Abstract
Background
Parkinson’s disease (PD) is a neurodegenerative disease characterized by motor impairments and resulting from progressive degenerative loss of midbrain dopaminergic (DAergic) neurons in the substantia nigra. Although the main cause of the loss of DAergic neurons is still unknown, various etiopathogenic mechanisms are distinguished, including release and accumulation of endogenous excitotoxic mediators along with the production of oxidative free radicals. Several neurotrophic and growth factors are known to increase DAergic neuronal survival and enhance antioxidant mechanisms. In this context, the micro-immunotherapy (MI) approach consists to regulate the immune system in order to protect DAergic neurons and control oxidative stress.
Purpose
The aim of the present study was to investigate the effect of the MI medicine (MIM), 2LPARK® (Labo’Life), on oxidative stress and on the number of neurons positive for tyrosine hydroxylase (TH), in an in vitro model of PD.
Methods
Rat primary mesencephalic DAergic neurons cultures were pre-treated for 1 hr with the MIM (10 μM and 10 mM), placebo (10 μM and 10 mM) or brain-derived neurotrophic factor (BDNF; 3.3 μM) and then intoxicated with 6-hydroxydopamine (6-OHDA; 20 μM) for 48 hrs. After incubation, cells were incubated 30 mins at 37°C with CellROX green reagent and number of labeled cells were quantified. Then, cells were fixed and incubated with anti-TH antibody and the number of TH+ neurons was evaluated.
Results
We showed that, contrary to placebo, MIM was able to reduce oxidative stress and protect DAergic neurons from 6-OHDA-induced cell death.
Conclusion
Our results demonstrate the in vitro efficacy of MIM on two essential mechanisms of PD and propose the MI approach as a new ally in the regulation of neuroinflammation and in the treatment of this degenerative disease.
Introduction
Parkinson’s disease (PD) is a chronic and progressive, age-related, neurodegenerative disease characterized by tremor, slowness of movement (bradykinesia), postural instability, and rigidity. Other symptoms include depression, mood swings, difficulties with swallowing and chewing, changes in speech, urinary or bowel problems (constipation), and skin and sleep disorders. The prevalence of PD generally increases with age and being male, PD incidence being 1.5 times higher in men than women. The presence of symptoms, such as severe motor impairment, psychosis, and dementia, has been associated with earlier death among people with PD.Citation1
Progressive loss of 60–70% of the dopaminergic (DAergic) neurons in the substantia nigra pars compacta (SNpc) is first reported in patients.Citation2 Actually, PD is histologically characterized by the loss of neurons in the substantia nigra and in some pigmented nuclei of the integuments, gliosis in the same regions, and a larger quantity of Lewy’s bodies.Citation3 Following the loss of dopamine (DA), the chemical messenger responsible for transmitting signals between substantia nigra and striatum, nerve cells in the striatum act out of control, leaving patients unable to direct or control movements in a normal way. However, to date, almost all investigators have agreed that the main cause of the loss of DAergic neurons in the substantia nigra is still unknown.
Nevertheless, although the motor dysfunctions of PD can be primarily attributed to degeneration of the DAergic nigrostriatal system, multiple studies indicate that PD symptomatology extends beyond motor dysfunctions and that PD neuropathology involves disruptions in non-DAergic neurotransmission systems as well as DAergic systems.Citation4
PD is probably a multifactorial disease, both environmental and genetic factors are involved in PD and various etiopathogenic mechanisms are distinguished. At the cellular and molecular level, the current hypothesis points to the release and accumulation of endogenous excitotoxic mediators and to the cytometabolic production of oxidative free radicals (inducing neuronal degeneration). These abnormalities cause cumulative wounds and neuronal damages, promoting premature SNpc DAergic degeneration, abnormal protein modification, and accumulation. In damaged neurons, the excess amount of cytosolic DA outside the synaptic vesicle is metabolized via monoamine oxidase or by auto-oxidation to cytotoxic reactive oxygen species (ROS), suggesting that DA itself may be a source of oxidative stress.Citation5 Oxidative stress is thought to be the common underlying mechanism that leads to cellular dysfunction and eventually to cell death. Mitochondria are the main site of ROS generation within the cell and a mitochondrial respiratory defect can occur in PD.Citation6 Neuronal damages induced by environmental risks or neurotoxic substances, such as 1-methyl-4-phenyl-1,2,5,6 tetrahydropyridine and paraquat, may also contribute to the oxidative stress situation.Citation7,Citation8 Interestingly, toxic agents contribute to a collapse of synaptic vesicle capacity to sequester DA which, not being in their acidic stabilizing environment, enters the cytoplasm where the physiological pH promotes its auto-oxidation and the ensuing production of ROS.Citation9
The neuroinflammatory chain reaction induced by ROS would be responsible for the deterioration of the substantia nigra and DAergic neuronal death. Many different factors (neurotrophic and growth factors, hormones), including brain-derived neurotrophic factor (BDNF),Citation10 glial cell line-derived neurotrophic factor (GDNF),Citation11 erythropoietin (EPO),Citation12 insulin-like growth factor-1 (IGF-1),Citation13 and platelet-derived endothelial cell growth factor (PD-ECGF),Citation14 increase DAergic neuronal survival and enhance antioxidant mechanisms. The stimulation of these factors through micro-immunotherapy (MI) approach could regulate the immune system both in terms of DAergic brainstem neurons protection and regeneration but also in controlling inflammation and oxidative stress.Citation15 MI is a therapeutic strategy which uses immune regulators, including cytokines, in association with nucleic acids (plant-derived total deoxyribonucleic acid [DNA] and ribonucleic acid [RNA]) and specific nucleic acids (SNA®). The active substances, prepared in low doses (LD) and/or ultra-low doses (ULD), are used in sequential formulas of 5 or 10 capsules, developed to treat different acute and chronic diseases.Citation16
6-hydroxydopamine (6-OHDA) is a selective catecholaminergic neurotoxin that is not only used as a pharmacological agent able to trigger PD-like stigmataCitation17,Citation18 but also likely corresponds to a natural DAergic catabolite that accumulates in PD-affected brains and that appears to strongly contribute to this pathology.Citation19 For this reason, 6-OHDA provides a useful model of PD by inducing neurodegeneration of DAergic neurons in vitro.
In this study, we tested the effect of one capsule of the MI medicine (MIM) 2LPARK® (Labo’Life) on the oxidative stress and neuronal survival in an in vitro model of 6-OHDA-induced PD.
Materials and methods
DAergic neuron cell cultures
The experimental procedures were carried out in accordance to European guidelines for the care and use of laboratory animals (Directive 2010/63/UE). Every effort was done to minimize animal suffering and reduce the number of animals used in the experiments. The animals, which were used to prepare mesencephalic cell cultures, were naive to previous administration of drugs.
The study was performed in France according to the decree 2013–118 of February 1, 2013 on the protection of animal used for scientific purposes (https://www.recherche-animale.org/sites/default/files/decret_2013-118.pdf). The pregnant rats were received at the animal house at the latest the day before the sacrifice and they were killed according to the authorized procedures. The nervous tissues of interest were then recovered from the embryos in order to put them in culture.
Rat DAergic neurons were cultured as described by Schinelli et al.Citation20 Briefly pregnant female rats (Rats Wistar; Janvier) of 15 days’ gestation were killed by cervical dislocation and the fetuses were removed from the uterus. The embryonic midbrains were removed and placed in ice-cold medium of Leibovitz 15 (L15; PanBiotech, Aidenbach, Germany) containing 2% of Penicillin-Streptomycin (PS; PanBiotech, Aidenbach, Germany) and 1% of bovine serum albumin (BSA; PanBiotech, Aidenbach, Germany). Only the ventral portions of the mesencephalic flexure were used for the cell preparations as this region of the developing brain is rich in DAergic neurons. The midbrains were dissociated by trypsinization for 20 mins at 37°C (Trypsin EDTA 1X; PanBiotech, Aidenbach, Germany). The reaction was stopped by the addition of Dulbecco’s modified Eagle’s medium (DMEM; PanBiotech, Aidenbach, Germany) containing DNase I grade II (0.1 mg/mL; PanBiotech, Aidenbach, Germany) and 10% of fetal calf serum (FCS; Invitrogen, California, USA). Cells were then mechanically dissociated by 3 passages through a 10-mL pipette. Cells were then centrifuged at 180 x g for 10 mins at +4°C on a layer of BSA (3.5%) in L15 medium. The supernatant was discarded and the cell pellets were re-suspended in a defined culture medium consisting of Neurobasal (Invitrogen, California, USA) supplemented with B27 2% (Invitrogen, California, USA), L-glutamine (2 mM; PanBiotech, Aidenbach, Germany) and 2% of PS, 10 ng/mL of BDNF (PeproTech, Neuilly-Sur-Seine, France), and 1 ng/mL of GDNF (PanBiotech, Aidenbach, Germany). Viable cells were counted in a Neubauer cytometer using the trypan blue exclusion test. The cells were seeded at a density of 40,000 cells/well in 96-well plates (pre-coated with poly-D-lysine; Greiner, Kremsmünster, Austria) and were cultured at 37°C in a humidified air (95%)/CO2 (5%) atmosphere. Half of the medium was changed every 2 days with fresh medium. In these conditions, after 5 days of culture, astrocytes are present in the culture and release growth factor allowing neurons differentiation. On day 6 of culture, cells were pre-treated for 1 hr with MIM (2LPARK®; 10 μM and 10 mM), or placebo (10 μM and 10 mM), or BDNF (3.3 μM), and then intoxicated with 6-OHDA (20 μM) for 48 hrs. For each set of experiments, one culture was done with 6 wells per condition. One plate was done for CellRox imaging and one plate to assess DAergic neuron survival.
Investigational product
The MIM is manufactured by Labo’Life Belgium and notified to the Belgian Federal Agency for Medicines and Health Products (FAMHP) under notification number 1507CH173F1. MI medicines consist of lactose-saccharose globules for oromucosal administration, impregnated with ethanolic preparations of immune mediators and nucleic acids at LD and/or ULD. LD and ULD of active substances are obtained through a “sequential kinetic process”, consisting of a 1/100 dilution process followed by vertical shaking, reproduced a defined number of times. Medicines composition is expressed as CH (Centesimal Hahnemannian dilutions), and it indicates the number of times by which the two proceedings are carried out for each active substance. Being sequentially developed medicines to transmit consecutive information to the body, the content of each capsule is specific, and the intake should follow the ascending order indicated on the blister. The composition of the MIM tested capsule is showed in . The placebo and MIM lactose-saccharose globules concentration as well as BDNF concentration are shown in .
Table 1 Composition of the MIM tested capsule (Labo’Life Belgium, Les Isnes, Belgium)
Table 2 Concentrations of the tested compounds
Measure of oxidative stress, number of tyrosine hydroxylase (TH) positive neurons and neurite length
In order to investigate the protective effect of the MIM on oxidative stress, cultures of primary neurons were pre-treated during 1 hr with MIM (10 μM and 10 mM) or with its placebo (10 μM and 10 mM) and then injured by 6-OHDA (20 μM) for 48 hrs. After 48 hrs of incubation, cells were incubated 30 mins at 37°C with CellROX green reagent (Invitrogen, California, USA) that is nonfluorescent in a reduced state and fluoresces bright green upon oxidation. 4',6-diamidino-2-phenylindole (DAPI) incubation was done in the same time at 1/5000 (Sigma-Aldrich, Missouri, USA). Twelve images per well were taken using InCell AnalyzerTM 2200 (GE Healthcare, La Chapelle-sur-Erdre, France) with 20x magnification. All images were taken under the same condition. Number of cells labeled with CellROX green reagent and DAPI were quantified. Then, cells were fixed by a solution of 4% paraformaldehyde (Sigma-Aldrich, Missouri, USA) for 20 mins at room temperature. The cells were then permeabilized and non-specific sites were blocked with a solution of phosphate-buffered saline (PBS; PanBiotech; Aidenbach, Germany) containing 0.1% of saponin (Sigma-Aldrich, Missouri, USA) and 1% fetal calf serum (FCS, Invitrogen, California, USA) for 15 mins at room temperature.
In order to study the preventive effect of the MIM on DAergic neurons survival, DAergic neurons culture was intoxicated for 48 hrs with 6-OHDA (20 μM) after a pre-treatment (1 hr) with MIM (10 μM and 10 mM) or its placebo or BDNF (3.3 μM). Differentiated neuronal cultures contained DAergic cells that were specifically identified by staining TH, the rate-limiting enzyme in DAergic synthesis.Citation21 Cells were incubated with monoclonal anti-TH antibody produced in mouse (antibodies-Sigma-Aldrich, Missouri, USA) and in PBS containing 1% FCS, 0.1% saponin, overnight at 4°C.
Monoclonal anti-TH antibody was revealed with Alexa Fluor 568 anti-mouse (Molecular probe, Oregon, USA) in PBS with 1% FCS, 0.1% saponin, for 1 hr at room temperature.
For DAergic neuron survival quantification, 12 pictures per well were taken by using InCell AnalyzerTM 2200 (GE Healthcare, La Chapelle-sur-Erdre, France) with 20x magnification.
Analysis of neurite length of TH positive neurons was performed using Developer software (GE healthcare, La Chapelle-sur-Erdre, France).
Statistical analysis
Statistical analyses have been performed using GraphPad Prism version 7.0 for MacOS (GraphPad Software, La Jolla, CA, USA). The data were expressed as mean±SEM (of 6 data per condition, 1 culture). A global analysis of the data was performed using a one-way analysis of variance (ANOVA) following by Dunnett’s test. Bonferroni’s post hoc test was used to analyze differences between MIM (10 μM and 10 mM) vs placebo (10 μM and 10 mM) and MIM (10 μM and 10 mM) vs BDNF (3.3 μM). The level of significance is set at p<0.05.
Results
MIM protects against oxidative stress in an in vitro model of 6-OHDA-induced stress
We showed that 6-OHDA induced an increase in oxidative stress (). Being able to reduce the 6-OHDA-induced neurodegeneration in vitro,Citation22 BDNF (3.3 μM; pre-treatment for 1 hr before 6-OHDA-induced stress) was used to validate our experimental conditions. We found that placebo did not protect neurons against oxidative stress, whereas MIM was able to reverse 6-OHDA injury at the tested concentrations, in a statistically significant manner. Moreover, MIM at 10 mM had a significantly greater effect than BDNF used in the current study as a positive control (control, placebo [10 μM and 10 mM], MIM [10 μM and 10 mM] and BDNF [3.3 μM] vs 6-OHDA [20 μM], one-way ANOVA followed by Dunnett’s test: p=0.0001, and p<0.0001, . Placebo [10 μM] vs MIM [10 μM], Bonferroni’s multiple comparison test: p=0.0016, ; placebo [10 mM] vs MIM [10 mM] and MIM [10 mM] vs BDNF [3.3 μM], Bonferroni’s multiple comparison test: p<0.0001 and p=0.036, respectively). Representative pictures of DAergic neurons staining with CellRox reagent are reported in .
Figure 1 Action of MIM on oxidative stress. Cultures of primary neurons treated for 1 hr with MIM and its placebo at (A) 10 μM and (B) 10 mM or BDNF (3.3 μM) and then injured by 6-OHDA (20 μM) for 48 hrs (results expressed as percentage of control). 6-OHDA induced an increase of oxidative stress ((A) 171.30% and (B) 158.44% of the control). BDNF (3.3 μM; positive control) inverted the effect of 6-OHDA ((A) 117.79% and (B) 112.13% of the control). MIM prevented 6-OHDA injury ((A) 109.17% and (B) 74.21% of the control). Quantification represents mean±S.D. of 6 wells per condition ((A) n=2 and (B) n=1); *p<0.05; **p<0.01; ***p<0.005; control, placebo (10 μM and 10 mM), MIM (10 μM and 10 mM), and BDNF (3.3 μM) vs 6-OHDA (20 μM); one-way ANOVA followed by Dunnett’s test; placebo (10 μM and 10 mM) vs MIM (10 μM and 10 mM); MIM (10 μM and 10 mM) vs BDNF (3.3 μM); Bonferroni’s multiple comparison test. Abbreviations: 6-OHDA, 6-hydroxydopamine; MIM, micro-immunotherapy medicine; BDNF, brain-derived neutrophic factor.
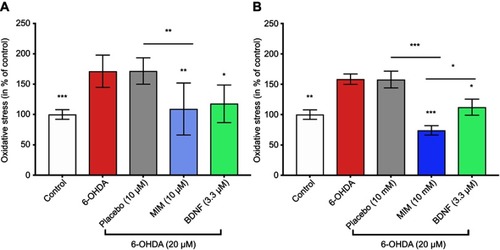
MIM increases DAergic neurons survival in an in vitro model of 6-OHDA-toxicity
We showed that 6-OHDA induced a large and significant decrease of TH positive neurons and that the pre-treatment with BDNF (3.3 μM) displayed a protective effect against 6-OHDA injury (see ). These results validated the model. We found that DAergic neurons survival was significantly increased in 6-OHDA cultures treated with MIM (10 μM and 10 mM) compared to placebo (control, placebo [10 μM and 10 mM], MIM [10 μM and 10 mM], and BDNF [3.3 μM] vs 6-OHDA [20 μM], one-way ANOVA followed by Dunnett’s test: p<0.0001 ( and ). Placebo [10 μM] vs MIM [10 μM], Bonferroni’s multiple comparison test: p=0.0002 (); placebo [10 mM] vs MIM [10 mM], Bonferroni’s multiple comparison test: p=0.0008 (). Representative pictures of DAergic neurons staining with TH antibody are reported in . 6-OHDA (20 μM for 48 hrs) induced a large and significant decrease of neurite length and BDNF (3.3 μM) displayed a protective effect against 6-OHDA injury. These results validated the model. Finally, MIM (10 μM and 10 mM) showed a statistically protective effect on neurite length against 6-OHDA compared to placebo (control, placebo [10 μM and 10 mM], MIM [10 μM and 10 mM], and BDNF [3.3 μM] vs 6-OHDA [20 μM], one-way ANOVA followed by Dunnett’s test: p<0.0001. Placebo [10 μM] vs MIM [10 μM], Bonferroni’s multiple comparison test: p=0.018; placebo [10 mM] vs MIM [10 mM], Bonferroni’s multiple comparison test: p<0.0147; ).
Figure 3 Action of MIM on neurons survival. Cultures of primary neurons treated for 1 hr with MIM and its placebo at (A) 10 μM and (B) 10 mM or BDNF (3.3 μM) and then injured by 6-OHDA (20 μM) for 48 hrs (results expressed as percentage of control). 6-OHDA induced a decrease of neuronal survival ((A) 65.32% and (B) 64.55% of the control). BDNF (3.3 μM; positive control) reduced 6-OHDA-induced cell-death ((A) 93.16% and (B) 91.26% of the control). MIM prevented 6-OHDA injury ((A) 89.84% and (B) 97.61% of the control). Quantification represents mean±S.D. of 6 wells per condition ((A) n=3 and (B) n=2); *p<0.05, **p<0.01, ***p<0.005, #p<0.0001; control, placebo (10 μM and 10 mM), MIM (10 μM and 10 mM) and BDNF (3.3 μM) vs 6-OHDA (20 μM); one-way ANOVA followed by Dunnett’s test; placebo (10 μM and 10 mM) vs MIM (10 μM and 10 mM); MIM (10 μM and 10 mM) vs BDNF (3.3 μM); Bonferroni’s multiple comparison test.
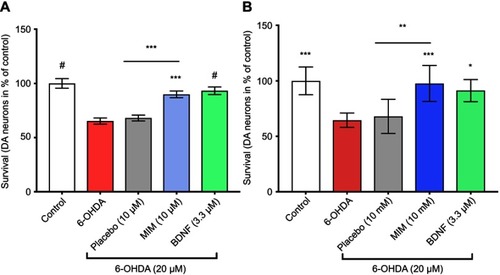
Discussion
The causes of PD are still unknown. However, although several genetic mutations responsible for 10–15% of cases of PD have been identified,Citation23,Citation24 it is hypothesized that the interaction of genetic and environmental factors is necessary to determine its onset.Citation25,Citation26 The hypothesized mechanisms involve oxidative stress, inflammation, abnormal accumulation of protein aggregates, trophic factor deficiency, apoptosis, and premature death of DAergic neurons.
Using LD and ULD of specific substances following a physiological sequence, MI aims at regulating the immune system, both in terms of protection and regeneration of DAergic neurons, but also in a way of controlling inflammation and oxidative stress. The immune substances contained in MI formulas are prepared in low concentrations through a “sequential kinetic process”, consisting of a 1/100 dilution process followed by vertical shaking, reproduced a defined number of times, thereby guaranteeing high tolerance. Furthermore, depending on whether stimulation, modulation, or inhibition on the organism is desired, the levels of concentration used can vary within the same formula. In the current study, the in vitro PD model demonstrated that the LD of neurotrophic and growth factors, contained in the MIM capsule (see ), were able to protect DAergic neurons from the cell death induced by 6-OHDA and to reduce oxidative stress.
Neurotrophic factors play essential roles for survival, protection, and maintenance of neurons. The alteration of their expression and signaling pathways seems to be linked to the development and/or progression of neurodegenerative disorders, including PD.
Early studies demonstrated that BDNF supports the survival of nigral DAergic neurons in the ventral tegmental area and medial SNpc,Citation10 and the expression of BDNF protein and messenger RNA (mRNA) is reduced in nigral neurons in PD.Citation27 Moreover, treatment with BDNF in rodent models prevented the loss of DAergic neurons in the substantia nigra after 6-OHDA or 1-methyl-4-phenylpyridinium (MPP)-induced lesions.Citation28,Citation29 BDNF neurotrophic functions also include the development, differentiation of cerebellar granule and Purkinje cellsCitation30 and nigrostriatal apoptosis inhibition by BDNF/Tropomyosin receptor kinase B (TrkB) signaling.Citation31 Moreover, the expressions of DA D3 receptor and TH are mediated by BDNF.Citation32
Similar to BDNF, GDNF has an important role in the survival, differentiation, organization, and maintenance of DAergic neurons.Citation33,Citation34 GDNF is released by glial cells, and it has been considered as the most promising drug in the treatment of animal models of PD,Citation35 preserving neuronal survival under stress conditions and inhibiting apoptotic cell death in DAergic neurons.Citation36
However, it has been showed that high concentrations of DA in a weak neuroprotective status blunt the activity of neurotrophic factors, thus contributing to the vulnerability of the nigral DAergic neurons.Citation37,Citation38
One of the neurotrophic factors that might protect neurons in this deleterious microenvironment is IGF-1. The substantia nigra is one of the brain regions with highest density of IGF-1 receptors,Citation39 and IGF-1 has been found to increase survival of DAergic neurons and to protect DAergic neurons from DA-induced neurotoxicity both in vitro and in animal models of PD.Citation13,Citation40
The neurotrophic action of PD-ECGF on the development and regeneration in the central nervous system (CNS) has also been shown in embryonic rat cortical neurons cultures.Citation14 Furthermore, it has been proved that gliostatin, a factor chemically and biologically identical to PD-ECGF, promotes the survival of neurons in vitro, playing a neutrophilic action on cortical neuronsCitation14 and a physiological role in neuronal and glial development.Citation41
Neurotrophic and neuroprotective actions are also performed by EPO, a well-known cytokine produced in CNS.Citation42 Several authors address the neural protective effects of EPO in experimental PD models. In these studies, EPO reversed behavioral deficits,Citation43 attenuated inflammation, reduced DAergic neuronal cell loss,Citation44 and exerts anti-apoptotic effects.Citation45 Moreover, the protective, anti-inflammatory, antioxidant, and restorative effects of EPO in a rotenone-induced neurodegenerative animal model were reported by Erbaş et al.Citation46
Several studies have examined the therapeutic efficacy of neurotrophins in animal models of PD. In one initial study the co-transplantation of GDNF overexpressing neural stem cells (NSCs) with primary DAergic neurons in a rat 6-OHDA lesion model of PD, resulted in significantly increased survival of co-transplanted neurons.Citation47 The ability of fetal-derived human NSCs overexpressing various neurotrophins (including IGF-1 or GDNF) to protect DAergic neurons and rescue motor deficits rat lesion model of PD was also investigated.Citation48
During the last years, several in vivo/in vitro studies and clinical trials have reported the effectiveness and safety of the therapeutic intervention based on the oral administration of LD of signaling molecules in different pathological conditions, including inflammation and oxidative stress.Citation49,Citation50 The proposed action mechanism of LD cytokines, hormones, neuropeptides, and growth factors involves the activation of cellular and plasmatic receptors by virtue of their LD. This process restores the immunological homeostasis by stimulating a pathologically impaired cellular pathway, using the same factors physiologically involved in the cellular signaling. The neurotrophic and growth factors of the MIM tested capsule point to increase the survival and regeneration of DAergic neurons, limiting inflammation and reducing oxidative stress, proposing a valid and safe approach to the treatment of PD.
Conclusion
This study showed the positive effect of a pre-treatment with MIM on cultures of primary neurons injured by 6-OHDA, demonstrating the in vitro efficacy of MIM in the regulation of oxidative stress and neuronal survival in an in vitro model of PD. Nevertheless, further pre-clinical and clinical studies are required to demonstrate the efficacy of MIM medicine in PD patients.
Acknowledgments
We would like to thanks Dr Alain Lejeune from Labo’Life Belgium for having provided MIM capsules and Mrs Anne Naedts from Labo’Life Belgium for having prepared and provided the placebo globules. We are grateful to Dr Ilaria Floris for reviewing the manuscript.
Disclosure
NLL works for Labo’Life France, the company service provider for Labo’Life, specializing in preclinical, clinical, and regulatory affairs. NLL reports personal fees during the conduct of the study and outside the submitted work from Labo'Life France. BL is employed by Labo’Life Belgium, the pharmaceutical company that commercializes the micro-immunotherapy medicine tested in the study. BL reports personal fees during the conduct of the study and outside the submitted work from Labo'Life Belgium. NLL and BL declare that their professional relationships with Labo’Life France and Labo’Life Belgium do not imply any misconduct in this study. DR and SR work for Syncrosome, a contract research organization that specializes in preclinical research. The authors report no other conflicts of interest in this work.
Supplementary material
Figure S1 Action of MIM on neurite length. Cultures of primary neurons treated for 1 hr with MIM (10 μM and 10 mM) and its placebo (10 μM and 10 mM) or BDNF (3.3 μM) and then injured by 6-OHDA (20 μM) for 48 hrs (results expressed as percentage of control). 6-OHDA induced a decrease of neurite length (67.98% of the control). BDNF (3.3 μM; positive control) reduced 6-OHDA-induced neurite length reduction (102.05% of the control). MIM prevented 6-OHDA -induced neurite length reduction (MIM 10 μM 99.37% and MIM 10 mM 100.5% of the control). Quantification represents mean±S.D. of 6 wells per condition (n=2); *p<0.05, **p<0.01, ***p<0.005, # p<0.0001; control, placebo (10 μM and 10 mM), MIM (10 μM and 10 mM), and BDNF (3.3 μM) vs 6-OHDA (20 μM); one-way ANOVA followed by Dunnett’s test; placebo (10 μM and 10 mM) vs MIM (10 μM and 10 mM); MIM (10 μM and 10 mM) vs BDNF (3.3 μM); Bonferroni’s multiple comparison test.
Abbreviations: 6-OHDA, 6-hydroxydopamine; MIM, micro-immunotherapy medicine; BDNF, brain-derived neutrophic factor.
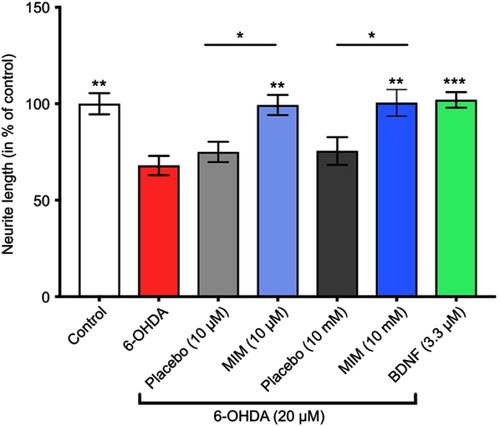
References
- Forsaa EB, Larsen JP, Wentzel-Larsen T, Alves G. What predicts mortality in Parkinson disease? A prospective population-based long-term study. Neurology. 2010;75(14):1270–1276. doi:10.1212/WNL.0b013e3181f6131120921512
- Goswami P, Joshi N, Singh S. Neurodegenerative signaling factors and mechanisms in Parkinson’s pathology. Toxicol In Vitro. 2017;43:104–112. doi:10.1016/j.tiv.2017.06.00828627426
- Rajput AH, Offord KP, Beard CM, Kurland LT. Epidemiology of parkinsonism: incidence, classification, and mortality. Ann Neurol. 1984;16(3):278–282. doi:10.1002/ana.4101603036333204
- Barone P. Neurotransmission in Parkinson’s disease: beyond dopamine. Eur J Neurol. 2010;17(3):364–376. doi:10.1111/j.1468-1331.2009.02900.x20050885
- Segura‐Aguilar J, Paris I, Muñoz P, Ferrari E, Zecca L, Zucca FA. Protective and toxic roles of dopamine in Parkinson’s disease. J Neurochem. 2014;129(6):898–915. doi:10.1111/jnc.1268624548101
- Brieger K, Schiavone S, Miller FJ Jr, Krause KH. Reactive oxygen species: from health to disease. Swiss Med Wkly. 2012;142:w13659.22903797
- Choi Y, Cho K, Yoon S, Lee H, Choi Y. A case of paraquat intoxication caused by intravenous injection. Am J Emerg Med. 2008;26(7):836–e3. doi:10.1016/j.ajem.2007.12.016
- Langston JW, Ballard PA Jr. Parkinson’s disease in a chemist working with l-methyl-4-phenyl-l, 2, 5, 6-tetrahydropyridine. N Engl J Med. 1983;309(5):310.
- Lotharius J, O’Malley KL. The parkinsonism-inducing drug 1-methyl-4-phenylpyridinium triggers intracellular dopamine oxidation A novel mechanism of toxicity. J Biol Chem. 2000;275(49):38581–38588. doi:10.1074/jbc.M00538520010969076
- Baquet ZC, Bickford PC, Jones KR. Brain-derived neurotrophic factor is required for the establishment of the proper number of dopaminergic neurons in the substantia nigra pars compacta. J Neurosci. 2005;25(26):6251–6259. doi:10.1523/JNEUROSCI.4601-04.200515987955
- Gash DM, Zhang Z, Ai Y, Grondin R, Coffey R, Gerhardt GA. Trophic factor distribution predicts functional recovery in parkinsonian monkeys. Ann Neurol. 2005;58(2):224–233. doi:10.1002/ana.2054916049934
- Demers EJ, McPherson RJ, Juul SE. Erythropoietin protects dopaminergic neurons and improves neurobehavioral outcomes in juvenile rats after neonatal hypoxia-ischemia. Pediatr Res. 2005;58(2):297. doi:10.1203/01.pdr.0000183658.17854.2816055937
- Offen D, Shtaif B, Hadad D, Weizman A, Melamed E, Gil-Ad I. Protective effect of insulin-like-growth-factor-1 against dopamine-induced neurotoxicity in human and rodent neuronal cultures: possible implications for Parkinson’s disease. Neurosci Lett. 2001;316(3):129–132.11744219
- Asai K, Nakanishi K, Isobe I, et al. Neurotrophic action of gliostatin on cortical neurons. Identity of gliostatin and platelet-derived endothelial cell growth factor. J Biol Chem. 1992;267(28):20311–20316.1400349
- Thomas G, Cluzel H, Lafon J, Bruhwyler J, Lejeune B. Efficacy of 2LPAPI®, a micro-immunotherapy drug, in patients with high-risk papillomavirus genital infection. AID. 2016;6(01):7–14. doi:10.4236/aid.2016.61002
- Floris I, Lechner J, Lejeune B. Follow-up of patients with systemic immunological diseases undergoing fatty-degenerative osteolysis of the jawbone surgery and treated with RANTES 27CH. J Biol Regul Homeost Agents. 2018;32(1):37–45.
- Sauer H, Oertel WH. Progressive degeneration of nigrostriatal dopamine neurons following intrastriatal terminal lesions with 6-hydroxydopamine: a combined retrograde tracing and immunocytochemical study in the rat. Neuroscience. 1994;59(2):401–415.7516500
- Cass WA, Harned ME, Bailey SL. Enhanced effects of 6-hydroxydopamine on evoked overflow of striatal dopamine in aged rats. Brain Res. 2002;938(1–2):29–37.12031532
- Jellinger K, Linert L, Kienzl E, Herlinger E, Youdim MB. Chemical evidence for 6-hydroxydopamine to be an endogenous toxic factor in the pathogenesis of Parkinson’s disease. J Neural Transm Suppl. 1995;46:297–314.8821067
- Schinelli S, Zuddas A, Kopin IJ, Barker JL, Di Porzio U. 1‐Methyl‐4‐Phenyl‐1, 2, 3, 6‐Tetrahydropyridine metabolism and l‐methyl‐4‐phenylpyridinium uptake in dissociated cell cultures from the embryonic mesencephalon. J Neurochem. 1988;50(6):1900–1907.3259619
- Kuhar MJ, Couceyro PR, Lambert PD. Catecholamines. Basic Neurochem. 1999;243–261.
- Chen G, Bower KA, Ma C, Fang S, Thiele CJ, Luo JIA. Glycogen synthase kinase 3β (GSK3β) mediates 6-hydroxydopamine-induced neuronal death. FASEB J. 2004;18(10):1162–1164. doi:10.1096/fj.04-1551fje15132987
- Ascherio A, Schwarzschild MA. The epidemiology of Parkinson’s disease: risk factors and prevention. Lancet Neurol. 2016;15(12):1257–1272. doi:10.1016/S1474-4422(16)30230-727751556
- Klein C, Westenberger A. Genetics of Parkinson’s disease. Cold Spring Harb Perspect Med. 2012;2(1):a008888. doi:10.1101/cshperspect.a00888822315721
- Fleming SM. Mechanisms of gene-environment interactions in Parkinson’s disease. Curr Environ Health Rep. 2017;4(2):192–199. doi:10.1007/s40572-017-0143-228417442
- Delamarre A, Meissner WG. Epidemiology, environmental risk factors and genetics of Parkinson’s disease. Presse Med. 2017;46(2 Pt 1):175–181. doi:10.1016/j.lpm.2017.01.00128189372
- Parain K, Murer MG, Yan Q, et al. Reduced expression of brain-derived neurotrophic factor protein in Parkinson’s disease substantia nigra. Neuroreport. 1999;10(3):557–561.10208589
- Frim DM, Uhler TA, Galpern WR, Beal MF, Breakefield XO, Isacson O. Implanted fibroblasts genetically engineered to produce brain-derived neurotrophic factor prevent 1-methyl-4-phenylpyridinium toxicity to dopaminergic neurons in the rat. Proc Natl Acad Sci U S A. 1994;91(11):5104–5108. doi:10.1073/pnas.91.11.51048197193
- Levivier M, Przedborski S, Bencsics C, Kang UJ. Intrastriatal implantation of fibroblasts genetically engineered to produce brain-derived neurotrophic factor prevents degeneration of dopaminergic neurons in a rat model of Parkinson’s disease. J Neurosci. 1995;15(12):7810–7820.8613721
- Schwartz PM, Borghesani PR, Levy RL, Pomeroy SL, Segal RA. Abnormal cerebellar development and foliation in BDNF−/− mice reveals a role for neurotrophins in CNS patterning. Neuron. 1997;19(2):269–281.9292718
- Lui NP, Chen LW, Yung WH, Chan YS, Yung KKL. Endogenous repair by the activation of cell survival signalling cascades during the early stages of rat Parkinsonism. PLoS One. 2012;7(12):e51294. doi:10.1371/journal.pone.005129423251488
- Guillin O, Diaz J, Carroll P, Griffon N, Schwartz JC, Sokoloff P. BDNF controls dopamine D3 receptor expression and triggers behavioural sensitization. Nature. 2001;411(6833):86. doi:10.1038/3507507611333982
- Chermenina M, Schouten P, Nevalainen N, Johansson F, Orädd G, Strömberg I. GDNF is important for striatal organization and maintenance of dopamine neurons grown in the presence of the striatum. Neuroscience. 2014;270:1–11. doi:10.1016/j.neuroscience.2014.04.00824726488
- Henderson CE, Phillips HS, Pollock RA, et al. GDNF: a potent survival factor for motoneurons present in peripheral nerve and muscle. Science. 1994;266(5187):1062–1064.7973664
- Hong M, Mukhida K, Mendez I. GDNF therapy for Parkinson’s disease. Expert Rev Neurother. 2008;8(7):1125–1139. doi:10.1586/14737175.8.7.112518590482
- Clarkson ED, Zawada WM, Freed CR. GDNF reduces apoptosis in dopaminergic neurons in vitro. Neuroreport. 1995;7(1):145–149.8742438
- Offen D, Ziv I, Sternin H, Melamed E, Hochman A. Prevention of dopamine-induced cell death by thiol antioxidants: possible implications for treatment of Parkinson’s disease. Exp Neurol. 1996;141(1):32–39. doi:10.1006/exnr.1996.01368797665
- Ziv I, Melamed E, Nardi N, et al. Dopamine induces apoptosis-like cell death in cultured chick sympathetic neurons-a possible novel pathogenetic mechanism in Parkinson’s disease. Neurosci Lett. 1994;170(1):136–140.8041491
- Quesada A, Romeo HE, Micevych P. Distribution and localization patterns of estrogen receptor‐β and insulin‐like growth factor‐1 receptors in neurons and glial cells of the female rat substantia nigra: localization of ERβ and IGF‐1R in substantia nigra. J Comp Neurol. 2007;503(1):198–208. doi:10.1002/cne.2135817480015
- Kao SY. Rescue of α-synuclein cytotoxicity by insulin-like growth factors. Biochem Biophys Res Commun. 2000;385(3):434–438. doi:10.1016/j.bbrc.2009.05.089
- Ueki T, Nakanishi K, Asai K, et al. Neurotrophic action of gliostatin on cocultured neurons with glial cells. Brain Res. 1993;622(1–2):299–302.8242371
- Jelkmann W. Regulation of erythropoietin production. J Physiol. 2011;589(6):1251–1258. doi:10.1113/jphysiol.2010.19505721078592
- Signore AP, Weng Z, Hastings T, et al. Erythropoietin protects against 6‐hydroxydopamine‐induced dopaminergic cell death. J Neurochem. 2006;96(2):428–443. doi:10.1111/j.1471-4159.2005.03587.x16336625
- Xue YQ, Zhao LR, Guo WP, Duan WM. Intrastriatal administration of erythropoietin protects dopaminergic neurons and improves neurobehavioral outcome in a rat model of Parkinson’s disease. Neuroscience. 2007;146(3):1245–1258. doi:10.1016/j.neuroscience.2007.02.00417363174
- Kadota T, Shingo T, Yasuhara T, et al. Continuous intraventricular infusion of erythropoietin exerts neuroprotective/rescue effects upon Parkinson’s disease model of rats with enhanced neurogenesis. Brain Res. 2009;1254:120–127. doi:10.1016/j.brainres.2008.11.09419101524
- Erbaş O, Çınar BP, Solmaz V, Çavuşoğlu T, Ateş U. The neuroprotective effect of erythropoietin on experimental Parkinson model in rats. Neuropeptides. 2015;49:1–5. doi:10.1016/j.npep.2014.10.00325464888
- Ostenfeld T, Tai YT, Martin P, Déglon N, Aebischer P, Svendsen CN. Neurospheres modified to produce glial cell line‐derived neurotrophic factor increase the survival of transplanted dopamine neurons. J Neurosci Res. 2002;69(6):955–965. doi:10.1002/jnr.1039612205689
- Ebert AD, Barber AE, Heins BM, Svendsen CN. Ex vivo delivery of GDNF maintains motor function and prevents neuronal loss in a transgenic mouse model of Huntington’s disease. Exp Neurol. 2010;224(1):155–162. doi:10.1016/j.expneurol.2010.03.00520227407
- Barygina V, Becatti M, Lotti T, Taddei N, Fiorillo C. Low dose cytokines reduce oxidative stress in primary lesional fibroblasts obtained from psoriatic patients. J Dermatol Sci. 2016;83(3):242–244. doi:10.1016/j.jdermsci.2016.06.00227317477
- Mancini F, Milardi D, Carfagna P, et al. Low-dose SKA progesterone and Interleukin-10 modulate the inflammatory pathway in endometriotic cell lines. Int Immunopharmacol. 2018;55:223–230. doi:10.1016/j.intimp.2017.12.00829272819