Abstract
Purpose
Fractures in patients with type 2 diabetes mellitus are at a high risk of delayed union or non-union. Previous studies have shown a protective effect of liraglutide on bone. In the present study, we aimed to investigate the effects of a combination of liraglutide and insulin on fracture healing in a rat model of diabetes and the mechanisms involved.
Materials and Methods
Closed femoral mid-shaft fractures were established in male Sprague-Dawley rats with or without diabetes mellitus, and the diabetic rats were administered insulin and/or liraglutide. Six weeks after femoral fracture, the femoral callus was evaluated by immunohistochemistry, histology, and micro-computed tomography. Additionally, the effects of liraglutide on high-glucose-stimulated MC3T3-E1 cells were analyzed by Western blotting.
Results
Micro-computed tomography and safranin O/fast green staining showed that fracture healing was delayed in the diabetic rats, and this was accompanied by much lower expression of osteogenic markers and greater osteoclast activity. However, treatment with insulin and/or liraglutide prevented these changes. Liraglutide in combination with insulin treatment resulted in lower blood glucose concentrations and significantly higher osteocalcin (OCN) and collagen I (Col I) expression six weeks following fracture. Western blot analysis showed that liraglutide prevented the low expression of the bone morphogenetic protein-2, osterix/SP7, OCN, Col I, and β-catenin in high-glucose-stimulated MC3T3-E1 cells.
Conclusion
These results demonstrate that insulin and/or liraglutide promotes bone fracture healing in the DF model. The combination was more effective than either single treatment, which may be because of the two drugs’ additive effects on the osteogenic ability of osteoblast precursors.
Introduction
Skeletal fracture is a rapidly growing problem that seriously affects human health. Type 2 diabetes mellitus (T2DM) is a common chronic metabolic disease that accounts for >90% of cases of diabetes. With recent changes in lifestyle, the prevalence of T2DM is increasing annually.Citation1 T2DM impairs bone formation by affecting osteoblast survival and suppressing the expression and function of osteogenic factors.Citation2 It has also been recognized to inhibit tissue healing, cause poor fracture healing, and induce other complications in patients.Citation3
Fracture healing normally progresses through inflammatory, reparative, and remodeling phases. An inflammatory reaction is activated immediately after a fracture has occurred, and activated immune cells subsequently interact with bone cells. The bone fragments are bridged, which is followed by a slow bone reconstruction process.Citation4 This series of biological events is affected by numerous factors. For example, negative effects of T2DM on fracture healing have been documented,Citation2,Citation5,Citation6 which include disorders of both bone resorption and formation, characterized by the presence of fewer osteoblasts and larger numbers of osteoclasts. However, osteoclast-mediated bone resorption is an important component of bone remodeling.Citation7 Hyperglycemia, glycation end-products, and oxidative stress lead to delayed fracture healing or even non-union.Citation5
Insulin previously has been shown to promote fracture healing in patients with T2DM.Citation8–10 Glucagon-like peptide-1 (GLP-1) has effects in a variety of organs through binding to its receptors and promoting insulin release. Nuche-Berenguer et al showed that GLP-1 treatment ameliorates the high level of anisotropy in trabecular bone that characterizes T2DM and insulin resistance in rats.Citation11 Liraglutide is a long-acting GLP-1 receptor agonist drug that can reduce the incidence of fracture.Citation12 However, although the switch from the use of a dipeptidyl peptidase-4 inhibitor (DPP-4i) to a GLP-1RA is associated with better glycemic control, it is less beneficial for bone than continuing DPP-4i use.Citation13 In some previous animal studies, liraglutide treatment has been shown to improve bone mineral density (BMD) and ameliorate bone microstructural damage in diabetic rats by stimulating the expression of the osteogenic marker osteocalcin (OCN).Citation14 However, to the best of our knowledge, there have been no studies of the efficacy of treatment with a combination of liraglutide and insulin on fracture healing in T2DM. Therefore, in the present study, we aimed to determine whether liraglutide in combination with insulin would promote better fracture healing in diabetic rats.
Materials and Methods
Animals and Diet
Eight-week-old male Sprague-Dawley rats (HFK Biological Technology Co., Ltd., Beijing, China) were used in the present study. The experiment was approved by the North China University of Science and Technology Animal Ethics Committee and conducted in the Laboratory Animal Center of North China University of Science and Technology, Tangshan City, Hebei province. Six rats were assigned to a control (CF) group and fed a standard diet (1025, HFK Biological). The remaining 24 rats were fed a high-fat diet (HFD) (H10141, HFK Biological). All the rats received feed and water ad libitum. After eight weeks, the HFD-fed rats were injected with streptozotocin (STZ) (35 mg/kg) (18,883–66-4, Sigma, Chemical Company, St. Louis, MO, USA) and one week later, their non-fasting blood glucose concentrations were measured. The rats were regarded as having diabetes when they had blood glucose concentrations of >16.7 mmol/L at this time.Citation15 Oral glucose tolerance testing was also performed (50% glucose, 2 g/kg), and a combination of fasting blood glucose concentrations >7 mmol/L and 2-hour concentrations >11.1 mmol/L was regarded as indicative of diabetes. The diabetic rats were randomly allocated to a diabetic (DF, n = 6) group, an insulin-treated DF (DFI, n = 6) group, a liraglutide-treated DF (DFL, n = 6) group, and an insulin and liraglutide-treated DF (DFIL, n = 6) group. The fasting blood glucose concentration and body mass of each rats were measured every week. Insulin (Novolin N, Novo Nordisk A/S, Copenhagen, Denmark) was injected subcutaneously at doses of 2–4 iu and 4–6 iu in the morning and evening, respectively, adjusted according to the blood glucose concentration. Liraglutide (Novo Nordisk) was injected subcutaneously at a daily dose of 0.6 mg/kg.Citation16,Citation17
Surgical Procedure
On successful establishment of the diabetes model, femoral fracture was induced. A simple transverse or short oblique closed fracture of the femur, based on the Einhorn closed fracture modeling method, was created in each animal.Citation18–20 This procedure was performed under inhalation anesthesia with isoflurane. Through the femoral intercondylar fossa, which was exposed by a 1-cm incision over the stifle, a 0.45-mm Steinmann pin was retrogradely inserted to fix the femoral fracture. After closing the incision, a femoral fracture was established using the blunt trauma equipment introduced by Einhorn. The fracture status was confirmed by X-ray, and rats with comminuted fractures were excluded. The DFI, DFL, and DFIL groups were administered liraglutide, insulin, or both drugs subcutaneously, and the insulin dose was adjusted according to the blood glucose concentrations of the rats. In addition, the DF and CF groups were administered subcutaneous injections of normal saline. Six weeks after fracture, the rats were anesthetized with isoflurane (2% in oxygen) and euthanized using CO2. The fractured femur was collected after removal of the surrounding soft tissue.
Micro-Computed Tomography (Micro-CT)
The femoral fracture area was scanned using a micro-CT system (SkyScan 1176, Bruker, Kontich, Belgium). The BMD, percentage bone volume, trabecular number (TB.N), and trabecular separation (TB.SP) were calculated using the analysis software provided with the machine (CT Analyser 1.17.7.2, Data Viewer 1.5.1.2, CTvox 3.0.0 r1114, Bruker micro-CT) to characterize the bone structure.
Safranin O/Fast Green Staining
Femurs were suspended in 0.5 mmol/L EDTA solution at room temperature for eight weeks for decalcification, and the solution was replaced every week. Following decalcification, the femurs were dehydrated, wax-embedded, and sectioned at 6 µm. The tissue sections were deparaffinized and rehydrated, then safranin O/fast green staining was performed. Using Image-Pro Plus 6.0, the percentage mineralized area per unit periosteal callus area and the cartilage area per unit periosteal callus area were calculated after manual delineation.Citation21
Tartrate-Resistant Acid Phosphatase (TRAP) Staining
TRAP staining was carried out according to the manufacturer’s instructions (G1492, Solarbio, Beijing, China). The area of the callus was defined as the region of interest. TRAP-positive cells were counted using image analysis software.Citation22
Alkaline Phosphatase (ALP) Staining
A BCIP/NBT kit was used to stain the sections for ALP activity (ZD315, Zoman Biotechnology Co., Ltd., Beijing, China) and Image-Pro Plus 6.0 was used to count the osteoblasts.
Immunohistochemical Analysis
Immunohistochemical staining was performed using commercially available antibodies against Col I, OCN, and β-catenin. Six-micrometer-thick sections of decalcified bone were incubated overnight at room temperature with anti-Col I (1:200, ab34710; Abcam, Cambridge, UK), anti-OCN (1:200, ARG65886, Arigo, Shanghai, China), or anti-β-catenin (1:200, 612536, BD, Franklin Lakes, NJ, USA) monoclonal antibodies. The sections were then incubated at 37°C for 30 min with peroxidase-conjugated goat anti-rabbit antibody (PV-6000, Zhongshan Jinqiao, Beijing, China), after which diaminobenzidine (ZLI-9018; Zhongshan Jinqiao) was used to visualize the immunoreactivity. The proportion of immunohistochemically positive cells was determined by counting under a microscope (BX53 Optical Microscope and DP80 CCD Image Acquisition System, Olympus, Tokyo, Japan). Yellow-brown granules in the cytoplasm were used to identify positive cells and semi-quantitatively assess expression levels.
Cell Culture and Treatment
The MC3T3-E1 murine embryonic osteoblast precursor cell line was obtained from the Chinese Academy of Sciences cell library (Shanghai, China) and cultured in α-MEM medium (8121506, Gibco, Waltham, MA, USA). Cells were seeded into 25-cm2 flasks and treated with or without one or both of 10−3 mmol/L liraglutide and 22.4 mmol/L D-glucose (S11022, Yuanye, Shanghai, China) for 24 h.
Alizarin Red S (ARS) Staining
Following osteoblast induction and differentiation, the cells were fixed at room temperature for 15 minutes with 4% paraformaldehyde, then washed three times with phosphate-buffered saline. The cells were stained with alizarin red S at pH 4.2. Image-Pro Plus 6.0 was used to count the osteoblasts.
Western Blotting
We performed Western blot analysis as previously described.Citation23 The primary antibodies used were polyclonal rabbit antibodies against BMP2 (ARG57829, Arigo), osterix/SP7 (ARG65886, Arigo), OCN (ARG65886, Arigo), Col I (ab34710, Abcam), β-catenin (612536, BD), and α-tubulin (AF7010, Affinity Biosciences, Cincinnati, OH, USA), at a dilution of 1:1000 at 4°C overnight. Subsequently, incubation was performed with anti-mouse or goat anti-rabbit secondary antibodies at a dilution of 1:5000 in blocking buffer at room temperature for 40 minutes. ECL Prime Western Blotting Detection Reagent was used to visualize specific bands (ZD310A, Zoman, Biotechnology Co., Ltd.). The band intensities corresponding to the target proteins were normalized to the corresponding control group and the α-tubulin expression.
Statistical Analysis
We used SPSS v.20.0 (IBM, Inc., Armonk, NY, USA) for statistical analysis. The Shapiro–Wilk test was used to evaluate the distribution of each dataset and Levene’s test was used to evaluate the homogeneity of variance. ANOVA was used to analyze normally distributed data, followed by the LSD test, as appropriate; and the Kruskal–Wallis test was used to analyze non-normally distributed data. The data are expressed as mean ± standard deviation, and p<0.05 was taken to indicate statistically significant differences.
Results
Blood Glucose Concentration and Body Mass
Six weeks after fracture induction, the blood glucose concentration of the DF group was significantly higher than that of the other groups. The blood glucose concentrations of the DFI and DFL groups were significantly lower than that of the DF group, and the blood glucose concentration of the DFIL group was significantly lower than those of the DFI and DFL groups ().
Figure 1 (A) Blood glucose concentrations during the study. Data are expressed as mean±SD. *p<0.05 vs the CF group; #p<0.05 vs the DF group; +p<0.05 DFIL group vs the DFI group; ¥p<0.05 DFIL group vs the DFL group. (B) Body masses during the study. Data are expressed as mean±SD. *p<0.05 vs CF group; #p<0.05 DFI group vs DF group; +p<0.05 DFL group vs DF group; ¥p<0.05 DFIL group vs DF group.
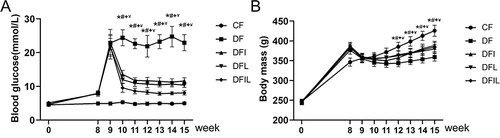
The rats that were injected with STZ showed immediate reductions in body mass, but this had slightly increased three weeks later. At the end of the study period, the body mass of the CF group was significantly higher than those of the other groups, and the DF had the lowest mass, which was much lower than those of the other groups (p<0.05). There were no significant differences in the masses of the DFIL, DFI, and DFL groups ().
Liraglutide Promotes Osteoblast Formation and Inhibits Osteoclast Differentiation in Diabetic Rats
Safranin O/fast green staining and ALP staining of rat bone tissue ( and ) six weeks after fracture showed that the transformation from cartilage callus to mineralized callus was delayed in the DF group versus the CF group, because the Cg.Ar/Ps.Cl.Ar of the DF group was significantly higher than that of the CF group. The DFL, DFI, and DFIL groups had intermediate values. The Md.Ar/Ps.Cl.Ar of the DF group was less than that of the CF group, and the opposite trend was apparent in the DFIL group ( and ).
Figure 2 (A) Safranin O/fast green-stained longitudinal sections of calluses six weeks after fracture. Woven bone is stained green and cartilage tissue is stained red. Black bars, 2 mm. (B) TRAP-stained longitudinal sections of calluses 6 weeks after fracture. TRAP-positive multinucleated cells are stained red in the callus. Black bars, 100 μm. (C) ALP-stained longitudinal sections of calluses 6 weeks after fracture. Osteoblasts are stained blue. Black bars, 100 μm. (D and E) Mineralized area per unit periosteal callus area: Md.Ar/Ps.Cl.Ar (%) (D) and the cartilage area per unit periosteal callus area: Cg.Ar/Ps.Cl.Ar (%) (E) for the five groups of rats. (F) Number of Trap-positive osteoclasts per unit callus area for the five groups. (G) Number of osteoblasts per unit callus area for the five groups. Data are expressed as mean ± SD. *p<0.05 vs CF group; #p<0.05 vs DF group; +p<0.05 vs DFI group. n = 6 per group.
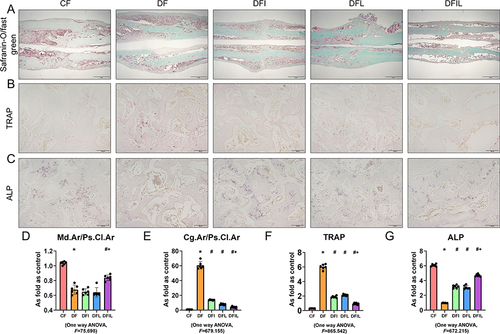
The ALP expression of the DF group was lower than that of the CF group, and those of the DFI, DFL, and DFIL groups were intermediate, with the highest value in the DFIL group (). These results suggest that liraglutide promotes osteoblast differentiation during fracture healing.
TRAP staining was used to quantify the number and activity of the osteoclasts. Six weeks following fracture, there were only a few TRAP-positive cells in the CF group (), whereas there were a large number of TRAP-positive cells around the healing lesion in the DF group. Insulin, liraglutide, and the combination reduced the number of osteoclasts. Moreover, the number of TRAP-positive cells in the DFIL group was significantly lower than that in the DFI group (). This suggests that liraglutide in combination with insulin is more effective at inhibiting osteoclast formation.
Liraglutide Promotes Fracture Healing in Diabetic Rats
As shown in , compared with the CF group, the callus associated with the femoral fracture in the DF group was poor; the BMD, percentage bone volume, and TB.N were significantly lower; and the trabecular separation was significantly greater. Following treatment with insulin and/or liraglutide, fracture healing was significantly better, and the TB.N of the DFIL group was significantly higher than that of the DFI group.
Liraglutide Treatment Increases OCN, Col I, and β-Catenin Expression in Diabetic Rats
OCN is the most abundant collagen protein in bone and is exclusively produced by osteoblasts. Moreover, the serum OCN concentration is regarded as a marker of bone formation.Citation24 The Col I molecule accounts for approximately 90% of the organic matrix of bone and is an important component of bone structure.Citation25 In addition, β-catenin plays a crucial role in the growth and development of mineralized tissues.Citation26 Six weeks after femoral fracture, immunohistochemical staining showed that OCN, Col I, and β-catenin expression was significantly lower in the DF group than in the CF group, significantly higher in the DFI and DFL groups than in the DF group, and significantly higher in the DFIL group than in the DFI group (). These results show that osteoblast formation is inhibited following fracture in diabetic rats and that osteoblast formation is increased by the combination treatment.
Figure 4 (A, C, and E) Immunohistochemical staining for Col I, OCN, and β-catenin in the callus of the five groups. Black bars, 100 μm. OCN (B), Col I (D), and β-catenin (F) expression in the callus of the five groups. Data are expressed as mean ± SD. *p<0.05 vs CF group; #p<0.05 vs DF group; +p<0.05 vs DFI group. n = 6 per group.
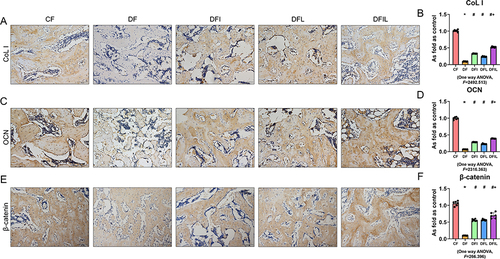
Liraglutide Activates D-Glucose-Inhibited Osteoblast Signaling in MC3T3-E1 Cells
To confirm a direct effect of liraglutide on osteoblast differentiation, MC3T3-E1 cells were treated with a high concentration of glucose and/or liraglutide. The area of ARS-positive cells was reduced by D-glucose administration and increased by liraglutide treatment ( and ). Western blot analysis was performed to quantify the expression of the osteoblast markers BMP2, SP7, OCN, and Col I. D-glucose dose-dependently reduced the expression of BMP2, SP7, OCN, Col I, and β-catenin in MC3T3-E1 cells ( and ) and liraglutide dose-dependently increased the expression of all of these proteins ( and ). Following pretreatment with liraglutide, stimulation of MC3T3-E1 cells with D-glucose was associated with significantly higher BMP2, SP7, OCN, Col I, and β-catenin expression, compared with D-glucose treatment alone ( and ). These results suggest that liraglutide promotes osteoblast differentiation.
Figure 5 Effects of liraglutide in MC3T3 cells. (A and B) ARS-stained MC3T3 cells treated with a high glucose concentration and/or liraglutide. (C–H) Assessment of the effects of D-glucose (C and D) and liraglutide (E and F) on the protein expression of BMP2, SP7, OCN, Col I, and β-catenin in MC3T3-E1 cells, using Western blotting. (G and H) Liraglutide increased the BMP2, SP7, OCN, Col I, and β-catenin expression in D-glucose-treated MC3T3-E1 cells. *p<0.05 vs C group. Data are expressed as mean ± SD. n = 3 per group.
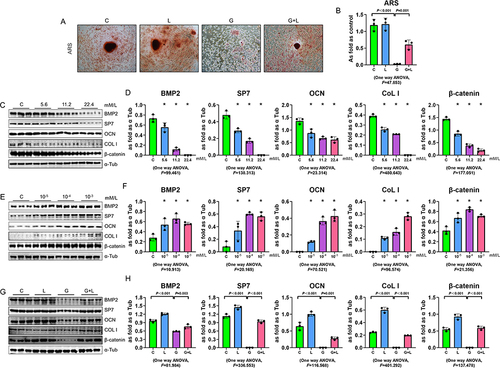
Discussion
In the present study, we have provided in vivo and in vitro evidence that diabetes impairs fracture healing in rats, and that liraglutide alone or in combination with insulin ameliorates these defects. Moreover, the use of a combination of insulin and liraglutide had significant advantages over that of insulin alone.
Patients with T2DM are characterized by hyperglycemia and high circulating concentrations of advanced glycation end-products, which increase the number of osteoclasts and affect osteoblast formation, and thereby have an adverse impact on fracture healing.Citation27 High glucose concentrations induce higher expression of proinflammatory factors, reduce the activity of osteoblasts, increase the apoptosis of osteoblasts, interfere with matrix mineralization, and can directly affect fracture healing.Citation28–30
In the present study, a rat model of T2DM was established by feeding an HFD and administering STZ.Citation15 A series of findings confirmed that fracture healing in diabetic rats is significantly delayed. Micro-CT examination showed lower BMD, which was consistent with the results of previous studies;Citation31,Citation32 and safranin O/fast green staining showed that there was less bony callus in the DF rats. TRAP staining, a marker of osteoclast activity, was previously shown to be high in a rat model of T2DM.Citation33 OCN is a marker of endochondral ossification and Col I is the main component of fibrous collagen in bone matrix, and both are secreted by osteoblasts and are markers of osteoblast activation.Citation12,Citation34 In addition, β-catenin is essential for osteoblast differentiation.Citation35 The transformation from cartilage into bone is the crucial process in fracture healing; this involves the orderly apoptosis of hypertrophic chondrocytes and the transition from chondrocytes to osteoblasts.Citation36–38 In the present study, safranin O/fast green staining showed the presence of more cartilage callus in the DF group, which indicates that apoptosis and the transformation of hypertrophic chondrocytes into osteoblasts in diabetic rats was disrupted, indicating the presence of delayed fracture healing in this animal model. Further analysis using immunohistochemical staining showed that OCN and Col I expression was lower in DF rats, providing molecular evidence for the effect of diabetes on osteogenesis during fracture healing. In summary, the delay of fracture healing in diabetic rats was found to be principally due to the disruption of osteogenesis and the mineralization of cartilage callus.
Insulin treatment improves the bone mineral composition and bone mass of diabetic rats.Citation39 Moreover, insulin treatment increases the BMD of middle-aged male patients with T2DM.Citation40 In the present study, the BMD of diabetic rats was lower. Although one study showed that insulin use was negatively associated with BMD, this finding may have been the result of the presence of other confounding factors.Citation41 In addition to regulating glucose metabolism, insulin increases osteoblast activity and the area of new bone.Citation42 In the present study, the expression of OCN, Col I, and β-catenin was high in the DFI group. In addition, the extent of TRAP staining was lower, suggesting that insulin may also reduce the activity of osteoclasts. This evidence indicates that insulin pretreatment promotes fracture healing in diabetic rats. However, in the clinical context, insulin can only be used therapeutically, and not preventatively.
Liraglutide is an effective therapy for T2DM, and it has also been shown to reduce fracture risk in patients with T2DM in some clinical studies and animal experiments.Citation17,Citation43 However, to date, little research has been undertaken regarding the effects of liraglutide on fracture healing in diabetic rats. Therefore, we hypothesized that liraglutide treatment would promote healing in diabetic rats with bone fracture. We tested this hypothesis by administering liraglutide alone or in combination with insulin to diabetic rats with femoral fracture, and found that both treatments promoted the fracture healing, increased OCN, Col I, and β-catenin expression, and reduced TRAP activity. Similar findings were made in previous studies: liraglutide was found to increase the expression of osteoblast markers and inhibit osteoclastogenesis in ovariectomized rats.Citation17,Citation44 In addition, another GLP-1 receptor agonist, exendin-4, had the same effects.Citation45 More importantly, the combination treatment had superior effects to liraglutide or insulin alone in terms of bone mass, microarchitecture parameters, and histological and immunohistochemical parameters. This set of findings show that combination-treated rats had more mature callus than the DF group or rats treated with single agents. Considering the effects of high serum glucose concentrations on bone cells and thereby the process of fracture healing, the superior effects of combination treatment may be at least in part the result of more stable circulating glucose concentrations in the combination group than in the rats treated with liraglutide or insulin alone. Similar findings were made in the study by Sassenrath et al, which showed that the combination of insulin and a GLP-1RA improves blood glucose control and reduces the dose of insulin required, without increasing the incidence of hypoglycemia.Citation46
To further evaluate the effects of liraglutide on osteoblast function, we pretreated MC3T3-E1 cells with liraglutide and then stimulated them with glucose. The expression of the osteoblast markers BMP2 and SP7 was significantly higher in the liraglutide-treated group. Furthermore, we have provided preliminary data regarding the molecular mechanism whereby liraglutide protects osteoblasts against the effects of a high glucose concentration. We found that the Wnt signaling pathway may be involved in this, because the expression of β-catenin was reduced by high-glucose stimulation, and this was markedly ameliorated by liraglutide treatment, either alone or in combination with insulin. A previous study of the effects of liraglutide on MC3T3-E1 cells also showed effects on β-catenin, which is involved in cell differentiation and proliferation.Citation47 Further study is needed to determine the importance of the canonical Wnt signaling pathway in the protective effects of liraglutide on osteoblasts.
Finally, using the doses and timing stated in the present study, the combination of insulin and liraglutide had superior effects to insulin treatment alone. In the DFIL group, the number of osteoblasts was significantly higher and the number of osteoclasts was significantly lower. In the clinical setting, the combination of insulin and liraglutide may provide additional glycemic control with fewer adverse events in patients with poorly controlled diabetes who are being administered a GLP-1 RA or basal insulin alone.Citation48 In addition, other GLP-1 RAs, such as lixisenatide, in combination with insulin have been shown to have similar therapeutic effects in clinical trials.Citation49 Thus, this strategy may be an effective choice for the treatment of patients with T2DM who experience fracture.
Conclusion
Diabetes causes delayed fracture healing, and numerous factors may be involved in this. We have shown that insulin or liraglutide treatment alone improves fracture healing in diabetic rats. In addition, compared with treatment with insulin or liraglutide alone, a combination of liraglutide and insulin has superior effects to improve fracture healing in diabetic rats. However, the combined use of liraglutide and insulin in patients requires further study, to evaluate its potential applications for the treatment of patients with diabetes and fracture.
Abbreviations
OCN, osteocalcin; Col I, collagen I; BMP2, bone morphogenetic protein-2; T2DM, type 2 diabetes mellitus; GLP-1, glucagon-like peptide-1; DPP-4i, dipeptidyl peptidase-4 inhibitor; BMD, bone mineral density; TB.N, trabecular number; TB.SP, trabecular separation; STZ, streptozotocin; TRAP, tartrate-resistant acid phosphatase staining; ALP, alkaline phosphatase staining.
Data Sharing Statement
The datasets used and analyzed during the current study are available from the corresponding author on reasonable request.
Ethics Approval
All procedures were carried out in compliance with relevant guidelines and regulations for laboratory animal use. The animal study protocol was approved by the Institutional Animal Care and Use Committee of North China University of Science and Technology (LX2021040), Tangshan, China.
Disclosure
The authors report no conflicts of interest in this work.
Acknowledgments
We thank Liwen Bianji (Edanz) (www.liwenbianji.cn) for editing the English text of a draft of this manuscript.
Additional information
Funding
References
- Shaw JE, Sicree RA, Zimmet PZ. Global estimates of the prevalence of diabetes for 2010 and 2030. Diabetes Res Clin Pract. 2010;87(1):4–14. doi:10.1016/j.diabres.2009.10.007
- Sun HX, Lu N, Luo X, Zhao L, Liu JM. Liraglutide, the glucagon-like peptide-1 receptor agonist, has anabolic bone effects in diabetic Goto-Kakizaki rats. J Diabetes. 2015;7(4):584–588. doi:10.1111/1753-0407.12282
- Gortler H, Rusyn J, Godbout C, Chahal J, Schemitsch EH, Nauth A. Diabetes and healing outcomes in lower extremity fractures: a systematic review. Injury. 2018;49(2):177–183. doi:10.1016/j.injury.2017.11.006
- Claes L, Recknagel S, Ignatius A. Fracture healing under healthy and inflammatory conditions. Nat Rev Rheumatol. 2012;8(3):133–143. doi:10.1038/nrrheum.2012.1
- Napoli N, Chandran M, Pierroz DD, et al. Mechanisms of diabetes mellitus-induced bone fragility. Nat Rev Endocrinol. 2017;13(4):208–219. doi:10.1038/nrendo.2016.153
- Koromani F, Ghatan S, van Hoek M, et al. Type 2 diabetes mellitus and vertebral fracture risk. Curr Osteoporos Rep. 2021;19(1):50–57. doi:10.1007/s11914-020-00646-8
- Loi F, Cordova LA, Pajarinen J, Lin TH, Yao Z, Goodman SB. Inflammation, fracture and bone repair. Bone. 2016;86:119–130. doi:10.1016/j.bone.2016.02.020
- Kayal RA, Alblowi J, McKenzie E, et al. Diabetes causes the accelerated loss of cartilage during fracture repair which is reversed by insulin treatment. Bone. 2009;44(2):357–363. doi:10.1016/j.bone.2008.10.042
- Paglia DN, Wey A, Breitbart EA, et al. Effects of local insulin delivery on subperiosteal angiogenesis and mineralized tissue formation during fracture healing. J Orthop Res. 2013;31(5):783–791. doi:10.1002/jor.22288
- Gandhi A, Beam HA, O’Connor JP, Parsons JR, Lin SS. The effects of local insulin delivery on diabetic fracture healing. Bone. 2005;37(4):482–490. doi:10.1016/j.bone.2005.04.039
- Nuche-Berenguer B, Moreno P, Esbrit P, et al. Effect of GLP-1 treatment on bone turnover in normal, type 2 diabetic, and insulin-resistant states. Calcif Tissue Int. 2009;84(6):453–461. doi:10.1007/s00223-009-9220-3
- Su B, Sheng H, Zhang M, et al. Risk of bone fractures associated with glucagon-like peptide-1 receptor agonists’ treatment: a meta-analysis of randomized controlled trials. Endocrine. 2015;48(1):107–115. doi:10.1007/s12020-014-0361-4
- Huang CF, Mao TY, Hwang SJ. The effects of switching from dipeptidyl peptidase-4 Inhibitors to Glucagon-Like Peptide-1 receptor agonists on bone mineral density in diabetic patients. Diabetes Metab Syndr Obes. 2023;16:31–36. doi:10.2147/dmso.S389964
- Yang L, Yang J, Pan T, Zhong X. Liraglutide increases bone formation and inhibits bone resorption in rats with glucocorticoid-induced osteoporosis. J Endocrinol Invest. 2019;42(9):1125–1131. doi:10.1007/s40618-019-01034-5
- Srinivasan K, Viswanad B, Asrat L, Kaul CL, Ramarao P. Combination of high-fat diet-fed and low-dose streptozotocin-treated rat: a model for type 2 diabetes and pharmacological screening. Pharmacol Res. 2005;52(4):313–320. doi:10.1016/j.phrs.2005.05.004
- Cao X, Xu P, Oyola MG, et al. Estrogens stimulate serotonin neurons to inhibit binge-like eating in mice. J Clin Invest. 2014;124(10):4351–4362. doi:10.1172/JCI74726
- Wen B, Zhao L, Zhao H, Wang X. Liraglutide exerts a bone-protective effect in ovariectomized rats with streptozotocin-induced diabetes by inhibiting osteoclastogenesis. Exp Ther Med. 2018;15(6):5077–5083. doi:10.3892/etm.2018.6043
- Bonnarens F, Einhorn TA. Production of a standard closed fracture in laboratory animal bone. J Orthop Res. 1984;2(1):97–101. doi:10.1002/jor.1100020115
- Cao GL, Tian FM, Liu GY, et al. Strontium ranelate combined with insulin is as beneficial as insulin alone in treatment of fracture healing in ovariectomized diabetic rats. Med Sci Monit. 2018;24:6525–6536. doi:10.12659/MSM.911573
- Liu GY, Cao GL, Tian FM, et al. Parathyroid hormone (1-34) promotes fracture healing in ovariectomized rats with type 2 diabetes mellitus. Osteoporos Int. 2017;28(10):3043–3053. doi:10.1007/s00198-017-4148-3
- Schmidmaier G, Wildemann B, Cromme F, Kandziora F, Haas NP, Raschke M. Bone morphogenetic protein-2 coating of titanium implants increases biomechanical strength and accelerates bone remodeling in fracture treatment: a biomechanical and histological study in rats. Bone. 2002;30(6):816–822. doi:10.1016/s8756-3282(02)00740-8
- Miao D, Scutt A. Recruitment, augmentation and apoptosis of rat osteoclasts in 1,25-(OH)2D3 response to short-term treatment with 1,25-dihydroxyvitamin D3 in vivo. BMC Musculoskelet Disord. 2002;3:16. doi:10.1186/1471-2474-3-16
- Shifeng L, Hong X, Xue Y, et al. Ac-SDKP increases alpha-TAT 1 and promotes the apoptosis in lung fibroblasts and epithelial cells double-stimulated with TGF-beta1 and silica. Toxicol Appl Pharmacol. 2019;369:17–29. doi:10.1016/j.taap.2019.02.015
- Komori T. Functions of osteocalcin in bone, pancreas, testis, and muscle. Int J Mol Sci. 2020;21:20. doi:10.3390/ijms21207513
- Depalle B, McGilvery CM, Nobakhti S, Aldegaither N, Shefelbine SJ, Porter AE. Osteopontin regulates type I collagen fibril formation in bone tissue. Acta Bio. 2021;120:194–202. doi:10.1016/j.actbio.2020.04.040
- Duan P, Bonewald LF. The role of the wnt/beta-catenin signaling pathway in formation and maintenance of bone and teeth. Int J Biochem Cell Biol. 2016;77:23–29. doi:10.1016/j.biocel.2016.05.015
- Jiao H, Xiao E, Graves DT. Diabetes and Its Effect on Bone and Fracture Healing. Curr Osteoporos Rep. 2015;13(5):327–335. doi:10.1007/s11914-015-0286-8
- Bai XC, Lu D, Bai J, et al. Oxidative stress inhibits osteoblastic differentiation of bone cells by ERK and NF-kappaB. Biochem Biophys Res Commun. 2004;314(1):197–207. doi:10.1016/j.bbrc.2003.12.073
- Figeac F, Tencerova M, Ali D, et al. Impaired bone fracture healing in type 2 diabetes is caused by defective functions of skeletal progenitor cells. Stem Cells. 2022;40(2):149–164. doi:10.1093/stmcls/sxab011
- Gonzalez Y, Herrera MT, Soldevila G, et al. High glucose concentrations induce TNF-α production through the down-regulation of CD33 in primary human monocytes. BMC Immunol. 2012;13:19. doi:10.1186/1471-2172-13-19
- Picke AK, Gordaliza Alaguero I, Campbell GM, et al. Bone defect regeneration and cortical bone parameters of type 2 diabetic rats are improved by insulin therapy. Bone. 2016;82:108–115. doi:10.1016/j.bone.2015.06.001
- Creecy A, Uppuganti S, Merkel AR, et al. Changes in the fracture resistance of bone with the progression of type 2 diabetes in the ZDSD rat. Calcif Tissue Int. 2016;99(3):289–301. doi:10.1007/s00223-016-0149-z
- Eom YS, Gwon AR, Kwak KM, et al. Protective effects of vildagliptin against pioglitazone-induced bone loss in type 2 diabetic rats. PLoS One. 2016;11(12):e0168569. doi:10.1371/journal.pone.0168569
- Lee NK, Sowa H, Hinoi E, et al. Endocrine regulation of energy metabolism by the skeleton. Cell. 2007;130(3):456–469. doi:10.1016/j.cell.2007.05.047
- Komori T. Regulation of osteoblast differentiation by transcription factors. J Cell Biochem. 2006;99(5):1233–1239. doi:10.1002/jcb.20958
- Julien A, Perrin S, Duchamp de Lageneste O, et al. FGFR3 in periosteal cells drives cartilage-to-bone transformation in bone repair. Stem Cell Rep. 2020;15(4):955–967. doi:10.1016/j.stemcr.2020.08.005
- Kayal RA, Siqueira M, Alblowi J, et al. TNF-alpha mediates diabetes-enhanced chondrocyte apoptosis during fracture healing and stimulates chondrocyte apoptosis through FOXO1. J Bone Miner Res. 2010;25(7):1604–1615. doi:10.1016/j.stemcr.2020.08.005
- Wong SA, Hu DP, Slocum J, et al. Chondrocyte-to-osteoblast transformation in mandibular fracture repair. J Orthop Res. 2021;39(8):1622–1632. doi:10.1002/jor.24904
- Shi P, Hou A, Li C, et al. Continuous subcutaneous insulin infusion ameliorates bone structures and mechanical properties in type 2 diabetic rats by regulating bone remodeling. Bone. 2021;153:116101. doi:10.1016/j.bone.2021.116101
- Hou K, Lin C, Wu B, et al. Exogenetic insulin increased bone mineral density in middle-aged male patients with type 2 diabetes mellitus. Pak J Pharm Sci. 2015;28(6 Suppl):2227–2230.
- de Waard EAC, Driessen JHM, de Jong JJA, et al. The association between insulin use and volumetric bone mineral density, bone micro-architecture and bone strength of the distal radius in patients with type 2 diabetes - The Maastricht study. Bone. 2017;101:156–161. doi:10.1016/j.bone.2017.05.004
- Hou CJ, Liu JL, Li X, Bi LJ. Insulin promotes bone formation in augmented maxillary sinus in diabetic rabbits. Int J Oral Maxillofac Surg. 2012;41(3):400–407. doi:10.1016/j.ijom.2011.10.004
- Gilbert MP, Marre M, Holst JJ, et al. Comparison of the long-term effects of liraglutide and glimepiride monotherapy on bone mineral density in patients with type 2 diabetes. Endocr Pract. 2016;22(4):406–411. doi:10.4158/EP15758.OR
- Lu N, Sun H, Yu J, et al. Glucagon-like peptide-1 receptor agonist Liraglutide has anabolic bone effects in ovariectomized rats without diabetes. PLoS One. 2015;10(7):e0132744. doi:10.1371/journal.pone.0132744
- Ma X, Meng J, Jia M, et al. Exendin-4, a glucagon-like peptide-1 receptor agonist, prevents osteopenia by promoting bone formation and suppressing bone resorption in aged ovariectomized rats. J Bone Miner Res. 2013;28(7):1641–1652. doi:10.1002/jbmr.1898
- Sassenrath K, Phillips BB, Stone RH. Evaluation of GLP-1 receptor agonists in combination with multiple daily insulin injections for type 2 diabetes. J Pharm Pract. 2022;35(6):979–990. doi:10.1177/08971900211010678
- Hou HW, Xue P, Wang Y, Li YK. Liraglutide regulates proliferation, differentiation, and apoptosis of preosteoblasts through a signaling network of Notch/Wnt/Hedgehog signaling pathways. Eur Rev Med Pharmacol Sci. 2020;24(23):12408–12422. doi:10.26355/eurrev_202012_24037
- Harris K, Nealy KL. The clinical use of a fixed-dose combination of insulin degludec and liraglutide (Xultophy 100/3.6) for the treatment of type 2 diabetes. Ann Pharmacother. 2018;52(1):69–77. doi:10.1177/1060028017726348
- Wysham CH, Campos C, Kruger D. Safety and Efficacy of Insulin Degludec/Liraglutide (IDegLira) and Insulin Glargine U100/Lixisenatide (iGlarLixi), two novel co-formulations of a basal insulin and a glucagon-like peptide-1 receptor agonist, in patients with diabetes not adequately controlled on oral antidiabetic medications. Clin Diabetes. 2018;36(2):149–159. doi:10.2337/cd17-0064