Abstract
Normal function of the vestibulo-ocular reflex (VOR) coordinates eye movement with head movement, in order to provide clear vision during motion and maintain balance. VOR is generated within the semicircular canals of the inner ear to elicit compensatory eye movements, which maintain stability of images on the fovea during brief, rapid head motion, otherwise known as gaze stability. Normal VOR function is necessary in carrying out activities of daily living (eg, walking and riding in a car) and is of particular importance in higher demand activities (eg, sports-related activities). Disruption or damage in the VOR can result in symptoms such as movement-related dizziness, blurry vision, difficulty maintaining balance with head movements, and even nausea. Dizziness is one of the most common symptoms following traumatic brain injury (TBI) and is considered a risk factor for a prolonged recovery. Assessment of the vestibular system is of particular importance following TBI, in conjunction with oculomotor control, due to the intrinsic neural circuitry that exists between the ocular and vestibular systems. The purpose of this article is to review the physiology of the VOR and the visual-vestibular symptoms associated with TBI and to discuss assessment and treatment guidelines for TBI. Current challenges and future prospects will also be addressed.
Introduction
Vestibular symptoms can occur immediately following traumatic brain injury (TBI) and can be some of the most problematic sequelae, which contribute to a prolonged recovery.Citation1–Citation3 Assessment of the vestibular system post TBI generally focuses on sensory organization or balance testing,Citation4–Citation7 while testing the vestibulo-ocular reflex (VOR) continues to be largely overlooked. The literature is particularly sparse in incorporating VOR exercises into a rehabilitation program post injury, or articulating the importance of peripheral versus central vestibular involvement.Citation8 VOR originates from the semicircular canals (SCCs) of the inner ear to elicit compensatory eye movements that stabilize images on the fovea during brief and rapid head motion. The compensatory eye movements involved in gaze stability ensure clear vision during angular head motion and non-angular head movement, which are necessary for activities of daily living (ADL) as well as higher demand activities associated with sports. VOR is vulnerable to disruption and even damage by mild TBI, resulting in symptoms such as movement-related dizziness, blurry vision, unsteadiness, and even nausea.Citation1,Citation7,Citation9,Citation10 Dizziness is reported in 50% of concussed athletes (the second most common symptom following a headache),Citation3 suggesting visual and/or vestibular impairments.Citation9–Citation13 Further, dizziness at the time of injury is the single greatest risk factor for a prolonged recovery.Citation14 The purpose of this article is to review the physiology of the VOR in order to address the pathology and symptoms as a consequence of TBI, as well as the challenges and future prospects for vestibulo-ocular involvement in TBI management.
Peripheral vestibular system anatomy
The peripheral vestibular system is composed of the bony labyrinth, the membranous labyrinth, and the specialized sensory hair cells within the inner ear (). The inner ear has two distinct divisions: 1) the cochlea for hearing and 2) the vestibular system for balance (). The bony labyrinth is located within the temporal bone on each side of the head and is filled with the perilymph. The membranous labyrinth is suspended within the bony labyrinth and is filled with endolymph. The inner ear fluids are maintained at constant volumes and contain specific concentrations of sodium, potassium, chloride, and other electrolytes. The perilymph has a higher concentration of sodium than potassium, whereas the endolymph has a higher concentration of potassium than sodium.Citation15 Mechanical conduction of sound waves and head motion cause the specialized sensory hair cells to move within the endolymph to detect sound wave pressure in the cochlea and head acceleration in the labyrinth (). The labyrinth consists of two distinct sensory mechanisms: SCCs, which detect angular head accelerations, and the otolith organs that detect linear head accelerations (). The three SCCs (anterior, posterior, and horizontal) provide the sensory neural input related to angular head acceleration, which is processed to coordinate compensatory eye and head movements via the VOR.
Figure 1 Representation of the ear from the outer ear (A) to the middle ear (B) to the inner ear (C).
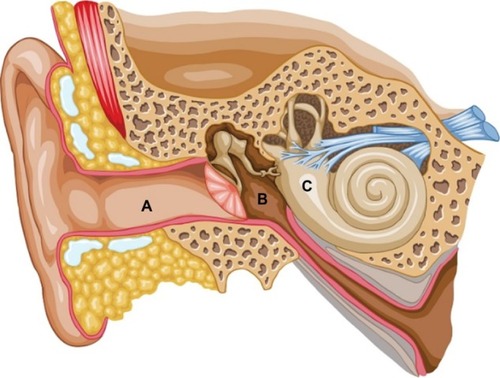
Figure 2 Representation of the two divisions of the inner ear.
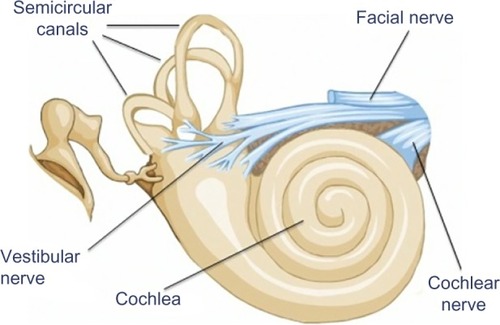
Figure 3 Representation of the endolymph (pink), which is the fluid contained in the membranous labyrinth of the inner ear and has a higher level of potassium than sodium.
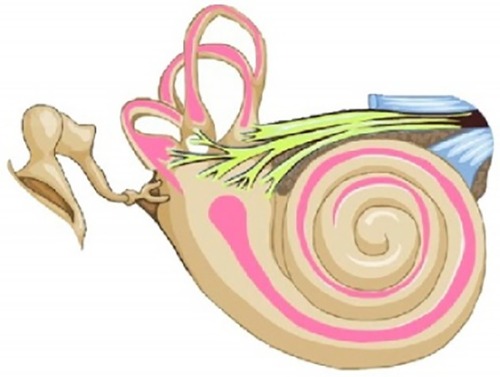
Figure 4 Representation of the three semicircular canals that detect angular head accelerations and the two otolith organs (utricle and saccule) that detect linear head accelerations.
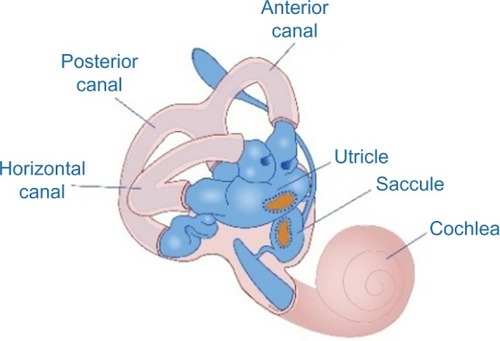
The otolith organs (utricle and saccule) provide sensory neural input related to linear head acceleration. The utricle senses linear movements of the head in the horizontal plane, while the saccule senses linear movements of the head in the vertical plane. Additionally, the utricle and saccule convey information about spinal and leg musculature for balance strategies (ankle, hip, and stepping) via the vestibular spinal reflex (VSR), which is primarily to maintain upright posture during movement. The otoliths further convey information regarding the direction of gravity through head tilt, which is incorporated into locomotion. The function of the otolith organs is accomplished by otoconia – calcium carbonate crystals that are denser than the surrounding endolymph – remaining partially stable while the head moves around them (). It is important to note that the utricle and saccule also have connections with eyes muscles as noted with the SCC. However, the direct pathways of rotational compensatory eye movements are much greater than those of linear translation, resulting in minimal contributions from the otoliths to the VOR as compared to the SCC.
Figure 5 The bottom image represents orientation of hair cells within the utricle when the head is upright.
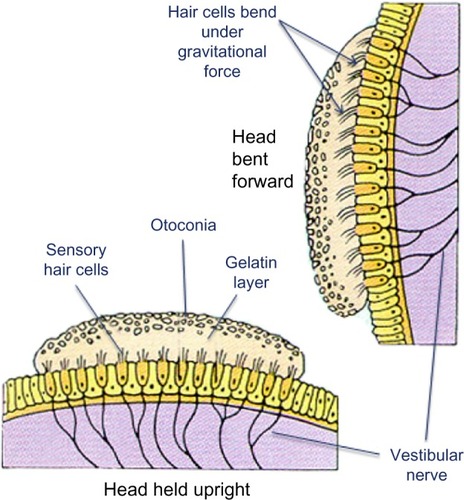
If otoconia become displaced from the utricle into the SCCs, then brief spells of vertigo are experienced related to position changes, a condition called benign paroxysmal positional vertigo (). TBI is a common cause of benign paroxysmal positional vertigo in the adult population, although it rarely occurs in adolescents.Citation10,Citation16,Citation17 The first-order information processing from the inner ear involves a number of associated reflexes: the vestibulocollic reflex (VCR), the cervico-ocular reflex (COR), the cervicospinal reflex (CSR), and the cervicocollic reflex (CCR). VCR acts on the neck muscles to stabilize the head in space during body movements and is considered a righting reflex. VCR lessens head oscillations that occur in more rapid, dynamic movements.Citation18 Although the neural pathways and actions are not fully understood, it is suspected that VCR is mediated through the otolith organs and medial VSR.Citation19 COR acts on eye movements similar to VOR, receiving input from the neck proprioceptors, and is of particular importance as a compensatory strategy if bilateral vestibular nerve loss occurs. COR is not thought to make any notable contributions to gaze stability in normal subjects because the gain is notably less than VOR.Citation20 CSR acts on the extremities and is synonymous with the tonic neck reflex. CSR interacts with VSR.Citation21 Finally, CCR acts on deep neck muscles to assist in head stabilization during head and body movements. Body rotation with the head stabilized elicits CCR, whereas head rotation with the body stabilized elicits VOR.Citation22 For purposes of this review, we focus primarily on VOR.
Figure 6 Representation of dislodged otoconia in both the posterior and horizontal canal.
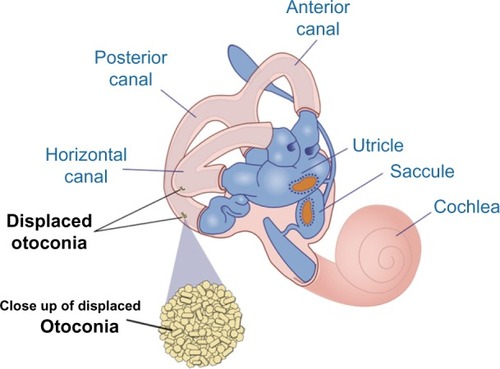
Mechanism of VOR
SCCs are approximately orthogonal (90°) to one another and provide information about the angular movements of the head in three independent planes. The anterior canal on one side is coplanar with the posterior canal on the opposite side, while the horizontal canals of each side are coplanar to one another. Thus, the six SCCs are grouped into three coplanar pairs: the right anterior and the left posterior; the left anterior and the right posterior; and the right and left horizontal canals. The horizontal canals tilt upward by an estimated 20°–30° from the horizontal plane when the head is upright, although three-dimensional multiplanar computer-ized tomography calculated the horizontal canal to be more commonly tilted by 18°.Citation23 SCCs’ responses are considered maximal when the head moves in the plane of the respective canal, which is the premise for testing the horizontal VOR function with ∼20° of flexion.Citation23,Citation24
Each SCC has an ampulla, an expanded bubble-like structure that contains the hair cells (crista ampullaris), as well as a fan-like structure known as the cupula, which separates the canal from the vestibule. The cupula has the same density as the endolymph and therefore SCC does not respond to changes in the orientation of the head with respect to gravity. With angular head movements, however, the inertia of the endolymph results in a pressure on the cupula, which stimulates the hair cells through facilitation (increased firing rate) or inhibition (decreased firing rate). If the fluid is moving toward the ampulla, the horizontal canal is facilitated, while the canals in the vertical plane on the same side are inhibited. Hence, neural activities of the SCC pairs are opposite, which results in a push–pull mechanism of facilitation on one side and inhibition on the other.Citation15 The resting firing rate in the absence of motion would therefore be equal for the right and left labyrinths.
VOR neural pathways have been well documented; for purposes of this discussion, pathways will be addressed as a three-arc process: 1) detection of head rotation occurs as a result of endolymph displacement and the subsequent deflection of the cupula (as shown in ), 2) extraocular muscles on opposite sides of the head undergo inhibition and excitation, and 3 generation of eye movements takes place with the primary purpose of compensating for head movement.Citation15 These compensatory eye movements stabilize images on the fovea and allow for clear vision during head movements.
Figure 7 Representation of the endolymphatic flow.
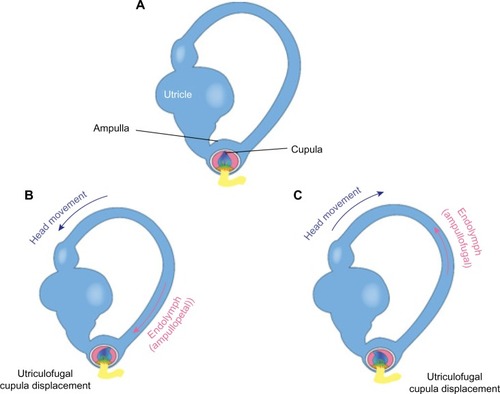
To better understand the VOR function, we will briefly review the role of the extraocular muscles. There are six extraocular muscles that contribute to eye movements: lateral and medial rectus muscles for horizontal eye movements, superior and inferior rectus muscles primarily for vertical movements but also contributing to torsional eye movements, and superior and inferior oblique muscles primarily for torsional movements but also contributing to vertical eye movements. The extraocular muscles reside in planes that are approximately parallel to the plane of respective SCCs. The SCCs are responsible for facilitating muscles on the same side and inhibiting muscles on the opposite side, as noted in . These anatomical relationships are also highlighted in and are known as Ewald’s Law 1.Citation25 VOR maintains gaze stability through compensatory eye movements equal to head movements, which is referred to as VOR gain. For example, if the head turns 30°/sec to the right, then the eyes turn 30°/sec to the left to maintain stability of the image on the fovea. In a healthy person, the gain is a 1:1 ratio.
Table 1 Represents the excitatory and inhibitory connections of each SCC with the muscles of both eyes, which results in specific compensatory responses between the vestibular system and eye muscles
Table 2 Ewald’s three laws, which govern vestibular diagnostic testing
Central connections
Vestibular information is primarily processed in the brainstem via afferent fibers of the eighth crania nerve (CN VIII). The signals enter the brainstem at the medulla and pons, which is known as the vestibular nuclear complex. Vestibular nuclear complex is comprised of four major nuclei (versus up to seven minor nuclei) in the floor of the fourth ventricle: superior, inferior, medial, and lateral.Citation15,Citation26 Most of the SCC inputs synapse with the superior and medial vestibular nuclei via first-order sensory neurons of CN VIII, while second-order sensory neurons send signals ipsilaterally and contralaterally via the medial longitudinal fasciculus to the oculomotor cranial nerves III, IV, and VI. A third motor neuron excites the extraocular muscles, causing conjugate eye movements that are equal to and opposite of head movements.Citation27 This results in gaze stabilization during head movements and represents the three-arc model previously discussed.Citation15,Citation27
The vestibular system is unique in that it also sends col-laterals to the cerebellum via the inferior cerebellar peduncle, with a role in modulating or fine-tuning the VOR. More specifically, the flocculonodular lobe and fastigial nuclei regulate vestibular and oculomotor function. The cerebellum is also considered the adaptive processor of vestibular information, specifically as related to error messages, and assists in coordination, maintaining posture, and modulation of vestibular reflexes.Citation28
There are additional vestibular connections to the thalamus, cerebral (vestibular) cortex, and reticular formation. These extensive connections result in a coordinated effort between the vestibular system and other central networks to contribute to arousal and conscious awareness of the head and body in space.Citation27
The vestibular blood supply
The anterior inferior cerebellar artery branches into the labyrinthine artery, which is the main blood supply route for the peripheral vestibular system. Anterior inferior cerebellar artery bifurcates with the superior vestibular artery supplying the utricle, superior and horizontal SCC, and a small portion to the saccule. The other branch (common cochlear artery) further bifurcates to supply the cochlea, majority of the posterior SCC, and remainder of the saccule. The venous flow is similar to the arterial flow, of which the superior vein drains the superior and horizontal SCC and utricle, while the inferior vein drains the saccule, posterior SCC, and the majority of the saccule.Citation15
Visual-vestibular functional integration
As previously discussed, the primary purpose of the VOR is gaze stability – maintaining fixation of images on the fovea during head motion. The fovea is responsible for the sharpest central vision and is of particular importance in reading, driving, and feature detection. The oculomotor system (pursuit, saccades and optokinetic) fulfill this role when the object of interest changes. For example, compensation occurs through smooth pursuit (tracking) at slower speeds, but faster speeds require more reflexive responses via saccades that are not capable by the pursuit system.
The slow phase of optokinetic nystagmus (OKN) is the same as smooth pursuit, and the fast phase is the same as saccades. The OKN system, however, is considered a reflexive mechanism that is the equivalent of the reflexive vestibular system. When tracking an object during sustained head movements, the VOR must be suppressed. This visual-vestibular interaction is essential for individuals to successfully move around in their environment and without provocation of symptoms. The optokinetic system allows for gaze stabilization in sustained head movements, or when the head is still but objects are moving in a timely fashion (eg, counting train cars that are passing by while the head is still). The optoki-netic system is also activated with full field or a large visual scene – objects that fill at least 80% of the visual field.Citation15 These features of the OKN and the VOR previously discussed can be used for the diagnosis of TBI symptoms and incorporated into approaches for treating persistent symptomatology.
When the head and eyes are moving together to track a single object, VOR must be suppressed in order to maintain focus on the target.Citation29 Dysfunction in suppression of VOR is a contributing factor to motion sensitivity, a symptom associated with slower recovery from postconcussion syndrome (PCS) symptoms.Citation2,Citation3,Citation30 As previously stated, additional symptoms associated with impairments in the visual-vestibular systems can include dizziness, unsteadiness, headaches, blurriness, decreased concentration, difficulty reading, and even nausea.Citation1,Citation7,Citation9,Citation10
TBI and VOR
VOR remains sensitive and vulnerable to TBI of all severities.Citation1,Citation30–Citation32 Dizziness is one of the most common symptoms following head traumaCitation3 and serves as a primary predictor of a prolonged recovery.Citation1–Citation3 Identifying the etiology of the dizziness, nausea, and/or balance problems following TBI is particularly challenging, as similar symptoms can be the result of brain, inner ear, visual, and/or spinal injury.
Vestibular disorders associated with TBI can be central or peripheral, although peripheral disorders are much more common.Citation33 The more serious life-threatening central causes of central dizziness are typically identified by imaging, whereas imaging has low sensitivity for peripheral vestibular dysfunction.Citation34 For these injuries, the clinical examination must include a comprehensive history regarding symptoms, identifying risk factors for PCS, which include dizziness, history of migraines, previous concussions, collision-sport participation, female sex, persistent headaches, amnesia, younger age, history of mood disorder, and/or presence of a learning disorder.Citation2,Citation35–Citation37
Further, it is critical to identify activities that exacerbate and/or decrease the symptoms. Since dizziness is a primary symptom of PCS, but represents an umbrella term with different meanings to each individual, it is recommended to ask the individual to describe their dizziness without using the word itself. The goal is to distinguish dizziness symptoms into the following four categories:Citation38
Vertigo: It is an illusion of movement, which always includes a spinning or rotational sensation. The individual feels as if 1) the room is spinning, and/or 2) it is a feeling inside their head. The individual may also describe their vertigo as a sudden falling sensation or that the ground moving, which can be provoked or exacerbated by anxiety.
Presyncope: The feeling of nearly passing out, fainting and/or blacking out.
Lightheadedness: It is often associated with a faint-like feeling or as if the individual may pass out. Lightheadedness is typically associated with a non-vestibular disorder such as hypoglycemia, orthostatic hypotension, and/or anxiety.
Unsteadiness: It is commonly described as feeling “off,” and the individual may be afraid he or she will fall. Some individuals may associate their unsteadiness with their feet, which is suggestive of loss of sensation and/or lower extremity weakness. Some also describe a drunk-like, intoxicated feeling common in both peripheral and central vestibular disorders.
identifies common characteristics of symptoms associated with peripheral vestibular dysfunction after TBI.Citation15 In TBI and concussion management, an accurate diagnosis is critical to developing the most appropriate plan of care. An effective diagnosis can identify those individuals for whom starting a rehabilitation program is not suitable and may require additional referral(s). A comprehensive initial examination, understanding of the patient’s symptoms, and clinical examination by an experienced clinician can provide an accurate diagnosis, which is the cornerstone for a successful recovery.Citation39,Citation40
Table 3 Summary of vestibular disorders associated with head trauma and corresponding clinical presentation (adapted from Ernst).Citation10
Clinical assessment of the oculomotor system
Disruption in oculomotor control, or eye movement, has long been associated with TBI,Citation41 even dating back to ancient times.Citation42 Prior to assessing the VOR, examination of the oculomotor system provides valuable information to distinguish central from peripheral vestibular pathology. At a minimum, assessment of the oculomotor system should include the following.
Alignment
Evaluation of eye position is the first step in the examination of oculomotor control, because misalignment can affect all eye movement systems. More obvious misalignments can be identified through simple observation and, if noted, warrants referral to an optometrist or ophthalmologist, especially if strabismus is a new onset post injury. More subtle horizontal or vertical misalignments can be detected using the cover test, which is considered reliable even among more inexperienced examiners.Citation43 Misalignment of the eyes can cause blurriness, dizziness, headaches, and/or diplopia.
Range of motion
The range of oculomotor control can be performed during smooth pursuit testing, but requires observations through the nine cardinal positions of gaze versus observation during H-pattern only. In the absence of damage, individuals can move his or her eyes with conjugate gaze into all extremes of the visual field.
Smooth pursuit
Normal smooth pursuit is a smooth movement of the eyes tracking a slow moving target from one point of fixation to another in a timely manner. Evaluation of the tracking system assesses how timely and smoothly the eyes follow a target across the field of vision while the head is in the neutral position. Smooth pursuit is often tested in the H-pattern across the visual field to examine cranial nerves III, IV, and VI. Damage to smooth pursuit systems can be detected as imprecise following of the target, observed by corrective, or catch-up, saccades similar to nystagmus. Normal tracking declines with age and is sensitive to medications, inattention, and anxiety.Citation44 Notable eye movement asymmetry during tracking suggests a cranial nerve palsy, which may be associated with TBI or the central nervous system dysfunction. Following TBI, it is also important to assess the interaction between the smooth pursuit system and the VOR via the VOR cancellation test.
Saccades
The saccadic system produces a rapid eye movement from one point of fixation to another point of fixation. Normal saccades bring the image of the new target onto the fovea for clear viewing. Abnormal saccades can include decreased velocity to the target, increased latency to initiate the movement, and/or corrections (either under or overshooting) the target.Citation45 Abnormal saccades suggest disruption in normal brainstem and/or cerebellum functionCitation44 and have been measured in symptomatic individuals following whiplash injury.Citation46
Vergence
The vergence system aligns both foveas onto a common target at varying distances through disjunctive eye movements; each eye moves in a direction opposite to the other. Normal vergence results in symmetrical and coordinated eye movement inward (convergence) or outward (divergence), depending on the distance of the target. Disruption of the vergence system has long been associated with TBI,Citation47 where the majority of studies focus on combat-related injuries and more recently sports-related concussions.Citation30
VOR cancellation
The VOR cancellation test is essentially a higher demand test of the smooth pursuit system. The subject is asked to fixate on a moving target while the head is moved in the same direction. For example, the subject can extend out their arms, clasp their hands together, and extend their thumbs. The head and body rotate together back and forth while the subjects maintain their gaze on their thumbs. Findings should be consistent with results in the smooth pursuit test. An abnormal finding is observation of corrective saccadesCitation44 and/or provocation of symptoms.Citation30
Assessment of the vestibulo-ocular system
As previously discussed, VOR functions primarily to achieve clear vision during head movement. With a dysfunction in VOR, the gain error is too great and can result in symptoms of dizziness, unsteadiness, and even nausea. In more severe VOR dysfunction, the individual may experience oscillopsia, the sensation that objects are jumping or even the room moving during head movements. Oscillopsia and vertigo are less common with mild TBI, although movement-related dizziness is very common. Bedside tests for the VOR include the following.
Head thrust: The head thrust test is a single, rapid head turn based on the oculocephalic reflex, or “Doll’s Eye” reflex, which is performed as a standard for individuals who are in a coma or unresponsive. The head of the patient is rapidly turned in the horizontal plane to one side (∼30°), while the clinician observes for compensatory conjugate eye movements in the opposite direction. Halmagyi and CurthoysCitation48 expanded the clinical application of this test to conscious patients with complete unilateral vestibular loss. The test is considered positive (abnormal) if the examiner observes corrective saccades back to the target (typically the examiner’s nose). Although the head thrust test may provoke symptoms (eg, dizziness) in individuals following mild TBI, corrective saccades due to retinal slippage are rarely observed unless the injury involved significant unilateral vestibular weakness.
Head shake test: The head shake test is used to identify the possibility of asymmetrical vestibular input due to peripheral or central pathology.Citation29 The examiner passively performs head oscillations, or the subject does so actively. Head oscillations should occur with head flexion of ∼20° to align the horizontal canals parallel to the ground and cover a 60° arc (30° side to side) at ∼2 Hz (2 cycles/s) for 20 repetitions. The higher velocity of the head movement exceeds pursuit system, which can only maintain gaze sta-bilization up to 1 Hz. Normally, no nystagmus is expected. Some individuals with peripheral or central vestibular dysfunction show a transient nystagmus (∼30 seconds) with the fast phase toward the unaffected ear after the head movement is stopped.Citation38,Citation49 A slower nystagmus that switches directions may follow. This test has improved sensitivity with Frenzel goggles or infrared video goggles, but it has low sensitivity overall as a screening tool for vestibular dysfunction.Citation50 Abnormal results are detected in individuals with significant vestibular loss, which is typically not associated with mild TBI.
Dynamic visual acuity (DVA) test: The DVA test was originally discussed in 1984 for assessing vestibular loss and has since become a standard clinical test for vestibular therapists.Citation51 The test is performed in the sitting position, and the individual wears corrective lenses as needed. The individual reads the lowest line on a visual acuity chart (eg, Snellen chart), recording the smallest line read with less than three errors (eg, 20/20). The examiner flexes the patient’s head by 20º and continues to hold the patient’s head with both hands and rotates it back and forth in the horizontal plane in a 40°–60° arc (20°–30° side to side to side) at ∼2 Hz (exceeding compensation by the pursuit system), all while the patient reads the visual acuity chart. Again, the smallest line read with less than three errors is recorded while the head is moving (eg, 20/50). A normal result is a drop of no more than two lines when the head is moving from the baseline static acuity. An abnormal result is a difference of two or more lines between static and dynamic acuity, likely with provocation of symptoms. Sensitivity and specificity of the DVA test varies greatly, as a result of varied methodology, such as passive (examiner moves head) versus active (subject moves head) oscillations, frequency of head movements (1–7 Hz), and position of head movement. Further, although it is recommended to perform DVA testing with the head tilted 20° into flexion to isolate the horizontal canal, vision can be obscured for those wearing corrective glasses and the overall the range of visual acuity diminishes in upward gaze. Regardless, provocation of symptoms during the DVA test indicates injury-induced damage.
Vestibular/ocular motor screen (VOMS): A modified version (or screen) of the DVA is incorporated in the VOMS by holding a 14-size type at arm’s length and performing rapid head movements as described earlier. The VOMS includes five domains: 1) smooth pursuit, 2) saccades, 3) near point convergence, 4) VOR, and 5) visual motion sensitivity. The examiner measures for symptom provocation according to a graded subjective scale for each assessment, ranging from no symptoms (0) to severe (10) after each test and also includes three measurements of near point convergence.Citation30 In a recent study of the VOMS, concussed athletes reported the highest percentage of symptom provocation (61%) during administration of VOR testing.Citation30 Because the VOMS relies primarily on symptom provocation, it should not be used in isolation.
Advanced VOR testing: More sophisticated VOR testing is typically conducted in specialized dizziness and balance centers. Rotary chair testing assesses VOR function at slower speeds that are less associated with ADL but is sensitive to detect bilateral vestibular loss. High-velocity head rotation testing can better assess higher demands of VOR function associated with ADL and more dynamic activities. These active head rotation systems are often computerized, with recent incorporation of infrared video based on Halmagyi and Curthoys’ work to more objectively measure the corrective saccades noted in the head thrust test. These systems can measure smaller corrective saccades (covert saccades) that are not otherwise observable, and indicate vestibular disruption; however, the evidence for VOR dysfunction after TBI is sparse. Advanced VOR testing holds the potential to identify subtle dysfunction in the vestibular system following TBI, especially if the individual reports vestibular symptoms.
Treatment of VOR dysfunction
Successful treatments depend on an accurate diagnosis to guide a management strategy. In regard to concussion, recom mended components of the clinical assessment include a graded symptom checklist scale, review of past medical history to identify risk factors associated with a prolonged recovery, cognitive testing, cranial nerve assessment (with a focus on oculomotor control, including vergence), cervical range of motion, balance, gait, and vestibular testing.Citation52–Citation54
As an initial screening tool, VOMS can identify visual and/or vestibular dysfunction, although more diagnostic tests to confirm findings are advised before integrating visual and/or vestibular exercises in managing the rehabilitation process. Once the specific impairments localize the etiology, an appropriate care plan can be developed, which may include referrals, continued rest, and/or recommendations to begin a “return-to-activity” rehabilitation program.
With regard to sports-related concussion, the most recog-nized return-to-play program is the “Graded Exertional Step-Wise Approach” protocol endorsed by the National Athletic Trainers’ Association.Citation52 This protocol forms a foundation for the most widely recognized concussion guidelines described in the Zurich Consensus Concussion StatementCitation55 and is a mandate in the majority of states’ concussion legislation, as well as the college athletics and professional sports concussion management guidelines.
The stepwise approach provides a foundation and standardized guideline suitable for the majority of individuals with mild TBI, as >80% of concussed adolescents resolve symptoms within 21 days of the injury with adequate rest and a gradual return to activities.Citation3–Citation6 For those individuals with residual visual-vestibular associated symptoms, the stepwise approach with exertion is not sufficient; rehabilitative interventions are needed.
The potential to rehabilitate VOR varies. VOR function frequently recovers spontaneously with time, which has been attributed to neuroplasticity, and does not require any intervention. For those individuals who do not recover with time, Herdman describes two primary approaches for vestibular intervention: 1) adaptation and 2) substitution or compensation due to significant vestibular loss of which both are accomplished through an exercise-based treatment programCitation56,Citation57 In cases of significant vestibular loss, the VOR, especially at higher velocities, does not return to its pre-morbid level of functionCitation58; however this is unlikely for concussion. When to begin vestibular therapy is debatable, although the VOR adaptation exercises themselves need to be context specific.Citation44 Through repetitive exercises that induce retinal slippage, the central vestibular mechanisms (brainstem and cerebellum) will correct and thereby rehabilitate these error messages. Retinal slippage can be induced by rapid head movements while maintaining visual fixation on a target in the distance, known as gaze stability exercises. VOR exercises should follow testing parameters but be performed under a variety of distances (targets at arm’s length to distances further away), under a variety of conditions (seated, standing, and walking) and in various planes (horizontal, vertical, and/or with head tilt). Of note, the cerebellum limits of learning are <2.5 Hz, whereas the VOR performs at much higher speeds. Thus, neuroplasticity outside the cerebellum may be involved in VOR rehabilitation.Citation59,Citation60
Exercises should begin slowly while the individual is in a seated (static) position to assess whether symptoms of anxiety, dizziness, and nausea are exacerbated. VOR exercises should be gradually increased in velocity, but as speed increases, amplitude of the head movement should decrease. This conservative approach to gradually increase intensity is more effective than generating error messages that are too large for the capacity of the VOR system.Citation56 This gradual exercise approach based on tolerance and recovery of exercise is also consistent with the graded stepwise approach for mTBI.Citation52
In predicting recovery, risk factors for negative outcomes overlap, but not entirely, between vestibular rehabilitation and PCS. For instance, the sex of the individual does not appear to influence recovery from vestibular symptoms as it does for PCS symptoms, although a history of migraine, younger age, and mood disorder similarly predict outcome for vestibular impairment and PCS.Citation61–Citation64
Current challenges in clinical management of TBI
Vestibular function is vulnerable to TBI of any severity. The primary challenge for the diagnosis and management of VOR impairments following TBI is the limited knowledge arising from a small body of research. Sensory organization and balance testing post TBI have been studied predominantly, but static balance testing performed as a standard post-injury examination does not adequately represent the vestibular system dynamics. As a result, static balance testing shows a perceived lack of sensitivity to detect concussion-related impairments. Additionally, balance testing alone incompletely describes vestibular function, as VOR and VSR have unique neuronal pathways that can sustain damage independent of one another. Hence, VOR function more accurately represents vestibular system dynamics and is a priority for post-injury examination.Citation30 With an absence of clear normative data for VOR function in specialized populations (eg, athletes and elderly), diagnosis and treatment of vestibular impairments become more complex, which can be mitigated partially by conducting baseline/pre-injury testing, where possible. Although VOMS is thought to have a high level of confidence in accurately identifying a concussion, it is primarily a symptom provocation test and should not be used in isolation to diagnose or implement exercises. Challenges with VOMS include inter-tester reliability, symptom provocation with testing after head trauma often having an anxiety component (especially with a prolonged recovery), and relying on symptom reporting, which can result in recall bias (especially if the person wants to be allowed to return to sports). Comprehensive vestibular therapy is indicated for a subset of individuals in the acute phase post injury showing uncompensated vestibular dysfunctionCitation65–Citation67 but typically considered only with more chronic vestibular dysfunction (10–12 weeks).Citation68 The shared circuitry between the visual and vestibular systems indicates that a comprehensive clinical examination is required to accurately identify central and peripheral involvement, including the ability to differentiate between a component of anxiety and/or cervicogenic involvement. The complexity of testing, interpretation, and rehabilitation programs necessitate trained providers, who may not be readily available in all clinics and even multidisciplinary centers. Concussion specialists are licensed health care professionals who have training in concussion management, adhere to best practices, and see a sufficient number of cases.Citation54 These factors can not only limit access to care but also prescribe a financial burden that deters marketplace saturation.
Future prospects
The elevated public awareness and associated legislation regarding TBI will continue to grow and focus research efforts in the area of diagnosis and management. Standard clinical testing discussed here will be complemented by advances in imaging and biomarker diagnostic tools. Further refinements through iterative processes will continue to refine and develop specific tools for visual and vestibular dysfunction related to TBI, as evidenced by the VOMS as the newest screening tool. Concussion specialists must continue to collaborate and communicate with other clinicians, specialists, and researchers to build knowledge for the comprehensive diagnosis and management of concussion. Advancements and accessibility with mobile- and web-based technology could potentially fill this void and pave the way for best practices through data mining to recognize practice patterns and track individuals in real time. This platform provides opportunities for increased collaboration and communication to deliver evidence-based clinical care to improve quality of life. Regardless of TBI severity, successful outcomes depend on awareness, education, and a comprehensive interdisciplinary approach. The continuing inclusion of visual, vestibular, and cervicogenic symptoms in TBI assessment and management continues to develop best practices for TBI.
Acknowledgments
The authors thank Dr Barin and Dr Polec for providing technical assistance and reviewing the manuscript.
Disclosure
The authors report no conflicts of interest in this work.
References
- HofferMEGottshallKRMooreRBaloughBJWesterDCharacteri-zing and treating dizziness after mild head traumaOtol Neurotol200425213513815021772
- LauBCKontosAPCollinsMWMuchaALovellMRWhich on-field signs/symptoms predict protracted recovery from sport-related concussion among high school football players?Am J Sports Med201139112311231821712482
- KontosAPElbinRJSchatzPA revised factor structure for the Post-Concussion Symptom Scale: baseline and postconcussion factorsAm J Sports Med201240102375238422904209
- McCreaMGusckiewiczKMMarshallSWAcute effects and recovery time following concussion in collegiate football players: the NCAA Concussion StudyJAMA2003290102556256314625332
- GuskiewiczKMAssessment of postural stability following sport-related concussionCurr Sports Med Rep200321243012831673
- CovassinTElbinRJHarrisWParkerTKontosAThe role of age and sex in symptoms, neurocognitive performance, and postural stability in athletes after concussionAm J Sports Med2012353185196
- CrippsALivingstonSCThe value of balance-assessment measurement in identifying and monitoring acute postural instability among concussed athletesJ Sport Rehabil201322677123037313
- NaguibMBMadianYRefaatMMohsenOEl TabakhMAbo-SettaACharacterisation and objective monitoring of balance disorders following head trauma, using videonystagmographyJ Laryngol Otol20121261263322035505
- ChamelianLFeinsteinAOutcome after mild to moderate traumatic brain injury: The role of dizzinessArch Phys Med Rehabil2004851662166615468028
- ErnstABastaDSeidlROTodtISchererHClarkeAManagement of posttraumatic vertigoOtolaryngol Head Neck Surg200524554558
- FitzgeraldDCHead trauma: hearing loss and dizzinessJ Trauma19961324554558
- KapoorNCiuffredaKJVision disturbances following traumatic brain injuryCurr Treat Options Neurol2002427128012036500
- Capó-AponteJEUrosevichTGTemmeLATarbettAKSangheraNKVisual dysfunctions and symptoms during the subacute stage of blast-induced mild traumatic brain injuryMil Med2012177780422808887
- SuhMKolsterRSarkarRGhajarJDeficits in predictive smooth pursuit after mild traumatic brain injuryNeurosci Lett200640110811316554121
- JacobsonGPShepardNTBalance Function Assessment and Management2nd edCaliforniaPlural Publishing, Inc2016
- DixMRHallpikeCSThe pathology, symptomatology and diagnosis of certain common disorders of the vestibular systemAnn Otol Rhinol Laryngol195261987101613008328
- DoriguetoRSMazzettiKRGabilanYPGanancaFFBenign paroxysmal positional vertigo recurrence and persistenceBraz J Otorhinolaryngol200975456557219784427
- GoldbergJMCullenKEVestibular control of the head: possible functions of the vestibulocollic reflexExp Brain Res20112103–433134521442224
- PozzoTBerthozAPapvovCThe effect of gravity on the coordination between posture and movementTaguchiKIgarashiMMoriSVestibular and Neural Front, Proceedings of the 12th International Symposium on Posture and GaitAmsterdamElsevier1994589602
- BronsteinAMHoodJDThe cervico-ocular reflex in normal subjects and patients with absent vestibular functionBrain Res19863731–23994083487371
- PompeianoOThe tonic neck reflex: supraspinal controlPetersonBWRichmondFJControl of Head MovementNew YorkOxford University Press1988108119
- PetersonBWGoldbergJBilottoGFullerJHCervicocollic reflex: its dynamic properties and interaction with vestibular reflexesJ Neurophysiol1985541901093162006
- Della SantinaCCPotyagayloVMigliaccioAAMinorLBCareyJPOrientation of human semicircular canals measured by three-dimensional multiplanar CT reconstructionJ Assoc Res Otolaryngol20056319120616088383
- BalohRWSillsAWHonrubiaVImpulsive and sinusoidal rotatory testing. A comparison with results of caloric testingLaryngoscope197989646431264
- JongkeesLBEwaldJRArch Otolaryngol19668366156195328009
- CarletonSCCarpenterMBAfferent and efferent connections of the medial, inferior and lateral vestibular nuclei in the cat and monkeyBrain Res198327829516315158
- BrandtTDieterichMVestibular syndromes in the roll plane: topographic diagnosis from brainstem to cortexAnn Neurol1994363373478080241
- PurvesDAugustineGJFitzpatrickDCentral vestibular pathways: eye, head, and body reflexes Neuroscience2nd edSunderland, MASinauer Associates2001
- LeighRJZeeDSThe Neurology of Eye Movements4th edNew YorkOxford University Press2006
- MuchaACollinsMWElbinRJA brief vestibular/ocular motor screening (VOMS) assessment to evaluate concussions: preliminary findingsAm J Sports Med201442102479248625106780
- GottshallKDrakeAGrayNMcDonaldEHofferMEObjective vestibular tests as outcome measures in head injury patientsLaryngoscope20031131746175014520100
- DaviesRALuxonLMDizziness following head injury: a neuro-tological studyJ Neurol19952422222307798121
- ThompsonTLAmedeeRVertigo: a review of common peripheral and central vestibular disordersOchsner J200991202621603405
- GizziMRileyEMolinariSThe diagnostic value of imaging the patient with dizzinessArch Neurol199653129913048970460
- ScopazKAHatzenbuehlerJRRisk modifiers for concussion and prolonged recoverySports Health20132010537541
- MararMMcIllvainNMFieldsSKComstockRDEpidemiology of concussions among United States high school athletes in 20 sportsAm J Sports Med201240474775522287642
- McCreaMGuskiewiczKRandolphCIncidence, clinical course, and predictors of prolonged recovery time following sport-related concussion in high school and college athletesJ Int Neuropsychol Soc2013191223323058235
- BriscoeAMullurRSVertigo and DizzinessWashington Manual® of Outpatient Internal MedicinePhiladelphiaLippincott Williams and Wilkins2012
- PetersonMCHolbrookJHVon HalesDSmithNLStakerLVContributions of the history, physical examination, and laboratory investigation in making medical diagnosesWest J Med19921561631651536065
- HamptonJRHarrisonMJGMitchellJRAPrichardJSSeymourCRelative contribution of history-taking, physical examination, and laboratory investigation to diagnosis and management of medical outpatientsBr Med J197524864891148666
- GanzJCEdwin Smith papyrus case 8: a reappraisalJ Neurosurg20141201238123924484229
- HeitgerMHJonesRDMacleodADSnellDLFramptonCMAndersonTJImpaired eye movements in post-concussion syndrome indicate suboptimal brain function beyond the influence of depression, malingering or intellectual abilityBrain20091322850287019617197
- AndersonHAMannyRECotterSAMitchellGLIraniJAEffect of examiner experience and technique on the alternate cover testOptom Vis Sci201087316817520125058
- ZeeDSVestibular adaptationHerdmanSJVestibular Rehabilitation2nd edPhiladelphia, PAFA Davis19997787
- TirelliGRigoSBulloFMeneguzziCGregoriDGattoASaccades and smooth pursuit eye movements in central vertigoActa Otorhino-laryngol Ital201131296102
- MosimannUPMuriRMFelblingerJRadanovBPSaccadic eye movement disturbances in whiplash patients with persistent complaintsBrain2000123482883510734013
- CohenMGroswasserZBarchadskiRAppelAConvergence insufficiency in brain-injured patientsBrain Inj1989321871912471568
- HalmagyiGMCurthoysISA clinical sign of canal paresisArch Neurol1988457377393390028
- HainTCFetterMZeeDSHead-shaking nystagmus in patients with unilateral peripheral vestibular lesionsAm J Otolaryngol1987836473578675
- JacobsonGPNewmanCWSafadiISensitivity and specificity of the head-shaking test for detecting vestibular system abnormalitiesAnn Otol Rhinol Laryngol19909263271
- LongridgeNSMallinsonALThe dynamic illegible E-test. A technique for assessing the vestibular-ocular reflexActa Otolaryngol19871033–4273279
- LeddyJJSandhuHSodhiVBakerJGWillerBRehabilitation of concussion and post-concussion syndromeSports Health20124214715423016082
- BroglioSPCantuRCGioiaGANational Athletic Trainer’s AssociationNational Athletic Trainers’ Association: management of sport concussionJ Athl Train201449224526524601910
- Parachutecanada.org [webpage on the Internet]Solving the Concussion Crisis: Practical Solutions (Consensus 2015)Toronto Available from: http://www.parachutecanada.org/research/item/solving-the-concussion-crisis-practical-solutionsAccessed November 15, 2015
- McCroryPMMeeuwisseWHAubryMConsensus statement on concussion in sport: the 4th International Conference on Concussion in Sport held in Zurich, November 2012Br J Sports Med20134725025823479479
- HerdmanSJAdvances in the treatment of vestibular disordersPhys Ther1997776026189184686
- HerdmanSJRole of vestibular adaptation in vestibular rehabilitationOtolaryngol Head Neck Surg199811949549674514
- CurthoysISHalmagyiGMVestibular compensation: a review of the oculomotor, neural, and clinical consequences of unilateral vestibular lossJ Vestib Res199552671077743004
- DieringerNVestibular compensation: neural plasticity and its relations to functional recovery after labyrinthine lesions in frogs and other vertebratesProg Neurobiol1995462–3971297568917
- PorrillJDeanPCerebellar motor learning: when is cortical plasticity not enough?PLoS Comput Biol2007310e197
- WhitneySLRossiMMEfficacy of vestibular rehabilitationOtolaryngol Clin North Am200033365967210815042
- WhitneySLWrisleyDMMarchettiGFFurmanJMThe effect of age on vestibular rehabilitation outcomesLaryngoscope2002112101785179012368616
- WrisleyDMWhitneySLFurmanJMVestibular rehabilitation outcomes in patients with a history of migraineLaryngoscope2002234483487
- TopuzOTopuzBArdiçFNSarhuMOgmenGArdiçFEfficacy of vestibular rehabilitation on chronic unilateral vestibular dysfunctionClin Rehabil20041817683
- ShepardNTTelianSAProgrammatic vestibular rehabilitationOtolaryngol Head Neck Surg19951121173827816453
- HanBISongHSKimJSVestibular rehabilitation therapy: review of indications, mechanisms and key exercisesJ Clin Neurol2011718419622259614
- EllisMJLeddyJJWillerBPhysiological, vestibuloocular and cervicogenic post-concussion disorders: An evidence-based classification system with directions for treatmentBrain Inj201529223824825314613
- Valvovich-McLeodTCHaleTDVestibular and balance issues following sport-related concussionBrain Inj201529217518425291297