Abstract
Ferroptosis, a unique form of programmed cell death, is initiated by an excess of iron accumulation and lipid peroxidation-induced damage. There is a growing body of evidence indicating that ferroptosis plays a critical role in the advancement of tumors. The increased metabolic activity and higher iron levels in tumor cells make them particularly vulnerable to ferroptosis. As a result, the targeted induction of ferroptosis is becoming an increasingly promising approach for cancer treatment. This review offers an overview of the regulatory mechanisms of ferroptosis, delves into the mechanism of action of traditional small molecule ferroptosis inducers and their effects on various tumors. In addition, the latest progress in inducing ferroptosis using new means such as proteolysis-targeting chimeras (PROTACs), photodynamic therapy (PDT), sonodynamic therapy (SDT) and nanomaterials is summarized. Finally, this review discusses the challenges and opportunities in the development of ferroptosis-inducing agents, focusing on discovering new targets, improving selectivity, and reducing toxic and side effects.
Introduction
The latest data released by the International Agency for Research on Cancer (IARC) indicates that there were 20 million new cancer cases globally in 2022, resulting in 9.7 million deaths. This data highlights the increasing global burden of cancer.Citation1 However, the effectiveness of existing cancer treatments is concerning, as tumor cell resistance is reducing the efficacy of conventional therapies like radiotherapy and chemotherapy.Citation2 Additionally, refractory cancers like small cell lung cancer (SCLC),Citation3 triple-negative breast cancer (TNBC),Citation4 and pancreatic cancerCitation5 present significant challenges in oncology treatment due to their resistance to existing therapies. Ferroptosis, a novel iron-dependent form of programmed cell death first proposed by Dr. Brent R. Stockwell in 2012, is distinct from apoptosis, necrosis, and autophagy.Citation6 It is triggered by glutathione (GSH) depletion, decreased activity of glutathione peroxidase 4 (GPX4), failure of lipid oxides to be metabolized by GPX4, and Fe2+-mediated oxidation of lipids leading to the generation of reactive oxygen species (ROS) that promote ferroptosis.Citation7 Ferroptosis provides a new way of thinking about cancer treatment. Ferroptosis induction therapy has several natural advantages for cancer treatment. Firstly, the unique metabolism of cancer cells, the high load of ROS, and their specific mutations make some of them sensitive to ferroptosis,Citation8 eg, clear cell renal cell carcinoma (ccRCC),Citation9 pancreatic ductal adenocarcinoma,Citation10 and TNBC.Citation11 Cancer cells typically require more iron than normal cells to proliferate, and this iron dependence makes them more susceptible to ferroptosis.Citation12 Cancer cells with a high mesenchymal state were found to be more resistant to a wide range of cancer treatments but were particularly susceptible to ferroptosis inducers.Citation13 Also, ferroptosis induction therapy can be used in combination with conventional tumor treatments to enhance their therapeutic effects in certain malignancies.Citation14
Ferroptosis-inducing therapies have emerged as a crucial strategy in oncology, with ongoing advancements in the development and study of various small molecule drugs targeting ferroptosis-regulating pathways.Citation15 This review provides an overview of the regulatory mechanisms of ferroptosis and delves into the mechanisms of traditional small molecule ferroptosis inducers and their effects on various tumors. It also highlights the recent progress in inducing ferroptosis through innovative methods such as PROTACs, PDT, SDT, and nanomaterials. Furthermore, the review addresses the challenges and opportunities in developing ferroptosis-inducing agents, including the exploration of new targets, enhancement of selectivity, and mitigation of toxic and side effects ().
Ferroptosis Mechanism
Ferroptosis is a new form of cell death characterized by iron-dependent accumulation of lipid peroxidation to lethal levels.Citation16 It has been shown that in mammalian cells, ferroptosis is mainly regulated by iron homeostasis, lipid metabolism, and glutathione-dependent redox homeostasis ().
Figure 2 Ferroptosis mechanisms and their associated inducers of ferroptosis.
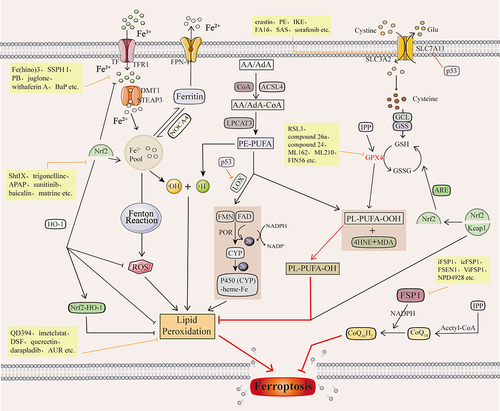
Iron Homeostasis
Iron is a trace element essential for maintaining the life of living organisms. It is involved in various metabolic pathways and exists in the human body mainly in Fe2+ and Fe3+.Citation17 Two molecules of Fe3+ can bind to a transferrin (TRF) for transport in vivo and transport iron into the cell by binding to the transferrin receptor TFR1 on the cell surface to form the TF-Fe3+-TFR1 complex.Citation18 Fe3+ is reduced to Fe2+ by the Six-Transmembrane Epithelial Antigen of Prostate 3 (STEAP3),Citation19 which accumulates in cells to form the labile iron pool (LIP).Citation20 SLC11A2 regulates this process. Fe2+ in LIP participates in the Fenton reaction to generate reactive oxygen species (ROS) substances represented by hydroxyl radicals (·OH).Citation21 Accumulated ROS will undergo lipid peroxidation, resulting in loss of cellular function and cellular ferroptosis.Citation22 Autophagic ferritin degradation in lysosomes mediated by nuclear receptor coactivator 4 (NCOA4) can induce ferroptosis by releasing free iron from ferritin.Citation23 In iron metabolism, the Feature Pyramid Network (FPN) is the only protein that can translocate iron from the cell, thereby reducing the intracellular Fe2+ concentration.Citation24
Lipid Metabolism
Lipid metabolism is strongly associated with ferroptosis.Citation25 Lipid peroxidation, a process in which oxygen combines with lipids to form peroxyl radicals to produce lipid peroxides, is critical to the onset of ferroptosis.Citation26 Polyunsaturated fatty acids (PUFAs) containing two or more double bonds are involved in the lipid oxidation process of ferroptosis.Citation27 Arachidonic acid (AA) or adrenaline (AdA) was acylated to AA/AdA-CoA by Abstract Long-chain acyl-coenzyme A (CoA) synthase 4 (ACSL4) and then by Lysophosphatidylcholine acyltransferase 3 (LPCAT3) to PE-AA/AdA.Citation28 PE-AA/AdA is involved in downstream ferroptosis’s non-enzymatic and enzymatic oxidation reactions.Citation29 Non-enzymatic reactions refer to the reaction of ·OH produced by the Fenton reaction with lipids to form lipid peroxides, which eventually undergo lipid peroxidation, leading to ferroptosis.Citation30 Enzymatic reactions include two kinds. One is that PE-PUFA is catalyzed by lipoxygenase (LOX) to form toxic products such as Malondialdehyde (MDA) and 4-Hydroxynonenal (4-HNE), which react with DNA bases, proteins, and other nucleophilic molecules to cause severe harmful effects.Citation31 The other is Cytochrome P450 reductase (POR), which promotes lipid peroxidation and leads to ferroptosis.Citation32 Thus, ACSL4, LOX, and LPCAT3 are compelling targets for the action of ferroptosis inducers.
Antioxidant Pathways
The intracellular antioxidant pathway resists the onset of ferroptosis by neutralizing lipid peroxides and is a critical ferroptosis defense system in the body.Citation33 Inhibiting antioxidant pathways disrupts ferroptosis defenses, leading to the induction of ferroptosis. Described below are a few of the major pathways involved in the induction of ferroptosis.
Xc−-GSH-GPX4
The Xc−-GSH-GPX4 axis is a central regulatory pathway for ferroptosis.Citation34 The Cystine/Glutamate Antiporter (System Xc−) is on the cytoplasmic membrane. It contains solute carrier family 3 member 2 Gene (SLC3A2) and solute carrier family 7 member 11 Gene (SLC7A11, xCT), which is responsible for the transfer of cysteine(Cys) into the cell and glutamate(Glu) out of the cell.Citation35 Cysteine that is moved into the cell by system Xc− can synthesize glutathione (GSH), which has antioxidant properties and strong reducing properties, with glutamate and glycine catalyzed by Glutathione synthetase (GSS) and Glutamate-cysteine ligase (GCL).Citation36 GPX4 converts GSH in cells to oxidized glutathione (GSSG).Citation37 In this process, GSH acts as an electron donor to convert toxic lipid peroxides in the cell to non-toxic Lipid alcohol (PL-PUFA-OH).Citation38 GSH and GPX4 are essential for maintaining intracellular redox balance and inhibiting the occurrence of ferroptosis. Inhibition of GSH synthesis through inhibition of system Xc− and direct inhibition of GPX4 are important mechanisms of action of ferroptosis inducers. Selenocysteine is one of the essential amino acids for the active group of GPX4.Citation39 Thus, selenocysteine is an important target for a variety of GPX4 inhibitors.
FSP1-CoQ10-NAD (P) H Pathway
The FSP1-CoQ10-NAD(P)H pathway and the GSH-GPX4 pathway are two parallel lipid antioxidant pathways.Citation40 CoQ10 (ubiquinone) is a lipophilic free radical trapping antioxidant that blocks the propagation of lipid peroxides and inhibits ferroptosis.Citation41 Its reduced form of CoQ10H2 (panthenol) reduces oxidative stress and inhibits lipid ROS accumulation.Citation42 Ferroptosis suppressor protein 1 (FSP1) reduces CoQ10 to CoQ10H2 in response to NADPH and catalyzes the regeneration of CoQ10 via NAD(P)H.Citation43 The FSP1-CoQ10-NAD(P)H pathway exerts antioxidant effects independently of the system Xc−-GSH-GPX4 axis and inhibits ferroptosis. Inhibition of FSP1 blocks the antioxidant effects of the FSP1-CoQ10-NAD(P)H pathway, thereby inducing ferroptosis.Citation40
Nrf2/ARE-GPX4 Pathway
Nuclear factor erythroid 2-related factor 2 (Nrf2) plays a crucial role in cellular antioxidant capacity. Nrf2 regulates the expression of antioxidant and pro-electrical stress genes. It also participates in other important pathways such as lipid metabolism, iron homeostasis and energy metabolism.Citation44 Kelch-like ECH-associated protein 1 (Keap1) is an active inhibitor of Nrf2. Under normal conditions, Nrf2 binds to Keap1. Under oxidative stress, Nrf2 is detached from the Keap1 binding site. It rapidly translocates to the nucleus, interacting with the Antioxidant Response Element (ARE) in the promoter region of mRNAs encoding detoxification enzymes and cytoprotective genes to enhance the expression of downstream target genes.Citation45 The downstream target genes of Nrf2 include genes related to iron metabolism, genes related to NADPH regeneration, and genes related to GSH metabolism.Citation46 Activation of these target genes will effectively maintain the redox homeostasis of the cell. Nrf2 also binds to Heme Oxygenase-1(HO-1) and inhibits the occurrence of lipid peroxidation.Citation47 Furthermore, activation of Nrf2 reduces cellular iron uptake, increases iron storage, and limits ROS production.Citation48 This shows that Nrf2 plays an important protective role against ferroptosis. Inhibition of Nrf2 is also a very important mechanism of action for ferroptosis inducers.Citation49
Mevalonate Pathway
The Mevalonate pathway (MVA pathway) includes the generation of isopentenyl pyrophosphate (IPP), squalene and CoQ10.Citation50 Selenocysteine is one of the amino acids in the active center of GPX4, and embedding it in GPX4 requires a special transporter, selenocysteine tRNA.Citation51 IPP promotes selenocysteine tRNA maturation and GPX4 synthesis. Also, IPP is a precursor of CoQ10 and can synthesize CoQ10 in the presence of acetyl-coenzyme A (Acetyl-CoA).Citation52 Thus, the MVA pathway connects the system Xc−-GSH-GPX4 axis and the NADPH-FSP1-CoQ10 pathway.
p53 Gene
The p53 gene is a classical oncogene. As a transcription factor, p53 activates or represses the transcription of multiple downstream target genes to perform its function.Citation53 The p53 can transcriptionally repress SLC7A11 expression at the transcriptional level, thereby promoting the occurrence of ferroptosis in cells.Citation54 Also, p53 releases Arachidonate 12-lipoxygenase (ALOX12). Free ALOX12 oxidizes cell membrane phospholipids’ polyunsaturated fatty acid chains, leading to ferroptosis.Citation55
Ferroptosis Inducers
Traditional Small Molecular Compounds
With the increasing research on ferroptosis, more and more inducers targeting ferroptosis have been identified and developed. Traditional small molecular compounds are an essential component of the ferroptosis inducer field. The following section will summarise these ferroptosis inducers in terms of the pathways of action of these drugs ().
Table 1 Small Molecular Ferroptosis Inducers
Targeting Antioxidant Pathways
Targeting System Xc−
The synthesis of glutathione (GSH) heavily relies on the cystine imported into the cell through system Xc−.Citation130 Hence, system Xc− significantly influences the progression of ferroptosis. Conversely, the inhibitory function of system Xc− hinders cystine uptake, leading to decreased GSH production, cellular stress, and ultimately triggering ferroptosis.Citation131
Erastin, a well-known inducer of ferroptosis, was first discovered in 2003 by Dolma et al.Citation132 This quinazolinone derivative inhibits system Xc− by targeting SLC7A11.Citation133 Moreover, erastin promotes oxidation and disruption of the mitochondrial permeability transition pore (mPTP) and acts through the Voltage-Dependent Anion Channel (VDAC), demonstrating anti-tumour effects.Citation134 Erastin effectively triggers ferroptosis in various cell types, including human promyelocytic leukemia cells (HL60),Citation135 endometriotic ectopic cyst cells (EESC),Citation136 and renal tubular cells.Citation137 Not only does erastin induce ferroptosis in cancer cells, but it also enhances the efficacy of traditional anticancer drugs like adriamycin,Citation138 cisplatin,Citation139 temozolomide,Citation140 and cytarabineCitation141 on specific cancer cell lines. However, Erastin’s limitations in stability, water solubility, and pharmacokinetic properties render it unsuitable for in vivo applications.Citation142
The quinazolinone present in erastin’s structure is crucial for its cell-inhibitory effects. By modifying the structure of erastin, researchers have developed several drugs that outperform erastin. Wan Seok Yang et al incorporated a Piperazine Fragment into erastin’s aniline to create Piperazine erastin (PE).Citation59 PE exhibits higher water solubility and metabolic stability compared to erastin (water solubility: 0.086 × 10−3M for erastin, 1.4 × 10−3M for PE). Imidazole ketone erastin (IKE) is an erastin analogue containing a carbonyl group, designed by Marie-Helene Larraufie et al.Citation143 IKE induces ferroptosis and demonstrates anti-tumour effects in a diffuse large B cell lymphoma (DLBCL) xenograft model.Citation144 IKE shows a superior inhibition rate and metabolic stability when compared to erastin, making it more suitable for in vivo assessments and offering promising application prospects.Citation144
Yuying Fang et al utilized phenotypic screening to discover a novel ferroptosis inducer known as 2-(trifluoromethyl)benzimidazole derivatives FA-S.Citation61 They further developed 30 derivatives based on this scaffold, with FA16, containing the 4-SO2NMe2 group, demonstrating the highest lethality. By inhibiting the Xc− transporter, FA16 effectively triggers ferroptosis in various human cancer cell lines.Citation61 The IC50 value of FA16 in HT1080 cells was measured at 1.26 μM. Moreover, in the HepG2 xenograft model, FA16 displayed significant inhibition of tumor growth. Both in vivo and in vitro studies have confirmed the favorable safety profile of FA16.Citation61
Lepadins, natural alkaloids derived from the marine didemnum family, exhibit diverse biological activities including anti-cancer and anti-malarial properties.Citation145 Wenjun Wang et al introduced a novel synthetic approach to get seven compounds within the Lepadins series. Notably, lepadins E and H demonstrated notable cytotoxicity in cellular assays, enhancing p53 expression, elevating ROS and lipid peroxidation levels, reducing SLC7A11 and GPX4 expression, up-regulating ACSL4, and triggering ferroptosis through the classical p53-SLC7A11-GPX4 pathway.Citation62 In animal studies, Lepadin H effectively suppressed melanoma growth. Nevertheless, Lepadins E and H exhibited only modest selectivity towards cancer cells over normal cells, necessitating further investigation into their safety profile.Citation62
Solasonine (SS) is a natural alkaloid-like compound extracted from potato tubers with anti-cancer and anti-arthritic effects.Citation146 Research has demonstrated that SS exhibits toxicity towards various cancer cell lines, including hepatocellular carcinoma,Citation147 lung cancer,Citation148 and gastric cancer cells.Citation149 The induction of ferroptosis in lung adenocarcinoma by SS is linked to redox imbalance and mitochondrial dysfunction.Citation150 In pancreatic cancer cells, SS triggers ferroptosis through the TFAP2A/OTUB1 SLC7A11 axis,Citation71 while in liver cancer cells, it disrupts the GSH-GPX4 redox system to induce ferroptosis.Citation151
Curcumin, a polyphenolic compound derived from turmeric rhizomes, possesses chologogic, antimicrobial, and anti-cancer properties.Citation152 Studies have shown that curcumin induces ferroptosis in various tumors such as non-small cell lung cancer,Citation73 breast cancer,Citation74 and glioblastoma,Citation153 each with distinct mechanisms. For instance, curcumin inhibits breast cancer by up-regulating SLC1A5 expression, a crucial glutamine transporter.Citation74 In follicular thyroid carcinoma, curcumin induces ferroptosis by increasing Heme Oxygenase-1 (HO-1) expression.Citation154 The induction of ferroptosis in non-small cell lung cancer and hepatic stellate cells involves the activation of autophagy.Citation155
Sulfasalazine (SAS) is an anti-inflammatory agent commonly used to treat chronic inflammatory diseases.Citation156 It is a potent inhibitor of system Xc− that characteristically inhibits cystine transport mediated by SLC7A11.Citation54 SAS can effectively induce ferroptosis in tumor cells such as Multiple myeloma (MM) cells,Citation157 Esophageal cancer (EC) cellsCitation63 and TNBC cells.Citation158 Sorafenib is a protein kinase inhibitor approved for the treatment of hepatocellular carcinoma and advanced renal cell carcinoma.Citation159 It attenuates hepatic fibrosis and liver injury by inhibiting systemic Xc− induced ferroptosis in hepatic stellate cells.Citation160 Sorafenib treatment was accompanied by a significant increase in Cyclooxygenase-2 (COX2) expression and a decrease in SLC7A11 and GPX4 proteins. Yun-Jeong Kim et al designed and synthesized JB3, a derivative compound of sorafenib, which showed significant tumor growth inhibition in both in vivo and in vitro experiments.Citation128 However, it has also been suggested that sorafenib fails to trigger ferroptosis in a wide range of cancer cell lines.Citation161 Its cytotoxic effects in various cell lines, including HT1080, HEK293T and B16F10 cells, could not be prevented by ferroptosis inhibitors. The ability and mechanism of action of sorafenib as an ferroptosis inducer remains controversial. Marketed drugs such as the PARP inhibitor olaparib,Citation162 the anti-malarial medicine flubendazole,Citation67 and the anti-leukemic drug 6-Thioguanine (6-TG),Citation68 which act on a variety of diseases, have also been found to Anti-tumour effect by inhibiting system Xc−-induced ferroptosis in cells. The classic Antidiabetic Drug metformin has also been found to inhibit breast cancer by inducing ferroptosis through down-regulation of SLC7A11 expression.Citation69
Targeting GSH-GPX4
GPX4 is a central regulator of ferroptosis, and inhibition of GPX4 is an important mechanism of action for ferroptosis inducers.
RAS-selective lethal 3 (RSL3) is a Tetrahydro-β-carboline (THBC) identified by Wan Seok Yang et al in 2008.Citation163 As a well-known inducer of ferroptosis, RSL3 effectively hinders the growth and proliferation of various cancer cells, such as those found in lung, breast, and liver cancers. By directly binding to selenocysteine residues in the GPX4 catalytic site, RSL3 deactivates them, leading to an increase in ROS and cellular LIP levels. This results in the accumulation of intracellular lipid peroxides and triggers ferroptosis.Citation164 It has been observed that while RSL3 alone does not induce ferroptosis in glioblastoma, its combination with NF-κB pathway activation does.Citation75 However, the clinical use of RSL3 is hindered by side effects and drug resistance, necessitating further research and improvement for its application.Citation165
Congjun Xu et al synthesized a series of novel and efficient GPX4 inhibitors using RSL3 as lead-compound and scaffold hopping strategy.Citation77 By determining the compounds’ IC50 values and GPX4 inhibition in various cells, compound 26a containing Oxazole Fragment was identified as the most superior GPX4 inhibitor. Compound 26a inhibited GPX4 by 71.7% at 1.0 μM with an IC50 value of 0.15 μM in HT1080 cells.Citation77 It significantly inhibited tumor growth in a 4T1 xenograft model without significant toxicity. 26a ameliorates the selectivity and cytotoxicity problems of conventional chloroacetamide-based GPX4 inhibitors.Citation77
In addition, John T. Randolph et al designed and synthesized ferroptosis inducer using RSL3 as a lead compound 24.Citation78 Compound 24 has similar cell-killing activity (IC50 = 0.117 μM) and higher plasma stability (t1/2 >5 h) in mouse plasma compared to RSL3, but it has limited efficacy as a single-agent tumor-targeting therapy.Citation78
Michel Weïwer et al identified two novel molecular probes ML162 and ML210 by high throughput screening.Citation79 Both ML162 and ML210 are covalent inhibitors of GPX4, inactivating GPX4 selenocysteine residues by covalently binding them. ML162 is similar to RSL3 and also has the chloroacetamido group. However, it is now proposed that RSL3 and ML162 are not direct inhibitors of GPX4 but Thioredoxin reductase 1 (TXNRD1).Citation166 TXNRD1 is another cytoplasmic selenoprotein that regulates intracellular redox and is a newly proposed key regulator and drug target for ferroptosis.Citation167 ML210 is a compound containing nitroisoxazoles. ML210 has an intact cell-dependent inability to interact with purified GPX4 protein. It must be activated in intact cells to produce the critical metabolite JKE-1674 and undergo additional cellular activation and dehydration to generate an electrophile JKE-1777 that binds to GPX4.Citation168 GPX4 inhibitors acting via nitrile oxide exhibited significant selectivity for GPX4 and reduced off-target effect compared to chloroacetamide-based GPX4 inhibitors.Citation168
Conventional chloroacetamide GPX4 inhibitors suffer from off-target effect, poor stability and selectivity, limiting their use in in vivo therapy.Citation169 In order to optimize these inhibitors, Tingting Chen et al performed structural optimization of RSL3 and ML162 based on a molecular docking model that retained the chloroacetone fragment and introduced N-benzylaniline and diphenylamine skeleton and successfully synthesized a series of novel GPX4 covalent inhibitors.Citation80 Among them, compound C18 covalently binds to Sec46 of GPX4 and inhibits the function of GPX4, leading to ferroptosis in TNBC cells. Compound C18 showed a higher inhibition rate, better GPX4 inhibitory activity and in vivo antitumor pharmacokinetic properties compared to RSL3 and ML162. At a concentration of 1 μM, C18 almost completely inhibited the activity of TNBC cell lines and showed high selective inhibition of these cell lines without significant organ toxicity.Citation80
Furong Ma et al used pharmacophore hybridization and bioisosterism strategies to construct a series of ML162-quinone conjugates.Citation129 Among them, GIC-20 was identified as the most active compound. The IC50 of GIC-20 in HT-1080 cells is 1.6 μM. GIC-20 not only induces ferroptosis through proteasome-mediated GPX4 degradation but also induces apoptosis by up-regulating the level of the apoptotic protein Bax and down-regulating the level of the antiapoptotic protein Bcl −2.Citation129 Meanwhile, GIC-20 showed no significant toxicity compared with ML162.
Ferroptosis inducers targeting GPX4, also identified by screening, include FIN56,Citation81 N6F11Citation170 and Metamizole sodium.Citation83 FIN56 not only promotes GPX4 degradation via Acetyl-CoA carboxylases (ACCs), but also degrades GPX4 by binding to and activating squalene synthase, an enzyme involved in cholesterol synthesis, thereby inducing ferroptosis.Citation171 FIN56 can effectively exert anti-tumour effects. N6F11 binds to a specific structural domain of the ubiquitin E3 ligase TRIM25, predominantly expressed in tumor cells, triggering ubiquitination and degradation of GPX4.Citation82 Thus, in contrast to conventional GPX4 inhibitors, N6F11 can selectively induce GPX4 protein degradation in tumor cells without causing damage to immune cells.
Unlike traditional GPX4 inhibitors targeting the active site selenocysteine, Hengrui Liu et al identified an unexpected variant site C66 on GPX4. They screened the lead compound LOC1886, which covalently binds this allosteric site to inhibit GPX4.Citation84 However, LOC1886 showed lower efficacy than RSL3 and ML162 in inducing cellular ferroptosis.
CH004 is a biologically active inhibitor of cystathionine β-synthase (CBS) with in vitro and in vivo effects designed and synthesized by Li Wang et al.Citation85 CH004 dose-dependently inhibits CBS activity in cells or in vivo, inhibiting cysteine and hydrogen sulfide production. CH004 can effectively inhibit tumor growth in vivo and in vitro with an IC50 value of 1 μM.Citation85 A compound that also indirectly inhibits GPX4 is Buthionine sulfoximine (BSO). BSO is a fast-acting and irreversible inhibitor of glutamate cysteine ligase (GCL), which inhibits glutathione synthesis, indirectly inactivates GPX4, induces lipid peroxidation, and thus inhibits cell viability, and has been shown to have an inhibitory effect on breast cancer, melanoma, and Others.Citation172 However, its therapeutic effect as an ferroptosis inducer is only seen in tumors with low levels of GSH expression. Simvastatin inhibits hepatic stellate cell (HSC) activation and induces ferroptosis in HSC by inhibiting the MVA pathway to downregulate GPX4 expression.Citation87
Orlistat is an anti-obesity drug approved by the US Food and Drug Administration (FDA), and fatty acid synthase (FASN) is the main lipomatoses it inhibits.Citation88 Studies have shown that Orlistat induces ferroptosis-like cell death of lung cancer cells.Citation89 Orlistat reduces GPX4 expression, upregulates phosphorylated MAPK/ERK, and induces lipid peroxidation in lung cancer cells. Orlistat also promotes lipid peroxidation and ferroptosis in pancreatic neuroendocrine tumors (pNETs) cells by inhibiting the expression of xCT and GPX4 and increasing the expression of CD71.Citation173
Tubastatin A, an inhibitor of Histone deacetylase-6 (HDAC6), significantly induces ferroptosis in ferroptosis-sensitive cell lines, and this process was not dependent on its inhibition of HDAC6.Citation174 Radiotherapy not only induces ferroptosis by promoting ROS generation and ACSL4 expression in cancer cells, but also increases ferroptosis-resistance and radio-resistance of cancer cells by activating Nrf2-mediated GPX4 transcription and inhibiting lysosome-mediated GPX4 degradation.Citation90 Tubastatin A can overcome ferroptosis-resistance and radio-resistance in cancer cells by directly binding to GPX4 and inhibiting GPX4 enzyme activity.Citation174 In the xenograft model, it significantly promotes radiotherapy-induced lipid peroxidation and effectively improves tumor suppression. However, Tubastatin A is a hydroxamic acid and may cause some side effects in subsequent clinical trials.Citation174 Therefore, optimization of the structure of Tubastatin A is essential.
GliotoxinCitation91 and Timosaponin AIIICitation92 are two natural products that induce ferroptosis by targeting GPX4. In addition to targeting GPX4, Gliotoxin was found to act on the protein SLC7A11 in H1975 cells.Citation91 Timosaponin AIII promotes ferroptosis in NSCLC by targeting and promoting heat shock protein 90 (HSP90)-mediated GPX4 ubiquitination and degradation.Citation92 Compound 31 is an indirubin derivative synthesized by Jiang-Min Zhu et al by combining the core of the natural product indirubin with the active fragment of a GPX4 covalent inhibitor via a linker.Citation93 The IC50 of compound 31 in HCT-116 cells was 0.49 μM. It significantly reduced GPX4 levels in HCT-116 cells by promoting GPX4 ubiquitination.
Sulforaphane (SFN) is a naturally occurring isothiocyanate found in various vegetables that has anti-cancer and anti-inflammatory properties.Citation175 Recently, Giulia Greco et al showed that in leukemia cells SFN triggers different patterns of cell death in a dose-dependent manner.Citation94 At 25 µM, SFN induced caspase-dependent apoptosis, and at 50 µM, ferroptosis was induced through GSH depletion, decreased GPX4 expression and lipid peroxidation. The anti-leukemic activity of SFN can be mediated by inducing both ferroptosis and apoptosis.Citation94
Targeting FSP1
The FSP1-CoQ10-NAD(P)H pathway and the xCT-GSH-GPX4 pathway are two parallel lipid antioxidant pathways.Citation176 The two pathways act synergistically to inhibit lipid peroxidation and ferroptosis. Inhibition of FSP1 would effectively inhibit the antioxidant effects of the FSP1-CoQ10-NAD(P)H pathway, thereby inducing the onset of ferroptosis.Citation177
Sebastian Doll et al conducted a screening of around 10,000 compounds, resulting in the discovery of a potent FSP1 inhibitor named iFSP1, which is the first targeted FSP1 inhibitor described in the literature.Citation178 iFSP1 induces ferroptosis to inhibit cancer cell growth in vitro by inhibiting GPX4. The IC50 value of iFSP1 is 103nM. iFSP1 is specific for hFSP1, and residue F360 within hFSP1 is closely related to the binding of iFSP1 and its target. iFSP1 can inhibit the growth of hepatocellular carcinoma (HCC) by inducing ferroptosis and potential immunomodulatory effects.Citation95 Up-regulated chemokines after iFSP1 treatment promote infiltration of antigen-presenting cells, macrophages and cytotoxic T cells into the tumor.
Toshitaka Nakamura et al predicted the physicochemical properties and drug similarities of about 10,000 small molecule drugs and finally identified 3-phenylquinazolinones as a class of potent inhibitors of FSP1.Citation96 Of these, icFSP1 is the most representative. icFSP1 is a specific inhibitor of hFSP1, which triggers the Phase separation of FSP1 by inducing the formation of FSP1 condensate in tumor cells to induce ferroptosis in cancer cells.Citation96 In contrast to iFSP1, icFSP1 did not show off-target effects even at higher concentrations.
Joseph M. Hendricks et al identified several structurally distinct FSP1 inhibitors through chemical screens. Among them, FSEN1, a non-competitive inhibitor of FSP1, showed the highest potency in inducing cell death in NCI-H460 GPX4KO cells (EC50 = 69.363 nM).Citation97 However, in most cells, FSEN1 could not induce ferroptosis by inhibiting FSP1 alone. In vitro experiments showed that FSEN1 synergizes with dihydroartemisinin, an endoperoxide-containing ferroptosis inducer, to induce ferroptosis in tumor cells.Citation97
iFSP1, icFSP1, and FSEN1 specifically target hFSP1 and fail to be tested in vivo in tumor transplantation models. Whether they inhibit FSP1 in vivo and whether they have an effect on tumor growth remains to be investigated. Toshitaka Nakamura et al identified the first non-species-dependent FSP1 inhibitor, versatile inhibitor of FSP1 (viFSP1), which targets the NAD(P)H binding pocket.Citation98 Treatment with viFSP1 in Pfa1 cells expressing mouse FSP1 or hFSP1 led to significant lipid peroxidation and ferroptosis.
Compound NPD4928, screened from a chemical library by Hiromasa Yoshioka et al, also targets binding to FSP1 and inhibits its activity.Citation99 The combination of NPD4928 and GPX4 inhibitors induced ferroptosis; however, using FSP1 alone did not induce ferroptosis and was not sensitive in some cells. In addition to these drugs, curcuminCitation179 and sorafenibCitation180 have been found to induce ferroptosis in cells via the FSP1 pathway.
The study reveals that most FSP1 inhibitors alone are unable to induce ferroptosis in cells, or can only do so in cells with low GPX4 expression.Citation181 Instead, these inhibitors primarily act as enhancers of ferroptosis and are often combined with other ferroptosis inducers to enhance their effects.Citation182
Targeting Nrf2
Nrf2 is involved in the regulation of oxidative homeostasis in vivo by acting on downstream target genes.Citation183 Inhibition of Nrf2, and thus its antioxidant effects, is also an important pathway of action for ferroptosis inducers.Citation184
S-3’-hydroxy-7’, 2’, 4’-trimethoxyisoxane (ShtIX) is a novel isoflavone compound derived from the heartwood of Dalbergia latifolia.Citation100 ShtIX has demonstrated broad-spectrum anticancer activity, with particularly high cytotoxicity against NSCLC cells. Research has shown that ShtIX induces ferroptosis in NSCLC cells by targeting the Nrf2/HO-1 signaling pathway, with an IC50 of 7.9 µM in A549 cells. Importantly, ShtIX selectively eliminates non-small cell lung cancer cells.Citation100
Trigonelline, the primary alkaloidal component of the legume herbaceous Trigonella foenum-graecum, exhibits anti-HSV-1, antibacterial, and antifungal activities.Citation185 Acting as an Nrf2 inhibitor, trigonelline reduces cellular resistance to ferroptosis and shows potential for combination therapy with other ferroptosis inducers.Citation101 In xenograft models, trigonelline enhances the anticancer effects of erastin, sorafenib, and RSL3, and increases the chemosensitivity of Etoposide in pancreatic cancer.Citation186
Baicalin, a flavonoid extracted from the dried root of Lamiaceae family plants Scutellaria baicalensis Georgi, possesses antibacterial, antiallergic, antitumor, and other pharmacological properties.Citation187 Research indicates that baicalin can induce ferroptosis in bladder cancer cells by activating Ferritin Heavy Chain 1 (FTH1).Citation104 In osteosarcoma cells, baicalein interacts with Nrf2, affecting their stability by promoting ubiquitination and degradation, leading to the inhibition of GPX4 and xCT expression, ultimately inducing ferroptosis in osteosarcoma cells.Citation188
Matrine, an alkaloid derived from the roots of Sophora flavescentis Radix (Kushen), exhibits notable antitumor properties.Citation105 Research has demonstrated that matrine can trigger ferroptosis in various types of tumor cells, including cervical cancer cells,Citation189 rectal cancer cells,Citation190 and hepatocytes.Citation191 The specific mechanisms through which matrine induces ferroptosis in different cell types vary. For instance, in cervical cancer, matrine hinders cervical cancer progression by activating the Piezo1-Ca2+ signaling pathway to induce ferroptosis.Citation189 Additionally, matrine induces ferroptosis in hepatocytes by suppressing the Nrf2/GPX4 antioxidant system.Citation191
Acetaminophen (APAP) is a commonly used antipyretic and analgesic medication that can lead to liver damage in a dose-dependent manner when taken in excess, potentially causing acute liver failure in severe cases.Citation192 Research indicates that the primary mechanism underlying APAP-induced liver damage is ferroptosis, although the exact process by which it triggers ferroptosis in liver cells remains unclear.Citation193 Studies have revealed that NSCLC cell lines exhibit reduced sensitivity to erastin-induced ferroptosis, but APAP can enhance this sensitivity by modulating the Nrf2/HO-1 signaling pathway.Citation102 Similarly, Sunitinib (SUN), a multi-targeted tyrosine kinase inhibitor known for its anti-angiogenic and anti-tumor properties, is associated with cardiotoxic effects that limit its clinical utility.Citation103 It has been found that Nrf2-dependent ferroptosis plays a critical role in SUN-induced cardiotoxicity.Citation194
Targeting Lipid Metabolism
Lipid peroxidation is central to the development of ferroptosis, and regulation of lipid metabolism is also a critical action pathway for ferroptosis inducers.
Shuai Hua et al developed a series of quinazolinediones (QD)-based ROS inducers and screened the optimal compound, QD325, after testing it in pancreatic cancer preclinical models.Citation114 They synthesized a set of QD derivatives by making structural modifications to QD325. QD394 was the optimal compound. The transcriptome profile of QD394 closely resembles that of napabucasin, a cancer stem cell inhibitor. Both small molecules inhibited STAT3 phosphorylation, increased cellular ROS, and decreased the GSH/GSSG ratio.Citation114 The IC50 value of QD394 in PANC-1 cells was 0.34 μM. It was optimized to give the derivative QD394-Me, which showed better plasma stability and reduced toxicity in mice compared to QD394. The IC50 value of QD394-Me in PANC-1 cells was 0.38 μM. QD394-Me, as ROS-inducing drugs, exhibited potent antitumor effects on human pancreatic cancer cells.Citation114
Imetelstat, a telomerase inhibitor, competitively inhibits telomerase activity by targeting the telomerase RNA template and is primarily used in the clinical treatment of myelofibrosis and myelodysplastic syndromes.Citation115 Claudia Bruedigam et al discovered that imetelstat, a potent inducer of ferroptosis, effectively reduced the burden of acute myeloid leukemia (AML) and delayed relapse after oxidative stress-induced therapy.Citation195 Imetelstat increases the synthesis and subsequent oxidation of PUFA phospholipids, and its induced lipophagy triggers ferroptosis in AML. Imetelstat causes cell death by involving two regulators of fatty acid metabolism (FADS2 and ACSL4), leading to excessive lipid reactive oxygen species formation and ferroptosis.Citation196
Tingting Liang et al designed and synthesized a series of novel urea derivatives, among which the compound 10p exhibited the most effective antiproliferative activity against HT-29 cells.Citation127 10p could induce both ferroptosis and autophagy in cells. It was shown that 10p-induced cell cycle arrest, ferroptosis, and autophagy may be related to the accumulation of ROS. 10p is a promising lead compound that can be used for further optimization.
Disulfiram (DSF), a specific inhibitor of aldehyde dehydrogenase type I (ALDH1), is commonly used in the treatment of alcoholism due to its acute sensitization to alcohol.Citation197 DSF has been found to possess antitumor properties in various tumor types, with induction of ferroptosis being one of its mechanisms.Citation197 DSF triggers lysosomal membrane permeabilization in a ROS-dependent manner, a key mechanism for inducing cell death.Citation116 Additionally, DSF enhances cell sensitivity to chemotherapy and radiotherapy.Citation198 Furthermore, DSF exhibits copper-dependent anticancer activity, and when combined with copper, its ability to induce tumor cell death is significantly enhanced. Studies have shown that DSF/Cu effectively induces ferroptosis in TNBC cells.Citation199
Quercetin is a flavonoid that has shown anticancer activity in various cancers, including lung, ovarian, and prostate cancers.Citation200 Induction of ferroptosis is one of its pathways of action against cancer. Quercetin increases ROS and transferrin receptor production, decreases mitochondrial membrane potential, GPX4 and SLC7A11 levels, and inhibits NF-κB and Nrf2.Citation117
Darapladib is an orally active, selective and reversible inhibitor of phospholipase A2s (PLA2s).Citation201 Lp-PLA2 is located in the cell membrane and cytoplasm, controls intracellular phospholipid metabolism and contributes to ferroptosis resistance.Citation118 In the xenograft model, darapladib, combined with the GPX4 inhibitor PACMA31, sensitized cells to ferroptosis and showed antitumor activity that inhibited tumor growth.Citation118
Auranofin (AUR), a therapeutic agent for rheumatoid arthritis, is a novel modulator of hepcidin expression.Citation119 AUR can reduce iron overload by upregulating Hepcidin expression through activation of the NF-κB/IL-6/JAK-STAT signaling pathway, suggesting that AUR is a potential new drug for the treatment of hereditary hemochromatosis and iron overload-associated diseases triggered by hepcidin deficiency. However, when given at high doses (25 mg/kg bw), AUR triggered hepatic ferroptosis and 100% mortality by inhibiting TXNRD activity and increasing lipid peroxidation.Citation119
Targeting Iron Metabolism
Abnormal iron metabolism plays a significant role in ferroptosis, with iron metabolic pathways being a key target for regulation of this process.
Hinokitiol, a natural compound derived from tropolone, acts as an iron chelator with various beneficial properties such as anti-inflammatory, antioxidant, antibacterial, and antifungal effects.Citation202 Research by Hongting Zhao et al revealed that the Hinokitiol-iron complex Fe(hino)3, formed by combining hinokitiol and iron in a 3:1 ratio, serves as an inducer of ferroptosis.Citation120 This redox-active complex triggers lipid peroxidation and decreases GSH-GPX4 production through the generation of ·OH via the Fenton reaction upon cellular entry, ultimately leading to ferroptosis induction in TNBC. Notably, serum biochemical indices of Fe(hino)3-treated tumor-bearing mice remained within normal ranges, suggesting a favorable safety profile of Fe(hino)3 in vivo.Citation120
Steroidal saponin SSPH I, extracted from plantain herb, has been identified as an anti-hepatocellular carcinoma compound.Citation203 Research has demonstrated that SSPH I exhibits significant antiproliferative and antimigratory effects on HepG2 cells. In these cells, SSPH I upregulates the expression of SLC7A5, a negative regulator of ferroptosis, while also increasing the expression of TFR and FPN proteins, which are positive feedback factors of ferroptosis. This leads to the accumulation of Fe2+ and subsequent iron overload, ultimately inducing ferroptosis.Citation121 Additionally, SSPH I induces a notable G2/M blockade in HepG2 cells.Citation121
Polyphyllin B (PB) is a dioscin isolated from Paris formosana with antitumor activity and immunomodulatory effects.Citation122 Can Hu et al found that PB could effectively inhibit the proliferation of GC cells by inducing ferroptosis and apoptosis, and could prevent the migration, invasion and cell cycle of tumor cells in vitro. PB can regulate iron metabolism by promoting iron ion transport and ferritin autophagy, directly binding to GPX4 and reducing GPX4 expression to ultimately induce ferroptosis.Citation122 Its anti-GC function may also be related to its regulation of the MAPK signaling pathway, PI3K-AKT signaling pathway, and TNF signaling pathway.
Ochratoxin A (OTA) is one of the fungal toxins, a toxic metabolite produced by toxin-producing strains of Aspergillus ochraceus and penicillium, which is severely nephrotoxic.Citation204 It has been shown that ferroptosis is a potential mechanism by which OTA causes renal injury OTA disrupts renal cell iron homeostasis and induces ferroptosis by up-regulating the expression levels of the iron input genes TFR1 and FTH, down-regulating the expression level of FPN, and significantly increasing its negative regulator hepcidin.Citation123
Juglone is a natural product discovered from Juglans regia that has a variety of pharmacological properties, including antibacterial, antitumor, antiviral, and anti-inflammatory properties.Citation205 Studies have shown that induction of ferroptosis is one of the mechanisms by which juglone is anti-tumor. The IC50 values of juglone in A549 cells and endometrial cancer cells were 18.5 µM and 20.81 µM. The mechanism of juglone-induced ferroptosis in A549 cells mainly involves an increase in iron accumulation and a decrease in GSH and MDA.Citation125 In endometrial cancer, juglone activates HMOX1, which induces ferroptosis. Similar to juglone, strigolactones Bufotalin (BT) isolated from Venenum Bufonis induced ferroptosis in A549 non-small cell lung cancer cells by accelerating the degradation of GPX4 and increasing the intracellular Fe2+ level.Citation206 The IC50 value for BT in A549 cells was 4.21 μM.
Withaferin A (WA) is a natural product isolated from withania that targets vimentin and has anti-inflammatory and anti-tumor properties.Citation207 By inducing ferroptosis, WA inhibited cell viability, invasive ability, vasculogenic mimicry (VM) formation, and VE-cadherin promoter levels in HepG2 and SNU449 cells, thereby attenuating hepatocellular carcinoma metastatic potential and sorafenib resistance.Citation126 WA has now been found to have multiple pathways for inducing ferroptosis. On the one hand, WA reduces the protein level and activity of GPX4. On the other hand, WA induces a novel atypical ferroptosis pathway by directly targeting Kelch-like ECH-Associated Protein 1 (Keap1), which increases unstable Fe2+ upon HMOX1 overactivation.Citation126 WA also elevates Keap1 expression, attenuating Nrf2 signaling activation-mediated Epithelial-Mesenchymal Transition (EMT) and xCT expression.
The environmental pollutant benzo-a-pyrene (BaP) is a carcinogenic Polycyclic aromatic hydrocarbon, and BPDE (benzo(a)pyrene-7,8-dihydrodiol-9,10-epoxide) is the end product of the breakdown of BAP in the human body.Citation124 BPDE is electrophilic and can covalently bind to the exocyclic amine terminus of guanine (G), a nucleophilic site of DNA, to form a BPDE-DNA complex. This complex causes DNA base mutations, thereby activating oncogenes and leading to malignant transformation of cells, ultimately resulting in tumorigenesis.Citation124 Studies have demonstrated that BPDE induces ferroptosis in human trophoblast cells by upregulating iron metabolism, promoting GPX4 proteasome degradation, and increasing intracellular free Fe2+ and excess ROS, leading to lipid peroxidation.Citation124
Dihydroartemisinin (DHA) in combination with cisplatin synergistically induces ferroptosis in pancreatic ductal adenocarcinoma cells by modulating iron metabolism.Citation208 The combination of DHA and DDP significantly increased the expression of TFR and the accumulation of unstable free iron in pancreatic ductal carcinoma cells, impairing mitochondrial homeostasis. Co-treatment of DHA with DDP also synergistically reduced GPX4 expression. Additionally, the combined treatment of lapatinib (LAP) and doxorubicin (Dox) resulted in increased Fe2+ content, suppression of GPX4 expression, and elevation of ASCL4 levels in cardiomyocytes.Citation209 These synergistic effects of LAP lead to pro-apoptotic, pro-mitochondrial dysfunction, pro-oxidative, and ferroptosis effects, resulting in cytotoxicity. Finally, the combination of lapatinib and the lysosomal pro-solubility drug siramesine induces ferroptosis by decreasing ferritin expression and increasing transferrin expression, ultimately leading to increased iron and ROS levels.Citation111
Others
While research on ferroptosis and related drugs is progressing, there are still some ferroptosis inducers with unclear mechanisms of induction. For instance, the natural product ginkgolic acid (GA) inhibits SUMO1-activating enzyme subunit 1 (SAE1), a potential anti-fibrotic target protein.Citation210 In both in vivo and in vitro experiments, GA was found to induce hepatic stellate cell ferroptosis by targeting SAE1, leading to an anti-hepatic fibrosis effect in a rat model of hepatic fibrosis.Citation210 However, the relationship between SAE1 and ferroptosis remains unclear.Citation210 On the other hand, small molecules EC330 and EC359 target the Transmembrane Receptor (LIFR) of the Leukemia inhibitory factor (LIF).Citation211 By binding to LIFR at specific sites within the ligand-receptor complex, EC330/EC359 inhibit LIFR-induced activation of the STAT3 and AKT pathways, ultimately leading to cell death. Treatment with EC330/EC359 results in GPX4 inactivation and GSH depletion, potentially preventing cells from eliminating toxic lipid peroxides and triggering ferroptosis.Citation212 However, the specific targets of EC330/EC359-induced ferroptosis require further investigation.
Statins are widely used, but atropostatin(Atv) administration leads to oxidative stress and cellular damage.Citation213 Indicators of ferroptosis, such as iron overload, ROS accumulation, and lipid peroxidation, were observed in Atv-treated muscle cells, especially in the mitochondria of the cells.Citation214 One study reported that simvastatin causes ferroptosis in cancer cells by inhibiting HMGCR expression.Citation215 However, it has been shown that Atv can attenuate myocardial dysfunction and remodeling after isoproterenol stimulation through inhibition of ferroptosis, as well as attenuate de-ironing-dependent myocardial injury and inflammation after coronary microembolization through the Hif1a/Ptgs2 pathway.Citation216 The specific role and mechanisms of Atv in ferroptosis continue to be studied.
JQ1, a small molecule inhibitor targeting the BET family protein BRD4, has been shown to inhibit the proliferation of cancer cells.Citation217 Studies have shown that ferroptosis is one of the mechanisms by which JQ1 exerts its anticancer effects in vivo. JQ1 enhances ferroptosis by increasing ferritin autophagy or suppressing BRD4 regulation of ferroptosis-related genes.Citation218 Expression of GPX4, SLC7A11, and SLC3A2 was downregulated under JQ1 treatment and BRD4 knockdown. However, the specific mechanism by which BRD4 regulates the expression of GPX4, SLC7A11 and SLC3A2 is unknown. JQ1 can induce ferroptosis in senescent cells by decreasing the expression of anti-ferroptosis genes in senescent cells,Citation219 thereby selectively eliminating senescent cells. However, JQ1-induced ferroptosis occurred in both cancer and normal cells, and therefore, its safety for therapeutic application as an ferroptosis inducer requires further consideration.
Artesunate is a water-soluble hemisuccinate derivative of artemisinin used to treat malaria, improve inflammation, protect nerves, and treat tumors.Citation220 In 2015, Edna Ooko et al noted that artemisinin and its derivatives induce ferroptosis in tumor cells.Citation221 Co-action of artesunate and sorafenib induces ferroptosis in hepatocellular carcinoma cells; the former increases lysosomal activity and lipid peroxidation, and synergistically with the latter induces histone B/L activation and ferritin degradation.Citation113 In addition, artesunate reduced GSH levels in head and neck cancer cells and ovarian cancer cells.Citation222 However, it has also been shown that artesunate may activate the NRF2 antioxidant response element pathway, leading to resistance to ferroptosis.Citation223
Proteolysis-Targeting Chimeras
Proteolysis-targeting chimeras (PROTACs) is a drug development technology that leverages the Ubiquitin-Proteasome System (UPS) to degrade target proteins.Citation224 A typical PROTACs consists of three key components: E3 ubiquitin ligase ligands, target protein-ligand, and a specially designed “linker” structure that connects the two bioactive ligands.Citation225 The application of PROTACs technology in modifying traditional ferroptosis drugs to enhance their targeting and antitumor properties represents a promising avenue for developing ferroptosis inducers ().
Figure 3 (a) Chemical structural formula of PROTAC-like ferroptosis inducers. (b) The process of PROTAC-mediated ubiquitination and proteasomal degradation of GPX4.
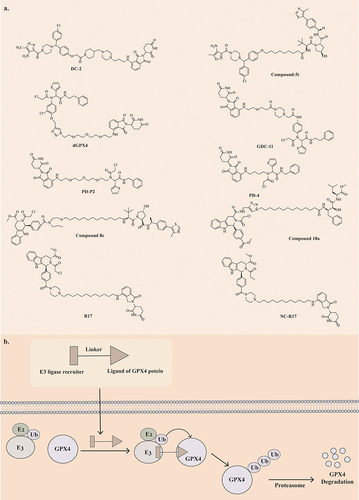
Han Wang et al synthesized a series of GPX4 PROTACs utilizing ML210 as the binding ligand for GPX4, CRBN as the E3 ligase-binding ligand, and various lengths of piperazine rings as linkers.Citation226 Among these, DC-2 exhibited the most potent degradation activity in HT1080 cells, with a DC50 value of 0.03 μM and a significant inhibitory effect on HT1080 cells, with an IC50 value of 0.1 μM. DC-2 not only triggers GPX4 degradation through the ubiquitin-proteasome pathway and the autophagy-lysosomal pathway but also induces the formation of GPX4 mitochondrial isoforms.Citation226 DC-2 outperformed ML210 by degrading GPX4 22-fold more and demonstrated equivalent or superior antiproliferative activity against HT1080, Calu-1, and A375 cells compared to ML210. Additionally, DC-2 exhibited better tumor cell selectivity and safety. Compound 5i, a PROTAC synthesized using ML210 as the GPX4 ligand and VHL as the E3 ligase ligand, degraded GPX4 through the ubiquitin-proteasome and autophagy-lysosome pathways. Additionally, 5i was found to induce ROS accumulation in HT1080 cells.Citation227
Tianli Luo et al designed a PROTAC dGPX4 targeting GPX4 by covalently coupling the GPX4 inhibitor ML162 with pomalidomide.Citation228 This compound effectively reduces tumor cell GPX4 levels through proteasome degradation, leading to ferroptosis. Compared to ML162, dGPX4 was 5 times more efficient in inducing ferroptosis in HT1080 cells, with an IC50 of 300 nM and a DC50 of 200 nM. The nanoparticle formulation, dGPX4@401-TK-12, enhanced biocompatibility and cell selectivity, effectively inhibiting tumor growth without observable side effects.Citation228 Maohua Cai et al also designed GPX4 PROTACs based upon ML162 and its derivatives, with CRBN-based PROTAC GDC-11 demonstrating balanced GPX4 degradation (33% at 10 μM), cytotoxicity (IC50 = 11.69 μM), and lipid peroxide accumulation. This compound exhibited typical ferroptosis characteristics.Citation229 Also based on ML162 are the PROTACs compounds PD-4 and PD-P2. They use pomalidomide as an E3 ligase ligand to promote efficient degradation of GPX4 via the ubiquitin-proteasome pathway.Citation230
Chao Wang et al synthesized six MSL3-based PROTACs, identifying compound 8e as a potent GPX4 degrader.Citation231 Compound 8e significantly inhibited proliferation in HT1080 (IC50 = 58 nM) and Calu-1 (IC50 = 25 nM) cells through GPX4 degradation via the ubiquitin-proteasome system and autophagy lysosomal pathway, with a preference for tumor cells carrying oncogenic Ras genes for ferroptosis induction.
Haoze Song et al designed 36 PROTACs by covalently coupling RSL3 to four different ubiquitin ligase ligands. Among them, compound 18a, based on cIAP-E3 ligase ligands, showed the most potent inhibitory effect on GPX4 levels (DC50, 48 h=1.68 μM) and HT1080 cell proliferation (IC50 = 2.37 μM).Citation232 Compound 18a induces cell ferroptosis by forming a ternary complex with cIAP and GPX4 to induce GPX4 degradation. The antiproliferative effect on HT1080 cells was enhanced when compound 18a was combined with FeCl2.Citation232
PROTACs also constructed based on RSL3 are compound R17 and compound NC-R17. R17 is composed of carbon chain linker and lenalidomide E3 ligand. Compound NC-R17 is a non-covalent GPX4 degrader constructed by optimising the POI ligand of R17 and replacing propionamide with chloracetylamine. Both compounds showed the ability to degrade GPX4 in tumour cells.Citation233
Compared with traditional small molecule ferroptosis inducers, PROTACs class of ferroptosis inducers have better targeting and anti-tumour effects with lower side effects. Therefore, PROTACs technology is a very promising development direction for ferroptosis inducers.
Nanomaterials
Nanomaterials are increasingly being investigated for their role in inducing ferroptosis due to their biocompatibility, nano effect, and permeabilityCitation234 ().
Table 2 Nanomaterials for Inducing Ferroptosis
Delivery of exogenous iron ions into the body via nanomaterials is a crucial approach for inducing ferroptosis.Citation276
Mingqi Chen et al developed a ferroptosis inducer, D@FMN-M, to trigger ferroptosis in tumor cells and M2-type macrophages, enhancing tumor immunotherapy.Citation235 They incorporated DHA into iron-ion doped hollow mesoporous silica nanoparticles (Fe-HMSNs) and further modified them with Outer Membrane Vesicles (OMVs) to create D@FMN-M. These nanoparticles accumulate at the tumor site and release Fe3+ ions and DHA in response to the slightly acidic tumor microenvironment. The released Fe3+ is then converted to Fe2+ by depleting intratumoral GSH, leading to the production of free radicals through the Fenton reaction and Fe2+-DHA reaction. This accumulation of lipid peroxides ultimately induces ferroptosis in tumor cells.Citation235
Min Mu et al designed and synthesized a nanomaterial DF-NP consisting of epigallocatechin gallate (EGCG) and Fe3+ to deliver both doxorubicin hydrochloride (Dox) and iron ions to the tumor site.Citation236 DOX/Fe3+/EGCG (DF) NPs have strong solubility and long-term storage stability and can efficiently release DOX and Fe3+ by cell internalization under high GSH levels and tumor acidic environment. On the one hand, DOX enters the cell nucleus and binds to DNA, inducing apoptosis in tumor cells. On the other hand, due to its high reducing capacity, EGCG can chemically reduce Fe3+ to Fe2+, which reacts with H2O2 and continuously induces ROS production through the Fenton reaction, thus inducing ferroptosis.Citation236
Iron oxide nanoparticles (IONPs) are a class of nanomaterials composed of iron oxide (Fe3O4 or Fe2O3).Citation277 Intracellular iron is the basis for the occurrence of ferroptosis. With the development of nanotechnology, IONPs have shown excellent therapeutic advantages due to their ability to deliver exogenous iron to activate tumor ferroptosis. IONPs are also one of the most classical nanoenzymes with intrinsic enzyme-like activity. IONPs have a peroxidase-like activity based on the Fenton reaction, which enables lipid peroxidation of unsaturated fatty acids and induces lipid peroxidation and ROS production.Citation278
Xiangrong Tian et al synthesized iron oxide nanoparticles of different sizes (2–100 nm) to study their antitumor effects and toxicity mechanisms.Citation258 Their study found that ultrasmall nanoparticles (<∼5 nm) release Fe2+ faster and have greater cytotoxicity. However, Fe3O4-10 nm NPs showed the best antitumor efficiency due to the balanced abilities in tumor accumulation, intratumoral penetration, and cellular internalization. It showed an optimal antitumor effect in vivo, and its therapeutic effect was correlated with tumor accumulation and intratumor distribution of nanoparticles.Citation258
Qifang Chen et al designed and synthesized a poly(lactic-co-glycolic acid) (PLGA) nanosystem Fe3O4-PLGA-Ce6 encapsulating Fe3O4 and Ce6.Citation237 The Fe3O4-PLGA-Ce6 nanosystem can dissociate in an acidic tumor microenvironment, releasing iron ions and Ce6, followed by the generation of hydroxyl radicals via the Fenton reaction, which induces ferroptosis in tumor cells. At the same time, under laser irradiation, the released Ce6 increases the generation and accumulation of ROS, providing photodynamic therapy.Citation237 The loading of iron ions and Ce6 allowed the nanoparticles to be fluorescence imaging and MRI-guided, respectively. The nanoparticles were injected intravenously into the body with high biocompatibility, permeability, and retention.Citation237
Hanren Chen et al designed and synthesized an iron oxide (Fe3O4) nanoparticle AFN@CM coated with Arsenic trioxide (ATO) encapsulated by HCC cell membrane.Citation259 For the first time, they demonstrated in their study that ATO can effectively induce lipid peroxidation and ferroptosis in HCC. Moreover, the iron oxide nanoparticles AFN@CM synthesized from ATO can target-induce ferroptosis in tumor cells, with excellent anti-cancer effects and almost no side effects. In addition to the synergistic effect of ATO and Fe, the stronger antitumor effect of AFN@CM may also be attributed to the HCC cell membrane wrapping design feature. This feature endows AFN@CM with better biocompatibility and tumor targeting ability.Citation259
Meanwhile, IONPs can also be used in therapeutic approaches such as chemotherapy, phototherapy, gene therapy and immunotherapy.Citation279
Xiaolong Liang et al designed and synthesized a theranostic nanoplatform based on a relatively smaller (<20 nm) iron oxide loaded porphyrin-grafted lipid nanoparticles (Fe3O4@PGL NPs).Citation254 Fe3O4@PGL NPs could generate ROS in the absence of light via the Fenton reaction at relatively high Fe2+ concentrations. At the same time, the presence of PDT further accelerated the Fenton reaction and oxidative stress on macrophages to generate synergistic ROS. Both in vivo and in vitro experiments showed that Fe3O4@PGL NPs could almost completely inhibit tumor growth by combined PDT under fluorescence and magnetic resonance (MR) imaging guidance. Compared with other nanomaterials, Fe3O4@PGL NPs have relatively small size, high PS loading efficiency, negligible dark toxicity and excellent biocompatibility.Citation254
Amir Jalali et al synthesized Fe3O4@Glu-menthol magnetic nanoparticles (MNPs) by encapsulating iron oxide (Fe3O4) nanoparticles with glucose and affixing them with menthol.Citation280 Studies have shown that Fe3O4@Glu-menthol MNPs can induce apoptosis in gastric cancer cells. Moreover, cells treated with Fe3O4@Glu-menthol MNPs showed 1.6-fold higher expression of the oncogene Caspase 8 gene than untreated cells.Citation280
In addition to Fe3O4@PGL NPs and Fe3O4@Glu-menthol MNPs, many other IONPs are associated with conventional tumor treatments such as chemotherapy, radiotherapy and immunotherapy. Zhuangzhuang Zhang et al designed and synthesized an acid-responsive, iron-coordinated polymer nanoparticle (PPA/TF) encapsulating the mitochondria-targeting drug alpha-tocopheryl succinate (α-TOS).Citation260 In tumors’ weakly acidic intracellular environment, PPA/TF promotes pH-responsive drug release, ROS generation, and GSH depletion.PPA/TF effectively inhibits tumor growth by combining ferroptosis induction with Chemodynamic therapy (CDT).Citation260 Hyaluronan (HA)-encapsulated IONP (IONP-HA) can be combined with photothermal therapy to effectively induce ferroptosis in recurrent bladder cancers that have acquired chemoresistance after chemotherapy.Citation261 Phospholipid-modified Pt(IV) prodrug-loaded iron oxide nanoparticle (IONP)-filled micelles (mIONP-PL-Pt(IV)), which can be used in combination with chemotherapy for antimelanoma treatment.Citation262 Qingxin Mu et al designed and synthesized a multifunctional nanoparticle containing an iron oxide core. This NP can deliver chemotherapeutic and immunotherapeutic agents to breast cancer and dendritic cells simultaneously, thus enabling simultaneous chemo-immunotherapy and effective inhibition of TNBC growth and metastasis.Citation281
Another class of ferroptosis inducer nanomaterial combines traditional ferroptosis inducers with nanomaterials to address issues like low solubility, poor biocompatibility, and adverse reactions.Citation282
BSO was encapsulated in a ZIF-8 matrix, followed by the growth of radiation-sensitive gold nanoparticles (NPs) on the surface to create BSO/ZIF-8@Au.Citation238 This material served as a template for the formation of a Metal-phenolic network (MPN), which was further modified with hyaluronic acid (HA) to produce the BSO/ZIF-8@Au@MPN@HA (BZAMH) nanohybrid. Upon exposure to tumor microenvironmental stimuli, the nanohybrids disassembled, with BSO inhibiting γ-GCS to block GSH biosynthesis, Fe2+ triggering a favorable Fenton reaction, and Au NPs enhancing the deposition of X-ray radiation doses within the tumor.Citation238 The inactivation of GPX4 and the local generation of hydroxyl radicals collectively induced potent ferroptosis, enabling intense radiotherapy and effective treatment of TNBC while suppressing TNBC lung metastasis. Furthermore, the inclusion of Fe and Au elements in the nanomedicine provides diagnostic capabilities for MRI and CT imaging, allowing for the tracking of nanomedicine distribution and movement while monitoring the therapeutic efficacy of cancer treatment.Citation238
MTX-LDH@MnO2 was developed by Liu et al through the incorporation of methotrexate (MTX) within layered double hydroxides (LDHs) interlayers and the electrostatic absorption of biomineralization ovalbumin (OVA)-MnO2 onto the LDH surface, resulting in a multifunctional and dual-responsive nanosheet [250]. Within the tumor microenvironment, MTX-LDH@MnO2 exhibited rapid dual-response release of MTX and Mn2+. This nanosheet effectively induced ferroptosis in tumor cells by synergistically enhancing ROS generation and modulating the GPX4/GSH and GCH1/BH4 pathways to facilitate LPO accumulation. Moreover, MTX-LDH@MnO2 triggered the release of damage-associated molecular patterns (DAMPs), promoting immunogenic cell death (ICD) within tumors. This led to dendritic cell (DC) maturation and infiltration of CD4+/CD8+ T-cells, resulting in robust anti-tumor immune responses [250].
Ling-Xiao Zhao et al designed a GFR nanoplatform, GDY-FIN56-RAP (GFR), with the potential for photothermal therapy (PTT) for the treatment of GBM by encapsulating a RAP-polymer and an ferroptosis inducer, FIN56, on graphdiyne (GDY).Citation240 GFR combined with 808 nm near-infrared radiation significantly triggered GPX4 down-regulation-mediated ferroptosis of GBM cells, which inhibited GBM growth and prolonged the lifespan of the GBM in situ xenograft mouse model. Near-infrared radiation facilitated the release of FIN56 from the GFR and directly induced GBM cell death through temperature elevation—directly induced GBM cell death. The GFR nanoplatforms can penetrate the blood-brain barrier and induce an acidic environment-induced in situ release of FIN56.Citation240
Cheng et al successfully developed an injectable hydrogel, Gel@WA-cRGD, that combines the induction of ferroptosis and immunomodulation effects. The hydrogel was created through Ca2+-mediated cross-linking of natural sodium alginate, incorporating crgd-modified redox-responsive Withaferin prodrugs (WA-cRGD) and programmed cell death ligand 1 antibody (aPD-L1) for targeted tumor treatment. Following injection into postoperative trauma cavities, the hydrogels degrade in a microenvironment-responsive manner, releasing β-cyclodextrin (β-CD) and aPD-L1 to the trauma margins. These components are selectively taken up by tumor cells and activated by excess GSH in the cytoplasm, leading to WA-induced ferroptosis and the release of tumor-derived immune substances for recognition and activation of surrounding antigen-presenting cells (APCs). Additionally, aPD-L1 inhibits the PD-1/PD-L1 interaction between tumor and immune cells, reversing the immunosuppressive tumor microenvironment (TME). Gel@WA-cRGD shows promise for postoperative treatment of resected solid tumors to prevent primary tumor recurrence and suppress distal tumors.Citation241
There are many similar drugs that use nanomaterials to encapsulate ferroptosis inducers to enhance their efficacy. The toxicity of IKE was significantly reduced by using biodegradable nanoparticles for its delivery.Citation144 The nanomedicine Fe3O4@PCBMA formed by loading SIM into magnetic nanoparticle-coated zwitterionic polymers induced ferroptosis and improved the therapeutic efficacy of TNBC.Citation242
In addition to the above nanomaterials, there are many other nanomaterials with ferroptosis-inducing effects.
Lei et al developed biocompatible manganese molybdate nanoparticles (MnMoOx NPs) through a high-temperature liquid-phase method and DSPE-PEG5k modification [254]. MnMoOx NPs demonstrate antitumor effects by inducing ferroptosis and enhancing immunogenicity. These nanoparticles deplete GSH in tumors and inhibit GPX4 expression, triggering ferroptosis in tumor cells. Moreover, MnMoOx NPs induce immunogenic cell death in tumor cells, releasing DAMPs, promoting DC maturation, enhancing T cell infiltration, activating anti-tumor immune responses, and reversing the immunosuppressive tumor microenvironment. When combined with immune checkpoint inhibitors (ICIs) like α-PD-L1, MnMoOx NPs further enhance antitumor effects and suppress tumor metastasis.
Despite their many advantages, such as high drug loading capacity, increased water solubility, and improved selectivity, nanomedicines still have many problems at this stage. Excessive use of exogenous metals may cause potentially adverse effects on human health, including acute and chronic damage.Citation283 The enhanced permeability and retention (EPR) effect is influenced by factors such as the nature of the nanomedicine itself, tumor growth, and patient heterogeneity. These factors make drug targeting inefficient and make it difficult to achieve complete drug enrichment in the tumor.Citation284 This leads to an increased likelihood of damage to normal cells. Although a large number of tumor-targeted nanomedicines have been developed at this stage, factors such as tumor complexity and heterogeneity have led to off-target effects, and the expected targeting effect cannot be achieved. Studies have shown that the pharmacokinetics of different nanomedicines vary between species.Citation285 However, at this stage, most of the studies on the behavior of nanoparticles in vivo are derived from animal experimental data, and few of them are relevant enough to predict their safety and efficiency in humans based on the results of animal experiments. There are no detailed findings on the immunogenic responses generated based on nanomedicine carriers and their impact on pharmacokinetic characterization, therapeutic efficacy and potential toxicity. After applying very small IONPs (ESIONPs) to a zebrafish model, Zhaojie Lyu et al found that ESIONPs may be neurotoxic and induce ferroptosis.Citation283 The abnormal accumulation of iron ions may increase the risk of early degenerative neurological diseases. Therefore, the biosafety and selectivity of nanomedicines for therapeutic use remain highly questionable. In addition, due to the difficulty of synthesizing the same nanoparticles quickly, precisely, and reproducibly, large-scale and reproducible synthesis of nanoparticles is even more complicated when it involves multi-step or complex techniques.Citation286 All these make the clinical translation of nanomedicines a great challenge.
Ferroptosis-Inducing Therapy in PDT and SDT
Photodynamic Therapy
Photodynamic Therapy (PDT) is a new approach to treating tumors and pre-cancerous lesions with photosensitizing drugs and laser-activated treatment.Citation287 Irradiating the lesion site with a specific wavelength activates photosensitizing drugs that selectively accumulate in the lesion tissue, causing the tumor to take up molecular oxygen to produce ROS, which leads to tumor destruction. Synthesizing photodegradation-targeting chimeras (PDTACs) by coupling photosensitizers with ferroptosis-inducing drugs is a new idea in the development of ferroptosis inducer (). Protein targeting and photoactivation coupling makes PDTAC highly controllable compared to conventional therapies, enabling precise and effective treatment.Citation288 This therapy has few side effects, is safer, minimally invasive and better tolerated by the elderly, and has broad clinical applications in combination therapy.Citation289
Qiao Hu et al synthesized the photosensitizer ENBS-ML210 by covalently linking the photosensitizer ENBS with the GPX4 inhibitor ML210.Citation290 The hypoxic microenvironment of NSCLC severely inhibits ferroptosis, leading to the severely limited therapeutic efficacy of some ferroptosis inducers.Citation291 However, in vitro experiments showed that ENBS-ML210 effectively induced ferroptosis in H1299 cells under 21% and 2% O2 conditions. ENBS-ML210 could directly target GPX4 in H1299 cells to produce superoxide anion radical (O2·-)under 660 nm light irradiation. Both in vitro and in vivo experiments confirmed its sound anti-tumor effects.Citation290
Sijin Liu et al synthesized two PDTACs, PV1 and PV2, by coupling the photosensitizer verteporfin (VPF) to GPX4-targeting peptides.Citation292 PV1 and PV2 have neither inhibitory nor degradative activity, but they can selectively degrade intracellular GPX4 and generate ROS under red light irradiation, leading to ferroptosis of tumor cells. Dead cells induced by PDTACs exhibit robust immunogenicity in vitro and efficiently trigger anti-tumor immunity in vivo.Citation292 The coupling of protein degradation and photoactivation makes PDTAC highly controllable, which will greatly help further drug development and clinical application studies.
The second-generation photosensitizer talaporfin sodium (TS) is rapidly cleared from the body, requiring a shorter shading period (2 weeks) than the first-generation photosensitizer porfimer sodium (4 weeks), and the antitumor effect penetrates deeper into the submucosa and extends to the muscle layer.Citation293 PDTAC synthesized by TS-PDT induces ferroptosis in tumor cells by generating ROS and inhibiting system Xc−. In vivo, TS-PDT combined with ferroptosis inducers (IKE or sorafenib) reduced tumor volume.Citation293
TCSVP, a mitochondria-targeted porfimer sodium with aggregation-induced emission (AIE) properties, was developed by Shan Wang et al AIE-PS, in comparison to traditional porfimer sodium, maximizes energy conservation from heat dissipation, leading to enhanced reactive oxygen species (ROS) generation and fluorescence emission capabilities.Citation294 Due to the more negative mitochondrial potential of cancer cells relative to normal cells, the positively charged AIEgen can effectively differentiate between cancerous and normal cells. TCSVP specifically targets cancer cell mitochondria, efficiently producing ROS in the presence of light, thereby sensitizing tumor cells to ferroptosis induction by RSL3.Citation294
Xinyang Zhao et al synthesized the Iridium(III) complex Ir1, derived from hydrated Iridium, with adjustable photophysical and chemical properties. By incorporating triphenylphosphine as a mitochondria-targeting ligand and Py-RSL as a ferroptosis-inducing ligand, the complex not only triggers ferroptosis by inhibiting GPX4 and FSP1 but also suppresses the ErbB signaling pathway to enhance tumor suppression.Citation295 The metal coordination in Ir1 confers fluorescent properties, enabling intracellular localization monitoring under visible light excitation (488 nm) for real-time cell tracking, while effectively reducing off-target toxicity of the Py-RSL ligand.Citation295 With a dual inhibitory mechanism, Ir1 exhibits superior anticancer and ferroptosis-inducing capabilities with reduced toxicity and fewer side effects.
Sonodynamic Therapy
Sonodynamic therapy (SDT) is an innovative non-invasive treatment method that uses ultrasound (US) to activate a sensitizer and produce reactive oxygen species (ROS) to destroy cancer cellsCitation296 (). Unlike light-based therapies, US has the advantage of deeper tissue penetration, making SDT particularly effective for treating tumors located deep within the body.
Yidan Lai et al designed and synthesized a platinum(II)-cyanine complex, Pt-Cy, which was shown to generate ROS under both ultrasound (US) and light irradiation in in vitro experiments. Pt-Cy exhibited US-induced cytotoxicity with an IC50 of 6.94 mM under US irradiation, while showing minimal dark-cytotoxicity to 4T1 cells. Pt-Cy also reduced GSH content in cells under US irradiation, leading to the accumulation of lipid peroxides and triggering ferroptosis. In terms of in vivo tumor therapy, Pt-Cy demonstrated superior acoustic kinetic therapeutic effects compared to PDT.Citation297
Liqiang Zhou et al designed a liposomal nanosystem called Lipo-PpIX@Ferumoxytol, which combines the nanosonosensitizer PpIX and the iron supplement ferumoxytol. Ferumoxytol was used to deliver excess iron to tumor cells, leading to ferroptosis and increased susceptibility to SDT-induced apoptosis. Additionally, SDT was found to enhance the sensitivity to ferroptosis by modulating the ferritinophagy process. The dual ferroptosis/apoptosis pathway was triggered by Lipo-PpIX@Ferumoxytol, resulting in the inhibition of tumor growth.Citation298
Jing Yu et al developed ultra-small iron-doped zinc oxide nanoparticles, FZO-NP, and surface-modified FZO nanoparticles to create FZO-ASP, which exhibited good water stability. FZO-ASP was capable of initiating the Fenton reaction and inducing ferroptosis in tumor cells. When combined with ultrasonic energy, FZO-ASP showed enhanced inhibition of cancer cell proliferation and mouse mammary tumor growth, with no significant toxicity observed in treated mice.Citation234
Challenges and Opportunities in the Development of Ferroptosis Inducers
With in-depth research on the mechanisms and targets of ferroptosis, various ferroptosis inducers have been discovered and developed. Although many ferroptosis inducers have been reported, multiple types of ferroptosis inducers have different advantages and disadvantages (). Meanwhile, the clinical application of ferroptosis inducers is still in slow progress. Therefore, the research, development, and clinical translation of ferroptosis inducers still need to be continuously advanced. Improving the drawbacks of the existing inducers and developing ferroptosis inducers that can be better applied to the clinic is the focus of the current research and development of ferroptosis inducers.
Table 3 Comparing the Advantages and Disadvantages of Different Ferroptosis Inducers Methods
Discovery of New Targets for Ferroptosis Inducers
Most of the existing ferroptosis-inducing agents act on classical ferroptosis-regulating targets such as system Xc−, GPX4, FSP1 and Nrf2, which have limited induction and tend to make cells resistant to drugs.Citation309 Therefore, new targets for ferroptosis inducer action still need to be explored.
Xuejun Jiang et al found that membrane-bound O-Acyltransferase Domain Containing 2 (MBOAT2) could re-regulate the lipid composition of cell membranes through phospholipid metabolism by replacing monounsaturated fatty acid (MUFA)-rich phospholipids with polyunsaturated fatty acid (PUFA), thereby inhibiting ferroptosis. (MUFA)-rich phospholipids replace polyunsaturated fatty acid (PUFA), which is easily oxidized and thus inhibits ferroptosis.Citation310 MBOAT2 is a new regulator independent of GPX4 and FSP1. Meanwhile, MBOAT2 is regulated by the androgen receptor (AR), suggesting that AR becomes a new regulatory target for ferroptosis.
Ping Wang et al recently found that 7-Dehydrocholesterol (7-DHC) directly inhibits phospholipid peroxidation by relying on its unsaturated key structural property.Citation311 The enzymes involved in distal cholesterol biosynthesis can affect the ferroptosis sensitivity of cells by regulating the level of 7-DHC.Citation311 The enzymes involved in distal cholesterol biosynthesis can affect the ferroptosis sensitivity of cells by regulating differences in the levels of 7-DHC. Targeted regulation of 7-DHC levels is expected to be a therapeutic strategy for cancer. Zhigang Hu et al found that Apurinic/apyrimidinic endonuclease 1 (APE1) regulates ferroptosis in hepatocellular carcinoma cells by modulating the AKT/GSK3β/NRF2 axis.Citation312 This suggests that APE1 is a novel ferroptosis regulator.
mTOR is a critical factor that regulates cellular protein synthesis, nutrient metabolism, and growth and proliferation.Citation313 Disheveled Egl-10 and pleckstrin domain-containing protein 5 (DEPDC5) is a newly discovered negative regulator upstream of the mTOR pathway in recent years.Citation314 Song li et al found that DEPDC5-deficient CD8+ T cells produced high levels of xanthine oxidase and lipid ROS due to high mTORC1-induced ATF4 expression, leading to spontaneous ferroptosis.Citation315 This suggests that DEPDC5 protects CD8+ T cells from ferroptosis by limiting mTORC1-mediated purine catabolism. Thus, DEPDC5 is also a target for ferroptosis regulation.
Furthermore, many studies have shown that epigenetic modifications and protein post-translational modifications (PTMs) play important roles in developing diseases involving ferroptosis.Citation316 Epigenetic mechanisms such as DNA methylation, histone acetylation and miRNAs may regulate cellular sensitivity to ferroptosis. DNA methylation can affect the expression of iron metabolism genes such as FPN, TFR2, HAMP, HJV, and bone morphogenetic protein 6 (BMP6) by regulating the promoter region of the methylation level.Citation317 Several miRNAs can target genes such as DMT1, FPN, TFR1, TFR2, and Ferritin H and affect the process of iron absorption, transport, storage, and utilization by inhibiting the expression of these key genes of iron metabolism.Citation318 Epigenetic regulation is also a very promising mechanism of action for ferroptosis inducers. The discovery of these new mechanisms and targets provides new ideas and directions for developing ferroptosis inducers.
Drug Potency Enhancement
The limited potency of the action of ferroptosis inducers is an important reason that prevents their use in clinical practice.Citation319 How to make it more effective in killing tumor cells and thus inhibiting tumor growth is an important issue faced during the development of ferroptosis inducers.
Usually, drugs have a single target, but the pathophysiologic processes of most diseases are so complex that therapeutic modalities directed at a single target have limited effect.Citation320 Thus, drugs targeting multiple cell death modes are better suited for clinical use. Existing ferroptosis inducers, such as matrine,Citation321 PBCitation122 and LAP,Citation322 have the dual effect of inducing ferroptosis and apoptosis in cells. Meanwhile, drugs that act on multiple ferroptosis regulatory targets can better induce ferroptosis in tumor cells than on a single ferroptosis regulatory target.
In addition to developing drugs that can act on multiple targets, combining ferroptosis inducers with different mechanisms of action is a very important therapeutic strategy. For example, FSEN1 synergizes with DHA to induce ferroptosis in tumor cells.Citation97 Ferroptosis inducers can also be used in combination with conventional tumor therapies. Ferroptosis inducers such as Tubastatin A,Citation174 BZAMHCitation238 and DSFCitation198 are effective in enhancing the therapeutic effect of radiotherapy on tumors when combined with radiotherapy.Citation323
With the increasing research on ferroptosis, more and more natural products have been found to have ferroptosis-inducing effects.Citation324 Although these natural products themselves have limited effects in inducing ferroptosis in tumor cells, their discovery has provided lead compounds for the development of ferroptosis inducers. For example, compound 31, synthesized by combining the core of the natural product indirubin with the active fragment of a GPX4 covalent inhibitor via a linker, effectively inhibited human colon cancer cells.Citation93
The continuous development of new technologies such as PROTACs, PDTACs and nanomaterials in ferroptosis inducers also contributes to drug potency. PROTC DC-2 with ML210 as ligand degraded GPX4 22-fold more than ML210 and inhibited it better than ML210 in a wide range of cells.Citation226 PROTC dGPX4 with ML162 as ligand-induced ferroptosis 5-fold more efficiently than ML162.Citation228 Developing these new technological fields is a significant direction for the future development of ferroptosis inducers.
Reduction of Toxic Side Effects of Drugs
As tumors are chronic wasting diseases, tumor patients are usually in poor general condition and their body energy is in negative balance.Citation325 The side effects of the drug will cause serious physical burden to tumor patients. This is also a serious obstacle to the clinical application of ferroptosis inducers. Tumor cells are derived from normal tissues and are very similar to normal tissues in terms of internal and external cellular structure and survival conditions (eg, relationship to the immune system).Citation326 For example, ferroptosis induced by Lepadins E, Lepadins H and JQ1 occurs in cancer cells and normal cells. How to selectively kill tumor cells without harming normal cells in therapy is an important issue in the development of ferroptosis inducers.Citation24
In selecting drug targets, the selection of tumor-specific targets can selectively induce ferroptosis in tumor cells.Citation327 For example, N6F11 can bind to a specific structural domain of the E3 ubiquitin ligase TRIM25, predominantly expressed in tumor cells, thereby selectively causing GPX4 protein degradation in tumor cells. Studies have shown that the toxic effects of compounds are related to the structure of the compound.Citation328 For example, nitro is the leading toxic group separating nitroaromatics and can covalently bind to hemoglobin.Citation329 Therefore, in the design stage of compounds, the drug’s toxicity can be reduced from the starting point of the structure-activity relationship.
Technologies such as PROTACs, PDTACs, and nanomaterials have been shown to improve the targeting of ferroptosis inducers.Citation330 PROTAC-based ferroptosis inducers such as DC-2, dGPX4, and compound 8e have been shown to enhance the rate of inhibition while effectively improving the ability of the drugs to target the tumor cells and reducing the toxicity of the drugs. In photodynamic therapy, irradiating the lesion area with specific wavelengths activates the drugs that selectively accumulate in the lesion tissue. This treatment has better precision and controllability.Citation331 Nanomedicines, on the other hand, are more biocompatible and soluble and cause fewer toxic side effects than conventional ferroptosis inducers.Citation332
Summary
Ferroptosis is an iron-dependent mode of programmed cell death. Ferroptosis has become a hot research topic since it was proposed in 2012. With in-depth research on the mechanism of ferroptosis and its targets, various ferroptosis inducers have been discovered and developed. At the same time, research on its application in cancer prevention and treatment has also deepened. Under the predicament that tumors are resistant to traditional therapies such as chemotherapy and radiotherapy and have poor therapeutic effects, the proposal and development of ferroptosis-inducing therapies have provided new ideas and prospects for tumor treatment.
In this article, we introduce the mechanism of ferroptosis, the various types of ferroptosis inducers, and the application of ferroptosis-inducing therapies in the field of PDT and SDT. The comprehensive and systematic summary of small molecule drug classes of ferroptosis inducers provides ideas for designing and optimizing a new generation of small molecule drugs. The summary of the research progress of ferroptosis inducers in PROTACs, nanomaterials, PDT, and SDT provides a reference for the development and clinical translation of ferroptosis inducers in new fields. We also compare the advantages and disadvantages of various ferroptosis inducers. More importantly, we present the challenges and opportunities for developing ferroptosis inducers. We not only show the problems encountered in developing ferroptosis inducers but also propose solutions to solve the problems. In conclusion, we hope that the comprehensive summary and in-depth discussion of ferroptosis inducers will provide a direction for further research and development and clinical translation of ferroptosis inducers, thus bringing more effective treatment for tumor patients.
Author Contributions
YL.L, XY.B, L.Z is responsible for conceptualizing, designing, and writing the content of the article. QQ.H, N.Z, JZ.C, MZ.H is responsible for literature research, interpretation, and data collection. X.L.L is responsible for conceptualizing, funding, and guiding this article. All authors have critically reviewed the knowledge content of the final version of the article and approved the submitted version.
All authors made a significant contribution to the work reported, whether that is in the conception, study design, execution, acquisition of data, analysis and interpretation, or in all these areas; took part in drafting, revising or critically reviewing the article; gave final approval of the version to be published; have agreed on the journal to which the article has been submitted; and agree to be accountable for all aspects of the work.
Disclosure
The authors report no conflicts of interest in this work.
Acknowledgment
YiLin Luo, XinYue Bai and Lei Zhang share first authorship.
Additional information
Funding
References
- Siegel RL, Giaquinto AN, Jemal A. Cancer statistics, 2024. CA Cancer J Clin. 2024;74(1):12–49. doi:10.3322/caac.21820
- Wu Y, Li Y, Gao Y, et al. Immunotherapies of acute myeloid leukemia: rationale, clinical evidence and perspective. Biomed Pharmacother. 2024;171:116132. doi:10.1016/j.biopha.2024.116132
- Murayama T, Nakayama J, Jiang X, et al. Targeting DHX9 triggers tumor-intrinsic interferon response and replication stress in small cell lung cancer. Cancer Discov. 2024;14(3):468–491. doi:10.1158/2159-8290.Cd-23-0486
- Xu B, Sun H, Liu S, et al. IFI35 limits antitumor immunity in triple-negative breast cancer via CCL2 secretion. Oncogene. 2024;43(10):693–702. doi:10.1038/s41388-023-02934-w
- Shishido SN, Lin E, Nissen N, et al. Cancer-related cells and oncosomes in the liquid biopsy of pancreatic cancer patients undergoing surgery. NPJ Precis Oncol. 2024;8(1):36. doi:10.1038/s41698-024-00521-0
- Dixon SJ, Lemberg KM, Lamprecht MR, et al. Ferroptosis: an iron-dependent form of nonapoptotic cell death. Cell. 2012;149(5):1060–1072. doi:10.1016/j.cell.2012.03.042
- Jiang X, Peng Q, Peng M, et al. Cellular metabolism: a key player in cancer ferroptosis. Cancer Commun. 2024;44(2):185–204. doi:10.1002/cac2.12519
- Zhao X, Li X, Xu Y. Ferroptosis: a dual-edged sword in tumour growth. Front Pharmacol. 2023;14:1330910. doi:10.3389/fphar.2023.1330910
- Yang Z, Wei X, Ji C, et al. OGT/HIF-2α axis promotes the progression of clear cell renal cell carcinoma and regulates its sensitivity to ferroptosis. iScience. 2023;26(11):108148. doi:10.1016/j.isci.2023.108148
- Tang R, Wu Z, Rong Z, et al.. Ferroptosis-related lncRNA pairs to predict the clinical outcome and molecular characteristics of pancreatic ductal adenocarcinoma. Brief Bioinform. 2022;23(1). doi:10.1093/bib/bbab388
- Li J, He D, Li S, Xiao J, Zhu Z. Ferroptosis: the emerging player in remodeling triple-negative breast cancer. Front Immunol. 2023;14:1284057. doi:10.3389/fimmu.2023.1284057
- Mojarad-Jabali S, Mahdinloo S, Farshbaf M, et al. Transferrin receptor-mediated liposomal drug delivery: recent trends in targeted therapy of cancer. Expert Opin Drug Deliv. 2022;19(6):685–705. doi:10.1080/17425247.2022.2083106
- Lee J, Roh JL. Epithelial-mesenchymal plasticity: implications for ferroptosis vulnerability and cancer therapy. Crit Rev Oncol Hematol. 2023;185:103964. doi:10.1016/j.critrevonc.2023.103964
- Bae C, Hernández Millares R, Ryu S, et al. Synergistic effect of ferroptosis-inducing nanoparticles and X-ray irradiation combination therapy. Small. 2024;20:e2310873. doi:10.1002/smll.202310873
- Shi TM, Chen XF, Ti H. Ferroptosis-based therapeutic strategies toward precision medicine for cancer. J Med Chem. 2024;67(4):2238–2263. doi:10.1021/acs.jmedchem.3c01749
- Zhang DD. Ironing out the details of ferroptosis. Nat Cell Biol. 2024. doi:10.1038/s41556-024-01361-7
- Hinokuma H, Kanamori Y, Ikeda K, et al. Distinct functions between ferrous and ferric iron in lung cancer cell growth. Cancer Sci. 2023;114(11):4355–4364. doi:10.1111/cas.15949
- Hong X, Roh W, Sullivan RJ, et al. The lipogenic regulator SREBP2 induces transferrin in circulating melanoma cells and suppresses ferroptosis. Cancer Discov. 2021;11(3):678–695. doi:10.1158/2159-8290.Cd-19-1500
- Han Y, Fu L, Kong Y, Jiang C, Huang L, Zhang H. STEAP3 affects ovarian cancer progression by regulating ferroptosis through the p53/SLC7A11 pathway. Mediators Inflamm. 2024;2024:4048527. doi:10.1155/2024/4048527
- Aron AT, Loehr MO, Bogena J, Chang CJ. An endoperoxide reactivity-based FRET probe for ratiometric fluorescence imaging of labile iron pools in living cells. J Am Chem Soc. 2016;138(43):14338–14346. doi:10.1021/jacs.6b08016
- Kirbas Cilingir E, Besbinar O, Giro L, et al. Small warriors of nature: novel red emissive chlorophyllin carbon dots harnessing Fenton-fueled ferroptosis for in vitro and in vivo cancer treatment. Small. 2024;20:e2309283. doi:10.1002/smll.202309283
- Wang X, He M, Zhao Y, et al. Bimetallic PtPd atomic clusters as apoptosis/ferroptosis inducers for antineoplastic therapy through heterogeneous catalytic processes. ACS Nano. 2024. doi:10.1021/acsnano.3c11610
- Wang Y, Ding H, Zheng Y, et al. Alleviated NCOA4-mediated ferritinophagy protected RA FLSs from ferroptosis in lipopolysaccharide-induced inflammation under hypoxia. Inflamm Res. 2024;73(3):363–379. doi:10.1007/s00011-023-01842-9
- Ye L, Jin F, Kumar SK, Dai Y. The mechanisms and therapeutic targets of ferroptosis in cancer. Expert Opin Ther Targets. 2021;25(11):965–986. doi:10.1080/14728222.2021.2011206
- Ye L, Wen X, Qin J, et al. Metabolism-regulated ferroptosis in cancer progression and therapy. Cell Death Dis. 2024;15(3):196. doi:10.1038/s41419-024-06584-y
- Li X, Meng F, Wang H, et al. Iron accumulation and lipid peroxidation: implication of ferroptosis in hepatocellular carcinoma. Front Endocrinol (Lausanne). 2023;14:1319969. doi:10.3389/fendo.2023.1319969
- Qiu B, Zandkarimi F, Bezjian CT, et al. Phospholipids with two polyunsaturated fatty acyl tails promote ferroptosis. Cell. 2024;187(5):1177–1190.e18. doi:10.1016/j.cell.2024.01.030
- Samovich SN, Mikulska-Ruminska K, Dar HH, et al. Strikingly high activity of 15-Lipoxygenase towards di-polyunsaturated arachidonoyl/adrenoyl-phosphatidylethanolamines generates peroxidation signals of ferroptotic cell death. Angew Chem Int Ed Engl. 2024;63(9):e202314710. doi:10.1002/anie.202314710
- Bai XY, Liu XL, Deng ZZ, et al. Ferroptosis is a new therapeutic target for spinal cord injury. Front Neurosci. 2023;17:1136143. doi:10.3389/fnins.2023.1136143
- Fang X, Ardehali H, Min J, Wang F. The molecular and metabolic landscape of iron and ferroptosis in cardiovascular disease. Nat Rev Cardiol. 2023;20(1):7–23. doi:10.1038/s41569-022-00735-4
- Huang Y, Sarkhel S, Roy A, Mohan A. Interrelationship of lipid aldehydes (MDA, 4-HNE, and 4-ONE) mediated protein oxidation in muscle foods. Crit Rev Food Sci Nutr. 2023;1–17. doi:10.1080/10408398.2023.2245029
- Zou Y, Li H, Graham ET, et al. Cytochrome P450 oxidoreductase contributes to phospholipid peroxidation in ferroptosis. Nat Chem Biol. 2020;16(3):302–309. doi:10.1038/s41589-020-0472-6
- Zheng X, Zhang C. The regulation of ferroptosis by noncoding RNAs. Int J Mol Sci. 2023;24:17.
- Liu L, Ye Y, Lin R, et al. Ferroptosis: a promising candidate for exosome-mediated regulation in different diseases. Cell Commun Signal. 2024;22(1):6. doi:10.1186/s12964-023-01369-w
- Lara O, Janssen P, Mambretti M, et al. Compartmentalized role of xCT in supporting pancreatic tumor growth, inflammation and mood disturbance in mice. Brain Behav Immun. 2024;118:275–286. doi:10.1016/j.bbi.2024.03.001
- Kang YP, Mockabee-Macias A, Jiang C, et al. Non-canonical glutamate-cysteine ligase activity protects against ferroptosis. Cell Metab. 2021;33(1):174–189.e7. doi:10.1016/j.cmet.2020.12.007
- Zhang H, Pan J, Huang S, et al. Hydrogen sulfide protects cardiomyocytes from doxorubicin-induced ferroptosis through the SLC7A11/GSH/GPx4 pathway by Keap1 S-sulfhydration and Nrf2 activation. Redox Biol. 2024;70:103066. doi:10.1016/j.redox.2024.103066
- Zhang XD, Liu ZY, Wang MS, et al. Mechanisms and regulations of ferroptosis. Front Immunol. 2023;14:1269451. doi:10.3389/fimmu.2023.1269451
- Alborzinia H, Chen Z, Yildiz U, et al. LRP8-mediated selenocysteine uptake is a targetable vulnerability in MYCN-amplified neuroblastoma. EMBO Mol Med. 2023;15(8):e18014. doi:10.15252/emmm.202318014
- Miao H, Meng H, Zhang Y, Chen T, Zhang L, Cheng W. FSP1 inhibition enhances olaparib sensitivity in BRCA-proficient ovarian cancer patients via a nonferroptosis mechanism. Cell Death Differ. 2024;31:497–510. doi:10.1038/s41418-024-01263-z
- Fišar Z, Hroudová J. CoQ(10) and Mitochondrial dysfunction in alzheimer’s disease. Antioxidants (Basel). 2024;13:2.
- Huo J, Xu Z, Hosoe K, et al. Coenzyme Q10 prevents senescence and dysfunction caused by oxidative stress in vascular endothelial cells. Oxid Med Cell Longev. 2018;2018:3181759. doi:10.1155/2018/3181759
- Bersuker K, Hendricks JM, Li Z, et al. The CoQ oxidoreductase FSP1 acts parallel to GPX4 to inhibit ferroptosis. Nature. 2019;575:688–692. doi:10.1038/s41586-019-1705-2
- Díaz M, Valdés-Baizabal C, de Pablo DP, Marin R. Age-dependent changes in Nrf2/Keap1 and target antioxidant protein expression correlate to lipoxidative adducts, and are modulated by dietary N-3 LCPUFA in the hippocampus of mice. Antioxidants (Basel). 2024;13:2.
- Song Y, Qu Y, Mao C, Zhang R, Jiang D, Sun X. Post-translational modifications of Keap1: the state of the art. Front Cell Dev Biol. 2023;11:1332049. doi:10.3389/fcell.2023.1332049
- Gong Z, Xue L, Li H, et al. Targeting Nrf2 to treat thyroid cancer. Biomed Pharmacother. 2024;173:116324. doi:10.1016/j.biopha.2024.116324
- Li B, Nasser MI, Masood M, et al. Efficiency of traditional Chinese medicine targeting the Nrf2/HO-1 signaling pathway. Biomed Pharmacother. 2020;126:110074. doi:10.1016/j.biopha.2020.110074
- Zhang J, Zhang L, Yao G, Zhao H, Wu S. NRF2 is essential for iron-overload stimulated osteoclast differentiation through regulation of redox and iron homeostasis. Cell Biol Toxicol. 2023;39(6):3305–3321. doi:10.1007/s10565-023-09834-5
- Jiang X, Gao H, Cao Y, et al. SiNPs induce ferroptosis in HUVECs through p38 inhibiting NrF2 pathway. Front Public Health. 2023;11:1024130. doi:10.3389/fpubh.2023.1024130
- Liao P, Hemmerlin A, Bach TJ, Chye ML. The potential of the mevalonate pathway for enhanced isoprenoid production. Biotechnol Adv. 2016;34(5):697–713. doi:10.1016/j.biotechadv.2016.03.005
- Lee J, Roh JL. Targeting GPX4 in human cancer: implications of ferroptosis induction for tackling cancer resilience. Cancer Lett. 2023;559:216119. doi:10.1016/j.canlet.2023.216119
- Zheng J, Conrad M. The Metabolic Underpinnings of Ferroptosis. Cell Metab. 2020;32(6):920–937. doi:10.1016/j.cmet.2020.10.011
- Peuget S, Zhou X, Selivanova G. Translating p53-based therapies for cancer into the clinic. Nat Rev Cancer. 2024;24(3):192–215. doi:10.1038/s41568-023-00658-3
- Zhao C, Yu Y, Yin G, et al. Sulfasalazine promotes ferroptosis through AKT-ERK1/2 and P53-SLC7A11 in rheumatoid arthritis. Inflammopharmacology. 2024;32:1277–1294. doi:10.1007/s10787-024-01439-6
- Liu C, Shen Y, Cavdar O, Huang J, Fang H. Angiotensin II-induced vascular endothelial cells ferroptosis via P53-ALOX12 signal axis. Clin Exp Hypertens. 2023;45(1):2180019. doi:10.1080/10641963.2023.2180019
- Zhu T, Liu B, Wu D, Xu G, Fan Y. Autophagy regulates VDAC3 Ubiquitination by FBXW7 to promote erastin-induced ferroptosis in acute lymphoblastic leukemia. Front Cell Dev Biol. 2021;9:740884. doi:10.3389/fcell.2021.740884
- Luo M, Wu L, Zhang K, et al. miR-137 regulates ferroptosis by targeting glutamine transporter SLC1A5 in melanoma. Cell Death Differ. 2018;25(8):1457–1472. doi:10.1038/s41418-017-0053-8
- Sun X, Ou Z, Chen R, et al. Activation of the p62-Keap1-NRF2 pathway protects against ferroptosis in hepatocellular carcinoma cells. Hepatology. 2016;63(1):173–184. doi:10.1002/hep.28251
- Yang WS, SriRamaratnam R, Welsch ME, et al. Regulation of ferroptotic cancer cell death by GPX4. Cell. 2014;156(1–2):317–331. doi:10.1016/j.cell.2013.12.010
- Ferrada L, Barahona MJ, Vera M, Stockwell BR, Nualart F. Dehydroascorbic acid sensitizes cancer cells to system x(c)(-) inhibition-induced ferroptosis by promoting lipid droplet peroxidation. Cell Death Dis. 2023;14(9):637. doi:10.1038/s41419-023-06153-9
- Fang Y, Tan Q, Zhou H, Xu J, Gu Q. Discovery and optimization of 2-(trifluoromethyl)benzimidazole derivatives as novel ferroptosis inducers in vitro and in vivo. Eur J Med Chem. 2023;245(Pt 1):114905. doi:10.1016/j.ejmech.2022.114905
- Wang W, Ma F, Cheung YT, et al. Marine alkaloid lepadins E and H induce ferroptosis for cancer chemotherapy. J Med Chem. 2023;66(16):11201–11215. doi:10.1021/acs.jmedchem.3c00659
- Yin LB, Li ZW, Wang JL, et al. Sulfasalazine inhibits esophageal cancer cell proliferation by mediating ferroptosis. Chem Biol Drug Des. 2023;102(4):730–737. doi:10.1111/cbdd.14281
- Zhang D, Zhang M, Pang Y, Li M, Ma W. Folic acid-modified long-circulating liposomes loaded with sulfasalazine for targeted induction of ferroptosis in melanoma. ACS Biomater Sci Eng. 2024;10(1):588–598. doi:10.1021/acsbiomaterials.3c01223
- Ignarro RS, Facchini G, Vieira AS, et al. Sulfasalazine intensifies temozolomide cytotoxicity in human glioblastoma cells. Mol Cell Biochem. 2016;418(1–2):167–178. doi:10.1007/s11010-016-2742-x
- Guo L, Hu C, Yao M, Han G. Mechanism of sorafenib resistance associated with ferroptosis in HCC. Front Pharmacol. 2023;14:1207496. doi:10.3389/fphar.2023.1207496
- Zhou X, Zou L, Chen W, et al. Flubendazole, FDA-approved anthelmintic, elicits valid antitumor effects by targeting P53 and promoting ferroptosis in castration-resistant prostate cancer. Pharmacol Res. 2021;164:105305. doi:10.1016/j.phrs.2020.105305
- Zhang J, Gao M, Niu Y, Sun J. From DNMT1 degrader to ferroptosis promoter: drug repositioning of 6-Thioguanine as a ferroptosis inducer in gastric cancer. Biochem Biophys Res Commun. 2022;603:75–81. doi:10.1016/j.bbrc.2022.03.026
- Yang J, Zhou Y, Xie S, et al. Metformin induces Ferroptosis by inhibiting UFMylation of SLC7A11 in breast cancer. J Exp Clin Cancer Res. 2021;40(1):206. doi:10.1186/s13046-021-02012-7
- Deng C, Xiong L, Chen Y, Wu K, Wu J. Metformin induces ferroptosis through the Nrf2/HO-1 signaling in lung cancer. BMC Pulm Med. 2023;23(1):360. doi:10.1186/s12890-023-02655-6
- Liang X, Hu C, Han M, et al. Solasonine inhibits pancreatic cancer progression with involvement of ferroptosis induction. Front Oncol. 2022;12:834729. doi:10.3389/fonc.2022.834729
- Cui X, Gong Y, Ge J, et al. α-Solanine induces ferroptosis in nasopharyngeal carcinoma via targeting HSP90α/p53 axis. J Funct Foods. 2023;104:105517. doi:10.1016/j.jff.2023.105517
- Tang X, Ding H, Liang M, et al. Curcumin induces ferroptosis in non-small-cell lung cancer via activating autophagy. Thorac Cancer. 2021;12(8):1219–1230. doi:10.1111/1759-7714.13904
- Cao X, Li Y, Wang Y, et al. Curcumin suppresses tumorigenesis by ferroptosis in breast cancer. PLoS One. 2022;17(1):e0261370. doi:10.1371/journal.pone.0261370
- Li S, He Y, Chen K, et al. RSL3 Drives Ferroptosis through NF-κB pathway activation and GPX4 depletion in glioblastoma. Oxid Med Cell Longev. 2021;2021:2915019. doi:10.1155/2021/2915019
- Xing K, Bian X, Shi D, et al. miR-612 Enhances RSL3-Induced Ferroptosis of Hepatocellular Carcinoma Cells via Mevalonate Pathway. J Hepatocell Carcinoma. 2023;10:2173–2185. doi:10.2147/jhc.S433332
- Xu C, Xiao Z, Wang J, et al. Discovery of a potent glutathione peroxidase 4 inhibitor as a selective ferroptosis inducer. J Med Chem. 2021;64(18):13312–13326. doi:10.1021/acs.jmedchem.1c00569
- Randolph JT, O’Connor MJ, Han F, et al. Discovery of a potent chloroacetamide GPX4 inhibitor with bioavailability to enable target engagement in mice, a potential tool compound for inducing ferroptosis in vivo. J Med Chem. 2023;66(6):3852–3865. doi:10.1021/acs.jmedchem.2c01415
- Weïwer M, Bittker JA, Lewis TA, et al. Development of small-molecule probes that selectively kill cells induced to express mutant RAS. Bioorg Med Chem Lett. 2012;22(4):1822–1826. doi:10.1016/j.bmcl.2011.09.047
- Chen T, Leng J, Tan J, et al. Discovery of novel potent covalent glutathione peroxidase 4 inhibitors as highly selective ferroptosis inducers for the treatment of triple-negative breast cancer. J Med Chem. 2023;66(14):10036–10059. doi:10.1021/acs.jmedchem.3c00967
- Zhang X, Guo Y, Li H, Han L. FIN56, a novel ferroptosis inducer, triggers lysosomal membrane permeabilization in a TFEB-dependent manner in glioblastoma. J Cancer. 2021;12(22):6610–6619. doi:10.7150/jca.58500
- Liu J, Li J, Kang R, Tang D. Cell type-specific induction of ferroptosis to boost antitumor immunity. Oncoimmunology. 2023;12(1):2282252. doi:10.1080/2162402x.2023.2282252
- Cao Y, Wu B, Xu Y, et al. Discovery of GPX4 inhibitors through FP-based high-throughput screening. Eur J Med Chem. 2024;265:116044. doi:10.1016/j.ejmech.2023.116044
- Liu H, Forouhar F, Lin AJ, et al. Small-molecule allosteric inhibitors of GPX4. Cell Chem Biol. 2022;29(12):1680–1693.e9. doi:10.1016/j.chembiol.2022.11.003
- Wang L, Cai H, Hu Y, et al. A pharmacological probe identifies cystathionine β-synthase as a new negative regulator for ferroptosis. Cell Death Dis. 2018;9(10):1005. doi:10.1038/s41419-018-1063-2
- Rodman SN, Spence JM, Ronnfeldt TJ, et al. Enhancement of radiation response in breast cancer stem cells by inhibition of thioredoxin- and glutathione-dependent metabolism. Radiat Res. 2016;186(4):385–395. doi:10.1667/rr14463.1
- Kitsugi K, Noritake H, Matsumoto M, et al. Simvastatin inhibits hepatic stellate cells activation by regulating the ferroptosis signaling pathway. Biochim Biophys Acta Mol Basis Dis. 2023;1869(7):166750. doi:10.1016/j.bbadis.2023.166750
- Shueng PW, Chan HW, Lin WC, Kuo DY, Chuang HY. Orlistat resensitizes sorafenib-resistance in hepatocellular carcinoma cells through modulating metabolism. Int J Mol Sci. 2022;23(12):6501. doi:10.3390/ijms23126501
- Zhou W, Zhang J, Yan M, et al. Orlistat induces ferroptosis-like cell death of lung cancer cells. Front Med. 2021;15(6):922–932. doi:10.1007/s11684-020-0804-7
- Lee J, Roh JL. Targeting Nrf2 for ferroptosis-based therapy: implications for overcoming ferroptosis evasion and therapy resistance in cancer. Biochim Biophys Acta Mol Basis Dis. 2023;1869(7):166788. doi:10.1016/j.bbadis.2023.166788
- Chen H, Zhao R, Ge M, Sun Y, Li Y, Shan L. Gliotoxin, a natural product with ferroptosis inducing properties. Bioorg Chem. 2023;133:106415. doi:10.1016/j.bioorg.2023.106415
- Zhou C, Yu T, Zhu R, et al. Timosaponin AIII promotes non-small-cell lung cancer ferroptosis through targeting and facilitating HSP90 mediated GPX4 ubiquitination and degradation. Int J Biol Sci. 2023;19(5):1471–1489. doi:10.7150/ijbs.77979
- Zhu JM, Chen C, Kong M, et al. Discovery and optimization of indirubin derivatives as novel ferroptosis inducers for the treatment of colon cancer. Eur J Med Chem. 2023;261:115829. doi:10.1016/j.ejmech.2023.115829
- Greco G, Schnekenburger M, Catanzaro E, et al. Discovery of sulforaphane as an inducer of ferroptosis in U-937 leukemia cells: expanding its anticancer potential. Cancers. 2021;14(1):76. doi:10.3390/cancers14010076
- Cheu JW, Lee D, Li Q, et al. Ferroptosis suppressor protein 1 inhibition promotes tumor ferroptosis and anti-tumor immune responses in liver cancer. Cell Mol Gastroenterol Hepatol. 2023;16(1):133–159. doi:10.1016/j.jcmgh.2023.03.001
- Nakamura T, Hipp C, Santos Dias Mourão A, et al.. Phase separation of FSP1 promotes ferroptosis. Nature. 2023:619(7969):371–377. doi:10.1038/s41586-023-06255-6
- Hendricks JM, Doubravsky CE, Wehri E, et al. Identification of structurally diverse FSP1 inhibitors that sensitize cancer cells to ferroptosis. Cell Chem Biol. 2023;30(9):1090–1103.e7. doi:10.1016/j.chembiol.2023.04.007
- Nakamura T, Mishima E, Yamada N, et al. Integrated chemical and genetic screens unveil FSP1 mechanisms of ferroptosis regulation. Nat Struct Mol Biol. 2023;30(11):1806–1815. doi:10.1038/s41594-023-01136-y
- Yoshioka H, Kawamura T, Muroi M, et al. Identification of a small molecule that enhances ferroptosis via inhibition of ferroptosis suppressor protein 1 (FSP1). ACS Chem Biol. 2022;17(2):483–491. doi:10.1021/acschembio.2c00028
- Chen J, Zhou S, Zhang X, Zhao H. S-3’-hydroxy-7’, 2’, 4’-trimethoxyisoxane, a novel ferroptosis inducer, promotes NSCLC cell death through inhibiting Nrf2/HO-1 signaling pathway. Front Pharmacol. 2022;13:973611. doi:10.3389/fphar.2022.973611
- Gjorgieva Ackova D, Maksimova V, Smilkov K, Buttari B, Arese M, Saso L. Alkaloids as Natural NRF2 inhibitors: chemoprevention and cytotoxic action in cancer. Pharmaceuticals (Basel). 2023;16:6.
- Gai C, Yu M, Li Z, et al. Acetaminophen sensitizing erastin-induced ferroptosis via modulation of Nrf2/heme oxygenase-1 signaling pathway in non-small-cell lung cancer. J Cell Physiol. 2020;235(4):3329–3339. doi:10.1002/jcp.29221
- Liu J, Lin WP, Su W, et al. Sunitinib attenuates reactive MDSCs enhancing anti-tumor immunity in HNSCC. Int Immunopharmacol. 2023;119:110243. doi:10.1016/j.intimp.2023.110243
- Kong N, Chen X, Feng J, et al. Baicalin induces ferroptosis in bladder cancer cells by downregulating FTH1. Acta Pharm Sin B. 2021;11(12):4045–4054. doi:10.1016/j.apsb.2021.03.036
- Li J, Wei S, Marabada D, Wang Z, Huang Q. Research Progress of Natural Matrine Compounds and Synthetic Matrine Derivatives. Molecules. 2023;28(15):1.
- Zhu X, Chen X, Qiu L, Zhu J, Wang J. Norcantharidin induces ferroptosis via the suppression of NRF2/HO-1 signaling in ovarian cancer cells. Oncol Lett. 2022;24(4):359. doi:10.3892/ol.2022.13479
- Liu X, Peng X, Cen S, Yang C, Ma Z, Shi X. Wogonin induces ferroptosis in pancreatic cancer cells by inhibiting the Nrf2/GPX4 axis. Front Pharmacol. 2023;14:1129662. doi:10.3389/fphar.2023.1129662
- Liu J, Yang G, Zhang H. Glyphosate-triggered hepatocyte ferroptosis via suppressing Nrf2/GSH/GPX4 axis exacerbates hepatotoxicity. Sci Total Environ. 2023;862:160839. doi:10.1016/j.scitotenv.2022.160839
- He X, Zhou Y, Chen W, et al. Repurposed pizotifen malate targeting NRF2 exhibits anti-tumor activity through inducing ferroptosis in esophageal squamous cell carcinoma. Oncogene. 2023;42(15):1209–1223. doi:10.1038/s41388-023-02636-3
- Zhu X, Huang N, Ji Y, et al. Brusatol induces ferroptosis in oesophageal squamous cell carcinoma by repressing GSH synthesis and increasing the labile iron pool via inhibition of the NRF2 pathway. Biomed Pharmacother. 2023;167:115567. doi:10.1016/j.biopha.2023.115567
- Ma S, Henson ES, Chen Y, Gibson SB. Ferroptosis is induced following siramesine and lapatinib treatment of breast cancer cells. Cell Death Dis. 2016;7(7):e2307. doi:10.1038/cddis.2016.208
- Xiang Y, Chen X, Wang W, et al. Natural product erianin inhibits bladder cancer cell growth by inducing ferroptosis via NRF2 inactivation. Front Pharmacol. 2021;12:775506. doi:10.3389/fphar.2021.775506
- Li ZJ, Dai HQ, Huang XW, et al. Artesunate synergizes with sorafenib to induce ferroptosis in hepatocellular carcinoma. Acta Pharmacol Sin. 2021;42(2):301–310. doi:10.1038/s41401-020-0478-3
- Kuang Y, Sechi M, Nurra S, Ljungman M, Neamati N. Design and Synthesis of Novel Reactive Oxygen Species Inducers for the Treatment of Pancreatic Ductal Adenocarcinoma. J Med Chem. 2018;61(4):1576–1594. doi:10.1021/acs.jmedchem.7b01463
- Platzbecker U, Santini V, Fenaux P, et al. Imetelstat in patients with lower-risk myelodysplastic syndromes who have relapsed or are refractory to erythropoiesis-stimulating agents (IMerge): a multinational, randomised, double-blind, placebo-controlled, phase 3 trial. Lancet. 2024;403(10423):249–260. doi:10.1016/s0140-6736(23)01724-5
- Qiu C, Zhang X, Huang B, et al. Disulfiram, a ferroptosis inducer, triggers lysosomal membrane permeabilization by up-regulating ROS in glioblastoma. Onco Targets Ther. 2020;13:10631–10640. doi:10.2147/ott.S272312
- Cruz-Gregorio A, Aranda-Rivera AK. Quercetin and Ferroptosis. Life. 2023;13(8):1.
- Oh M, Jang SY, Lee JY, et al. The lipoprotein-associated phospholipase A2 inhibitor Darapladib sensitises cancer cells to ferroptosis by remodelling lipid metabolism. Nat Commun. 2023;14(1):5728. doi:10.1038/s41467-023-41462-9
- Yang L, Wang H, Yang X, et al. Auranofin mitigates systemic iron overload and induces ferroptosis via distinct mechanisms. Signal Transduct Target Ther. 2020;5(1):138. doi:10.1038/s41392-020-00253-0
- Zhao H, Zhang M, Zhang J, et al. Hinokitiol-iron complex is a ferroptosis inducer to inhibit triple-negative breast tumor growth. Cell Biosci. 2023;13(1):87. doi:10.1186/s13578-023-01044-0
- Huang D, Dong X, Li J, et al. Steroidal saponin SSPH I induces ferroptosis in HepG2 cells via regulating iron metabolism. Med Oncol. 2023;40(5):132. doi:10.1007/s12032-023-02000-1
- Hu C, Zu D, Xu J, et al. Polyphyllin B suppresses gastric tumor growth by modulating iron metabolism and inducing ferroptosis. Int J Biol Sci. 2023;19(4):1063–1079. doi:10.7150/ijbs.80324
- Wang S, Ren H, Fan C, Lin Q, Liu M, Tian J. Ochratoxin A induces renal cell ferroptosis by disrupting iron homeostasis and increasing ROS. J Agric Food Chem. 2024;72(3):1734–1744. doi:10.1021/acs.jafc.3c04495
- Zhang Y, Yang Y, Chen W, et al. BaP/BPDE suppressed endothelial cell angiogenesis to induce miscarriage by promoting MARCHF1/GPX4-mediated ferroptosis. Environ Int. 2023;180:108237. doi:10.1016/j.envint.2023.108237
- Du J, Krishnamoorthy K, Ramabhai V, Yang D. Targeting ferroptosis as a therapeutic implication in lung cancer treatment by a novel naphthoquinone inducer: juglone. Mol Biotechnol. 2023. doi:10.1007/s12033-023-01004-6
- Zhang Y, Tan Y, Liu S, et al. Implications of Withaferin A for the metastatic potential and drug resistance in hepatocellular carcinoma cells via Nrf2-mediated EMT and ferroptosis. Toxicol Mech Methods. 2023;33(1):47–55. doi:10.1080/15376516.2022.2075297
- Liang T, Dong H, Wang Z, et al. Discovery of novel urea derivatives as ferroptosis and autophagy inducer for human colon cancer treatment. Eur J Med Chem. 2024;268:116277. doi:10.1016/j.ejmech.2024.116277
- Kim YJ, Lim B, Kim SY, et al. Remodeling of sorafenib as an orally bioavailable ferroptosis inducer for Lung Cancer by chemical modification of adenine-binding motif. Biomed Pharmacother. 2024;176:116758. doi:10.1016/j.biopha.2024.116758
- Ma F, Li Y, Cai M, et al. ML162 derivatives incorporating a naphthoquinone unit as ferroptosis/apoptosis inducers: design, synthesis, anti-cancer activity, and drug-resistance reversal evaluation. Eur J Med Chem. 2024;270:116387. doi:10.1016/j.ejmech.2024.116387
- Wang R, Song W, Zhu J, et al. Biomimetic nano-chelate diethyldithiocarbamate Cu/Fe for enhanced metalloimmunity and ferroptosis activation in glioma therapy. J Control Release. 2024;368:84–96. doi:10.1016/j.jconrel.2024.02.004
- Zheng Z, Zhang S, Liu X, et al.. LRRK2 regulates ferroptosis through the system Xc-GSH-GPX4 pathway in the neuroinflammatory mechanism of Parkinson’s disease. J Cell Physiol. 2024;239. doi:10.1002/jcp.31250
- Dolma S, Lessnick SL, Hahn WC, Stockwell BR. Identification of genotype-selective antitumor agents using synthetic lethal chemical screening in engineered human tumor cells. Cancer Cell. 2003;3(3):285–296. doi:10.1016/s1535-6108(03)00050-3
- Zhang W, Li Q, Zhang Y, et al. Multiple myeloma with high expression of SLC7A11 is sensitive to erastin-induced ferroptosis. Apoptosis. 2024;29(3–4):412–423. doi:10.1007/s10495-023-01909-2
- Huo H, Zhou Z, Qin J, Liu W, Wang B, Gu Y. Erastin disrupts mitochondrial permeability transition pore (mPTP) and induces apoptotic death of colorectal cancer cells. PLoS One. 2016;11(5):e0154605. doi:10.1371/journal.pone.0154605
- Yu Y, Xie Y, Cao L, et al. The ferroptosis inducer erastin enhances sensitivity of acute myeloid leukemia cells to chemotherapeutic agents. Mol Cell Oncol. 2015;2(4):e1054549. doi:10.1080/23723556.2015.1054549
- Li Y, Zeng X, Lu D, Yin M, Shan M, Gao Y. Erastin induces ferroptosis via ferroportin-mediated iron accumulation in endometriosis. Hum Reprod. 2021;36(4):951–964. doi:10.1093/humrep/deaa363
- Song J, Wang H, Sheng J, et al. Vitexin attenuates chronic kidney disease by inhibiting renal tubular epithelial cell ferroptosis via NRF2 activation. Mol Med. 2023;29(1):147. doi:10.1186/s10020-023-00735-1
- Xu C, Li S, Chen J, et al. Doxorubicin and erastin co-loaded hydroxyethyl starch-polycaprolactone nanoparticles for synergistic cancer therapy. J Control Release. 2023;356:256–271. doi:10.1016/j.jconrel.2023.03.001
- Cheng Q, Bao L, Li M, Chang K, Yi X. Erastin synergizes with cisplatin via ferroptosis to inhibit ovarian cancer growth in vitro and in vivo. J Obstet Gynaecol Res. 2021;47(7):2481–2491. doi:10.1111/jog.14779
- Chen L, Li X, Liu L, Yu B, Xue Y, Liu Y. Erastin sensitizes glioblastoma cells to temozolomide by restraining xCT and cystathionine-γ-lyase function. Oncol Rep. 2015;33(3):1465–1474. doi:10.3892/or.2015.3712
- Woodley K, Dillingh LS, Giotopoulos G, et al. Mannose metabolism inhibition sensitizes acute myeloid leukaemia cells to therapy by driving ferroptotic cell death. Nat Commun. 2023;14(1):2132. doi:10.1038/s41467-023-37652-0
- Liu ZY, Chen G, Wang X, et al. Synergistic photochemo effects based on light-activatable dual prodrug nanoparticles for effective cancer therapy. Adv Healthc Mater. 2023;12(27):e2301133. doi:10.1002/adhm.202301133
- Larraufie MH, Yang WS, Jiang E, Thomas AG, Slusher BS, Stockwell BR. Incorporation of metabolically stable ketones into a small molecule probe to increase potency and water solubility. Bioorg Med Chem Lett. 2015;25(21):4787–4792. doi:10.1016/j.bmcl.2015.07.018
- Zhang Y, Tan H, Daniels JD, et al. Imidazole ketone erastin induces ferroptosis and slows tumor growth in a mouse lymphoma model. Cell Chem Biol. 2019;26(5):623–633.e9. doi:10.1016/j.chembiol.2019.01.008
- Nuzzo G, Gallo C, Crocetta F, et al. Identification of the marine alkaloid lepadin a as potential inducer of immunogenic cell death. Biomolecules. 2022;12(2):246. doi:10.3390/biom12020246
- Zhou J, Wu J, Fu F, et al. α-Solanine attenuates chondrocyte pyroptosis to improve osteoarthritis via suppressing NF-κB pathway. J Cell Mol Med. 2024;28(4):e18132. doi:10.1111/jcmm.18132
- Fekry MI, Ezzat SM, Salama MM, Alshehri OY, Al-Abd AM. Bioactive glycoalkaloides isolated from Solanum melongena fruit peels with potential anticancer properties against hepatocellular carcinoma cells. Sci Rep. 2019;9(1):1746. doi:10.1038/s41598-018-36089-6
- Zou T, Gu L, Yang L, et al. Alpha-solanine anti-tumor effects in non-small cell lung cancer through regulating the energy metabolism pathway. Recent Pat Anticancer Drug Discov. 2022;17(4):396–409. doi:10.2174/1574892817666220113144635
- Li TC, Chen NJ, Chen YY, He BJ, Zhou ZF. Solasonine induces apoptosis of the SGC-7901 human gastric cancer cell line in vitro via the mitochondria-mediated pathway. J Cell Mol Med. 2022;26(12):3387–3395. doi:10.1111/jcmm.17343
- Zeng YY, Luo YB, Ju XD, et al. Solasonine causes redox imbalance and mitochondrial oxidative stress of ferroptosis in lung adenocarcinoma. Front Oncol. 2022;12:874900. doi:10.3389/fonc.2022.874900
- Jin M, Shi C, Li T, Wu Y, Hu C, Huang G. Solasonine promotes ferroptosis of hepatoma carcinoma cells via glutathione peroxidase 4-induced destruction of the glutathione redox system. Biomed Pharmacother. 2020;129:110282. doi:10.1016/j.biopha.2020.110282
- Gao TH, Liao W, Lin LT, et al. Curcumae rhizoma and its major constituents against hepatobiliary disease: pharmacotherapeutic properties and potential clinical applications. Phytomedicine. 2022;102:154090. doi:10.1016/j.phymed.2022.154090
- Hesari A, Rezaei M, Rezaei M, et al. Effect of curcumin on glioblastoma cells. J Cell Physiol. 2019;234(7):10281–10288. doi:10.1002/jcp.27933
- Chen H, Li Z, Xu J, et al. Curcumin induces ferroptosis in follicular thyroid cancer by upregulating HO-1 expression. Oxid Med Cell Longev. 2023;2023:6896790. doi:10.1155/2023/6896790
- Zheng Y, Zhao T, Wang J, et al. Curcumol alleviates liver fibrosis through inducing autophagy and ferroptosis in hepatic stellate cells. FASEB j. 2022;36(12):e22665. doi:10.1096/fj.202200933RR
- Cheng HP, Bao XW, Luo YY, et al. Sulfasalazine ameliorates lipopolysaccharide-induced acute lung injury by inhibiting oxidative stress and nuclear factor-kappaB pathways. Int J Biochem Cell Biol. 2024;169:106530. doi:10.1016/j.biocel.2024.106530
- Wang F, Oudaert I, Tu C, et al. System Xc(-) inhibition blocks bone marrow-multiple myeloma exosomal crosstalk, thereby countering bortezomib resistance. Cancer Lett. 2022;535:215649. doi:10.1016/j.canlet.2022.215649
- Takatani-Nakase T, Ikushima C, Sakitani M, Nakase I. Regulatory network of ferroptosis and autophagy by targeting oxidative stress defense using sulfasalazine in triple-negative breast cancer. Life Sci. 2024;339:122411. doi:10.1016/j.lfs.2023.122411
- Giannitrapani L, Di Gaudio F, Cervello M, et al. Genetic biomarkers of sorafenib response in patients with hepatocellular carcinoma. Int J Mol Sci. 2024;25(4):2197. doi:10.3390/ijms25042197
- Yuan S, Wei C, Liu G, et al. Sorafenib attenuates liver fibrosis by triggering hepatic stellate cell ferroptosis via HIF-1α/SLC7A11 pathway. Cell Prolif. 2022;55(1):e13158. doi:10.1111/cpr.13158
- Zheng J, Sato M, Mishima E, Sato H, Proneth B, Conrad M. Sorafenib fails to trigger ferroptosis across a wide range of cancer cell lines. Cell Death Dis. 2021;12(7):698. doi:10.1038/s41419-021-03998-w
- Hong T, Lei G, Chen X, et al. PARP inhibition promotes ferroptosis via repressing SLC7A11 and synergizes with ferroptosis inducers in BRCA-proficient ovarian cancer. Redox Biol. 2021;42:101928. doi:10.1016/j.redox.2021.101928
- Yang WS, Stockwell BR. Synthetic lethal screening identifies compounds activating iron-dependent, nonapoptotic cell death in oncogenic-RAS-harboring cancer cells. Chem Biol. 2008;15(3):234–245. doi:10.1016/j.chembiol.2008.02.010
- Sui X, Zhang R, Liu S, et al. RSL3 Drives Ferroptosis Through GPX4 Inactivation and ROS Production in Colorectal Cancer. Front Pharmacol. 2018;9:1371. doi:10.3389/fphar.2018.01371
- Chen H, Qi Q, Wu N, et al. Aspirin promotes RSL3-induced ferroptosis by suppressing mTOR/SREBP-1/SCD1-mediated lipogenesis in PIK3CA-mutant colorectal cancer. Redox Biol. 2022;55:102426. doi:10.1016/j.redox.2022.102426
- Cheff DM, Huang C, Scholzen KC, et al. The ferroptosis inducing compounds RSL3 and ML162 are not direct inhibitors of GPX4 but of TXNRD1. Redox Biol. 2023;62:102703. doi:10.1016/j.redox.2023.102703
- Liu S, Wu W, Chen Q, et al. TXNRD1: a key regulator involved in the ferroptosis of CML cells induced by cysteine depletion in vitro. Oxid Med Cell Longev. 2021;2021:7674565. doi:10.1155/2021/7674565
- Eaton JK, Furst L, Ruberto RA, et al. Selective covalent targeting of GPX4 using masked nitrile-oxide electrophiles. Nat Chem Biol. 2020;16(5):497–506. doi:10.1038/s41589-020-0501-5
- Hou DY, Cheng DB, Zhang NY, et al. In vivo assembly enhanced binding effect augments tumor specific ferroptosis therapy. Nat Commun. 2024;15(1):454. doi:10.1038/s41467-023-44665-2
- Li J, Liu J, Zhou Z, et al. Tumor-specific GPX4 degradation enhances ferroptosis-initiated antitumor immune response in mouse models of pancreatic cancer. Sci Transl Med. 2023;15(720):eadg3049. doi:10.1126/scitranslmed.adg3049
- Shimada K, Skouta R, Kaplan A, et al. Global survey of cell death mechanisms reveals metabolic regulation of ferroptosis. Nat Chem Biol. 2016;12(7):497–503. doi:10.1038/nchembio.2079
- Fruehauf JP, Zonis S, al-Bassam M, et al. Selective and synergistic activity of L-S, R-buthionine sulfoximine on malignant melanoma is accompanied by decreased expression of glutathione-S-transferase. Pigment Cell Res. 1997;10(4):236–249. doi:10.1111/j.1600-0749.1997.tb00490.x
- Ye M, Lu F, Chen J, et al. Orlistat induces ferroptosis in pancreatic neuroendocrine tumors by inactivating the MAPK pathway. J Cancer. 2023;14(8):1458–1469. doi:10.7150/jca.83118
- Liu S, Zhang HL, Li J, et al. Tubastatin A potently inhibits GPX4 activity to potentiate cancer radiotherapy through boosting ferroptosis. Redox Biol. 2023;62:102677. doi:10.1016/j.redox.2023.102677
- Baralić K, Živanović J, Marić Đ, et al. Sulforaphane-A compound with potential health benefits for disease prevention and treatment: insights from pharmacological and toxicological experimental studies. Antioxidants (Basel). 2024;13(2). doi:10.3390/antiox13020147
- Li W, Liang L, Liu S, Yi H, Zhou Y. FSP1: a key regulator of ferroptosis. Trends Mol Med. 2023;29(9):753–764. doi:10.1016/j.molmed.2023.05.013
- Bebber CM, von Karstedt S. FSP1 inhibition: pick your pocket. Nat Struct Mol Biol. 2023;30(11):1618–1619. doi:10.1038/s41594-023-01145-x
- Doll S, Freitas FP, Shah R, et al. FSP1 is a glutathione-independent ferroptosis suppressor. Nature. 2019;575:693–698. doi:10.1038/s41586-019-1707-0
- Zhou J, Zhang L, Yan J, Hou A, Sui W, Sun M. Curcumin Induces Ferroptosis in A549 CD133(+) Cells through the GSH-GPX4 and FSP1-CoQ10-NAPH Pathways. Discov Med. 2023;35(176):251–263. doi:10.24976/Discov.Med.202335176.26
- Liu MR, Shi C, Song QY, et al.. Sorafenib induces ferroptosis by promoting TRIM54-mediated FSP1 ubiquitination and degradation in hepatocellular carcinoma. Hepatol Commun. 2023;7(10). doi:10.1097/HC9.0000000000000246
- Kim JW, Kim MJ, Han TH, et al. FSP1 confers ferroptosis resistance in KEAP1 mutant non-small cell lung carcinoma in NRF2-dependent and -independent manner. Cell Death Dis. 2023;14(8):567. doi:10.1038/s41419-023-06070-x
- Sun S, Shen J, Jiang J, Wang F, Min J. Targeting ferroptosis opens new avenues for the development of novel therapeutics. Signal Transduct Target Ther. 2023;8(1):372. doi:10.1038/s41392-023-01606-1
- Yan R, Lin B, Jin W, Tang L, Hu S, Cai R. NRF2, a Superstar of Ferroptosis. Antioxidants (Basel). 2023;12(9):1.
- Yu X, Wang Y, Tan J, et al. Inhibition of NRF2 enhances the acute myeloid leukemia cell death induced by venetoclax via the ferroptosis pathway. Cell Death Discov. 2024;10(1):35. doi:10.1038/s41420-024-01800-2
- Kar A, Mukherjee SK, Barik S, Hossain ST. Antimicrobial activity of trigonelline hydrochloride against pseudomonas aeruginosa and its quorum-sensing regulated molecular mechanisms on biofilm formation and virulence. ACS Infect Dis. 2024;10(2):746–762. doi:10.1021/acsinfecdis.3c00617
- Fouzder C, Mukhuty A, Mukherjee S, Malick C, Kundu R. Trigonelline inhibits Nrf2 via EGFR signalling pathway and augments efficacy of Cisplatin and Etoposide in NSCLC cells. Toxicol In Vitro. 2021;70:105038. doi:10.1016/j.tiv.2020.105038
- Bajek-Bil A, Chmiel M, Włoch A, Stompor-Gorący M. Baicalin-current trends in detection methods and health-promoting properties. Pharmaceuticals (Basel). 2023;16(4):570. doi:10.3390/ph16040570
- Wen RJ, Dong X, Zhuang HW, et al. Baicalin induces ferroptosis in osteosarcomas through a novel Nrf2/xCT/GPX4 regulatory axis. Phytomedicine. 2023;116:154881. doi:10.1016/j.phymed.2023.154881
- Jin J, Fan Z, Long Y, et al. Matrine induces ferroptosis in cervical cancer through activation of piezo1 channel. Phytomedicine. 2024;122:155165. doi:10.1016/j.phymed.2023.155165
- Chen MH, Gu YY, Zhang AL, Sze DM, Mo SL, May BH. Biological effects and mechanisms of matrine and other constituents of Sophora flavescens in colorectal cancer. Pharmacol Res. 2021;171:105778. doi:10.1016/j.phrs.2021.105778
- Wang X, Zhu W, Xing M, Zhu H, Chen E, Zhou J. Matrine disrupts Nrf2/GPX4 antioxidant system and promotes hepatocyte ferroptosis. Chem Biol Interact. 2023;384:110713. doi:10.1016/j.cbi.2023.110713
- Zhou Y, Fan X, Jiao T, et al. SIRT6 as a key event linking P53 and NRF2 counteracts APAP-induced hepatotoxicity through inhibiting oxidative stress and promoting hepatocyte proliferation. Acta Pharm Sin B. 2021;11(1):89–99. doi:10.1016/j.apsb.2020.06.016
- Yamada N, Komada T, Ohno N, Takahashi M. Acetaminophen-induced hepatotoxicity: different mechanisms of Acetaminophen-induced ferroptosis and mitochondrial damage. Arch Toxicol. 2020;94(6):2255–2257. doi:10.1007/s00204-020-02722-5
- Li D, Song C, Song C, et al. Sunitinib induces cardiotoxicity through modulating oxidative stress and Nrf2-dependent ferroptosis in vitro and in vivo. Chem Biol Interact. 2024;388:110829. doi:10.1016/j.cbi.2023.110829
- Bruedigam C, Porter AH, Song A, et al. Imetelstat-mediated alterations in fatty acid metabolism to induce ferroptosis as a therapeutic strategy for acute myeloid leukemia. Nat Cancer. 2024;5(1):47–65. doi:10.1038/s43018-023-00653-5
- Linkermann A. Telomerase inhibitor imetelstat kills AML cells via lipid ROS and ferroptosis. Nat Cancer. 2024;5(1):12–13. doi:10.1038/s43018-023-00654-4
- Zeng M, Wu B, Wei W, et al. Disulfiram: a novel repurposed drug for cancer therapy. Chin Med J (Engl). 2024. doi:10.1097/cm9.0000000000002909
- Xu Y, Lu L, Luo J, et al. Disulfiram alone functions as a radiosensitizer for pancreatic cancer both in vitro and in vivo. Front Oncol. 2021;11:683695. doi:10.3389/fonc.2021.683695
- Wu L, Meng F, Dong L, et al. Disulfiram and BKM120 in combination with chemotherapy impede tumor progression and delay tumor recurrence in tumor initiating Cell-Rich TNBC. Sci Rep. 2019;9(1):236. doi:10.1038/s41598-018-35619-6
- Wang H, Dong Z, Liu J, Zhu Z, Najafi M. Mechanisms of Cancer-killing by Quercetin; A Review on Cell Death Mechanisms. Anticancer Agents Med Chem. 2023;23(9):999–1012. doi:10.2174/1871520623666230120094158
- Kiran KS, Kameshwar VH, Mudnakudu Nagaraju KK, et al. Diosmin: a Daboia russelii venom PLA(2)s inhibitor- purified, and characterized from Oxalis corniculata L medicinal plant. J Ethnopharmacol. 2024;318(Pt B):116977. doi:10.1016/j.jep.2023.116977
- Ido Y, Muto N, Inada A, et al. Induction of apoptosis by hinokitiol, a potent iron chelator, in teratocarcinoma F9 cells is mediated through the activation of caspase-3. Cell Prolif. 1999;32(1):63–73. doi:10.1046/j.1365-2184.1999.3210063.x
- Zhou JL, Huang XY, Qiu HC, et al. SSPH I, a novel anti-cancer saponin, inhibits autophagy and induces apoptosis via ROS Accumulation and ERK1/2 signaling pathway in hepatocellular carcinoma cells. Onco Targets Ther. 2020;13:5979–5991. doi:10.2147/ott.S253234
- Lee HJ, Kim HD, Ryu D. Practical strategies to reduce ochratoxin A in foods. Toxins (Basel). 2024;16(1):58. doi:10.3390/toxins16010058
- Zhang J, Fu M, Wu J, et al. The anti-glioma effect of juglone derivatives through ROS generation. Front Pharmacol. 2022;13:911760. doi:10.3389/fphar.2022.911760
- Zhang W, Jiang B, Liu Y, Xu L, Wan M. Bufotalin induces ferroptosis in non-small cell lung cancer cells by facilitating the ubiquitination and degradation of GPX4. Free Radic Biol Med. 2022;180:75–84. doi:10.1016/j.freeradbiomed.2022.01.009
- Xing Z, Su A, Mi L, et al. Withaferin A: a dietary supplement with promising potential as an anti-tumor therapeutic for cancer treatment - pharmacology and mechanisms. Drug Des Devel Ther. 2023;17:2909–2929. doi:10.2147/dddt.S422512
- Du J, Wang X, Li Y, et al. DHA exhibits synergistic therapeutic efficacy with cisplatin to induce ferroptosis in pancreatic ductal adenocarcinoma via modulation of iron metabolism. Cell Death Dis. 2021;12(7):705. doi:10.1038/s41419-021-03996-y
- Hsu WT, Huang CY, Yen CYT, Cheng AL, Hsieh PCH. The HER2 inhibitor lapatinib potentiates doxorubicin-induced cardiotoxicity through iNOS signaling. Theranostics. 2018;8(12):3176–3188. doi:10.7150/thno.23207
- Zhang S, Liu Z, Xia T, et al. Ginkgolic acid inhibits the expression of SAE1 and induces ferroptosis to exert an anti-hepatic fibrosis effect. Phytomedicine. 2024;126:155148. doi:10.1016/j.phymed.2023.155148
- Viswanadhapalli S, Luo Y, Sareddy GR, et al. EC359: a first-in-class small-molecule inhibitor for targeting oncogenic LIFR signaling in triple-negative breast cancer. Mol Cancer Ther. 2019;18(8):1341–1354. doi:10.1158/1535-7163.Mct-18-1258
- Feng CZ, Li NZ, Hu XB, et al. The LIFR-targeting small molecules EC330/EC359 are potent ferroptosis inducers. Genes Dis. 2023;10(3):735–738. doi:10.1016/j.gendis.2022.10.016
- Sinitsky M, Asanov M, Sinitskaya A, et al. Atorvastatin can modulate DNA damage repair in endothelial cells exposed to mitomycin C. Int J Mol Sci. 2023;24(7):6783. doi:10.3390/ijms24076783
- Zhang Q, Qu H, Chen Y, et al. Atorvastatin induces mitochondria-dependent ferroptosis via the modulation of Nrf2-xCT/GPx4 Axis. Front Cell Dev Biol. 2022;10:806081. doi:10.3389/fcell.2022.806081
- Warita K, Ishikawa T, Sugiura A, et al. Concomitant attenuation of HMGCR expression and activity enhances the growth inhibitory effect of atorvastatin on TGF-β-treated epithelial cancer cells. Sci Rep. 2021;11(1):12763. doi:10.1038/s41598-021-91928-3
- Liu T, Shu J, Liu Y, et al. Atorvastatin attenuates ferroptosis-dependent myocardial injury and inflammation following coronary microembolization via the Hif1a/Ptgs2 pathway. Front Pharmacol. 2022;13:1057583. doi:10.3389/fphar.2022.1057583
- Feng M, Xu H, Zhou W, Pan Y. The BRD4 inhibitor JQ1 augments the antitumor efficacy of abemaciclib in preclinical models of gastric carcinoma. J Exp Clin Cancer Res. 2023;42(1):44. doi:10.1186/s13046-023-02615-2
- Sui S, Zhang J, Xu S, Wang Q, Wang P, Pang D. Ferritinophagy is required for the induction of ferroptosis by the bromodomain protein BRD4 inhibitor (+)-JQ1 in cancer cells. Cell Death Dis. 2019;10(5):331. doi:10.1038/s41419-019-1564-7
- Go S, Kang M, Kwon SP, Jung M, Jeon OH, Kim BS. The senolytic drug JQ1 removes senescent cells via ferroptosis. Tissue Eng Regen Med. 2021;18(5):841–850. doi:10.1007/s13770-021-00346-z
- Våtsveen TK, Myhre MR, Steen CB, et al. Artesunate shows potent anti-tumor activity in B-cell lymphoma. J Hematol Oncol. 2018;11(1):23. doi:10.1186/s13045-018-0561-0
- Ooko E, Saeed ME, Kadioglu O, et al. Artemisinin derivatives induce iron-dependent cell death (ferroptosis) in tumor cells. Phytomedicine. 2015;22(11):1045–1054. doi:10.1016/j.phymed.2015.08.002
- McDowell A, Hill KS, McCorkle JR, et al.. Preclinical evaluation of artesunate as an antineoplastic agent in ovarian cancer treatment. Diagnostics (Basel). 2021;11(3):395.
- Roh JL, Kim EH, Jang H, Shin D. Nrf2 inhibition reverses the resistance of cisplatin-resistant head and neck cancer cells to artesunate-induced ferroptosis. Redox Biol. 2017;11:254–262. doi:10.1016/j.redox.2016.12.010
- Hughes GR, Dudey AP, Hemmings AM, Chantry A. Frontiers in PROTACs. Drug Discov Today. 2021;26(10):2377–2383. doi:10.1016/j.drudis.2021.04.010
- Ignatov M, Jindal A, Kotelnikov S, et al. High accuracy prediction of PROTAC complex structures. J Am Chem Soc. 2023;145(13):7123–7135. doi:10.1021/jacs.2c09387
- Wang H, Wang C, Li B, et al. Discovery of ML210-Based glutathione peroxidase 4 (GPX4) degrader inducing ferroptosis of human cancer cells. Eur J Med Chem. 2023;254:115343. doi:10.1016/j.ejmech.2023.115343
- Hu M, Li X, Wang L, et al. ZX703: a small-molecule degrader of GPX4 inducing ferroptosis in human cancer cells. ACS Med Chem Lett. 2024;15(3):406–412. doi:10.1021/acsmedchemlett.3c00571
- Luo T, Zheng Q, Shao L, Ma T, Mao L, Wang M. Intracellular delivery of glutathione peroxidase degrader induces ferroptosis in vivo. Angew Chem Int Ed Engl. 2022;61(39):e202206277. doi:10.1002/anie.202206277
- Cai M, Ma F, Hu C, et al. Design and synthesis of proteolysis-targeting chimeras (PROTACs) as degraders of glutathione peroxidase 4. Bioorg Med Chem. 2023;90:117352. doi:10.1016/j.bmc.2023.117352
- Zhu L, Hu S, Yan X, et al. Ugi reaction-assisted assembly of covalent PROTACs against glutathione peroxidase 4. Bioorg Chem. 2023;134:106461. doi:10.1016/j.bioorg.2023.106461
- Wang C, Zheng C, Wang H, et al. Dual degradation mechanism of GPX4 degrader in induction of ferroptosis exerting anti-resistant tumor effect. Eur J Med Chem. 2023;247:115072. doi:10.1016/j.ejmech.2022.115072
- Song H, Liang J, Guo Y, et al. A potent GPX4 degrader to induce ferroptosis in HT1080 cells. Eur J Med Chem. 2024;265:116110. doi:10.1016/j.ejmech.2023.116110
- Zheng C, Wang C, Sun D, et al. Structure-activity relationship study of RSL3-based GPX4 degraders and its potential noncovalent optimization. Eur J Med Chem. 2023;255:115393. doi:10.1016/j.ejmech.2023.115393
- Yu J, Zhu F, Yang Y, et al. Ultrasmall iron-doped zinc oxide nanoparticles for ferroptosis assisted sono-chemodynamic cancer therapy. Colloids Surf B Biointerfaces. 2023;232:113606. doi:10.1016/j.colsurfb.2023.113606
- Chen M, Shen Y, Pu Y, et al. Biomimetic inducer enabled dual ferroptosis of tumor and M2-type macrophages for enhanced tumor immunotherapy. Biomaterials. 2023;303:122386. doi:10.1016/j.biomaterials.2023.122386
- Mu M, Wang Y, Zhao S, et al. Engineering a pH/glutathione-responsive tea polyphenol nanodevice as an apoptosis/ferroptosis-inducing agent. ACS Appl Bio Mater. 2020;3(7):4128–4138. doi:10.1021/acsabm.0c00225
- Chen Q, Ma X, Xie L, et al. Iron-based nanoparticles for MR imaging-guided ferroptosis in combination with photodynamic therapy to enhance cancer treatment. Nanoscale. 2021;13(9):4855–4870. doi:10.1039/d0nr08757b
- Zeng L, Ding S, Cao Y, et al. A MOF-based potent ferroptosis inducer for enhanced radiotherapy of triple negative breast cancer. ACS Nano. 2023;17(14):13195–13210. doi:10.1021/acsnano.3c00048
- Liu Z, Kang R, Yang N, et al. Tetrahydrobiopterin inhibitor-based antioxidant metabolic strategy for enhanced cancer ferroptosis-immunotherapy. J Colloid Interface Sci. 2024;658:100–113. doi:10.1016/j.jcis.2023.12.042
- Zhao LX, Gong ZQ, Zhang Q, et al. Graphdiyne nanoplatforms for photothermal-ferroptosis combination therapy against glioblastoma. J Control Release. 2023;359:12–25. doi:10.1016/j.jconrel.2023.05.035
- Cheng Z, Xue C, Liu M, et al. Injectable microenvironment-responsive hydrogels with redox-activatable supramolecular prodrugs mediate ferroptosis-immunotherapy for postoperative tumor treatment. Acta Biomater. 2023;169:289–305. doi:10.1016/j.actbio.2023.08.002
- Yao X, Xie R, Cao Y, et al. Simvastatin induced ferroptosis for triple-negative breast cancer therapy. J Nanobiotechnology. 2021;19(1):311. doi:10.1186/s12951-021-01058-1
- Wu F, Du Y, Yang J, et al. Peroxidase-like active nanomedicine with dual glutathione depletion property to restore oxaliplatin chemosensitivity and promote programmed cell death. ACS Nano. 2022;16(3):3647–3663. doi:10.1021/acsnano.1c06777
- Liang Y, Peng C, Su N, et al. Tumor microenvironments self-activated cascade catalytic nanoscale metal organic frameworks as ferroptosis inducer for radiosensitization. Chem Eng J. 2022;437:135309. doi:10.1016/j.cej.2022.135309
- Xu T, Ma Y, Yuan Q, et al. Enhanced ferroptosis by oxygen-boosted phototherapy based on a 2-in-1 nanoplatform of ferrous hemoglobin for tumor synergistic therapy. ACS Nano. 2020;14(3):3414–3425. doi:10.1021/acsnano.9b09426
- Bao W, Liu X, Lv Y, et al. Nanolongan with multiple on-demand conversions for ferroptosis-apoptosis combined anticancer therapy. ACS Nano. 2019;13(1):260–273. doi:10.1021/acsnano.8b05602
- Sang M, Luo R, Bai Y, et al. Mitochondrial membrane anchored photosensitive nano-device for lipid hydroperoxides burst and inducing ferroptosis to surmount therapy-resistant cancer. Theranostics. 2019;9(21):6209–6223. doi:10.7150/thno.36283
- Wan X, Song L, Pan W, Zhong H, Li N, Tang B. Tumor-targeted cascade nanoreactor based on metal-organic frameworks for synergistic ferroptosis-starvation anticancer therapy. ACS Nano. 2020;14(9):11017–11028. doi:10.1021/acsnano.9b07789
- Yao L, Zhao MM, Luo QW, et al. Carbon Quantum dots-based nanozyme from coffee induces cancer cell ferroptosis to activate antitumor immunity. ACS Nano. 2022;16(6):9228–9239. doi:10.1021/acsnano.2c01619
- Zhou LL, Guan Q, Li WY, Zhang Z, Li YA, Dong YB. A ferrocene-functionalized covalent organic framework for enhancing chemodynamic therapy via redox dyshomeostasis. Small. 2021;17(32):e2101368. doi:10.1002/smll.202101368
- Wang S, Li F, Qiao R, et al. Arginine-rich manganese silicate nanobubbles as a ferroptosis-inducing agent for tumor-targeted theranostics. ACS Nano. 2018;12(12):12380–12392. doi:10.1021/acsnano.8b06399
- Xue CC, Li MH, Zhao Y, et al. Tumor microenvironment-activatable Fe-doxorubicin preloaded amorphous CaCO(3) nanoformulation triggers ferroptosis in target tumor cells. Sci Adv. 2020;6(18):eaax1346. doi:10.1126/sciadv.aax1346
- Xu XL, Zhang NN, Shu GF, et al. A luminol-based self-illuminating nanocage as a reactive oxygen species amplifier to enhance deep tumor penetration and synergistic therapy. ACS Nano. 2021;15(12):19394–19408. doi:10.1021/acsnano.1c05891
- Liang X, Chen M, Bhattarai P, Hameed S, Tang Y, Dai Z. Complementing cancer photodynamic therapy with ferroptosis through iron oxide loaded porphyrin-grafted lipid nanoparticles. ACS Nano. 2021;15(12):20164–20180. doi:10.1021/acsnano.1c08108
- Xu Y, Guo Y, Zhang C, et al. Fibronectin-coated metal-phenolic networks for cooperative tumor chemo-/chemodynamic/immune therapy via enhanced ferroptosis-mediated immunogenic cell death. ACS Nano. 2022;16(1):984–996. doi:10.1021/acsnano.1c08585
- Cao K, Du Y, Bao X, et al. Glutathione-bioimprinted nanoparticles targeting of N6-methyladenosine fto demethylase as a strategy against leukemic stem cells. Small. 2022;18(13):e2106558. doi:10.1002/smll.202106558
- Zhang F, Li F, Lu GH, et al. Engineering magnetosomes for ferroptosis/immunomodulation synergism in cancer. ACS Nano. 2019;13(5):5662–5673. doi:10.1021/acsnano.9b00892
- Tian X, Ruan L, Zhou S, et al. Appropriate Size of Fe(3)O(4) nanoparticles for cancer therapy by ferroptosis. ACS Appl Bio Mater. 2022;5(4):1692–1699. doi:10.1021/acsabm.2c00068
- Liu J, Li X, Chen J, et al. Arsenic-loaded biomimetic iron oxide nanoparticles for enhanced ferroptosis-inducing therapy of hepatocellular carcinoma. ACS Appl Mater Interfaces. 2023;15(5):6260–6273. doi:10.1021/acsami.2c14962
- Zhang Z, Wang L, Guo Z, Sun Y, Yan J. A pH-sensitive imidazole grafted polymeric micelles nanoplatform based on ROS amplification for ferroptosis-enhanced chemodynamic therapy. Colloids Surf B Biointerfaces. 2024;237:113871. doi:10.1016/j.colsurfb.2024.113871
- Qi A, Wang C, Ni S, et al. Intravesical mucoadhesive hydrogel induces chemoresistant bladder cancer ferroptosis through delivering iron oxide nanoparticles in a three-tier strategy. ACS Appl Mater Interfaces. 2021;13(44):52374–52384. doi:10.1021/acsami.1c14944
- Bilbao‐Asensio M, Ruiz‐de‐Angulo A, Arguinzoniz AG, et al.. Redox‐triggered nanomedicine via lymphatic delivery: inhibition of melanoma growth by ferroptosis enhancement and a Pt(IV)‐prodrug chemoimmunotherapy approach. Adv Ther. 2022;6(2):1.
- Liu Y, Pi F, He L, Yang F, Chen T. Oxygen vacancy-rich manganese nanoflowers as ferroptosis inducers for tumor radiotherapy. Small. 2024;e2310118. doi:10.1002/smll.202310118
- Shao J, Zhang J, Xue K, et al. Upconversion dihydroartemisinin-loaded nanocomposites for NIR-enhanced ferroptosis of glioblastoma cells. ACS Appl Nano Mater. 2024;7(8):9106–9115. doi:10.1021/acsanm.4c00548
- Xu J, Zhang W, Cai Z, et al.. Tetrahedron DNA nanostructure/iron-based nanomaterials for combined tumor therapy. Chin. Chem. Lett. 2024:109620. doi:10.1016/j.cclet.2024.109620
- Lin X, Chen H, Deng T, et al. Improved immune response for colorectal cancer therapy triggered by multifunctional nanocomposites with self-amplifying antitumor ferroptosis. ACS Appl Mater Interfaces. 2024;16(11):13481–13495. doi:10.1021/acsami.3c16813
- Zhu X, Xie L, Tian J, Jiang Y, Song E, Song Y. A multi-mode Rhein-based nano-platform synergizing ferrotherapy/chemotherapy-induced immunotherapy for enhanced tumor therapy. Acta Biomater. 2024;180:383–393. doi:10.1016/j.actbio.2024.03.030
- Chen Y, Li X, Luo K, et al. Hyperthermia/glutathione-triggered ferritin nanoparticles amplify the ferroptosis for synergistic tumor therapy. Mater Today Bio. 2024;26:101085. doi:10.1016/j.mtbio.2024.101085
- Zhao X, Leng D, Wang H, et al. An acid-responsive iron-based nanocomposite for OSCC treatment. J Dent Res. 2024;103(6):612–621. doi:10.1177/00220345241238154
- Wang M, Yu A, Han W, Chen J, Lu C, Tu X. Self-assembled metal-phenolic nanocomplexes comprised of green tea catechin for tumor-specific ferroptosis. Mater Today Bio. 2024;26:101040. doi:10.1016/j.mtbio.2024.101040
- Zou W, Gao F, Meng Z, et al. Lactic acid responsive sequential production of hydrogen peroxide and consumption of glutathione for enhanced ferroptosis tumor therapy. J Colloid Interface Sci. 2024;663:787–800. doi:10.1016/j.jcis.2024.03.001
- Zhu J, Zhao S, Zhu Y, et al. Sorafenib sensitization in tumor therapy by iron overload and AMPK activation. Nano Res. 2024. doi:10.1007/s12274-024-6602-9
- Sun B, Zheng X, Zhang X, Zhang H, Jiang Y. Oxaliplatin-Loaded Mil-100(Fe) for chemotherapy-ferroptosis combined therapy for gastric cancer. ACS Omega. 2024;9(14):16676–16686. doi:10.1021/acsomega.4c00658
- Zhao X, Wang X, Zhang W, et al. A ferroptosis-inducing arsenene-iridium nanoplatform for synergistic immunotherapy in pancreatic cancer. Angew Chem Int Ed Engl. 2024;63(15):e202400829. doi:10.1002/anie.202400829
- Bai J, Zhang X, Zhao Z, et al.. CuO nanozymes catalyze cysteine and glutathione depletion induced ferroptosis and cuproptosis for synergistic tumor therapy. Small. 2024:e2400326. doi:10.1002/smll.202400326
- Wang Y, Wu X, Bao X, Mou X. Progress in the mechanism of the effect of Fe(3)O(4) nanomaterials on ferroptosis in tumor cells. Molecules. 2023;28(11):1.
- Wang S, He H, Mao Y, Zhang Y, Gu N. Advances in atherosclerosis theranostics harnessing iron oxide-based nanoparticles. Adv Sci. 2024;11(17):e2308298. doi:10.1002/advs.202308298
- Gao L, Fan K, Yan X. Iron oxide nanozyme: a multifunctional enzyme mimetic for biomedical applications. Theranostics. 2017;7(13):3207–3227. doi:10.7150/thno.19738
- Dadfar SM, Roemhild K, Drude NI, et al. Iron oxide nanoparticles: diagnostic, therapeutic and theranostic applications. Adv Drug Deliv Rev. 2019;138:302–325. doi:10.1016/j.addr.2019.01.005
- Jalali A, Bari IJ, Salehzadeh A. Menthol conjugated magnetic iron oxide nanoparticles induce apoptosis and increase caspase-8 gene expression in gastric cancer cell line. BioNanoScience. 2024. doi:10.1007/s12668-024-01377-1
- Mu QG, Lin G, Jeon M, et al. Iron oxide nanoparticle targeted chemo-immunotherapy for triple negative breast cancer. Mater Today (Kidlington). 2021;50:149–169. doi:10.1016/j.mattod.2021.08.002
- Jiang Q, Wang K, Zhang X, et al. Platelet membrane-camouflaged magnetic nanoparticles for ferroptosis-enhanced cancer immunotherapy. Small. 2020;16(22):e2001704. doi:10.1002/smll.202001704
- Lyu Z, Kou Y, Fu Y, et al. Comparative transcriptomics revealed neurodevelopmental impairments and ferroptosis induced by extremely small iron oxide nanoparticles. Front Genet. 2024;15:1402771. doi:10.3389/fgene.2024.1402771
- Biancacci I, De Lorenzi F, Theek B, et al. Monitoring EPR effect dynamics during nanotaxane treatment with theranostic polymeric micelles. Adv Sci. 2022;9(10):e2103745. doi:10.1002/advs.202103745
- Lebreton V, Legeay S, Saulnier P, Lagarce F. Specificity of pharmacokinetic modeling of nanomedicines. Drug Discov Today. 2021;26(10):2259–2268. doi:10.1016/j.drudis.2021.04.017
- Forgan RS. Reproducibility in research into metal-organic frameworks in nanomedicine. Communicat Mater. 2024;5(1). doi:10.1038/s43246-024-00475-7
- Alvarez N, Sevilla A. Current advances in photodynamic therapy (PDT) and the future potential of PDT-combinatorial cancer therapies. Int J Mol Sci. 2024;25(2):1023. doi:10.3390/ijms25021023
- Mishchenko TA, Balalaeva IV, Vedunova MV, Krysko DV. Ferroptosis and photodynamic therapy synergism: enhancing anticancer treatment. Trends Cancer. 2021;7(6):484–487. doi:10.1016/j.trecan.2021.01.013
- Huang Y, Li X, Zhang Z, Xiong L, Wang Y, Wen Y. Photodynamic therapy combined with ferroptosis is a synergistic antitumor therapy strategy. Cancers. 2023;15(20):5043. doi:10.3390/cancers15205043
- Hu Q, Zhu W, Du J, et al. A GPX4-targeted photosensitizer to reverse hypoxia-induced inhibition of ferroptosis for non-small cell lung cancer therapy. Chem Sci. 2023;14(34):9095–9100. doi:10.1039/d3sc01597a
- Zhang Y, Liu X, Zeng L, et al. Exosomal protein angiopoietin-like 4 mediated radioresistance of lung cancer by inhibiting ferroptosis under hypoxic microenvironment. Br J Cancer. 2022;127(10):1760–1772. doi:10.1038/s41416-022-01956-7
- Liu S, Zhao X, Shui S, et al. PDTAC: targeted Photodegradation of GPX4 triggers ferroptosis and potent antitumor immunity. J Med Chem. 2022;65(18):12176–12187. doi:10.1021/acs.jmedchem.2c00855
- Kojima Y, Tanaka M, Sasaki M, et al. Induction of ferroptosis by photodynamic therapy and enhancement of antitumor effect with ferroptosis inducers. J Gastroenterol. 2024;59(2):81–94. doi:10.1007/s00535-023-02054-y
- Tavakkoli Yaraki M, Wu M, Middha E, et al. Gold Nanostars-AIE theranostic nanodots with enhanced fluorescence and photosensitization towards effective image-guided photodynamic therapy. Nano-micro Lett. 2021;13(1):58. doi:10.1007/s40820-020-00583-2
- Zhao X, Zhang J, Zhang W, et al. A chiral fluorescent Ir(iii) complex that targets the GPX4 and ErbB pathways to induce cellular ferroptosis. Chem Sci. 2023;14(5):1114–1122. doi:10.1039/d2sc06171f
- Pan X, Wang H, Wang S, et al. Sonodynamic therapy (SDT): a novel strategy for cancer nanotheranostics. Sci China Life Sci. 2018;61(4):415–426. doi:10.1007/s11427-017-9262-x
- Lai Y, Lu N, Ouyang A, Zhang Q, Zhang P. Ferroptosis promotes sonodynamic therapy: a platinum(ii)-indocyanine sonosensitizer. Chem Sci. 2022;13(34):9921–9926. doi:10.1039/d2sc02597c
- Zhou L, Dong C, Ding L, et al. Targeting ferroptosis synergistically sensitizes apoptotic sonodynamic anti-tumor nanotherapy. Nano Today. 2021;39:101212. doi:10.1016/j.nantod.2021.101212
- Oliver DMA, Reddy PH. Small molecules as therapeutic drugs for Alzheimer’s disease. Mol Cell Neurosci. 2019;96:47–62. doi:10.1016/j.mcn.2019.03.001
- Zhang L, Luo YL, Xiang Y, et al.. Ferroptosis inhibitors: past, present and future. Front Pharmacol. 2024;15. doi:10.3389/fphar.2024.1407335
- Ma W, Hu N, Xu W, Zhao L, Tian C, Kamei KI. Ferroptosis inducers: a new frontier in cancer therapy. Bioorg Chem. 2024;146:107331. doi:10.1016/j.bioorg.2024.107331
- Weng G, Shen C, Cao D, et al. PROTAC-DB: an online database of PROTACs. Nucleic Acids Res. 2021;49(D1):D1381–d1387. doi:10.1093/nar/gkaa807
- Dhas N, Kudarha R, Tiwari R, et al. Recent advancements in nanomaterial-mediated ferroptosis-induced cancer therapy: importance of molecular dynamics and novel strategies. Life Sci. 2024;346:122629. doi:10.1016/j.lfs.2024.122629
- Wang Y, Liu T, Li X, Sheng H, Ma X, Hao L. Ferroptosis-inducing nanomedicine for cancer therapy. Front Pharmacol. 2021;12:735965. doi:10.3389/fphar.2021.735965
- Liu Q, Zhao Y, Zhou H, Chen C. Ferroptosis: challenges and opportunities for nanomaterials in cancer therapy. Regen Biomater. 2023;10:rbad004. doi:10.1093/rb/rbad004
- Jiang J, Lv X, Cheng H, et al. Type I photodynamic antimicrobial therapy: principles, progress, and future perspectives. Acta Biomater. 2024;177:1–19. doi:10.1016/j.actbio.2024.02.005
- Gao W, Wang Z, Lv L, et al. Photodynamic therapy induced enhancement of tumor vasculature permeability using an upconversion nanoconstruct for improved intratumoral nanoparticle delivery in deep tissues. Theranostics. 2016;6(8):1131–1144. doi:10.7150/thno.15262
- Loke YL, Beishenaliev A, Wang PW, et al. ROS-generating alginate-coated gold nanorods as biocompatible nanosonosensitisers for effective sonodynamic therapy of cancer. Ultrason Sonochem. 2023;96:106437. doi:10.1016/j.ultsonch.2023.106437
- Frye WJE, Huff LM, Dalmasy JMG, et al. The multidrug resistance transporter p-glycoprotein confers resistance to ferroptosis inducers. bioRxiv. 2023. doi:10.1101/2023.02.23.529736
- Liang D, Feng Y, Zandkarimi F, et al. Ferroptosis surveillance independent of GPX4 and differentially regulated by sex hormones. Cell. 2023;186(13):2748–2764.e22. doi:10.1016/j.cell.2023.05.003
- Li Y, Ran Q, Duan Q, et al. 7-Dehydrocholesterol dictates ferroptosis sensitivity. Nature. 2024;626(7998):411–418. doi:10.1038/s41586-023-06983-9
- Du Y, Zhou Y, Yan X, et al. APE1 inhibition enhances ferroptotic cell death and contributes to hepatocellular carcinoma therapy. Cell Death Differ. 2024;31:431–446. doi:10.1038/s41418-024-01270-0
- Jacinto E. mTOR takes charge: relaying uncharged tRNA levels by mTOR ubiquitination. Cell Metab. 2023;35(12):2097–2099. doi:10.1016/j.cmet.2023.11.006
- Nguyen LH, Xu Y, Nair M, Bordey A. The mTOR pathway genes mTOR, Rheb, Depdc5, Pten, and Tsc1 have convergent and divergent impacts on cortical neuron development and function. bioRxiv. 2024. doi:10.1101/2023.08.11.553034
- Li S, Ouyang X, Sun H, et al. DEPDC5 protects CD8(+) T cells from ferroptosis by limiting mTORC1-mediated purine catabolism. Cell Discov. 2024;10(1):53. doi:10.1038/s41421-024-00682-z
- Yang M, Luo H, Yi X, Wei X, Jiang DS. The epigenetic regulatory mechanisms of ferroptosis and its implications for biological processes and diseases. MedComm. 2023;4(3):e267. doi:10.1002/mco2.267
- Wang X, Kong X, Feng X, Jiang DS. Effects of DNA, RNA, and Protein Methylation on the Regulation of Ferroptosis. Int J Biol Sci. 2023;19(11):3558–3575. doi:10.7150/ijbs.85454
- Pandur E, Szabó I, Hormay E, et al. Alterations of the expression levels of glucose, inflammation, and iron metabolism related miRNAs and their target genes in the hypothalamus of STZ-induced rat diabetes model. Diabetol Metab Syndr. 2022;14(1):147. doi:10.1186/s13098-022-00919-5
- Murakami H, Hayashi M, Terada S, Ohmichi M. Medroxyprogesterone acetate-resistant endometrial cancer cells are susceptible to ferroptosis inducers. Life Sci. 2023;325:121753. doi:10.1016/j.lfs.2023.121753
- Cai Q, Guo X, Chen Y, Liu J, Liu Y. Selenium-Doped 3D porous molybdenum carbide nanospheres improve mitochondrial function by reducing oxidative stress in alzheimer’s disease. ACS Appl Nano Mater. 2023;6(23):22496–22505. doi:10.1021/acsanm.3c05296
- Xiao Y, Yu Y, Hu L, et al. Matrine alleviates sepsis-induced myocardial injury by inhibiting ferroptosis and apoptosis. Inflammation. 2023;46(5):1684–1696. doi:10.1007/s10753-023-01833-2
- Sun L, Wang H, Xu D, Yu S, Zhang L, Li X. Lapatinib induces mitochondrial dysfunction to enhance oxidative stress and ferroptosis in doxorubicin-induced cardiomyocytes via inhibition of PI3K/AKT signaling pathway. Bioengineered. 2022;13(1):48–60. doi:10.1080/21655979.2021.2004980
- Lei G, Mao C, Yan Y, Zhuang L, Gan B. Ferroptosis, radiotherapy, and combination therapeutic strategies. Protein Cell. 2021;12(11):836–857. doi:10.1007/s13238-021-00841-y
- Nie A, Shen C, Zhou Z, Wang J, Sun B, Zhu C. Ferroptosis: potential opportunities for natural products in cancer therapy. Phytother Res. 2024;38(3):1173–1190. doi:10.1002/ptr.8088
- Zhang W, Cui X, Li R, Ji W, Shi H, Cui J. Association between ICW/TBW ratio and cancer prognosis: subanalysis of a population-based retrospective multicenter study. Clin Nutr. 2024;43(2):322–331. doi:10.1016/j.clnu.2023.12.004
- Alcantara M, Tesio M, June CH, Houot R. CAR T-cells for T-cell malignancies: challenges in distinguishing between therapeutic, normal, and neoplastic T-cells. Leukemia. 2018;32(11):2307–2315. doi:10.1038/s41375-018-0285-8
- Wang T, Pulkkinen OI, Aittokallio T. Target-specific compound selectivity for multi-target drug discovery and repurposing. Front Pharmacol. 2022;13:1003480. doi:10.3389/fphar.2022.1003480
- Huang Y, Wang J, Wang S, et al. Discrimination of active and inactive substances in cytotoxicity based on Tox21 10K compound library: structure alert and mode of action. Toxicology. 2021;462:152948. doi:10.1016/j.tox.2021.152948
- Gooch A, Sizochenko N, Rasulev B, Gorb L, Leszczynski J. In vivo toxicity of nitroaromatics: a comprehensive quantitative structure-activity relationship study. Environ Toxicol Chem. 2017;36(8):2227–2233. doi:10.1002/etc.3761
- Huang M, Teng Q, Cao F, Huang J, Pang J. Ferroptosis and ferroptosis-inducing nanomedicine as a promising weapon in combination therapy of prostate cancer. Biomater Sci. 2024;12:1617–1629. doi:10.1039/d3bm01894f
- Liu X, Lu Y, Li X, Luo L, You J. Nanoplatform-enhanced photodynamic therapy for the induction of immunogenic cell death. J Control Release. 2024;365:1058–1073. doi:10.1016/j.jconrel.2023.11.058
- Deng W, Shang H, Tong Y, et al. The application of nanoparticles-based ferroptosis, pyroptosis and autophagy in cancer immunotherapy. J Nanobiotechnology. 2024;22(1):97. doi:10.1186/s12951-024-02297-8