Abstract
Purpose
The aim of the present study was to investigate the hypoglycemic activity and potential mechanism of tetrahydrocarbazole derivatives ZG02 in high-fat diet/streptozotocin-induced type 2 diabetes model.
Methods
C57BL/6 mice (n=30) were randomly assigned to three groups: control group (n=10) was fed with normal diet, the diabetes group (n=10) was fed with high-fat diet for eight weeks followed by intraperitoneal injection of streptozotocin (25 mg/kg) and the ZG02 group (n=10) injected intraperitoneally with ZG02 (30 mg/kg/day) for two weeks after successful modeling. The changes of weight, fasting blood glucose, oral glucose tolerance and fasting blood insulin levels in each group were evaluated. In addition, we also assessed the expression level of total AMPK, phosphorylation AMPK, SIRT1, PGC-1 and the activity of G6PC in liver.
Results
The results demonstrated that ZG02 could significantly antagonize the high-fat diet/streptozotocin-induced fasting hyperglycemia, restore fasting blood insulin levels and also improve activity of G6PC in liver. The results from Western blot indicated that ZG02 significantly restored the expression level of phosphorylation AMPK, Sirt1 and PGC-1.
Conclusion
ZG02 improve hepatic glucose metabolism and insulin sensitivity via activation AMPK/Sirt1 signaling pathways in type 2 diabetes mice model.
Introduction
Type 2 diabetes mellitus (T2DM)Citation1 is a chronic metabolic disease,Citation2,Citation3 mainly characterized by relative insulin deficiency caused by pancreatic β-cell dysfunction and insulin resistance in target organs.Citation4 T2DM is the leading cause of morbidity and mortality worldwide and a major economic burden.Citation5 The prevalence of T2DM is increasing year by year due to the global rising tide of obesity, physical inactivity, and caloric excess.Citation6 Recently, a survey estimated that there will be more than 700 million people (aged 18–99 years) suffering from diabetes in nearly all countries by the year 2045.Citation7 Currently, the majority of oral hypoglycemic drugs, such as sulfonylureasCitation8 (SUs), meglitinidesCitation9 (glinides), thiazolidinedionesCitation10 (TZDs), α-glucosidase inhibitors,Citation11 glucagon-like peptide (GLP)-1 receptor (GLP-1R) agonists,Citation12 dipeptidyl peptidase (DPP)-4 inhibitors,Citation13 and sodium glucose transporterCitation14 (SGL). Although treatment of diabetes has a hypoglycemic effect, it has demonstrated side effects and adverse reactions. Hence, treatment of diabetes used for the major research areas is discovering and developing alternate drugs with fewer side effects.
The liver is a particularly important metabolic organ for controlling blood glucose and ~90% of endogenous glucose is produced by the hepatic system.Citation15 It can maintain blood glucose balance by regulating various pathways such as gluconeogenesis,Citation16,Citation17 glycogenolysis,Citation18,Citation19 glycogen synthesis and glycolysis.Citation20 Numerous studies show that gluconeogenesis contributes approximately half of the total hepatic glucose production (HGP) in humans following an overnight fast and inhibition of hepatic gluconeogenesis can significantly improve blood glucose in individuals with T2DM.Citation21–Citation23 Our previous work has revealed that a tetrahydrocarbazole derivatives compound ZG02Citation24,Citation25 () improves glucose metabolism via inhibition of gluconeogenesis in HepG2 cells.Citation26,Citation27 Nevertheless, the antidiabetic effect of ZG02 in vivo and its potential underlying mechanisms is still unclear. Consequently, this study attempted to demonstrate the potential of the antidiabetic effects of ZG02 and their underlying mechanisms in high-fat diet/streptozotocin (STZ)-induced T2DM mice.
Materials and Methods
Materials
Streptozotocin (S0130, 500 mg) was purchased from Sigma-Aldrich Co. (St Louis, MO, USA). Primary antibodies to AMPK (ab131512), p-AMPK (ab45174), Sirt1 (ab110304), and PGC-1α (ab191838) were purchased from Abcam (Cambridge Science Park, Cambridge, UK). Anti-rabbit IgG (no. 5151) and anti-mouse IgG (no. 5257) were purchased from CST (Cell Signaling Technology, Inc., Danvers, MA, USA). Mouse insulin (INS) ELISA kit (CSB-E05071m) was purchased from CUSABIO (Wuhan, China). Compound ZG02 () was synthesized in our lab.
Animals
Five-week-old male C57BL/6 mice (20±2 g) were supplied by the Experimental Animal Centre of Guizhou Medical University (permissions SYXK, 2019–0001). All the mice were fed with standard food and water under constant environment with 25±2°C and 12/12 h light/dark cycle (light on at 08:00 am) and adaptively raised for a week before experiments. All experiments and procedures were carried out according to the Regulations of Experimental Animal Administration issued by the State Committee of Science and Technology of China.
Diet/Streptozotocin-Induced Type 2 Diabetes Model
After acclimation for a week, C57BL/6 mice were randomly separated into two groups. Control group (n=10) was fed with normal diet containing 10% kcal fat and the model group (n=20) was fed with high-fat diet consisting of 60% kcal fat (D12492, Research diets, BioPike, China). For the establishment of a C57BL/6 mice model of T2DM, streptozotocin (STZ) (25 mg/kg−1 dissolved in 100 mM citrate buffer (pH 4.4), once every other day, four times) was injected intraperitoneally (ip) after eight weeks of high-fat diet. The control group were given an identical volume of vehicle citrate buffer. At four weeks after STZ injection, the successfully established animal models (fasting blood glucose ≥200 mg/dL or ≥11.1 mmol/mL) were randomly divided into the diabetic group (n=10) and ZG02 group (n=10). The ZG02 group was administered ZG02 (30 mg/kg/day) by ip injection once a day for 14 days. The other group was concurrently administered an equivalent dose of sterile water. Diabetic group and ZG02 group continued to be fed the high-fat diet. The experimental procedure is shown in .
Blood Glucose and Oral Glucose Tolerance Tests
For measurement of fasting blood glucose (FBG) levels, mice were fasted overnight for 16 h and FBG levels were obtained from a small drop of blood from tail snip using a Glucometer (Yuwell, Jiangsu, China). The oral glucose tolerance test (OGTT) was performed by glucose gavage with 50% 2 g·kg–1 at 30 min after mice fasted overnight for 16 h. The blood glucose levels were determined at 0.5, 1, or 2 h after glucose loading, and OGTT was expressed by the area under curve (AUC).
ELISA Determination of Insulin
For measurement of fasting blood insulin levels, mice were fasted overnight for 16 h and blood was collected from the caudal vein. Plasma insulin levels were detected by insulin ELISA kit (CUSABIO, Wuhan, China) according to kit instructions. The Homeostasis Model Assessment of IR (HOMA-IR) has proved to be a robust tool for the surrogate assessment of insulin resistance.Citation28 HOMA-IR can be calculated with the following formula: HOMA-IR index=fasting glucose (mmol/L)×fasting insulin (mU/L)/22.5.Citation29,Citation30
Western Blotting
The liver tissues were homogenized in RIPA lysis buffer and the sample maintained on ice for 60 min. The homogenate was collected and centrifuged at 12,000×g for 30 min at 4°C. The final supernatant was stored at −80°C until further use. The protein concentrations of the supernatants were analyzed by the BCA protein assay kit (Beyotime).
The supernatants were diluted to 40 μg/lane with sample buffer and heated at 95°C for five minutes. The protein mixtures were loaded on a SDS-PAGE gel. The separated proteins were transferred to the PVDF membrane (Millipore, Billerica, MA, USA). The membranes were blocked by 5% nonfat milk in TBST for two hours, followed by probing with primary antibodies at 4°C overnight in 2% nonfat milk-TBST: anti-AMPK (1:2000), anti-p-AMPK (1:3000), anti-Sirt1 (1:1000), anti-PGC-1α (1:1000). Subsequently, the blots were incubated with anti-rabbit IgG or anti-mouse IgG for one hour at room temperature. The immunoreactivity was detected with infrared fluorescence and images captured by Odyssey Imagers (LI-COR Biosciences, USA). The relative quantity of protein expression was analyzed by the software Image J and rectified by the reference protein β-actin.
Statistical Analysis
All the results are expressed as the mean ±SD. Inter-group statistical significance was determined by one-way ANOVA using STATA 14.0 (StataCorp, College Station, TX, USA) with a statistical significance set at p<0.05.
Results
Effects of ZG02 on Body Weight
The results showed that the weight was remarkably increased in diabetes group (p<0.01) compared to the control group. ZG02 treatment significantly restored the weight level compared to the diabetes group (p<0.05). The weight of each group is shown in .
Figure 3 (A) The levels of weight in each group. (B) The levels of FBG in each group. (C) The levels of OGTT in each group. (D) The AUC values of each group. (E) The levels of blood insulin in each group. (F) The levels of HOMA-IR in each group. *p<0.01 vs control group; **p<0.05 vs diabetes group. (G) The activity of G6PC in the liver in each group. *p<0.01 vs control group; ***p<0.01 vs diabetes group.
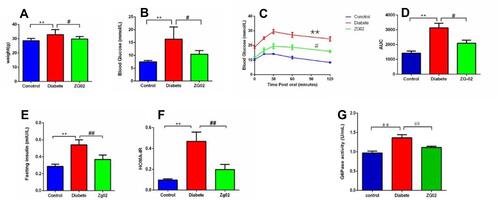
Effects of ZG02 on FBG
The FBG levels of each group are shown in . Compared with control group, the levels of FBG were significantly higher in the diabetes group (p<0.05). ZG02 treatment could decrease the FBG levels compared to the diabetes group.
Effects of ZG02 on OGTT
The OGTT was performed after an overnight fast (16 h) at week 15 of the experimentation. Following oral administration of glucose, blood glucose levels reached a maximum at 15–30 min postglucose gavage in the control group, and then gradually decreased. Blood glucose levels in diabetic mice were significantly higher than in the control group at 0, 15, 30, 60, and 120 min. After ZG02 treatment, the blood glucose levels in the ZG02 group were significantly lower than in the diabetes group at each time point (). The corresponding AUC profile in () is shown in (). The AUC values of the diabetes group were obviously increase 220% compared with the control group. The AUC values of the ZG02 group were obviously reduced 67% compared with the diabetes group ().
Effects of ZG02 on Serum Insulin Levels and HOMA-IR
Compared with the control group, serum insulin levels were significantly increased in the diabetes groups (p<0.001). Serum insulin levels of the ZG02 group were reduce compared with the diabetes group (). Similarly, the HOMA-IR in diabetes group were significantly increased compared with the control group (p<0.001). After ZG02 treatment, the HOMA-IR in the ZG02 group was significantly lower (p<0.001) compared with the diabetes group (). These results indicated that ZG02 can ameliorate insulin resistance in diabetic mice.
Effects of ZG02 on the Activity of G6PC
Compared with the control group (), the activity of G6PC in the liver was significantly increased in the diabetes groups (p<0.001). After ZG02 treatment, the activity of G6PC in the ZG02 group was significantly lower than in the diabetes group (p<0.001).
Effects of ZG02 on the Levels of Protein of AMPK-SIRT1-PGC-1 Pathway
Compared with the control group ( and ), the expression levels of phosphorylation AMPK, SIRT1 and PGC-1 were significantly decreased in the diabetes groups (p<0.01, p<0.01, p<0.01). After ZG02 treatment, the expression levels of phosphorylation AMPK, SIRT1, FoxO1 and PGC-1 were significantly increased (p<0.01, p<0.01, p<0.01). The expression levels of total AMPK were not changed in each group.
Discussion
The aim of the present study was to assess the importance of tetrahydrocarbazole derivatives ZG02 in improving hepatic glucose metabolism and insulin sensitivity via activation AMPK/Sirt1/FoxO1/PGC-1α signaling pathways in mice on a high-fat diet with streptozotocin-induced type 2 diabetes.
AMPK (adenosine monophosphate-activated protein kinase) belongs to the RD (Arg-Asp) kinases.Citation31 It is known as a serine/threonine kinase that functions as an intracellular energy sensor and has been implicated in the modulation of glucose and fatty acid metabolism. AMPK plays an important role in maintaining energy homeostasis and adaptive response to energy stress. It is activated in response to energy stress by sensing increases in AMP/ATP and ADP/ATP ratios and restores energy balance by inhibiting anabolic processes that consume ATP, while promoting catabolic processes that generate ATP.Citation32–Citation35 Under conditions of low energy, AMPK phosphorylates specific enzymes and growth control nodes to increase ATP generation and decrease ATP consumption.The hydrolysis of ATP to ADP provides the energy for driving virtually all of the processes associated with living cells. Maintaining an adequate supply of energy is an essential requirement for survival.AMPK plays an important role in maintaining energy homeostasis in eukaryotic cells.Citation33,Citation36 By activation of the AMPK, the pathway can improve the symptoms of type 2 diabetes, and currently metformin is accepted treatment for the syndrome caused by diabetes,Citation37–Citation41 and enhances insulin sensitivity by a decrease in the activity of AMPK-mediated signaling.Citation42 The antidiabetic effect of the first-line antidiabetic medicine metformin is based on the activation of the AMPK system,Citation43 SIRT1 is a NAD+-dependent protein deacetylases/deacylases that is frequently overexpressed in a wide variety of mechanisms of biological metabolism processes,Citation44,Citation45 phospho-AMPK can also lead to an increase in nicotinamide phosphoribosyltransferase (NAMPT) enzyme activity and thereby increasing NAD+/NADH ratio which in turn induces SIRT1.Citation46,Citation47 SIRT1 is a master repressor of inflammation in multiple organs including the liver. It was investigated whether liver SIRT1 deficiency in C57BL/6 mice had any impact on the high-fat diet/streptozotocin-induced type 2 diabetes model responses.Citation48 SIRT1 will act on the AMPK pathway, and then regulate the glycogen synthesis and gluconeogenesis,Citation49 PGC-1α (proliferator-activated receptor-γ coactivator 1α) is a direct substrate of SIRT1, maintained blood glucose concentration and insulin sensitivity by regulating the transcription of genes involved in glucose metabolism. Recent insight from different in vivo transgenic models clearly suggests that AMPK, SIRT1 and PGC-1a might act as an orchestrated network to improve metabolic fitness, dysregulation of these pathways will lead to metabolic diseases such as type 2 diabetes and obesity.
The current study found suggest that ZG02 could reduce the level of FBG, increase insulin sensitivity, and inhibit the enzyme activity of G6PC in high-fat diet/streptozotocin-induced type 2 diabetes C57BL/6 mice in vivo. These significant effects of ZG02 were mediated by activation of the AMPK/SIRT1/PGC-1α signal pathway and the result was consistent with our previous study in HepG2 cells. In addition, a large number of studies also have confirmed the therapeutic role of AMPK/SIRT1/PGC-1 pathway in diabetes or its complications.
Taken together, our data demonstrate that ZG02 has a therapeutic potential for the treatment of type 2 diabetes can be attributed to its regulation of the activation of the AMPK/SIRT1/PGC-1α signaling pathway. These results will lay the foundation for future ZG02 into medicinal development.
Acknowledgments
This work was supported by National-Local Joint Engineering Research Center for Innovative & Generic Chemical Drug, Guizhou High-level Innovative Talents Supporting Program (2016-4015), Guizhou Provincial Engineering Laboratory for Chemical Drug R&D and Guizhou High-School Engineering Research Center for Medicinal Chemistry (KY2014-219) and Support by Guizhou Provincial Natural Science Foundation (2019) 2785, (2017) 2835.
Disclosure
The authors report no conflicts of interest in this work.
References
- Jaeger A, Zollinger L, Saely CH, et al. Circulating microRNAs −192 and −194 are associated with the presence and incidence of diabetes mellitus. Sci Rep. 2018;8(1):14274. doi:10.1038/s41598-018-32274-9.
- Neuen BL, Young T, Heerspink HJL, et al. SGLT2 inhibitors for the prevention of kidney failure in patients with type 2 diabetes: a systematic review and meta-analysis. The Lancet Diabetes & Endocrinology. 2019;7(11):845–854. doi:10.1016/S2213-8587(19)30256-6.
- Parkman HP, Wilson LA, Farrugia G, et al. Delayed Gastric Emptying Associates With Diabetic Complications in Diabetic Patients With Symptoms of Gastroparesis. Am J Gastroenterol. 2019;114(11):1778–1794. doi:10.14309/ajg.0000000000000410.
- Bader E, Migliorini A, Gegg M, et al. Identification of Proliferative and Mature β-cells in the Islets of Langerhans. Nature. 2016;535(7612):430–434. doi:10.1038/nature18624.
- Amiel SA, Aschner P, Childs B, et al. Hypoglycaemia, cardiovascular disease, and mortality in diabetes: epidemiology, pathogenesis, and management. Lancet Diabetes Endo. 2019;7(5):385–396. doi:10.1016/S2213-8587(18)30315-2.
- Fletcher B, Gulanick M, Lamendola C, et al. Risk Factors for Type 2 Diabetes Mellitus. J Cardiovasc Nurs. 2002;16(2):17–23. doi:10.1097/00005082-200201000-00003.
- International Diabetes Federation. IDF Diabetes Atlas. 9. Brussels, Belgium: International Diabetes Federation; 2019. doi:10.1016/j.diabres.2019.107843.
- Khunti K, Chatterjee S, Gerstein HC, et al. Do Sulphonylureas Still Have a Place in Clinical Practice? Lancet Diabetes Endo. 2018;6(10):821–832. doi:10.1016/S2213-8587(18)30025-1.
- Kennedy KE, Teng C, Patek TM, et al. Hypoglycemia Associated With Antibiotics Alone and in Combination With Sulfonylureas and Meglitinides: an Epidemiologic Surveillance Study of the FDA Adverse Event Reporting System (FAERS). Drug Saf. 2020;43(4):363–369. doi:10.1007/s40264-019-00901-7.
- Kwon MJ, Lee YJ, Jung HS, et al. The direct effect of lobeglitazone, a new thiazolidinedione, on pancreatic beta cells: A comparison with other thiazolidinediones. Diabetes Res Clin Pract. 2019;151:209–223. doi:10.1016/j.diabres.2019.04.006.
- Moelands SV, Lucassen PL, Akkermans RP, et al. Alpha-glucosidase Inhibitors for Prevention or Delay of Type 2 Diabetes Mellitus and Its Associated Complications in People at Increased Risk of Developing Type 2 Diabetes Mellitus. Cochrane Database Syst Rev. 2018;12(12):CD005061. doi:10.1002/14651858.CD005061.pub3.
- Dhameja M, Gupta P. Synthetic Heterocyclic Candidates as Promising α-glucosidase Inhibitors: an Overview. Eur J Med Chem. 2019;176:343–377. doi:10.1016/j.ejmech.2019.04.025.
- Gentilella R, Pechtner V, Corcos A, et al. Glucagon-like peptide-1 receptor agonists in type 2 diabetes treatment: are they all the same? Diabetes Metab Res Rev. 2019;35(1):3070. doi:10.1002/dmrr.3070.
- Choi B, Lee S, Kim S-M, et al. Dipeptidyl Peptidase-4 Induces Aortic Valve Calcification by Inhibiting Insulin-Like Growth Factor-1 Signaling in Valvular Interstitial Cells. Circulation. 2017;135(20):1935–1950. doi:10.1161/CIRCULATIONAHA.116.024270.
- Katsiki N, Perakakis N, Mantzoros C. Effects of Sodium-Glucose co-transporter-2 (SGLT2) Inhibitors on Non-Alcoholic Fatty Liver disease/non-alcoholic Steatohepatitis: ex Quo Et Quo Vadimus? Metabolism. 2019;98:iii–ix. doi:10.1016/j.metabol.2019.07.009
- Trefts E, Gannon M, Wasserman DH. The Liver. Curr Biol. 2017;27(21):1147–1151. doi:10.1016/j.cub.2017.09.019.
- Yan H, Yang W, Zhou F, et al. Estrogen Improves Insulin Sensitivity and Suppresses Gluconeogenesis via the Transcription Factor Foxo1. Diabetes. 2019;68(2):291–304. doi:10.2337/db18-0638.
- Puigserver P, Rhee J, Donovan J, et al. Insulin-regulated hepatic gluconeogenesis through FOXO1–PGC-1α interaction. Nature. 2003;423(6939):550–555. doi:10.1038/nature01667.
- Eugenin EA, Valdebenito S, Gorska AM, et al. Gap Junctions Coordinate the Propagation of Glycogenolysis Induced by Norepinephrine in the Pineal Gland. J Neurochem. 2019;151(5):558–569. doi:10.1111/jnc.14846.
- Barr D, Szennyes E, Bokor É, et al. Identification of C -β-d-Glucopyranosyl Azole-Type Inhibitors of Glycogen Phosphorylase That Reduce Glycogenolysis in Hepatocytes: In Silico Design, Synthesis, in Vitro Kinetics, and ex Vivo Studies. ACS Chem Biol. 2019;14(7):1460–1470. doi:10.1021/acschembio.9b00172.
- Hirase H, Akther S, Wang X, et al. Glycogen Distribution in Mouse Hippocampus. J Neurosci Res. 2019;97(8):923–932. doi:10.1002/jnr.24386.
- Saslow LR, Mason AE, Kim S, et al. An Online Intervention Comparing a Very Low-Carbohydrate Ketogenic Diet and Lifestyle Recommendations Versus a Plate Method Diet in Overweight Individuals With Type 2 Diabetes: A Randomized Controlled Trial. J Med Internet Res. 2017;19(2):36. doi:10.2196/jmir.5806
- Chakravarthy M, Parsons S, Lassman ME, et al. Effects of 13-Hour Hyperglucagonemia on Energy Expenditure and Hepatic Glucose Production in Humans. Diabetes. 2017;66(1):36–44. doi:10.2337/db16-0746.
- Cuthbertson DJ, Shojaee-Moradie F, Sprung VS, et al. Dissociation Between Exercise-Induced Reduction in Liver Fat and Changes in Hepatic and Peripheral Glucose Homoeostasis in Obese Patients With Non-Alcoholic Fatty Liver Disease. Clin Sci. 2016;130(2):93–104. doi:10.1042/CS20150447.
- Zhang J-Q, Li S-M, Ma X, et al. Discovery of Tetrahydrocarbazoles With Potent Hypoglycemic and Hypolipemic Activities. Eur J Med Chem. 2018;150:102–112. doi:10.1016/j.ejmech.2018.02.069.
- Xuguang H, Aofei T, Tao L, et al. Hesperidin ameliorates insulin resistance by regulating the IRS1-GLUT2 pathway via TLR4 in HepG2 cells. Phytother Res. 2019;33(6):1697–1705. doi:10.1002/ptr.6358.
- Chen L, Teng H, Cao H, et al. Chlorogenic acid and caffeic acid from Sonchus oleraceus Linn synergistically attenuate insulin resistance and modulate glucose uptake in HepG2 cells. Food Chem Toxicol. 2019;127:182–187. doi:10.1016/j.fct.2019.03.038.
- Yu Z, Shiguo C, Yang WC, et al. Proanthocyanidins from Chinese bayberry leaves regulate lipid metabolism and glucose consumption by activating AMPK pathway in HepG2 cells.. J Funct Foods. 2014;29:217–225. doi:10.1016/j.jff.2016.12.030.
- Kuo C-S, Chen J-S, Lin L-Y, et al. Inhibition of Serine Protease Activity Protects Against High Fat Diet-Induced Inflammation and Insulin Resistance. Sci Rep. 2020;10(1):1725. doi:10.1038/s41598-020-58361-4
- Mesallamy HO, Hamdy NM, Mostafa DM, et al. The serine protease granzyme B as an inflammatory marker, in relation to the insulin receptor cleavage in human obesity and type 2 diabetes mellitus. J Interferon Cytokine Res. 2014;34(3):179–186. doi:10.1089/jir.2013.0059
- Chakraborty S, Datta S, Ghosh S. Induction of autophagy under nitrosative stress: A complex regulatory interplay between SIRT1 and AMPK in MCF7 cells. Cell Signal. 2018;8:109411. doi:10.1016/j.cellsig.2019.109411
- Shaw RJ, et al. The kinase LKB1 mediates glucose homeostasis in liver and therapeutic effects of metformin. Science. 2005;310(5754):1642–1646. doi:10.1126/science.1120781
- Carling D. AMPK signalling in health and disease.[J]. Curr Opin Cell Biol. 2017;45:31–37. doi:10.1016/j.ceb.2017.01.005.
- Boyer PD, Chance B, Ernster L, et al. Oxidative phosphorylation and photophosphorylation. Annual Review of Biochemistry. 2019;97(1):955–1026. doi:10.1002/jnr.24386
- Zang M, Zuccollo A, Hou X, et al. AMP-activated protein kinase is required for the lipid-lowering effect of metformin in insulin-resistant human HepG2 cells. J Biol Chem. 2004;279:47898–47905. doi:10.1074/jbc.M408149200.
- Carling D, Thornton C, Woods A, et al. AMP-activated protein kinase: new regulation, new roles? Biochem J. 2012;445:11–27. doi:10.1042/BJ20120546.
- Cokorinos EC, Delmore J, Reyes AR, et al. Activation of Skeletal Muscle AMPK Promotes Glucose Disposal and Glucose Lowering in Non-human Primates and Mice. Cell Metab. 2017;25:1147–1159. doi:10.1016/j.cmet.2017.04.010
- Fullerton MD, Galic S, Marcinko K, et al. Single phosphorylation sites in Acc1 and Acc2 regulate lipid homeostasis and the insulin-sensitizing effects of metformin. Nat Med. 2013;19:1649–1654. doi:10.1038/nm.3372.
- Settembre C, Zoncu R, Medina DL, et al. A lysosome-to-nucleus signalling mechanism senses and regulates the lysosome via mTOR and TFEB. EMBO J. 2012;31:1095–1108. doi:10.1038/emboj.2012.32.
- Myers RW, Guan HP, Ehrhart J, et al. Systemic pan-AMPK activator MK-8722 improves glucose homeostasis but induces cardiac hypertrophy. Science. 2017;357:507–511. doi:10.1126/science.aah5582.
- Chakraborty S, Datta S, Ghosh S, et al. Induction of autophagy under nitrosative stress: A complex regulatory interplay between SIRT1 and AMPK in MCF7 cells. Cell Signal. 2019;64:109411. doi:10.1016/j.cellsig.2019.109411.
- Dugan LL, You YH, Ali SS, et al. AMPK dysregulation promotes diabetes-related reduction of superoxide and mitochondrial function. J Clin Invest. 2013;123(11):4888–4899. doi:10.1172/JCI66218.
- Kim YD, Park KG, Lee YS, et al. Metformin inhibits hepatic gluconeogenesis through AMP-activated protein kinase-dependent regulation of the orphan nuclear receptor SHP. Diabetes. 2008;57(2):306–314. doi:10.2337/db07-0381.
- Lei Y, Wang J, Wang D, et al. SIRT1 in forebrain excitatory neurons produces sexually dimorphic effects on depression-related behaviors and modulates neuronal excitability and synaptic transmission in the medial prefrontal cortex. Mol Psychiatry. 2020;25(5):1094–1111. doi:10.1038/s41380-019-0352-1.
- Canto C, Auwerx J. PGC-1alpha, SIRT1 and AMPK, an energy sensing network that controls energy expenditure. Curr Opin Lipidol. 2009;20:98–105. doi:10.1097/MOL.0b013e328328d0a4.
- Fulco M, Cen Y, Zhao P, et al. Glucose restriction inhibits skeletal myoblast differentiation by activating SIRT1 through AMPK-mediated regulation of Nampt. Dev Cell. 2008;14(5):661–673. doi:10.1016/j.devcel.2008.02.004.
- Zhou Z, Ye TJ, DeCaro E, et al. Intestinal SIRT1 Deficiency Protects Mice from Ethanol-Induced Liver Injury by Mitigating Ferroptosis. Am J Pathol. 2020;190(1):82–92. doi:10.1016/j.ajpath.2019.09.012.
- Yan F, Zhang J, Zhang L, et al. Mulberry anthocyanin extract regulates glucose metabolism by promotion of glycogen synthesis and reduction of gluconeogenesis in human HepG2 cells. Food Funct. 2016;7:425–433. doi:10.1039/c5fo00841g.
- Anyamaneeratch K, Rojvirat P, Sukjoi W, et al. Insights into transcriptional regulation of hepatic glucose production. Int Rev Cell and Mol Biol. 2015;318:203–253. doi:10.1016/bs.ircmb.2015.05.004.