Abstract
Introduction
Diabetic nephropathy (DN) is one of the major complications of diabetes and podocyte injury plays an important role in the DN pathogenesis. MicroRNA (miR)-106a is predicated to be a target of long noncoding RNA (lncRNA) SNHG16 and has been identified as a therapeutic biomarker for diabetic kidney diseases. However, the role of SNHG16/miR-106a axis in DN has not been illustrated. This study aimed to investigate whether SNHG16 could regulate podocyte injury via miR-106a in DN and uncover the underlying mechanism.
Methods
MPC5 podocytes were treated with control or high glucose (HG) medium, and then miR-106a level was measured. MPC5 cells that exposed to HG were overexpressed with miR-106a or not, following by overexpression with or without KLF9 or SNHG16. Then, cell viability, apoptosis, reactive oxygen species and the protein expression of synaptopodin and podocin were evaluated.
Results
MiR-106a was down-regulated in the serum of DN patients and HG-induced MPC5 podocytes. Overexpression of miR-106a suppressed HG-induced decrease in cell viability, Bcl-2, synaptopodin and podocin expression, increase in ROS, apoptotic cells, Bax and cleaved-caspase 3 expression. MiR-106a could bind to both KLF9 and lncRNA SNHG16, which were up-regulated in the serum of DN patients and HG-induced MPC5 podocytes. The level of miR-106a was decreased by SNHG16 overexpression and miR-106a overexpression reduced KLF9 expression. Furthermore, overexpression of KLF9 or SNHG16 blunted the protective effects of miR-106a on HG-induced MPC5 injury.
Discussion
LncRNA SNHG16 could promote HG-stimulated podocytes injury via targeting miR-106a to enhance KLF9 expression. The intervention of SNHG16/miR-106a/KLF9 may be a therapeutic treatment for DN.
Introduction
Diabetic nephropathy (DN) is one of the chronic microvascular complications of diabetes. The impaired renal function caused by diabetes can also accelerate microvascular complications such as cardiovascular disease and stroke. Early DN is characterized by micro albuminuria, which can gradually progress to a large amount of albuminuria, and elevated serum creatinine and urea nitrogen levels, eventually leading to renal failure.Citation1 Clinically, effective interventions can prevent or delay the progression of DN. However, it has been reported that the current standard therapy only achieves partial renoprotection, which greatly increases the need for novel and effective therapeutic approaches.Citation2
The decreased number and the destructed structure or function of podocytes, which is one of the components of the glomerular filtration barrier, are strongly related to the production of proteinuria.Citation3 High glucose (HG) can promote reactive oxygen species (ROS) release and apoptosis of podocytes, leading to podocytes injury and aggravation of DN.Citation4 MicroRNAs (miRNAs) are small non-coding RNAs that function as post-transcriptional mediator of gene expression and are associated with various cellular processes including angiogenesis, differentiation, proliferation, and apoptosis. Furthermore, a large number of studies have demonstrated that miRNAs targeted against genes involved in the pathogenesis of DN.Citation5 MiRNAs can participate in the processes of apoptosis, fibrosis and extracellular matrix accumulation in the progression of DN through various signaling pathways, and at the same time protect or promote podocyte damage.Citation6 For example, miR-29c was up-regulated in HG-treated podocytes and was positively correlated with increased inflammatory cytokines.Citation7 On the contrary, miR-29a could ameliorate hyperglycemia-induced podocytes dysregulationCitation7 and miR-34c could inhibit HG-induced podocytes apoptosis.Citation8 Recently, data from bioinformatics analysis identified miR-106a as a therapeutic biomarker for diabetic kidney disease (DKD).Citation9 Yuanbo Wu et al found that in diabetic peripheral neuropathy (DPN), miR-106a level was decreased, and miR-106a overexpression reversed the oxidative/nitrifying stress induced by HG.Citation10 The overexpression of miR-106a significantly reduced the expression of HIF1α and VEGF, and prevented the increase of permeability induced by HG.Citation11 MiR-106a expression was also significantly down-regulated in the serum of diabetic mice.Citation12 These findings implicated that miR-106a may also be decreased and play an important role in DN.
Long noncoding RNA (lncRNA) SNHG16 is predicated to bind to miR-106a using starbase database (http://starbase.sysu.edu.cn/) for searching the targeted lncRNA of hsa-miR-106a-5p, and SNHG16 has been reported to regulate the proliferation and oxidative stress of various cells. LncRNAs have lengths >200 nt and can regulate the expression of the target gene, and many lncRNAs have been suggested to participate in the pathogenesis of DN, such as plasmacytoma variant translocation 1 (PVT1),Citation13 metastasis-associated lung adenocarcinoma transcript 1(MALAT1),Citation14 taurine-upregulated gene 1 (Tug1),Citation15 and Erbb4-IR.Citation16 Therefore, we speculated that SNHG16 may target miR-106a to involve in the progression of DN.
The Krüppel-like factor 9 (KLF9), a differentiation-associated transcription factor, participates in a broad range of processes such as cell differentiation, proliferation, and apoptosis. The up-regulation of KLF9 was also identified as a biomarker for DKDCitation9. However, its function in DKD remains to be clarified. Previous studies reported that KLF9 could aggravate oxidative stress and apoptosis, leading to stress-induced cell death.Citation17 KLF9 is predicted to be the target gene of miR-106a using starbase database (http://starbase.sysu.edu.cn/) for searching the targeted mRNA of has-miR-106a-5p. Therefore, miR-106a may target KLF9 to regulate the oxidative stress and apoptosis of podocytes and affect the progression of DN.
In the present study, we aimed to explore whether the SNHG16/miR-106a/KLF9 axis could participate in the HG-induced podocytes injury and uncover the potential mechanisms.
Materials and Methods
Cell Culture and Treatment
MPC5, a murine podocyte cell line was purchased from Cell Bank of the Chinese Academic of Sciences (Shanghai, China) was cultured in Dulbecco’s Modified Eagle’s Medium (DMEM, Gibco, USA) supplemented with 10% FBS (Gibco) in a humidified incubator at 37°C with 5% CO2. Cells were passaged at 33°C and treated with interferon (10 U IFN-γ/mL). Then, cells were harvested and incubated without IFN-γ at 37°C for two weeks to allow their differentiation.
For NG group, cells were maintained in normal glucose medium (5mM D-glucose) for 24h. Cells that exposed to HG or MA treatment were incubated in medium added high glucose (HG, 30 mM) or mannitol (MA, 30 mM) for 24 h.
In order to carry out miR-106a, KLF9 and SNHG16 overexpression, miR-106a mimic, plasmid pcDNA-KLF9 or pcDNA-SNHG16 were designed and constructed by GenePharma (Shanghai, China). Corresponding empty vectors were used as negative control (NC). All plasmids were transfected into MPC5 cells using Lipofectamine 2000TM reagent (Invitrogen, USA) according to the manufacturer’s instructions.
Collection of Serum
The serum of a total of 30 normal individuals and 30 DN patients was collected from the clinical laboratory center of the First Affiliated Hospital of Jinan University and approved by the Medical Ethics Committee of the First Affiliated Hospital of Jinan University.
Cell Viability Assessment
MPC5 podocytes that overexpressed with miR-106a, KLF9 and SNHG16 or not were seeded in 96-well plate with density of 2×104 cells per well, then subsequently maintained in Control or HG medium for 24 h. Thereafter, 10 μL cell counting kit-8 (CCK-8, Beyotime Biotechnology Co., LTD, China) solution was added to each well and incubated at 37°C for 2 h, and OD values were measured at 450 nm through a microplate reader (Bio-Rad Model 550).
TUNEL Staining
The apoptotic MCP5 cells were detected using in situ cell death detection kits TUNEL (KeyGen, Biotechnology, Co., LTD, China) according to the manufacturer’s protocol. Briefly, cells seeded on the slides were fixed with 4% paraformaldehyde at room temperature for 0.5 h. After treatment with 1% Triton X-100/10mM PBS for 5 minutes, proteinase K was added. Then, cells were incubated with TdT enzyme reaction solution, streptavidin-HRP working solution and DAB staining working solution in the dark, respectively. Hematoxylin was used to stain the nucleus.
RT-qPCR
The RNA expression level of miR-106a, KLF9 and SNHG16 was assessed using quantitative reverse-transcriptase-PCR (RT-qPCR). Total RNA of MCP5 was extracted from using Trizol reagent (Invitrogen, CA, USA). The cDNA Reverse Transcription Kit (Invitrogen, CA, USA) was used for reverse transcription. The SYBRTM Green PCR Master Mix (Applied Biosystems, CA, USA) was used for qPCR amplification on StepOne Plus real-time PCR system (Thermo Fisher, MA, USA). All qPCRs were carried out in triplicate for each sample and β-actin was used as endogenous control. Primers are as follows: miR-106a, forward 5ʹ-AAAAGUGCUUACAGUGCAGGUA-G-3ʹ, reverse, 5ʹ- ACCUGCACUGUAAGCACUUUUUU-3ʹ. KLF9: forward 5ʹ-TACATGGACTTCGTGGCTGC-3ʹ, reverse 5ʹ-AGGGCCGTTCACCTGTATGC-3ʹ. SNHG16: forward 5ʹ-GGACCCAAAGTGCCATGTCT-3ʹ, reverse 5ʹ-GATGAAGCC-CAAAGAACGCA-3ʹ. β-actin: forward 5ʹ-CATCTCACCTGAAGCACCCT-3ʹ, reverse 5ʹ-CGGAGTCCATCACAATGCCT-3ʹ.
Western Blotting
MCP5 podocytes were lysed in RIPA lysis buffer (Beyotime), supplemented with protease inhibitor mixture (Beyotime). The protein samples were separated by SDS-PAGE, followed by transferring to PVDF membranes (Millipore, USA) and blocked with 5% non-fat milk at room temperature for 2 h. The membranes were incubated overnight with primary antibodies at 4°C then incubated with secondary antibodies at room temperature for 2 h. The primary antibodies used for Western blotting were as follows: KLF9 (Abcam, 1:1000), Bcl-2 (CST, 1:1000), Bax (CST, 1:1000), (cleaved) caspase 3 (Abcam, 1:1000), synaptopodin (Abcam, 1:1000), podocin (Abcam, 1:1000) and GAPDH (Abcam, 1:1000). After washing with TBST, the membrane was incubated with appropriate secondary antibodies (Abcam, 1:10,000). Blots were visualized by the enhanced chemiluminescence system (Santa Cruz Biotechnology Inc., CA, USA).
Measurement of Reactive Oxygen Species (ROS)
Intracellular ROS generation in MPC5 cells was assayed using ROS kit (Abcam) according to the manufacturer’s protocol. Briefly, cells were plated in a 96-well plate and cultured with their respective treatments for 24 h. Followed by staining with DCFDA for 45 min. The absorbance was read using microplate reader (SpectraMax 340; Molecular Devices Co., USA) at a wavelength of 450 nm. The ROS level was determined by DCF fluorescence intensity.
Dual-Luciferase Assay
The target genes of miR-106a were analyzed and predicted by the biological prediction website starbase. To verify the interaction between miR-106a and KLF9 or SNHG16, the KLF9 and SNHG16 3ʹ-UTR or mutant was cloned into the luciferase reporter gene pmirGLO (Promega, USA), and the carrier was named KLF9-wild type (WT), KLF9 mutant (MUT), SNHG16 WT or MUT. WT and MUT reporter plasmids were transfected into MCP5 cells accompanied with pRL-TK plasmid (Promega), respectively. Meanwhile, miR-106a mimic or control (NC) was co-transfected with those reporter plasmids. At 48h post-transfection, luciferase activity was detected using Dual-Luciferase Reporter Assay System (Promega) and normalized by measuring renilla luciferase activity.
Statistical Analysis
All experiments were performed three times independently. The data were considered statistically significant if P < 0.05 and were expressed as mean ± SD. Statistical significance of differences was determined by student’s two-tailed t-test or one-way analysis of variance using SPSS 22.0 software.
Results
miR-106a is Down-Regulated in DN and HG-Induced Podocytes
To confirm whether miR-106a could play a role in DN, we detected the level of miR-106a in the serum of normal or DN patients. Results showed that miR-106a was significantly down-regulated in DN patients compared with normal individuals (). Subsequently, MPC5 cells were exposed to a series concentration of glucose (5, 10, 15, 20, 25 and 30 mM) and 30 mM MA for 24h, then miR-106a level was detected. Results showed that D-glucose with the concentration of 20–30 mM decreased the miR-106a level in a concentration-dependent manner (). At the same time, 30 mM glucose (HG) reduced miR-106a in a time-dependent manner (). These results suggested the potential role of miR-106a in the pathogenesis of DN.
Figure 1 MiR-106a was down-regulated upon HG stimulation. (A) The level of miR-106a in the serum of DN patients relative to that of normal healthy individuals (n=30). ***P <0.001 vs normal. (B) The level of miR-106a in MPC5 podocytes that exposed to different concentrations of D-glucose (5, 10, 15, 20, 25 and 30 mM) or 30 mM MA relative to that of control cells (n=3). **P<0.01, ***P<0.001 vs 0 (control) group. ##P<0.01, ###P<0.001 vs MA group. (C) The level of miR-106a in MPC5 podocytes that exposed to 30 mM D-glucose for different times (6, 12, 24 and 48 h) relative to that of control cells (n=3). *P<0.05, ***P<0.001 vs 0 (control) group.
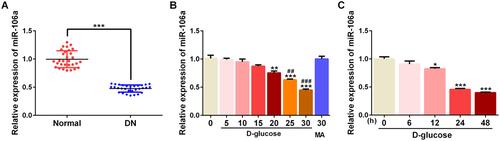
Overexpression of miR-106a Protects Podocytes Against HG-Induced Injury
Thereafter, we overexpressed miR-106a via transfecting HG-induced MPC5 cells with miR-106a mimic, and the efficiency of overexpression was verified by RT-PCR (). Then, we found that the HG-induced decrease in cell viability () while increase in ROS generation () was remarkably reversed by miR-106 overexpression. HG also induced cell apoptosis, which was blunted by miR-106 overexpression ( and ). The same result is observed in , miR-106 mimic considerably recovered the balance between anti-apoptotic protein Bcl-2 and pro-apoptotic proteins Bax and cleaved-caspase3, which was disturbed by HG stimulation (). Besides, miR-106a mimic also restored the expression of podocytes markers synaptopodin and podocin (). These findings demonstrated that miR-106a may play a protective role in HG-induced podocytes injury.
Figure 2 The effect of miR-106a mimic on HG-induced podocytes injury. (A) The mRNA level of miR-106a relative to control (n=3). ***P<0.001 vs control group. ###P<0.001 vs HG + miR-NC. (B) The cell viability of MPC5 in different groups (n=3). **P<0.01 vs control group. #P<0.05 vs HG + miR-NC. (C) The ROS level in MPC5 relative to control group (n=3). ***P<0.001 vs control group. ###P<0.001 vs HG + miR-NC. (D and E) Representative images (D) and quantitative analysis (E) for TUNEL staining, apoptotic cells were stained with dark brown (×200). ***P<0.001 vs control group. ##P<0.01 vs HG + miR-NC. (F) Representative blots and quantitative analysis for Bcl-2, Bax, caspase 3, synaptopodin and podocin (n=3). ***P<0.001 vs control group. #P<0.05, ##P<0.01, ###P<0.001 vs HG + miR-NC.
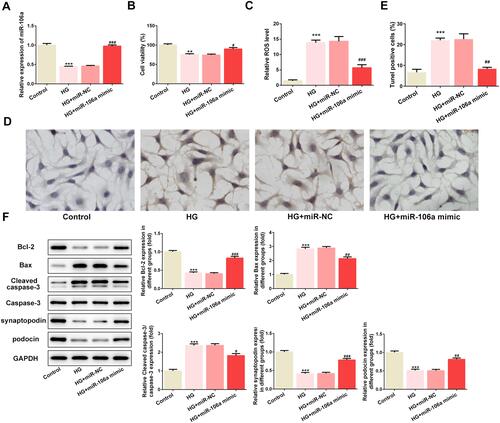
KLF9 Can Bind to miR-106a and is Up-Regulated in DN and HG-Induced Podocytes
KLF9 was predicated to be a target of miR-106a and dual-luciferase assay confirmed their interaction ( and ). Meanwhile, the presence of miR-106a mimic considerably reduced KLF9 mRNA and protein expression ( and ). Additionally, KLF9 was up-regulated in the serum of DN patients () and MPC5 cells that treated with HG ( and ). The above findings suggested that KLF9 may function as a downstream target of miR-106a to play a role in HG-induced podocytes injury or DN.
Figure 3 The relationship between miR-106a and KLF9. (A) The binding site between has-miR-106a-5p and KLF9. (B) The luciferase activity between miR-NC or miR-106a and KLF WT or MUT (n=3). **P <0.01 vs miR-NC. (C) The mRNA level of KLF9 in MPC podocytes (n=3). ***P<0.001 vs control. (D) The protein level of KLF9 in MPC podocytes (n=3). **P<0.01 vs control. (E) The level of KLF9 in the serum of DN patients relative to that of normal healthy individuals (n=30). ***P <0.001 vs normal. (F and G) The mRNA level (F) and protein level (G) of KLF9 in MPC5 podocytes that treated with 30 mM glucose (HG) or 30 mM MA relative to control cells (n=3). ***P<0.001 vs control. ###P<0.001 vs HG.
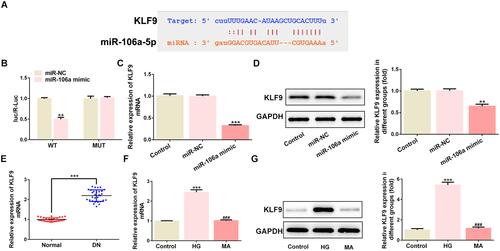
Overexpression of KLF9 Significantly Weakens the Protective Effect of miR-106a on HG-Induced Podocytes Injury
Subsequently, we overexpressed KLF9 in MPC5 cells and the transfection efficacy is demonstrated in . Results showed that compared with cells that overexpressed with miR-106a, cells that overexpressed with miR-106a and KLF9 exerted a lower cell viability (), a higher production of ROS () and a higher ratio of TUNEL-positive cells ( and ). The same results are observed in , KLF9 resulted in a decreased protein expression in Bcl-2, synaptopodin and podocin, but an increased protein expression in Bax and cleaved-caspase3, when compared to HG + miR-106a mimic + pcDNA-NC group. These results illustrated that KLF9 overexpression could blunt the protective effect of miR-106a on HG-induced podocytes.
Figure 4 KLF9 overexpression weakens the effects of miR-106a mimic on HG-induced MPC5 podocytes. (A) Representative blots and quantitative analysis for KLF9 (n=3). ***P<0.001 vs control group. (B) The cell viability of MPC5 in different groups (n=3). ***P<0.001 vs control group. #P<0.05 vs HG + miR-NC. ΔP<0.05 vs HG + miR-106 mimic + pcDNA-NC. (C) The ROS level in MPC5 relative to control group (n=3). ***P<0.001 vs control group. ###P<0.001 vs HG + miR-NC. ΔΔΔP<0.001 vs HG + miR-106 mimic + pcDNA-NC. (D and E) Representative images (D) and quantitative analysis (E) for TUNEL staining, apoptotic cells were stained with dark brown (×200). ***P<0.001 vs control group. ###P<0.001 vs HG + miR-NC. ΔΔΔP<0.001 vs HG + miR-106 mimic + pcDNA-NC. (F) Representative blots and quantitative analysis for Bcl-2, Bax, caspase 3, synaptopodin and podocin (n=3). ***P<0.001 vs control group. ###P<0.001 vs HG + miR-NC. ΔP<0.05, ΔΔP<0.01, ΔΔΔP<0.001 vs HG + miR-106 mimic + pcDNA-NC.
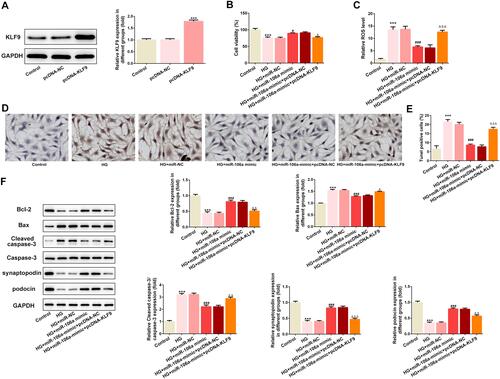
SNHG16 Can Bind to miR-106a and Overexpression of It Also Blunts the Effects of miR-106a on HG-Treated Podocytes
LncRNA SNHG16 was also predicted to bind to miR-106a (), and dual-luciferase assay determined their interaction (). and demonstrated that SNHG16 was significantly up-regulated in DN patients and HG-induced podocytes, suggesting that SNHG16 may also be involved in the pathogenesis of DN via binding to miR-106a. We then overexpressed SNHG16 in MPC5 cells () and found that miR-106a was down-regulated upon SNHG16 overexpression (). Furthermore, consistent with KLF9 overexpression, the elevated expression of SNHG16 also reversed the effects of miR-106a mimic on cell viability (), ROS production (), cell apoptosis ( and ) and proteins expression of apoptosis-related proteins as well as podocytes markers ( and ).
Figure 5 The relationship between miR-106a and SNHG16. (A) The binding site between has-miR-106a-5p and SNHG16. (B) The luciferase activity between miR-NC or miR-106a and SNHG16 WT or MUT (n=3). **P <0.01 vs miR-NC. (C) The level of KLF9 in the serum of DN patients relative to that of normal healthy individuals (n=30). ***P <0.001 vs normal. (D) The mRNA level of KLF9 in MPC5 podocytes that treated with 30 mM glucose (HG) or 30 mM MA relative to control cells (n=3). ***P<0.001 vs control. ###P<0.001 vs HG. (E) The mRNA level of SNHG16 in MPC5 that overexpressed with SNHG16 or not (n=3). **P<0.01 vs pcDNA-NC. (F) The mRNA level miR-106a in MPC5 that overexpressed with SNHG16 or not (n=3). ***P<0.001 vs pcDNA-NC.
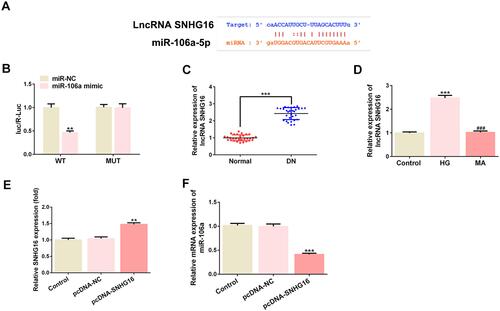
Figure 6 SNHG16 overexpression blunts the effects of miR-106a mimic on HG-induced MPC5 podocytes. (A) The cell viability of MPC5 in different groups (n=3). **P<0.01 vs control group. #P<0.05 vs HG + miR-NC. ΔP<0.05 vs HG + miR-106 mimic + pcDNA-NC. (B) The ROS level in MPC5 relative to control group (n=3). ***P<0.001 vs control group. ###P<0.001 vs HG + miR-NC. ΔΔΔP<0.001 vs HG + miR-106 mimic + pcDNA-NC. (C and D) Representative images (C) and quantitative analysis (D) for TUNEL staining, apoptotic cells were stained with dark brown (×200). ***P<0.001 vs control group. ###P<0.001 vs HG + miR-NC. ΔΔP<0.01 vs HG + miR-106 mimic + pcDNA-NC. (E and F) Representative blots and quantitative analysis for Bcl-2, Bax, caspase 3, synaptopodin and podocin (n=3). ***P<0.001 vs control group. ###P<0.001 vs HG + miR-NC. ΔΔP<0.01, ΔΔΔP<0.001 vs HG + miR-106 mimic + pcDNA-NC.
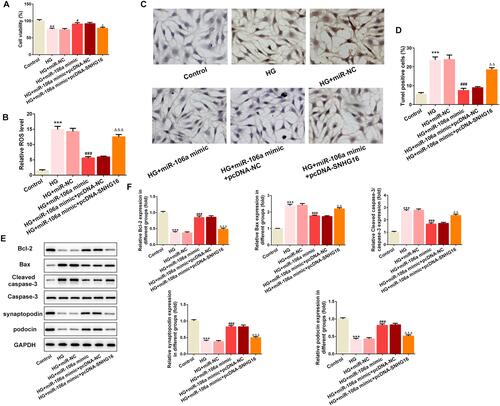
Discussion
Podocyte, the epithelial cell of the glomerulus, is one of the crucial components of the glomerular filtration barrier. Previous studies have confirmed that HG-induced podocyte injury is a major cause in the progression of DN, which is an important target for clinical treatment.Citation18 Consistent with the discoveries in previous studies, our data showed that HG stimulation reduced cell viability, increased ROS production, induced cell apoptosis and disrupted the expression of synaptopodin and podocin in podocytes.
Consistent with a previous finding that miR-106a expression was significantly down-regulated in the serum of diabetic mice,Citation12 we found that miR-106a expression was significantly reduced in the serum of DN patients and HG-induced podocytes. Meanwhile, miR-106a overexpression enhanced cell viability while inhibited ROS production and cell apoptosis. Oxidative stress is a common pathogenesis of diabetes and its complications including DN. Under the stimulation of HG, mitochondria releases a large amount of ROS, and excessive ROS can break the balance between the oxidation system and antioxidant system thus leading to cells damage or podocyte apoptosis. Synaptopodin and podocin are the crucial structural proteins of podocyte, the aberrant expression of which will result in structural and functional impairment of podocytes, which in turn cause renal dysfunction. The results from our study indicated that overexpression of miR-106a played a protective role against HG-induced podocytes injury.
Emerging evidences showed that aberrant expression of miR-106a is frequently observed in a variety of tumors.Citation19–Citation22 Liming Hong et al found that miR-106a was down-regulated in serum and granulosa cells of women with diminished ovarian reserve (DOR). Besides, miR-106a mimic increased cell viability and attenuated apoptosis of human granulosa cells. MiR-106a was also indicated to suppress tumor cells death in colorectal cancer.Citation21 However, enforced expression of miR-106a in gastric cancer could promote tumor metastasis.Citation23 Although the effects of miR-106a on cancers were controversial, our findings were in accordance with previous reports, in which miR-106a was identified as a therapeutic biomarker for DKD and showed beneficial effect in HG-induced injury.Citation9–Citation11 Our study for the first time demonstrated the protective effect of miR-106a on HG-induced podocytes injury.
According to a previous study, lncRNAs, mRNAs and other RNAs act as natural miRNA sponges to restrain intracellular miRNA function. For example, in breast cancer, lncRNA HOXA-AS2 could regulate the expression of SCN3A by sponging miR-106a.Citation24 In addition, miR-106a inhibited the cell proliferation and epithelial–mesenchymal transition of oral squamous cell carcinoma cells by directly decreasing LIM kinase 1 expression.Citation25 In the present study, via online database (http://starbase.sysu.edu.cn/agoClipRNA.php?source=circRNA) we predicted that KLF9 and lncRNA SNHG16 could bind to miR-106a. Consistent with our predictions, the data from dual-luciferase verified the direct binding between miR-106a and KLF9 together with miR-106a and SNHG16. Moreover, we found that miR-106a overexpression could inhibit KLF9 level, while SNHG16 overexpression could reduce miR-106a level. Importantly, in contrary to miR-106a, KLF9 and SNHG16 were remarkably up-regulated in serum of DN patients and HG-induced podocytes. Therefore, we inferred that lncRNA SNHG16 may function as a competing endogenous RNA (ceRNA) of miR-106a to down-regulate miR-106a, which could target KLF9 and inhibit its expression, thereby enhancing KLF9 expression. KLF9 represses multiple anti-oxidant defense genes including mitochondrial thioredoxin reductase (TXNRD2) leading to increased ROS levels. It has been publicly acknowledgement that KLF9 regulates the progression of various diseases via increasing ROS generation. For example, KLF9 was identified as an important mediator in premalignant melanocytes in vitro and in mouse skin via promoting ROS generation.Citation26 In pancreatic cancer, KLF9 could induce cell apoptosis.Citation27 In medullary thyroid cancer, the induction of KLF9 could promote oxidative stress-induced apoptosis.Citation28 KLF9 also aggravated ischemic injury in cardiomyocytes through augmenting oxidative stress.Citation17 In the present study, we found that the enforced expression of KLF9 reduced cell viability, increased ROS production, promoted cell apoptosis and reduced synaptopodin and podocin expression in HG-treated podocytes that overexpressed with miR-106a, when compared with cells that overexpressed with miR-106a and transfected with pcDNA-NC. These results indicated that the overexpression of KLF9 could remarkably weaken the protective effect of miR-106a on HG-induced podocytes injury. The same results were observed in cells that overexpressed with SNHG16, which showed that SNHG16 overexpression reversed the effects of miR-106a mimic on cell viability, ROS level and cell apoptosis, together with synaptopodin and podocin expression. These findings exactly further confirmed our speculation that miR-106a acted as a down-stream ceRNA of lncRNA SNHG16 to target KLF9, which could promote ROS production and apoptosis, thereby play an important role in HG-induced podocytes injury.
Conclusion
Taken together, in the current study, we for the first time identified miR-106a as a protective mediator and SNHG16 and KLF9 as detrimental mediators in HG-induced podocytes injury or even DN as well as uncovered the potential mechanism. Therefore, approaches that target SNHG16/miR-106a/KLF9 axis may provide a potential therapy for treating DN.
Ethics Approval
The study was approved by the Medical Ethics Committee of the First Affiliated Hospital of Jinan University.
Disclosure
The authors report no conflicts of interest in this work.
References
- Flyvbjerg A. The role of the complement system in diabetic nephropathy. Nat Rev Nephrol. 2017;13(5):311–318. doi:10.1038/nrneph.2017.31
- Bhattacharjee N, Barma S, Konwar N, Dewanjee S, Manna P. Mechanistic insight of diabetic nephropathy and its pharmacotherapeutic targets: an update. Eur J Pharmacol. 2016;791:8–24. doi:10.1016/j.ejphar.2016.08.022
- Tagawa A, Yasuda M, Kume S, et al. Impaired podocyte autophagy exacerbates proteinuria in diabetic nephropathy. Diabetes. 2016;65(3):755–767. doi:10.2337/db15-0473
- Han Y, Xu X, Tang C, et al. Reactive oxygen species promote tubular injury in diabetic nephropathy: the role of the mitochondrial ros-txnip-nlrp3 biological axis. Redox Biol. 2018;16:32–46. doi:10.1016/j.redox.2018.02.013
- Gholaminejad A, Abdul TH, Gholami FM. Identification of candidate microRNA biomarkers in diabetic nephropathy: a meta-analysis of profiling studies. J Nephrol. 2018;31:813–831.
- Dewanjee S, Bhattacharjee N. MicroRNA: a new generation therapeutic target in diabetic nephropathy. Biochem Pharmacol. 2018;155:32–47. doi:10.1016/j.bcp.2018.06.017
- Long J, Wang Y, Wang W, Chang BH, Danesh FR. MicroRNA-29c is a signature microRNA under high glucose conditions that targets sprouty homolog 1, and its in vivo knockdown prevents progression of diabetic nephropathy. J Biol Chem. 2011;286(13):11837–11848. doi:10.1074/jbc.M110.194969
- Liu XD, Zhang LY, Zhu TC, Zhang RF, Wang SL, Bao Y. Overexpression of miR-34c inhibits high glucose-induced apoptosis in podocytes by targeting Notch signaling pathways. Int J Clin Exp Pathol. 2015;8:4525–4534.
- Cui C, Cui Y, Fu Y, Ma S, Zhang S. Microarray analysis reveals gene and microRNA signatures in diabetic kidney disease. Mol Med Rep. 2018;17:2161–2168.
- Wu Y, Xu D, Zhu X, Yang G, Ren M. MiR-106a associated with diabetic peripheral neuropathy through the regulation of 12/15-LOX-mediated oxidative/nitrative stress. Curr Neurovasc Res. 2017;14(2):117–124. doi:10.2174/1567202614666170404115912
- Ling S, Birnbaum Y, Nanhwan MK, Thomas B, Bajaj M, Ye Y. MicroRNA-dependent cross-talk between VEGF and HIF1α in the diabetic retina. Cell Signal. 2013;25(12):2840–2847. doi:10.1016/j.cellsig.2013.08.039
- Platania CBM, Maisto R, Trotta MC, et al. Retinal and circulating miRNA expression patterns in diabetic retinopathy: an in silico and in vivo approach. Br J Pharmacol. 2019;176:2179–2194.
- Liu DW, Zhang JH, Liu FX, et al. Silencing of long noncoding RNA PVT1 inhibits podocyte damage and apoptosis in diabetic nephropathy by upregulating FOXA1. Exp Mol Med. 2019;51:1–15.
- Hu M, Wang R, Li X, et al. LncRNA MALAT1 is dysregulated in diabetic nephropathy and involved in high glucose-induced podocyte injury via its interplay with β-catenin. J Cell Mol Med. 2017;21(11):2732–2747. doi:10.1111/jcmm.13189
- Long J, Badal SS, Ye Z, et al. Long noncoding RNA Tug1 regulates mitochondrial bioenergetics in diabetic nephropathy. J Clin Invest. 2016;126(11):4205–4218. doi:10.1172/JCI87927
- Sun SF, Tang PMK, Feng M, et al. Novel lncRNA erbb4-IR promotes diabetic kidney injury in db/db mice by targeting miR-29b. Diabetes. 2018;67(4):731–744. doi:10.2337/db17-0816
- Yan Q, He B, Hao G, et al. KLF9 aggravates ischemic injury in cardiomyocytes through augmenting oxidative stress. Life Sci. 2019;233:116641. doi:10.1016/j.lfs.2019.116641
- Zhong F, Chen H, Xie Y, et al. Protein S protects against podocyte injury in diabetic nephropathy. J Am Soc Nephrol. 2018;29(5):1397–1410. doi:10.1681/ASN.2017030234
- Zhang N, Wei ZL, Yin J, et al. MiR-106a* inhibits oral squamous cell carcinoma progression by directly targeting MeCP2 and suppressing the Wnt/β-Catenin signaling pathway. Am J Transl Res. 2018;10:3542–3554.
- Pan YJ, Zhuang Y, Zheng JN, Pei DS. MiR-106a: promising biomarker for cancer. Bioorg Med Chem Lett. 2016;26(22):5373–5377. doi:10.1016/j.bmcl.2016.10.042
- Hao H, Xia G, Wang C, Zhong F, Liu L, Zhang D. MiR-106a suppresses tumor cells death in colorectal cancer through targeting ATG7. Med Mol Morphol. 2017;50:76–85.
- Pan YJ, Wei LL, Wu XJ, Huo FC, Mou J, Pei DS. MiR-106a-5p inhibits the cell migration and invasion of renal cell carcinoma through targeting PAK5. Cell Death Dis. 2017;8(10):e3155. doi:10.1038/cddis.2017.561
- Zhang HD, Jiang LH, Sun DW, Li J, Tang JH: MiR-139-5p: promising biomarker for cancer. Tumour Biol. 2015;36(3):1355–1365. doi:10.1007/s13277-015-3199-3
- Wu J, Li M, Zhang Y. Long noncoding RNA HOXA-AS2 regulates the expression of SCN3A by sponging miR-106a in breast cancer. J Cell Biochem. 2019;120(9):14465–14475. doi:10.1002/jcb.28706
- Shi B, Ma C, Liu G, Guo Y. MiR-106a directly targets LIMK1 to inhibit proliferation and EMT of oral carcinoma cells. Cell Mol Biol Lett. 2019;24(1):1. doi:10.1186/s11658-018-0127-8
- Bagati A, Moparthy S, Fink EE, et al. KLF9-dependent ROS regulate melanoma progression in stage-specific manner. Oncogene. 2019;38(19):3585–3597. doi:10.1038/s41388-019-0689-6
- Zhong Z, Zhou F, Wang D, et al. Expression of KLF9 in pancreatic cancer and its effects on the invasion, migration, apoptosis, cell cycle distribution, and proliferation of pancreatic cancer cell lines. Oncol Rep. 2018;40:3852–3860.
- Bagheri-Yarmand R, Sinha KM, Li L, et al. Combinations of tyrosine kinase inhibitor and ERAD inhibitor promote oxidative stress-induced apoptosis through ATF4 and KLF9 in medullary thyroid cancer. Mol Cancer Res. 2019;17(3):751–760. doi:10.1158/1541-7786.MCR-18-0354