance. The condensation of nucleic acid and protein materials was also observed in the inner cytoplasm of the cell because of UA efflux in the cytoplasm.Citation12
Furthermore, the synergistic interaction between OA and UA exhibited potent antibacterial activity at lower concentrations against various MTB strains, including H37Rv, isoniazid-resistant, rifampicin-resistant, ethambutol-resistant, and streptomycin-resistant strains.Citation14 The OA synergy with UA was reported to be more effective than a single compound for producing the intended outcomes and several immunoregulatory activities enable the control of pulmonary TB, thus yielding new antibiotics and immunotherapeutic agents that are beneficial for TB treatment. Based on these reports, UA can be considered as a potential candidate for TB treatment and immunotherapy.
In vivo Study
Recently, in vivo studies related to the development of UA for anti-TB have been limited. Jiménez-Arellanes et al reported that the combination of UA and OA demonstrates both antimicrobial and immunomodulatory effects in TB treatment. In vivo studies using BALB/C infected with MDR clinical isolates showed that the combination of UA and OA has synergistic activity. This combination proved effective against intracellular mycobacteria, showing significantly lower lung bacillary loads at 1 month of treatment and improvement in lung histopathology. Moreover, the combination of UA and OA was associated with increased concentrations of cytokines such as IFN-γ, TNF-α, and enhanced iNOS expression in the lungs when compared to the control.Citation14
A metered-dose inhaler (MDI) was also developed combining UA and OA for treat TB. It was develop to encounter the major obstacle of the effective delivery of these compounds. The pharmacokinetics study reported that the MDI can increase the biological half-life of UA (9.23±0.104 h) and OA (8.93±0.166 h) in combination therapy. Toxicity study also found that no adverse effect on body weight and vital organs in the treatment group compared to control conducted on Wistar rats.Citation50
UA as a New Treatment for Tuberculosis: Challenges
Complex Chemical Composition
The contents of natural products include not only UA, but also many complex mixtures of compounds, complicating the identification and isolation of the specific active components responsible for therapeutic effects.Citation51 Purification of UA is problematic due to its structural and physicochemical similarity to its isomer, which makes separation challenging. Numerous analytical separation techniques, including HPLC,Citation29,Citation33 HPTLC,Citation32,Citation34 GC,Citation52 ion-exchange chromatographic,Citation53 and others, have been developed. This process can be time-consuming and the intricate structures of these compounds may lead to challenges in optimizing pharmacokinetics and bioavailability.Citation53,Citation54 Ensuring consistency of UA in the natural product quality, potency, and safety of natural products also becomes a formidable task.Citation55
Furthermore, natural products often exhibit lower bioavailability and stability compared to synthetic drugs due to their molecular complexity structure and their intricate structure.Citation56 This complexity can render them susceptible to degradation or metabolism before reaching their target site in the body, resulting in reduced efficacy. Their poor stability and bioavailability can limit the therapeutic potential of UA from natural products, requiring additional formulation strategies or delivery systems to enhance their pharmacokinetic properties.Citation57 To overcome these issues, formulating UA from natural products into stable,Citation58,Citation59 soluble,Citation60 and effective dosage formsCitation61 become imperative. Achieving consistent delivery and bioavailability while preserving efficacy presents a substantial challenge that necessitates innovative formulation strategies.
Limited Availability and Supply
UA from natural product is found in plants and herbs,Citation62,Citation63 cranberries,Citation64 roots of Actinidia Arguta (berry fruit tree)Citation65 and Urtica dioica leavesCitation66 which can be difficult to locate and harvest sustainably. This scarcity not only impacts the accessibility of UA from natural products for drug development but also raises concerns regarding their environmental impact and conservation. The limited availability of natural products can hinder large-scale production and commercialization.
Toxicity
Firstly, UA-induced inflammation needs extra attention. UA activates NF-ĸB in mouse macrophages, leading to an increase in nitric oxide and TNF-α production.Citation67 UA also interacts with CD36 to activate p38 MAPK, ERK1/2, and caspase-1, release ROS, and begin an inflammatory chain reaction in pMphi cells in the peritoneum of mice and the colonic mucosa of ICR mice. This led to an upregulation of interleukin-1β (IL-1β), a crucial pro-inflammatory mediator.Citation68 According to Ikeda et alCitation69 putting UA on the skin also raised the amounts of mRNA for TNF-α, COX-2, and cyclooxygenase (COX)-1 in mouse skin by a large amount. Another thing that UA did was turn on extracellular signal-regulated kinase 2 (ERK2). This caused resting RAW264.7 murine macrophages to produce pro-inflammatory chemicals, such as intracellular macrophage migration inhibitory factor (MIF).Citation70
However, no unfavorable outcomes are reported when UA is extensively used as a dietary supplement, which supports the idea that UA toxicity is closely linked to the dosage and duration of UA administration. With an LD50 of 9.26 g/kg in an acute mouse toxicity test,Citation71 sustained administration of an excessive dose of UA may frequently result in hepatic cytotoxicity,Citation72 not genetic toxicity. Nevertheless, further study is needed to investigate the processes underlying UA toxicity in specific circumstances.
Lack of Intellectual Property
The lack of intellectual property protection for natural products poses a significant hurdle in their development as drug therapies. Since natural products cannot be patented in their natural state, pharmaceutical companies may hesitate to invest in their research and development due to the absence of exclusive commercial rights. This issue can hinder the funding and incentivization necessary for the extensive clinical trials and regulatory processes required for drug approval, limiting the progression of natural products into the market as mainstream therapies.Citation73
Many patents were filed between 2012 and 2016 for UA and its synthetic analogs describing both their syntheses and therapeutic applications. Most of the patents along with antihyperlipidemic, antihypercholesterolemic, and anticardiovascular activities.Citation74 However, any pharmaceutical innovation of UA-derivatives is dependent on raw material supply.
In addition, natural products can vary significantly in terms of composition due to several factors such as environmental conditions, harvesting methods, geographical location, and many more. This variability poses challenges in achieving consistent and reproducible therapeutic effects across different batches of natural products. Variability in composition of natural products from different sources hinders reproducibility and consistency in therapeutic effects.Citation56
Conclusion and Future Perspective
UA holds substantial promise as a novel anti-TB agent, with a multifaceted approach to combating TB. Its diverse pharmacological properties, including antimicrobial activity, anti-inflammatory effects, and immunomodulatory capabilities, have been demonstrated in various in silico, in vitro, and in vivo studies. While the potential therapeutic benefits of UA are promising, several challenges and research gaps need to be addressed before it can be integrated into the clinical management of TB. In particular, the purification and standardization of UA, particularly in natural sources, present considerable hurdles. Structural similarity with isomers makes separation challenging, and optimizing pharmacokinetics and bioavailability remains a complex task. Furthermore, the limited clinical data available necessitates more comprehensive human trials to establish its efficacy, safety, and optimal dosing regimens.
In the coming years, there are several important directions to consider regarding the potential use of UA as a treatment for TB. Firstly, rigorous clinical trials will be essential to thoroughly evaluate UA’s effectiveness, safety, and the best ways to administer it to patients. These trials will provide precise information on its therapeutic benefits. Secondly, research into advanced methods for formulating UA-based treatments will help improve how the drug is absorbed and used by the body, ensuring consistent and reliable results in various forms of medication. Exploring the combination of UA with existing TB drugs may offer a more effective treatment approach while reducing the risk of drug resistance development. Additionally, continuous monitoring for the development of resistance to UA, both in the MTB and other related microbes, is crucial to prevent the spread of drug resistance. Lastly, ensuring the sustainability of UA from natural origins will be important both for preserving the environment and making sure the compound remains available for future use.
Disclosure
The authors declare that they have no known competing financial interests or personal relationships that could have appeared to influence the work reported in this paper.
Acknowledgments
This study was funded by Directorate of Research and Community Service Universitas Padjadjaran, grant numbers 2361/UN6.3.1/PT.00/2024.
References
- Global tuberculosis report; 2022. Available from: https://www.who.int/teams/global-tuberculosis-programme/tb-reports/global-tuberculosis-report-2022. Accessed September 2, 2023.
- Chinthanom P, Vichai V, Dokladda K, Sappan M, Thongpanchang C, Isaka M. Semisynthetic modifications of antitubercular lanostane triterpenoids from Ganoderma. J Antibiot. 2021;74(7):435–442. doi:10.1038/s41429-021-00422-5
- Ku CM, Lin JY. Anti-inflammatory effects of 27 selected terpenoid compounds tested through modulating Th1/Th2 cytokine secretion profiles using murine primary splenocytes. Food Chem. 2013;141(2):1104–1113. doi:10.1016/j.foodchem.2013.04.044
- Shanmugam MK, Dai X, Kumar AP, Tan BKH, Sethi G, Bishayee A. Ursolic acid in cancer prevention and treatment: molecular targets, pharmacokinetics and clinical studies. Biochem Pharmacol. 2013;85(11):1579–1587. doi:10.1016/j.bcp.2013.03.006
- Liobikas J, Majiene D, Trumbeckaite S, et al. Uncoupling and antioxidant effects of ursolic acid in isolated rat heart mitochondria. J Nat Prod. 2011;74(7):1640–1644. doi:10.1021/np200060p
- D’Abrosca B, Fiorentino A, Monaco P, Pacifico S. Radical-scavenging activities of new hydroxylated ursane triterpenes from cv. Annurca apples. Chem Biodivers. 2005;2(7):953–958. doi:10.1002/cbdv.200590072
- Ali MS, Ibrahim SA, Jalil S, Choudhary MI. Ursolic acid: a potent inhibitor of superoxides produced in the cellular system. Phytother Res PTR. 2007;21(6):558–561. doi:10.1002/ptr.2108
- Mallavadhani UV, Mahapatra A, Jamil K, Reddy PS. Antimicrobial activity of some pentacyclic triterpenes and their synthesized 3-O-lipophilic chains. Biol Pharm Bull. 2004;27(10):1576–1579. doi:10.1248/bpb.27.1576
- e Silva M de L, David JP, Silva LCRC, et al. Bioactive oleanane, lupane and ursane triterpene acid derivatives. Mol. 2012;17(10):12197–12205. doi:10.3390/molecules171012197
- Kurek A, Nadkowska P, Pliszka S, Wolska KI. Modulation of antibiotic resistance in bacterial pathogens by oleanolic acid and ursolic acid. Phytomedicine Int J Phytother Phytopharm. 2012;19(6):515–519. doi:10.1016/j.phymed.2011.12.009
- Martins D, Carrion LL, Ramos DF, et al. Triterpenes and the antimycobacterial activity of duroia macrophylla Huber (Rubiaceae). BioMed Res Int. 2013;2013:e605831. doi:10.1155/2013/605831
- Jyoti M, Zerin T, Kim TH, et al. In vitro effect of ursolic acid on the inhibition of Mycobacterium tuberculosis and its cell wall mycolic acid. Pulm Pharmacol Ther. 2015;33:17–24. doi:10.1016/j.pupt.2015.05.005
- Pitaloka DAE, Sukandar EY. In vitro study of ursolic acid combination first-line antituberculosis drugs against drug-sensitive and drug-resistant strains of Mycobacterium tuberculosis. Asian J Pharm Clin Res. 2017;216–218. doi:10.22159/ajpcr.2017.v10i4.16582
- Jiménez-Arellanes A, Luna-Herrera J, Cornejo-Garrido J, et al. Ursolic and oleanolic acids as antimicrobial and immunomodulatory compounds for tuberculosis treatment. BMC Complement Altern Med. 2013;13:258. doi:10.1186/1472-6882-13-258
- Kashyap D, Tuli HS, Sharma AK. Ursolic acid (UA): a metabolite with promising therapeutic potential. Life Sci. 2016;146:201–213. doi:10.1016/j.lfs.2016.01.017
- PubChem. Ursolic Acid. Available from: https://pubchem.ncbi.nlm.nih.gov/compound/64945. Accessed September 4, 2023.
- Nguyen HN, Ullevig SL, Short JD, Wang L, Ahn YJ, Asmis R. Ursolic acid and related analogues: triterpenoids with broad health benefits. Antioxidants. 2021;10(8):1161. doi:10.3390/antiox10081161
- da Rosa RL, Nesello LÂN, Mariano LNB, et al. Gastroprotective activity of the methanol extract from peels of Plinia edulis (Vell.) Sobral fruits and its isolated triterpenes: maslinic and ursolic acids. Naunyn Schmiedebergs Arch Pharmacol. 2018;391(1):95–101. doi:10.1007/s00210-017-1442-8
- Lv Y, Tahir II, Olsson ME. Factors affecting the content of the ursolic and oleanolic acid in apple peel: influence of cultivars, sun exposure, storage conditions, bruising and Penicillium expansum infection. J Sci Food Agric. 2016;96(6):2161–2169. doi:10.1002/jsfa.7332
- Szakiel A, Pączkowski C, Pensec F, Bertsch C. Fruit cuticular waxes as a source of biologically active triterpenoids. Phytochem Rev Proc Phytochem Soc Eur. 2012;11(2–3):263–284. doi:10.1007/s11101-012-9241-9
- Oleanolic acid and ursolic acid in commercial dried fruits. Available from: https://www.jstage.jst.go.jp/article/fstr/19/1/19_113/_article. Accessed September 21, 2023.
- Kalogeropoulos N, Yanni AE, Koutrotsios G, Aloupi M. Bioactive microconstituents and antioxidant properties of wild edible mushrooms from the island of Lesvos, Greece. Food Chem Toxicol Int J Publ Br Ind Biol Res Assoc. 2013;55:378–385. doi:10.1016/j.fct.2013.01.010
- Qiang Z, Ye Z, Hauck C, et al. Permeability of rosmarinic acid in Prunella vulgaris and ursolic acid in Salvia officinalis extracts across Caco-2 cell monolayers. J Ethnopharmacol. 2011;137(3):1107–1112. doi:10.1016/j.jep.2011.07.037
- Chen Q, Luo S, Zhang Y, Chen Z. Development of a liquid chromatography-mass spectrometry method for the determination of ursolic acid in rat plasma and tissue: application to the pharmacokinetic and tissue distribution study. Anal Bioanal Chem. 2011;399(8):2877–2884. doi:10.1007/s00216-011-4651-x
- Liao Q, Yang W, Jia Y, Chen X, Gao Q, Bi K. LC-MS determination and pharmacokinetic studies of ursolic acid in rat plasma after administration of the traditional Chinese medicinal preparation Lu-Ying extract. Yakugaku Zasshi. 2005;125(6):509–515. doi:10.1248/yakushi.125.509
- López-Hortas L, Pérez-Larrán P, González-Muñoz MJ, Falqué E, Domínguez H. Recent developments on the extraction and application of ursolic acid. A review. Food Res Int. 2018;103:130–149. doi:10.1016/j.foodres.2017.10.028
- Xia E-Q, Yu -Y-Y, Xu X-R, Deng G-F, Guo Y-J, Li H-B. Ultrasound-assisted extraction of oleanolic acid and ursolic acid from Ligustrum lucidum Ait. Ultrason Sonochem. 2012;19(4):772–776. doi:10.1016/j.ultsonch.2011.11.014
- Wójciak-Kosior M, Sowa I, Kocjan R, Nowak R. Effect of different extraction techniques on quantification of oleanolic and ursolic acid in Lamii albi flos. Ind Crops Prod. 2013;44:373–377. doi:10.1016/j.indcrop.2012.11.018
- Idris FN, Nadzir MM. Comparative studies on different extraction methods of Centella asiatica and extracts bioactive compounds effects on antimicrobial activities. Antibiotics. 2021;10(4):457. doi:10.3390/antibiotics10040457
- Jin K, Shi X, Liu J, et al. Combinatorial metabolic engineering enables the efficient production of ursolic acid and oleanolic acid in Saccharomyces cerevisiae. Bioresour Technol. 2023;374:128819. doi:10.1016/j.biortech.2023.128819
- Liu X, Ding W, Jiang H. Engineering microbial cell factories for the production of plant natural products: from design principles to industrial-scale production. Microb Cell Fact. 2017;16(1):125. doi:10.1186/s12934-017-0732-7
- Arnesen JA, Belmonte Del Ama A, Jayachandran S, et al. Engineering of Yarrowia lipolytica for the production of plant triterpenoids: asiatic, madecassic, and arjunolic acids. Metab Eng Commun. 2022:14:e00197. doi:10.1016/j.mec.2022.e00197
- Wang S, Zhao F, Yang M, Lin Y, Han S. Metabolic engineering of Saccharomyces cerevisiae for the synthesis of valuable chemicals. Crit Rev Biotechnol. 2023;1–28. doi:10.1080/07388551.2022.2153008
- Huang L, Li J, Ye H, et al. Molecular characterization of the pentacyclic triterpenoid biosynthetic pathway in Catharanthus roseus. Planta. 2012;236(5):1571–1581. doi:10.1007/s00425-012-1712-0
- Lu C, Zhang C, Zhao F, Li D, Lu W. Biosynthesis of ursolic acid and oleanolic acid in Saccharomyces cerevisiae. AiChE J. 2018;64(11):3794–3802. doi:10.1002/aic.16370
- Unissa AN, Subbian S, Hanna LE, Selvakumar N. Overview on mechanisms of isoniazid action and resistance in Mycobacterium tuberculosis. Infect Genet Evol J Mol Epidemiol Evol Genet Infect Dis. 2016;45:474–492. doi:10.1016/j.meegid.2016.09.004
- Pitaloka DAE, Damayanti S, Artarini AA, Sukandar EY. Molecular Docking, Dynamics Simulation, and Scanning Electron Microscopy (SEM) Examination of Clinically Isolated Mycobacterium tuberculosis by Ursolic Acid: a Pentacyclic Triterpenes. Indones J Chem. 2019;19(2):328–336. doi:10.22146/ijc.33731
- Ramon-Luing LA, Palacios Y, Ruiz A, Tellez-Navarrete NA, Chavez-Galan L. Virulence factors of mycobacterium tuberculosis as modulators of cell death mechanisms. Pathogens. 2023;12(6):839. doi:10.3390/pathogens12060839
- Sundararajan S, Muniyan R. Latent tuberculosis: interaction of virulence factors in Mycobacterium tuberculosis. Mol Biol Rep. 2021;48(8):6181–6196. doi:10.1007/s11033-021-06611-7
- Jacobo-Delgado YM, Navarro-Tovar G, Rivas-Santiago B. Potential use of liposomes in tuberculosis treatment. Rev Medica Inst Mex Seguro Soc. 2023;61(5):661–669. doi:10.5281/zenodo.8316467
- Schaefer CM, Lu R, Nesbitt NM, Schiebel J, Sampson NS, Kisker C. FadA5 a thiolase from mycobacterium tuberculosis: a steroid-binding pocket reveals the potential for drug development against tuberculosis. Structure. 2015;23(1):21–33. doi:10.1016/j.str.2014.10.010
- Nesbitt NM, Yang X, Fontán P, et al. A thiolase of mycobacterium tuberculosis is required for virulence and production of androstenedione and androstadienedione from cholesterol. Infect Immun. 2010;78(1):275–282. doi:10.1128/IAI.00893-09
- Tolufashe GF, Lawal MM, Govender KK, Shode FO, Singh T. Exploring the bioactivity of pentacyclic triterpenoids as potential antimycobacterial nutraceutics: insights through comparative biomolecular modelling. J Mol Graph Model. 2021;105:107900. doi:10.1016/j.jmgm.2021.107900
- Chan H-C, Feng X, Ko T-P, et al. Structure and inhibition of tuberculosinol synthase and decaprenyl diphosphate synthase from Mycobacterium tuberculosis. J Am Chem Soc. 2014;136(7):2892–2896. doi:10.1021/ja413127v
- Shetye GS, Franzblau SG, Cho S. New targets for growth inhibition of mycobacterium tuberculosis: why do natural terpenoids exhibit antitubercular activity? Transl Res. 2020;229:68–97. doi:10.1016/j.trsl.2020.03.007
- Jee B, Kumar S, Yadav R, Singh Y, Kumar A, Sharma N. Ursolic acid and carvacrol may be potential inhibitors of dormancy protein small heat shock protein 16.3 of Mycobacterium tuberculosis. J Biomol Struct Dyn. 2018;36(13):3434–3443. doi:10.1080/07391102.2017.1389305
- Tembe N, Machaba KE, Ndagi U, Kumalo HM, Mhlongo NN. Ursolic acid as a potential inhibitor of Mycobacterium tuberculosis cytochrome bc1 oxidase-A molecular modelling perspective. J Mol Model. 2022;28(2):35. doi:10.1007/s00894-021-04993-w
- Singh A, Venugopala KN, Khedr MA, et al. Antimycobacterial, docking and molecular dynamic studies of pentacyclic triterpenes from Buddleja saligna leaves. J Biomol Struct Dyn. 2017;35(12):2654–2664. doi:10.1080/07391102.2016.1227725
- Pitaloka DAE, Cooper AM, Artarini AA, Damayanti S, Sukandar EY. Regulation of mitogen-activated protein kinase signaling pathway and proinflammatory cytokines by ursolic acid in murine macrophages infected with Mycobacterium avium. Infect Dis Rep. 2020;12:8717. doi:10.4081/idr.2020.8717
- Saini V, Debnath SK, Maske P, Dighe V, Srivastava R. Targeted delivery of ursolic acid and oleanolic acid to lungs in the form of an inhaler for the management of tuberculosis: pharmacokinetic and toxicity assessment. PLoS One. 2022;17(12):e0278103. doi:10.1371/journal.pone.0278103
- Motika SE, Hergenrother PJ. Re-engineering natural products to engage new biological targets. Nat Prod Rep. 2020;37(11):1395–1403.
- Jacobo-Delgado YM, Rodríguez-Carlos A, Serrano CJ, Rivas-Santiago B. Mycobacterium tuberculosis cell-wall and antimicrobial peptides: a mission impossible? Front Immunol. 2023;14:1194923. doi:10.3389/fimmu.2023.1194923
- Mopper K. Improved chromatographic separations on anion-exchange resins. II. Separation of uronic acids in acetate medium and detection with a noncorrosive reagent. Anal Biochem. 1978;86(2):597–601. doi:10.1016/0003-2697(78)90787-x
- Bandžuchová L, Šelešovská R. Voltammetric determination of folic Acid using liquid mercury free silver amalgam electrode. Acta Chim Slov. 2011;58(4).
- Ullevig SL, Zhao Q, Zamora D, Asmis R. Ursolic acid protects diabetic mice against monocyte dysfunction and accelerated atherosclerosis. Atherosclerosis. 2011;219(2):409–416. doi:10.1016/j.atherosclerosis.2011.06.013
- Okumu MO, Ochola FO, Onyango AO, et al. The legislative and regulatory framework governing herbal medicine use and practice in Kenya: a review. Pan Afr Med J. 2017;28:232. doi:10.11604/pamj.2017.28.232.12585
- Feng Z, Wu C, Zhou J, et al. Disturbance of the intestinal microbial community by ursolic acid contributes to its function as a regulator of fat deposition. J Funct Foods. 2015;14:456–468. doi:10.1016/j.jff.2015.02.015
- Kumadoh D, Ofori-Kwakye K. Dosage forms of herbal medicinal products and their stability considerations-an overview. J Crit Rev. 2017;4(4):1–8. doi:10.22159/JCR.2017V4I4.16077
- Puthli S, Vavia PR. Stability studies of microparticulate system with piroxicam as model drug. AAPS Pharm Sci Tech. 2009;10(3):872. doi:10.1208/s12249-009-9280-8
- Eloy JO, Marchetti JM. Solid dispersions containing ursolic acid in Poloxamer 407 and PEG 6000: a comparative study of fusion and solvent methods. Powder Technol. 2014;253:98–106. doi:10.1016/j.powtec.2013.11.017
- Ward A, Brogden RN, Heel RC, Speight TM, Avery GS. Ursodeoxycholic Acid. Drugs. 1984;27(2):95–131. doi:10.2165/00003495-198427020-00001
- Li J, Guo WJ, Yang QY. Effects of ursolic acid and oleanolic acid on human colon carcinoma cell line HCT15. World J Gastroenterol. 2002;8(3):493–495. doi:10.3748/wjg.v8.i3.493
- Fontanay S, Grare M, Mayer J, Finance C, Duval RE. Ursolic, oleanolic and betulinic acids: antibacterial spectra and selectivity indexes. J Ethnopharmacol. 2008;120(2):272–276. doi:10.1016/j.jep.2008.09.001
- Kondo M, MacKinnon SL, Craft CC, Matchett MD, Hurta RAR, Neto CC. Ursolic acid and its esters: occurrence in cranberries and other Vaccinium fruit and effects on matrix metalloproteinase activity in DU145 prostate tumor cells. J Sci Food Agric. 2011;91(5):789–796. doi:10.1002/jsfa.4330
- Kim J, Jang DS, Kim H, Kim JS. Anti-lipase and lipolytic activities of ursolic acid isolated from the roots of Actinidia arguta. Arch Pharm Res. 2009;32(7):983–987. doi:10.1007/s12272-009-1702-3
- Estimation of ursolic acid from Urtica dioica L. using validated HPTLC method. Available from: https://japsonline.com/abstract.php?article_id=1254&sts=2. Accessed September 20, 2023.
- You HJ, Choi CY, Kim JY, Park SJ, Hahm KS, Jeong HG. Ursolic acid enhances nitric oxide and tumor necrosis factor-alpha production via nuclear factor-kappaB activation in the resting macrophages. FEBS Lett. 2001;509(2):156–160. doi:10.1016/s0014-5793(01)03161-1
- Ikeda Y, Murakami A, Fujimura Y, et al. Aggregated ursolic acid, a natural triterpenoid, induces IL-1beta release from murine peritoneal macrophages: role of CD36. J Immunol Baltim Md. 2007;178(8):4854–4864. doi:10.4049/jimmunol.178.8.4854
- Ikeda Y, Murakami A, Nishizawa T, Ohigashi H. Ursolic acid enhances cyclooxygenases and tumor necrosis factor-α expression in mouse skin. Biosci Biotechnol Biochem. 2006;70(4):1033–1037. doi:10.1271/bbb.70.1033
- Ikeda Y, Murakami A, Ohigashi H. Ursolic acid promotes the release of macrophage migration inhibitory factor via ERK2 activation in resting mouse macrophages. Biochem Pharmacol. 2005;70(10):1497–1505. doi:10.1016/j.bcp.2005.08.008
- Acute and genetic toxicity of ursolic acid extract from ledum pulastre L. Available from: http://www.spkx.net.cn/EN/abstract/abstract10799.shtml. Accessed June 24, 2019.
- Danielewicz A, Morze J, Przybyłowicz M, Przybyłowicz KE. Association of the dietary approaches to stop hypertension, physical activity, and their combination with semen quality: a cross-sectional study. Nutrients. 2020;12(1):39. doi:10.3390/nu12010039
- Parveen A, Parveen B, Parveen R, Ahmad S. Challenges and guidelines for clinical trial of herbal drugs. J Pharm Bioallied Sci. 2015;7(4):329–333. doi:10.4103/0975-7406.168035
- Hussain H, Green IR, Ali I, et al. Ursolic acid derivatives for pharmaceutical use: a patent review (2012–2016). Expert Opin Ther Pat. 2017;27(9):1061–1072. doi:10.1080/13543776.2017.1344219