Abstract
Abnormal blood vessel growth in the retina is a hallmark of many retinal diseases, such as retinopathy of prematurity (ROP), proliferative diabetic retinopathy, and the wet form of age-related macular degeneration. In particular, ROP has been an important health concern for physicians since the advent of routine supplemental oxygen therapy for premature neonates more than 70 years ago. Since then, researchers have explored several animal models to better understand ROP and retinal vascular development. Of these models, the mouse model of oxygen-induced retinopathy (OIR) has become the most widely used, and has played a pivotal role in our understanding of retinal angiogenesis and ocular immunology, as well as in the development of groundbreaking therapeutics such as anti-vascular endothelial growth factor injections for wet age-related macular degeneration. Numerous refinements to the model have been made since its inception in the 1950s, and technological advancements have expanded the use of the model across multiple scientific fields. In this review, we explore the historical developments that have led to the mouse OIR model utilized today, essential concepts of OIR, limitations of the model, and a representative selection of key findings from OIR, with particular emphasis on current research progress.
Keywords:
History of the mouse model of OIR
Initial clinical observations
The first case reports of retrolental fibroplasia, now known as retinopathy of prematurity (ROP), were written by Theodore L Terry in the early 1940s, who described several abnormalities in the ocular vasculature of infants born prematurely, the cause of which was unknown.Citation1,Citation2 Around this time, clinical studies by Wilson et al found improved respiration in preterm babies when given high concentrations of oxygen,Citation3 and so the use of supplemental oxygen therapy for premature neonates became increasingly routine and widespread in the 1950s.Citation4 Concurrent with this rise in the liberal use of oxygen therapy in the neonatal setting, however, came a well-documented increase in the incidence of ROP.Citation4–Citation7 Thaddeus S Szewczyk was one of the first physicians to make the connection between oxygen and ROP from his clinical observations of changes in ROP disease pattern with changes in oxygen concentration administered.Citation8,Citation9 However, an intense controversy developed as to how oxygen could cause ROP. This debate could be divided into four general views: anoxia, toxic effects of high oxygen, relative anoxia following high oxygen administration, and factors unrelated to oxygen.Citation10 These clinical observations and the ensuing debates sparked research in the early 1950s to further explore the role of oxygen in ROP using various animal models.
Initial animal studies of the retinal vasculature
Decades before the initial oxygen-induced retinopathy (OIR) experiments in the 1950s, researchers were aware that the retina has a relatively higher rate of respiration and anaerobic glycolysis than most other tissues, and that developing retinas exhibit higher oxygen consumption than mature retinas.Citation11 Normal retinal vascular development was thoroughly studied in humans, rats, and cats by Michaelson in the 1940s, who found that vascular growth and patterning was overall similar among the different animals.Citation12 In light of these earlier findings and clinical observations of ROP, Ashton et al were among the first to explore the effect of changing oxygen concentrations on retinal vascular development in animals, using kittens given their close resemblance to human infants in terms of retinal vascular development.Citation13 By varying the onset, duration, and concentration of oxygen exposure in newborn kittens, they were able to characterize two phases of OIR (“vaso-obliterative” and “vaso-proliferative”) that closely mimic the early stages of ROP.Citation10 Despite the close approximation of this early kitten model of OIR to human ROP, obtaining adequate sample sizes to thoroughly assess a range of experimental variables posed a significant challenge, as noted by the authors at the time.Citation10,Citation14
Early murine models of OIR
The pathologic changes in the mouse retina exposed to hyperoxic conditions that resembled human ROP (eg, hemorrhages, retinal folding, fibrovascular changes in the vitreous body) were first noted around the same time as Ashton’s studies in kittens.Citation15,Citation16 Given the persistent discussion over the pathophysiology of ROP, many of the early experiments focused heavily on exploring the effects of low oxygen on retinal vascular development. Such experiments helped to disprove the theory that hypoxia was the sole driver of the pathologic retinal neovascularization (NV) seen in ROP and helped to establish hyperoxia as a key inciting factor.Citation10,Citation14,Citation16 Subsequent studies in mice reproduced the pathologic ocular findings seen in ROP, albeit to varying degrees and under differing experimental conditions.Citation11,Citation17 Although this variability prevented definitive conclusions or extrapolations, these early attempts to generate OIR in mice provided insights that would ultimately prove valuable to the model used today.
Gyllensten and Hellström were the first to document and consistently utilize a specific mouse strain for their OIR experiments in order to minimize the frequency of spontaneous eye anomalies that could complicate their results.Citation14 Interestingly, the strain they selected, C57Bl/6 mice, is one of the most commonly used strains for OIR studies today. Though most preliminary mouse studies involved high oxygen exposure at birth, Gerschman et al studied mice put in high oxygen at different times during their first week of life,Citation11 which would prove to be an important distinction given the dramatic changes in the mouse retinal vasculature with each day of development.Citation17 Further investigations into mouse retinal vascular development noted an inverse relationship between hyaloid and retinal vessel growth: hyaloid vessels are present at birth but regress over time, while retinal vessels are nearly absent at birth but proliferate almost fully by the first week of life.Citation17 This is a particularly critical observation, since early studies noted dilation and proliferation of hyaloid vessels during hyperoxiaCitation16–Citation18 but were unable to distinguish this hyaloidopathy from true retinopathy,Citation19 which may have contributed to the often conflicting results produced by various research groups.
Subsequent advances leading to the current mouse model of OIR
There was a relative paucity of new work on the mouse model of ROP in the few decades following the 1950s.Citation20 Although Ashton’s and Patz’s animal models of OIR were generally considered to be the most reliable for studying retinal NV,Citation21 there were still considerable discrepancies in OIR reproducibility.Citation20 For instance, several groups disagreed on the threshold of oxygen exposure that was needed to trigger retinopathy: OIR was observed in kittens with 40% oxygen,Citation10 but in mice, 70% oxygen was insufficient,Citation22 and retinopathy was only observed at 80% oxygen.Citation16 These observations led to the generalization that OIR can only be reliably reproduced at near complete oxygen saturation.Citation20 Further compounding the establishment of a standardized mouse OIR model was the lack of a uniform method to assess the severity of retinopathy, which was likely due in part to technological barriers.
Several advancements, from improved retinal vascular imaging to a better understanding of angiogenesis, set the stage for establishing a more rigorous and reliable mouse model of OIR. The advent in 1961 of fluorescein angiography for retinal vascular imaging by Novotny allowed for better visualization of smaller retinal vessels as well as in vivo retinal imaging.Citation23,Citation24 Lectin staining, which uses carbohydrate-binding proteins and is the most frequently used technique today for mouse OIR, was first advanced by Blanks and Johnson in the 1980s, who demonstrated that lectins selectively label retinal vasculature in developing mice.Citation25 Further studies confirmed the superiority of fluorescein and lectin staining in visualizing retinal vessels during mouse development.Citation26 Refinements to fluorescein staining, such as labeling dextrans with high-molecular weight fluorescein, minimized contrast leakage and allowed for better visualization of the entire rodent retina at higher resolutions compared to India ink, which was most widely used in the OIR experiments of the 1950s.Citation27
In the early 1970s, Folkman et al reported that ocular NV was triggered by tumor implants in the corneaCitation28; this indirectly demonstrated the existence of angiogenic factors and effectively established the field of angiogenesis. This was a particularly significant discovery, since Michaelson and Ashton first postulated the existence of a “vaso-proliferative factor” responsible for the NV seen in ROP decades earlier.Citation10,Citation12,Citation21 Another critical finding was the identificationCitation29 and partial purificationCitation30 of a retinal substance isolated from different animals that stimulated in vitro endothelial cell growth, which was the first direct evidence of angiogenic activity in the mammalian retina.Citation21 This discovery marked a major transition in research, from focusing on speculations and theories of retinal angiogenic activity to identifying specific angiogenic factors and mechanisms. Thus, these pioneering studiesCitation30,Citation31 inspired further research into retinal angiogenesis,Citation21 primarily in mice, given their rapid and proliferative breeding patterns.Citation20
Despite the scarcity of new literature on mouse OIR from the late 1950s to early 1990s, a few critical refinements in experimental techniques and understanding were made during this time frame. As researchers gradually came to better understand the pathophysiology underlying the two phases of OIR, mouse studies demonstrated that NV was most pronounced after newborn pups were put in hyperoxia for 5 days and then put in normoxia for 5 days.Citation32 This resembles the time course of oxygen exposure in the current mouse OIR model that maximizes NV, albeit using an earlier onset of hyperoxia exposure. Researchers had also noted that the extent of vaso-obliteration during phase 1 of OIR was positively correlated with the likelihood of developing NV during phase 2 of OIR.Citation21 This observation now serves as the basis for therapeutic strategies that indirectly reduce retinal NV by minimizing early vaso-obliteration. Although there was no consensus regarding an optimal method to assess the severity of retinopathy in mice,Citation20 a systematic grading system was established in the kitten model that assessed retinal flatmounts using four categories: extent of vessel growth, vessel pattern, degree of peri-arteriolar capillary free zone, and degree of capillary tuft formation.Citation33 Some researchers felt that the small size of the mouse pup retina posed a challenge for successful retinal flatmounts, and that alternative approaches should be pursued, such as manually counting vitreous capillaries in histological cross sections that passed through the optic nerve.Citation20 Ultimately, research by Smith et al greatly improved the quantitative assessment of OIR changes by distinguishing hyaloidopathy from retinopathy,Citation19 but quantifying NV continues to remain a challenge, as evidenced by the variety of approaches in the past 20 years.Citation34–Citation36 The challenges of quantification are explored in further detail later in this review.
Essential concepts of OIR
Comparison of human and murine retinal vascular development
There are several key differences between human and murine retinal developmental angiogenesis that allow the mouse model of OIR to adequately reflect human ROP. One major distinction is the temporal nature of retinal angiogenesis. Whereas development begins relatively early in human fetal development, it occurs postnatally in mice.Citation36,Citation37 As a result, human infants born at term possess fully mature retinal vessels, while newborn mice have immature vascular development similar to that seen in premature humans infants.Citation38 Fortunately, murine retinal vessel growth progresses in a rapid, predictable, and tightly regulated manner,Citation36,Citation39 with near-complete extension of the superficial vascular plexus achieved within a week (with some variability among different mouse strains).Citation40 This difference allows for the study and manipulation of retinal vasculature patterning, thereby providing a suitable model for human ROP, which is triggered by subjecting immature retinal vessels to relative hyperoxia at birth.
As mentioned earlier, ROP classically progresses in two phases: initial vaso-obliteration of immature vessels followed by pathological NV during the subsequent vascular repair phase. Granted, advancements in our understanding of ROP pathophysiology have led to the updated notion that phase 1 of ROP reflects more of a delay in retinal vascular development with modest vaso-obliteration in most cases.Citation37 Nevertheless, vaso-obliteration and NV remain the hallmarks of ROP, with the two processes temporally and pathophysiologi cally linked. The mouse model of OIR consistently reproduces these two key features over the span of 3 weeks with the advantage of genetic manipulation.Citation19,Citation34,Citation36 Additionally, the mouse OIR model exhibits spontaneous regression of the new vessels in the late stages of phase 2, which is consistent with the spontaneous involution seen in many cases of human ROP.Citation41 An in-depth comparison of human and murine retinal angiogenesis is provided in the review by Stahl et al.Citation36
Current mouse model of OIR
The model developed by Smith et al,Citation19 coupled with the method of quantifying vaso-obliteration and pathological NV established by Connor et al,Citation34 is widely regarded as the current standard for OIR experiments in mice. In this model (), mouse litters (C57Bl/6 mice are the most widely utilized) are placed in 75% oxygen at postnatal day (P)7, when there is an optimal balance between hyaloid vessel regression and immature retinal vasculature to allow visualization of subsequent NV.Citation19,Citation32 The hyperoxic conditions mimic the relative hyperoxia that premature human infants are exposed to at birth; thus, P7–P12 defines phase 1 of OIR, during which immature vessels regress ().Citation34 Regarding the kinetics of phase 1 of OIR, the majority of the vaso-obliteration occurs within the first 48 hours of high oxygen exposure.Citation34,Citation42 Vessel regrowth has been observed as early as P9, while the mice are still in hyperoxic conditionsCitation42,Citation43; this may be a response to increasing nutritional demands of the developing retina in the setting of marked vessel loss.Citation36,Citation44 The transfer of mice to room air at P12 results in hypoxia in the regions of vaso-obliteration that stimulates pro-angiogenic factors.Citation36 This is discussed in further detail in the next section. Vessel growth induced by these angiogenic factors includes regrowth of normal vessels into areas of vaso-obliteration and pathological NV at the junction of avascular and vascularized retina (). These processes occur simultaneously but to varying degrees depending on factors such as time courseCitation45 and extent of vessel regression during phase 1Citation21,Citation36; however, the mechanisms that govern normal versus pathological vessel growth are still poorly understood.Citation45
Figure 1 Murine retinal vascular changes during OIR.
Abbreviations: OIR, oxygen-induced retinopathy; P, postnatal day; ROP, retinopathy of prematurity; VEGF, vascular endothelial growth factor.
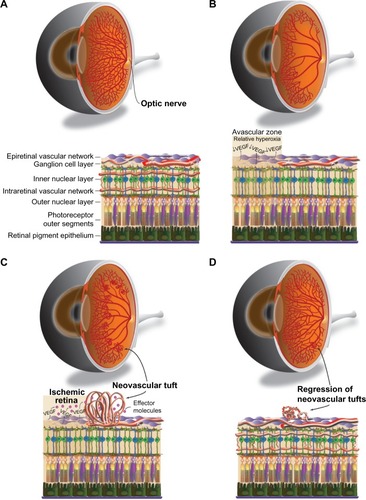
Figure 2 Control and OIR flatmounts at murine P17.
Abbreviations: OIR, oxygen-induced retinopathy; P, postnatal day.
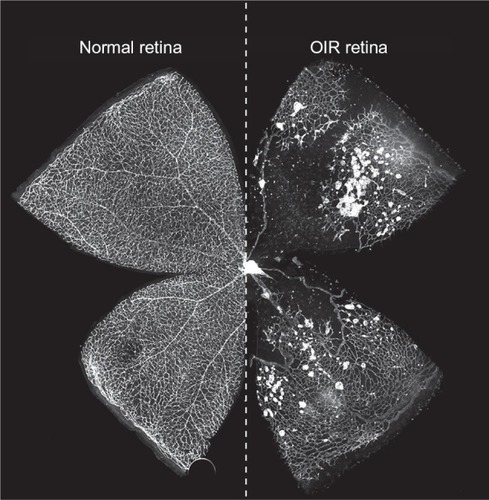
There has been some variability in how NV is assessed and quantified, as we explore later in this review, but retinal NV is generally defined as an unorganized configuration of leaky, small-caliber vessels (ie, preretinal tufts) located anterior to normal retinal vessels with frequent extension into the vitreal space ().Citation21,Citation36 NV, which develops beginning at P12 with rapid proliferation starting at P14 and peak density at P17,Citation19,Citation42 is the hallmark of phase 2 of OIR and the primary factor responsible for vision impairment. As vascular repair continues and levels of pro-angiogenic factors wane, NV begins to regress after P17 with almost complete resolution and replacement by normal vasculature by P25 (),Citation36 reflecting an inverse trend between normal revascularization and NV.Citation45 Although retinas can be harvested for analysis at any point during OIR, studies of phase 1 generally assess vaso-obliteration at P8 or P12, while NV is typically examined at P17.Citation36 A comprehensive protocol by Connor et al provides detailed instructions on experimental setup, sample collection, data analysis, and troubleshooting.Citation34
Role of angiogenic factors
Through various animal models of NV, researchers have uncovered a vast array of molecular pathways that govern developmental and pathological retinal angiogenesis. Among these, the role of vascular endothelial growth factor (VEGF) has been the most extensively studied, and VEGF is considered one of the most important mediators in this context. Initially identified as a modulator of vessel permeability,Citation46,Citation47 VEGF is a family of polypeptides produced as multiple isoforms that act via tyrosine kinase receptors.Citation48 As researchers began to explore the functions of VEGF in the eye in the 1990s, it became increasingly evident that VEGF was likely the elusive “vaso-proliferative factor” initially posited by Michaelson and Ashton almost half a century earlier.Citation48 The role of VEGF in ocular angiogenesis was initially demonstrated using a model of photocoagulation-induced ischemia in nonhuman primates. These studies revealed that ischemia-induced VEGF production in the retina is clearly associated with increased ocular NV and vascular permeability in vivo.Citation49,Citation50 Expanding on this work, Pierce et al explored the role of VEGF using the mouse OIR model, further strengthening the notion that VEGF induces pathologic retinal NV.Citation51 Stone et al demonstrated that VEGF expression is essential in normal retinal vascular development, and that high oxygen levels suppress VEGF production, thus inhibiting vessel growth and leading to the regression of nascent immature vessels.Citation52 Subsequently, Aiello et al showed that inhibition of VEGF suppresses murine retinal NV in vivo.Citation53 Taken together, these findings formed the basis for the development of anti-VEGF therapies that are now the mainstay for the treatment of choroidal NV in wet age-related macular degeneration.Citation36
Within the OIR model, retinal VEGF expression predictably correlates with disease progression. High oxygen exposure during phase 1 suppresses retinal VEGF levels and results in central retinal vaso-obliteration.Citation36,Citation54,Citation55 Upon return to room air, hypoxia in the central retina leads to the upregulation of VEGF, primarily by retinal glial cells, which in turn leads to the NV that is characteristic of phase 2.Citation36,Citation51,Citation54 The mechanisms that distinguish pathologic from developmental retinal angiogenesis are not fully understood, although it is clear that VEGF activity is vital to both processes.Citation52,Citation54 Independent of VEGF activity, the alternative complement pathway, a key component of innate immunity, has recently been shown to aid in the clearance of pathologic NV without damaging normal retinal vasculature.Citation56 Normally, retinal vessels express inhibitors of the complement system that prevent their clearance. However, a downregulation of complement inhibitors was observed exclusively on pathologic neovessels during phase 2 of OIR, thus allowing for their targeted removal by the alternative complement pathway.Citation56 Another important consideration is that specific VEGF isoforms have different roles in angiogenesisCitation57; murine VEGF164, which is analogous to human VEGF165, is the predominant isoform in the retina.Citation53,Citation56 In addition, different angiogenic responses have been observed in OIR models depending on the type of VEGF receptor activatedCitation36; it is thought that VEGF receptor-1 is involved in vascular permeability and chemotaxis, while VEGF receptor-2 is important for the survival and proliferation of endothelial cells.Citation58 Finally, it is important to note that VEGF is just one of many angiogenic factors that have been implicated in OIR; other factors, including erythropoietinCitation59 and insulin-like growth factor 1 (IGF-1, explored later in this review),Citation60 and omega-3-polyunsaturated fatty acidsCitation61 play notable roles as well.
Limitations of the mouse model of OIR
Strain and litter differences in OIR phenotype
Despite the improved reproducibility and widespread adoption of the mouse model of OIR, several important factors can result in considerable variability in the OIR phenotype if not addressed. Among these, strain-dependent variability has been a longstanding issue. Indeed, strain-dependent differences have plagued the use of the rat OIR model and may have been an obstacle to the widespread adoption of the model.Citation62 Many of the mouse OIR studies in the 1950s did not specify the strain type, and we now know that this partially contributed to the divergent results among research groups. Gyllensten and Hellström were among the first to explicitly emphasize the importance of the consistent use of a single mouse strain, C57Bl/6, given its low frequency of spontaneous eye abnormalities.Citation14 Through advances in our understanding of angiogenesis and progress in genetic techniques, it has been shown that there is considerable genetic heterogeneity in ocular angiogenesis in different strains of mice, both in response to exogenous angiogenic factorsCitation63 and in the resting vasculature.Citation64 In terms of OIR, strain-dependent differences have been observed in the context of light exposureCitation65 and expression of angiogenic factors.Citation66 Interestingly, C57Bl/6 mice were found to have increased photoreceptor vulnerability to oxygen stress compared to the BALB/cJ mouse strain,Citation65 and lower levels of angiogenesis compared to 129S3/SyIM mice.Citation66 Even when accounting for strain-dependent differences in OIR susceptibility, variability is still observed in strains purchased from different vendors, likely attributable to spontaneous mutations that have accumulated from generational inbreeding.Citation36 It is also important to account for variance within a given mouse litter,Citation34,Citation36,Citation67 which we explore in further depth through our discussions on postnatal weight gain (PWG) and hyperoxia-dependent changes on nursing mothers. In order to address these potential confounders, mouse OIR experiments should be conducted using the same strain obtained from a single vendor, intra-litter controls should be included when possible to minimize age and genetic heterogeneity, and there should be equal sampling from multiple litters. These variables are particularly important for studies using transgenic mice, in which the experimental and control mice should be from the same genetic background.Citation34,Citation36
Effect of hyperoxia on lactating mothers
One notable challenge with the use of the mouse OIR model is the toxicity of hyperoxia, not only for pups but also for their nursing mothers. The earliest OIR experiments reported that nursing mothers were less tolerant of hyperoxic conditions than their pups, prompting the use of surrogate mothers from room air controls.Citation11 Surrogate use in the mouse OIR model has varied among research groups but was often preemptively incorporated in anticipation of known complications, such as pulmonary oxygen toxicity.Citation20 However, the currently accepted OIR model limits room air surrogate use to only when the mother expires, a relatively rare occurrenceCitation19 that generally occurs on the last day of high oxygen exposure or upon return to room air (ie, P12).Citation34 Nevertheless, the stress of hyperoxia on nursing mothers can be potentially detrimental to nursing behavior and fertility. It has been reported that the detrimental effects of hyperoxia to the mother can be minimized by modifying the model to 22 hours of high oxygen and 2 hours of room air per day instead of continuous high oxygen exposure.Citation68 From our laboratory’s experience, nursing mothers are no longer fertile after exposure to OIR, and thus should not be used for breeding purposes. We have also observed an increased tendency for mothers to cannibalize their pups during exposure to hyperoxia, as well as after transfer to normoxia when their cages are handled frequently. In contrast to the OIR studies of the 1950s when pups were routinely and frequently taken from the chambers for examination and returned,Citation11 we recommend minimal handling after return to normoxia as the stress from handling can lead to decreased lactation and increased cannibalization. Since mouse pups are dependent on their nursing dam for nutrition for the first 21 days of life, essentially the time course of OIR, the health and behavior of the nursing mother or surrogate are critical factors in pup survival. We have found that switching nursing mothers with surrogates from the 129S mouse strain upon transfer to room air at P12 greatly improves PWG and survival of pups. In spite of this, it is not uncommon for OIR pups to expire more frequently than room air pups for a variety of reasons, ranging from the aforementioned maternal factors to pup nutritional status, which we explore next in this review. Thus, when calculating sample sizes, it is important to anticipate the poor survival of pups exposed to OIR conditions.
Postnatal weight gain and OIR
Despite decades of experience with the mouse OIR model, PWG has only recently come to the attention of researchers as a critical factor in disease progression. The topic has been of increasing interest in clinical studies of ROP in recent years, especially since clinical advances that account for supplemental oxygen level with respect to gestational age have decreased ROP incidence and severity.Citation69,Citation70 Indeed, screening methods that monitor PWG and IGF-1, a key factor in nutritional intake and retinal angiogenesis,Citation60,Citation71,Citation72 have been developed to reliably predict ROP.Citation73–Citation75 Nevertheless, the physiological mechanisms behind weight-dependent OIR changes are not entirely known, and this has prompted a more thorough examination of weight gain in mouse models.Citation67,Citation70 Prior research in a rat OIR model had noted an inverse relationship between the severity of NV and final weight.Citation76 Vanhaesebrouck et al observed a similar trend in mice; mice from larger litters had lower body weight, lower circulating levels of IGF-1, and more severe OIR.Citation67 Furthermore, they found that exogenous administration of IGF-1 was protective against OIR, providing the first strong evidence for IGF-1 as a therapeutic target. These findings highlight the importance of litter size as a proxy for postnatal growth and nutritional status, and current OIR protocols recommend limiting litter size to eight pups.Citation34 In another mouse OIR study by Stahl et al, pups with poor PWG (defined as <5 g at P17) had delayed and markedly prolonged vaso-obliteration and NV compared to medium weight gain (defined as 5–7.5 g) and extensive weight gain (defined as >7.5 g) pups.Citation70 The effect of surrogate use and litter size was also explored, with the results confirming the notion that smaller litter size (3–4/litter) correlates with improved weight gain and nutritional status, and that the use of additional surrogate dams at P1 leads to improved weight gain and NV similar to that observed in medium weight gain and extensive weight gain pups.Citation70 Thus, it is critical to document the use of surrogates in OIR experiments and to be mindful of litter size as these factors can markedly influence PWG and disease course.
In terms of the physiological mechanisms that may drive such changes, expression of VEGF, a potent stimulator of retinal angiogenesis,Citation51,Citation53,Citation55 was predictably elevated and prolonged in PWG pups, in a manner consistent with OIR disease course.Citation70 Additionally, IGF-1 was reduced early during phase 2 of OIR, which could lead to impaired revascularization.Citation70 Taken together, these findings highlight the importance of PWG as an independent factor in OIR disease course and severity. Recommendations have been made to record weight data in all OIR experiments, to exclude mice <6 g at P17,Citation34 and to ensure the use of consistent mouse weights when making comparisons.Citation70
Light exposure and OIR
The potential role of light exposure in ROP pathogenesis was first posited in 1942 by Terry.Citation1 Since then, clinical studies on the effect of light exposure on ROP incidence and severity have yielded conflicting results,Citation77,Citation78 likely due to the multiplicity of variables in premature infant health and treatment conditions.Citation79 Thus, in the 1990s, researchers began to explore this question in animal OIR models, initially using ratsCitation80 and kittens.Citation81 No significant differences in pattern or course of OIR were observed with changes in light exposure duration or intensity in either of these animal models.Citation80,Citation81 Expanding these studies to the mouse model of OIR, Wesolowski and Smith also did not note any adverse effects of light exposure on OIR but instead found that mice exposed to phase 1 hyper-oxia in the dark had more NV than corresponding mice in the light; they hypothesize that this difference may be due to higher metabolic demands of the retina at night.Citation79
It is important to note that rodent pups are born with fused eyelids, which means that higher light intensities are needed to reach the eye.Citation82 While no light-dependent vascular changes were noted in the OIR rat model, structural photoreceptor damage leading to progressive retinal degeneration has been observed with high-intensity light,Citation80 especially in albino Sprague Dawley rats.Citation82 Of particular interest, a recent study by Natoli et al demonstrates that exposure to 670 nm red light during OIR in mouse and rats can protect against ROP, as evidenced by decreased vaso-obliteration, NV, and abnormal vascular patterning.Citation83 This novel finding suggests that light exposure may play a more substantial role in OIR and ROP than previously thought. In the context of OIR, time-dependent variability in retinal vascular growth and the changes associated with circadian rhythms may play a bigger role than light exposure, and so it is important to start experiments and sample collection at the same time of day to minimize potential variability from such factors.Citation84
Quantifying OIR
Although the advent of lectin and fluorescein staining greatly improved the visualization of vessels in the mouse retina, assessing the pathologic vascular changes seen in OIR continues to pose a significant challenge. As mentioned previously, a major benefit of Smith’s model has been the establishment of a method to quantify NV.Citation19 Unlike prior methods that did not distinguish hyaloid and retinal NV, Smith et al were able to more accurately assess retinal NV by manually counting in retinal cross sections the nuclei of the cells of the microvessels that were invading into the vitreous.Citation19 While this cross-sectional technique has been utilized in other studies,Citation51,Citation53,Citation67,Citation85 the ability to successfully flatmount the whole mouse retina allowed for quantitative NV assessment that was previously thought to be too difficult to accomplish in mice.Citation20 The use of retinal whole-mounts has led to the development of a grading system for NV based on predefined characteristics.Citation86,Citation87 Since whole retinal flatmount analysis allows the investigator to obtain a full view of the extent of disease (versus a snapshot of particular areas seen in retinal cross sections), counting vascular nuclei in cross sections is no longer regarded as the ideal means to quantify OIR. With the advent of computer-aided imaging software, it became possible to more precisely measure the area of neovascular tufts in mouse retinal whole-mounts.Citation59,Citation61,Citation88,Citation89 Furthermore, image tracing and pixel calculations through software like Adobe Photoshop also allowed for the quantification of vaso-obliteration,Citation34 thereby providing a more comprehensive assessment of vascular changes throughout both phases of OIR.
Despite the improved accuracy of NV quantification afforded by these updated methods, all protocols are time-intensive and are somewhat subjective, given the need for manual counting, grading, or tracing of NV.Citation35 A new computer-aided method was developed in 2009 by Stahl et al using the free ImageJ software provided by the National Institutes of Health that makes the quantification process not only about five times faster but also less user-dependent and consequently more objective.Citation35 This method, termed SWIFT_NV, employs a set of macros that digitally subtract background fluorescence from overlaid images of retinal flatmounts, thus isolating neovascular tufts from normal vessels. While the approach requires manual tracing of areas of vaso-obliteration in order to overlay images, the authors argue that manual tracing of vaso-obliterated areas is much less time-consuming and subjective than manually tracing NV.Citation35 Notably, computer-aided image analysis of retinal NV was utilized in the rat model of OIRCitation90 more than a decade prior to its use in the mouse OIR model. Similar to the background subtraction method used in the SWIFT_NV approach that makes quantification more user-independent, Penn and Gay used image overlay and signal intensity threshold algorithms to the same effect ∼20 years earlier.Citation90
Even with the improved reliability, objectivity, and efficiency of the SWIFT_NV approach, a few issues still remain. The quantification of vaso-obliterated areas still requires manual tracing, making studies of phase 1 of OIR vulnerable to inter-user variability. Plus, the SWIFT_NV approach itself is technically vulnerable since it requires manual tracing. A possible solution to this potential source of bias would be to incorporate masking into image analysis, where the user would not know which experimental group he or she is quantifying until after analysis is complete. The vaso-obliterative phase of OIR is relatively understudied; however, this phase is likely to receive more attention as it has become well established that the severity of vessel regression in phase 1 influences the extent of later NV.Citation36 In addition to quantifying vascular changes in early OIR, another challenge is assessing the resolution of NV during the end stages of OIR. This is inherently difficult, given our relative lack of understanding regarding how pathological vessels regress,Citation34 although recent research from our laboratory has implicated the alternative complement pathway, a key component of our innate system, in the clearance of pathological NV.Citation56 That being said, there is a systematic approach that assesses the resolution of NV based on four features of retinal vessels: shape, diameter, fluorescent intensity, and extension from the superficial retinal layer.Citation34,Citation91
Finally, a major limitation of all of these quantitative approaches is that the mice must be sacrificed to allow for retinal isolation and staining, and so methods for in vivo quantification of NV are needed. Topical endoscopy fundus imaging (TEFI), a technique developed by Paques et al that provides high-resolution digital photographs of the retina in live mice,Citation92 has been recently adapted for use in the mouse OIR model.Citation93 Although the earliest time point that TEFI can detect retinal vascular changes is at P15, the images obtained reflect disease course that is consistent with conventional methods of assessment, and a 5-point grading system developed by Furtado et al demonstrates reasonable intra- and interobserver reliability.Citation93 The use of TEFI provides the opportunity to perform multiple retinal examinations in the same mouse to track disease course.Citation93 Another recent technique, proposed by Mezu-Ndubuisi et al, couples fluorescein angiography with oxygen-sensitive microelectrodes to quantify NV changes during OIR in live mice.Citation94 Although neither technique is as accurate as conventional methods, they allow each sample to serve as its own control, thus strengthening the robustness of OIR data.
Additional considerations
There are a few other issues to consider when using the mouse model of OIR. Genetic or immunohistochemical studies that require RNA or protein, respectively, may be hindered by the smallness of neonatal mouse pup retinas. Thus, retinas from a number of mice are often pooled to obtain adequate protein or RNA levels for quantification. Ishikawa et al have recommended that both eyes from the same mouse pup be used for RNA studies, four eyes from two pups raised under identical conditions be used for enzyme-linked immunosorbent assay, and biological replicates be used to reduce variability.Citation95 For gene expression studies, it is important to keep in mind that variable oxygen tensions can induce differential gene expression within different regions of the mouse retina.Citation96
Sex-related differences in OIR have not been thoroughly explored, possibly because of the difficulty in determining the sex of mouse pups. Smith et al claimed no such differences in their original 1994 OIR study, but they had also claimed no weight-related OIR differences in the same study,Citation19 which we know not to be true. Interestingly, Miyamoto et al have found that administration of estrogen, specifically the ovarian sex hormone E2, during either phase of OIR can modulate VEGF expression and mitigate OIR severity.Citation97 In humans, it has been observed that female preterm infants with very low birth weights have a slight survival advantage compared to their male counterparts.Citation98,Citation99 Although clinical studies have not observed sex-related differences in ROP incidence,Citation100 Yang et al found that sex was an independent risk factor for ROP that warrants surgery, with male infants more likely to undergo such procedures.Citation101 Regardless of whether the sex of mouse pups affects OIR disease course, modulation of sex hormones may play a therapeutic role for ROP in the clinical setting.
Finally, it is worth noting that the mouse model of OIR does not replicate certain aspects of ROP seen in humans. Given improvements in supplemental oxygen dosing for preterm infants, the constant exposure of mice to 75% oxygen is much higher and less cyclical than what premature human infants experience,Citation37 which is actually closer to the 50/10 fluctuating OIR model in rats.Citation38 Additionally, no gestational manipulation occurs with the mouse OIR model since experiments are conducted postnatally, which means that other complications of premature birth are not reproduced.Citation37 While other animal OIR models (eg, kittens, beagles, rats) more closely resemble human ROP,Citation37,Citation102 the mouse OIR model remains the quickest and least expensive, with the broadest transgenic and antibody-staining capabilities.
Conclusion
Application of the mouse OIR model to current and future research
A Google Scholar search of “oxygen induced retinopathy mice” (July 29, 2015) indicates that there are ∼35,500 publications to date on the mouse model of OIR, with about half of these publications coming after the last such search conducted by Stahl et al in 2009.Citation36 This vast number reflects the ease with which researchers using this model can investigate a broad range of topics. Among these, the role of the immune system has been of particular interest, with hypotheses on the role of inflammation in ocular NV well preceding Smith’s work.Citation103 The complement system, a fundamental component of innate immunity, has recently been studied in the context of OIR, and has been shown to mediate retinal angiogenesis through clearance of pathologic neovessels.Citation56,Citation104 Although leukocytes have also been shown to mediate retinal vascular remodeling in OIR,Citation105 neuroinflammation in the retina is still a relatively unexplored topic. Potential neurovascular crosstalk, specifically between retinal glial and neuronal cells, is becoming of interest in this context.Citation106–Citation108 Additionally, the role of oxidative stress has been aptly studied in relation to the model,Citation109–Citation112 with recent work demonstrating a critical role for endogenous and synthetic antioxidants in countering OIR.Citation68,Citation113 Modulation of beta-adrenergic receptor activity has been shown to alter angiogenic activity in OIR.Citation114,Citation115 Indeed, insights gained from the mouse OIR model, coupled with technological advancements in immunostaining and genetic manipulation, may help improve or lead to new animal models of vaso-proliferative retinopathies,Citation116 such as the development of a zebrafish model of hypoxia-induced retinopathy.Citation117,Citation118 Undoubtedly, the impact of the mouse OIR model has been significant: what began as an attempt to replicate ROP in mice has developed into a robust platform for investigating angiogenesis, neuroinflammation, and novel therapeutic strategies for many ocular conditions.
Acknowledgments
This study was supported by US National Institutes of Health/National Eye Institute Grant R01EY022084/S1 (to KMC), Research to Prevent Blindness (RPB) Special Research Scholar Award (to KMC), RPB Medical Student Fellowship Grant (to CBK), and a Fight for Sight, Inc. Student Fellowship Award (to CBK).
Disclosure
The authors report no conflicts of interest related to this work.
References
- TerryTLFibroblastic overgrowth of persistent tunica vasculosa lentis in infants born prematurely: II. Report of cases-clinical aspectsTrans Am Ophthalmol Soc19424026228416693285
- TerryTLRetrolental fibroplasia in the premature infant: V. Further studies on fibroplastic overgrowth of the persistent tunica vasculosa lentisTrans Am Ophthalmol Soc19444238339616693360
- WilsonJLLongSBHowardPJRespiration of premature infantsAm J Dis Child19426361080
- TinWGuptaSOptimum oxygen therapy in preterm babiesArch Dis Child Fetal Neonatal Ed2007922F143F14717337663
- BedrossianRHCarmichaelPRitterJRetinopathy of prematurity (retrolental fibroplasia) and oxygenAm J Ophthalmol1954371788613114325
- ZachariasLReynoldsWEChisholmJFKingMJThe incidence and severity of retrolental fibroplasia in relation to possible causative factors. I. Observations on the occurrence of retrolental fibroplasia. II. Studies of the relationship of retrolental fibroplasia to degree of prematurity, oxygen therapy, general health, and date of birth of premature infantsAm J Ophthalmol195438331733613188934
- ParmeleeAHPilgerISAustinWORetrolental fibroplasia; a reduction in incidence following a decrease in use of oxygen therapy for premature infantsCalif Med195684642442613316553
- SzewczykTSRetrolental fibroplasia; etiology and prophylaxisAm J Ophthalmol195235330131114903018
- SzewczykTSRetrolental fibroplasia and related ocular diseases; classifica tion, etiology, and prophylaxisAm J Ophthalmol195336101336136113092191
- AshtonNWardBSerpellGEffect of oxygen on developing retinal vessels with particular reference to the problem of retrolental fibroplasiaBr J Ophthalmol195438739743213172417
- GerschmanRNadigPWSnellACNyeSWEffect of high oxygen concentrations on eyes of newborn miceAm J Physiol1954179111511813207397
- MichaelsonICThe mode of development of the vascular system of the retina, with some observations on its significance for certain retinal diseasesTrans Ophthalmol Vis Sci194868137180
- AshtonNWardBSerpellGRole of oxygen in the genesis of retrolental fibroplasiaBr J Ophthalmol195337951352013081949
- GyllenstenLJHellströmBEExperimental approach to the pathogenesis of retrolental fibroplasiaBr J Ophthalmol195539740941513240034
- GyllenstenLJHellströmBERetrolental fibroplasia; animal experiments: the effect of intermittently administered oxygen on the postnatal development of the eyes of full term miceActa Paediatr195241657758213016280
- PatzAEasthamAHigginbothamDHKlehTOxygen studies in retrolental fibroplasiaAm J Ophthalmol195336111511152213104558
- HabeggerHRandolf PhilbrookFCurleyFJIngallsTHRetinopathy of immaturityAm J Ophthalmol195642337739213362441
- GyllenstenLJHellströmBEExperimental approach to the pathogenesis of retrolental fibroplasia. I. Changes of the eye induced by exposure of newborn mice to concentrated oxygenActa Paediatr Suppl19544310013114813228020
- SmithLEHWesolowskiEMcLellanAOxygen-induced retinopathy in the mouseInvest Ophthalmol Vis Sci19943511011117507904
- GoleGABrowningJEltsSMThe mouse model of oxygen-induced retinopathy: a suitable animal model for angiogenesis researchDoc Ophthalmol19907431631692209373
- PatzAI. Studies on retinal NVInvest Ophthalmol Vis Sci1980101011331138
- BischoffPMWajerSDFlowerRWScanning electron microscopic studies of the hyaloid vascular system in newborn mice exposed to O2 and CO2Graefe’s Arch Clin Exp Ophthalmol198322062572636195049
- NovotnyHRAlvisDLA method of photographing fluorescence in circulating blood in the human retinaCirculation196124828613729802
- KeanePASaddaSRRetinal imaging in the twenty-first centuryOphthalmology2014121122489250025282252
- BlanksJCJohnsonLVSelective lectin binding of the developing mouse retinaJ Comp Neurol1983221131416643744
- ConnollySEHoresTASmithLEHD’AmorePACharacterization of vascular development in the mouse retinaMicrovasc Res19883632752902466191
- D’AmatoRWesolowskiESmithLEMicroscopic visualization of the retina by angiography with high-molecular-weight fluorescein-labeled dextrans in the mouseMicrovasc Res19934621351427504160
- FolkmanJMerlerEAbernathyCWilliamsGIsolation of a tumor factor responsible for angiogenesisJ Exp Med197113322752884332371
- GlaserBMD’AmorePAMichelsRGThe demonstration of angiogenic activity from ocular tissues. Preliminary reportOphthalmology19808754404466157142
- GlaserBMD’AmorePAMichelsRGPatzAFenselauADemonstration of vasoproliferative activity from mammalian retinaJ Cell Biol19808422983046155381
- FolkmanJKlagsbrunMAngiogenic factorsScience198723547874424472432664
- AshtonNSome aspects of the comparative pathology of oxygen toxicity in the retinaOphthalmologica1970160154715415321
- PhelpsDLRosenbaumALEffects of marginal hypoxemia on recovery from oxygen-induced retinopathy in the kitten modelPediatrics1984731166546319
- ConnorKMKrahNMDennisonRJQuantification of oxygen-induced retinopathy in the mouse: a model of vessel loss, vessel regrowth and pathological angiogenesisNat Protoc20094111565157319816419
- StahlAConnorKMSapiehaPComputer-aided quantification of retinal NVAngiogenesis200912329730119757106
- StahlAConnorKMSapiehaPThe mouse retina as an angiogenesis modelInvest Ophthalmol Vis Sci20105162813282620484600
- HartnettMEPathophysiology and mechanisms of severe retinopathy of prematurityOphthalmology2015122120021025444347
- MadanAPennJSAnimal models of oxygen-induced retinopathyFront Biosci200381210301043
- DorrellMIFriedlanderMMechanisms of endothelial cell guidance and vascular patterning in the developing mouse retinaProg Retin Eye Res200625327729516515881
- AguilarEDorrellMIFriedlanderDChapter 6. Ocular models of angiogenesisMethods Enzymol200844411515819007663
- RepkaMXPalmerEATungBInvolution of retinopathy of prematurity. Cryotherapy for Retinopathy of Prematurity Cooperative GroupArch Ophthalmol2000118564564910815156
- LangeCEhlkenCStahlAMartinGHansenLAgostiniHTKinetics of retinal vaso-obliteration and NV in the oxygen-induced retinopathy (OIR) mouse modelGraefe’s Arch Clin Exp Ophthalmol200924791205121119526246
- GuXSamuelSEl-ShabraweyMEffects of sustained hyperoxia on revascularization in experimental retinopathy of prematurityInvest Ophthalmol Vis Sci200243249650211818396
- OzakiHYuAYDellaNHypoxia inducible factor-1alpha is increased in ischemic retina: temporal and spatial correlation with VEGF expressionInvest Ophthalmol Vis Sci19994011821899888442
- ScottAFruttigerMOxygen-induced retinopathy: a model for vascular pathology in the retinaEye (Lond)201024341642120010791
- SengerDGalliSDvorakAPerruzziCHarveyVDvorakHTumor cells secrete a vascular permeability factor that promotes accumulation of ascites fluidScience198321945879839856823562
- FerraraNHenzelWJPituitary follicular cells secrete a novel heparin-binding growth factor specific for vascular endothelial cellsBiochem Biophys Res Commun198916128518582735925
- D’AmorePAMechanisms of retinal and choroidal NVInvest Ophthalmol Vis Sci19943512397439797525506
- MillerJWAdamisAPShimaDTVascular endothelial growth factor/vascular permeability factor is temporally and spatially correlated with ocular angiogenesis in a primate modelAm J Pathol199414535745847521577
- ShimaDTGougosAMillerJWCloning and mRNA expression of vascular endothelial growth factor in ischemic retinas of Macaca fascicularisInvest Ophthalmol Vis Sci1996377133413408641836
- PierceEAAveryRLFoleyEDAielloLPSmithLEVascular endothelial growth factor/vascular permeability factor expression in a mouse model of retinal NVProc Natl Acad Sci U S A19959239059097846076
- StoneJItinAAlonTDevelopment of retinal vasculature is mediated by hypoxia-induced vascular endothelial growth factor (VEGF) expression by neurogliaJ Neurosci1995157 Pt 1473847477623107
- AielloLPPierceEAFoleyEDSuppression of retinal NV in vivo by inhibition of vascular endothelial growth factor (VEGF) using soluble VEGF-receptor chimeric proteinsProc Natl Acad Sci U S A1995922310457104617479819
- AlonTHemoIItinAPe’erJStoneJKeshetEVascular endothelial growth factor acts as a survival factor for newly formed retinal vessels and has implications for retinopathy of prematurityNat Med1995110102410287489357
- PierceEAFoleyEDSmithLERegulation of vascular endothelial growth factor by oxygen in a model of retinopathy of prematurityArch Ophthalmol199611410121912288859081
- SweigardJHYanaiRGaissertPThe alternative complement pathway regulates pathological angiogenesis in the retinaFASEB J20142873171318224668752
- StalmansINgYSRohanRArteriolar and venular patterning in retinas of mice selectively expressing VEGF isoformsJ Clin Invest2002109332733611827992
- FerrariGCookBDTerushkinVPintucciGMignattiPTransforming growth factor-beta 1 (TGF-beta1) induces angiogenesis through vascular endothelial growth factor (VEGF)-mediated apoptosisJ Cell Physiol2009219244945819180561
- ChenJConnorKMAdermanCMSmithLEHErythropoietin deficiency decreases vascular stability in miceJ Clin Invest2008118252653318219389
- LofqvistCWillettKLAspegrenOQuantification and localization of the IGF/insulin system expression in retinal blood vessels and neurons during oxygen-induced retinopathy in miceInvest Ophthalmol Vis Sci20095041831183718997086
- ConnorKMSanGiovanniJPLofqvistCIncreased dietary intake of omega-3-polyunsaturated fatty acids reduces pathological retinal angiogenesisNat Med200713786887317589522
- Van WijngaardenPCosterDJBreretonHMGibbinsILWilliamsKAStrain-dependent differences in oxygen-induced retinopathy in the inbred ratInvest Ophthalmol Vis Sci20054641445145215790914
- RohanRMFernandezAUdagawaTYuanJD’AmatoRJGenetic heterogeneity of angiogenesis in miceFASEB J200014787187610783140
- ChanCKPhamLNChinnCMouse strain-dependent heterogeneity of resting limbal vasculatureInvest Ophthalmol Vis Sci200445244144714744883
- WalshNBravo-NuevoAGellerSStoneJResistance of photoreceptors in the C57BL/6-c2J, C57BL/6J, and BALB/cJ mouse strains to oxygen stress: evidence of an oxygen phenotypeCurr Eye Res200429644144715764088
- ChanCKPhamLNZhouJSpeeCRyanSJHintonDRDifferential expression of pro- and antiangiogenic factors in mouse strain-dependent hypoxia-induced retinal NVLab Invest200585672173315856049
- VanhaesebrouckSDanielsHMoonsLVanholeCCarmelietPDe ZegherFOxygen-induced retinopathy in mice: amplification by neonatal IGF-I deficit and attenuation by IGF-I administrationPediatr Res200965330731019092722
- TanSMStefanovicNTanGWilkinson-BerkaJLde HaanJBLack of the antioxidant glutathione peroxidase-1 (GPx1) exacerbates retinopathy of prematurity in miceInvest Ophthalmol Vis Sci201354155556223287791
- SearsJEPietzJSonnieCDolciniDHoppeGA change in oxygen supplementation can decrease the incidence of retinopathy of prematurityOphthalmology2009116351351819157560
- StahlAChenJSapiehaPPostnatal weight gain modifies severity and functional outcome of oxygen-induced proliferative retinopathyAm J Pathol201017762715272321056995
- BergmanPBMoravskiCJEdmondsonSRExpression of the IGF system in normal and diabetic transgenic (mRen-2)27 rat eyeInvest Ophthalmol Vis Sci20054682708271516043842
- Wilkinson-BerkaJLWraightCWertherGThe role of growth hormone, insulin-like growth factor and somatostatin in diabetic retinopathyCurr Med Chem200613273307331717168853
- HellströmAHårdA-LEngströmEEarly weight gain predicts retinopathy in preterm infants: new, simple, efficient approach to screeningPediatrics20091234e638e64519289449
- LöfqvistCAnderssonESigurdssonJLongitudinal postnatal weight and insulin-like growth factor I measurements in the prediction of retinopathy of prematurityArch Ophthalmol2006124121711171817159030
- LöfqvistCHansen-PuppIAnderssonEValidation of a new retinopathy of prematurity screening method monitoring longitudinal postnatal weight and insulinlike growth factor IArch Ophthalmol2009127562262719433710
- HolmesJMDuffnerLAThe effect of postnatal growth retardation on abnormal NV in the oxygen exposed neonatal ratCurr Eye Res19961544034098670740
- AckermanBSherwonitEWilliamsJReduced incidental light exposure: effect on the development of retinopathy of prematurity in low birth weight infantsPediatrics19898369589622726352
- GlassPAveryGBSubramanianKNKeysMPSostekAMFriendlyDSEffect of bright light in the hospital nursery on the incidence of retinopathy of prematurityN Engl J Med198531374014043839567
- WesolowskiESmithLEEffect of light on oxygen-induced retinopathy in the mouseInvest Ophthalmol Vis Sci19943511121197507905
- RicciBLeporeDIossaMSantoAD’ursoMMaggianoNEffect of light on oxygen-induced retinopathy in the rat modelDoc Ophthalmol19907442873011701697
- KremerILevittAGoldbergGWilunskyEMerlobPNissenkornIThe effect of light on oxygen-induced vasoproliferative retinopathy in newborn kittensInvest Ophthalmol Vis Sci1992335159515981559758
- DorfmanALJolySHardyPChemtobSLachapellePThe effect of oxygen and light on the structure and function of the neonatal rat retinaDoc Ophthalmol20081181375418483821
- NatoliRValterKBarbosaM670nm photobiomodulation as a novel protection against retinopathy of prematurity: evidence from oxygen induced retinopathy modelsPLoS One201388e7213523951291
- EmbersonJRWhincupPHWalkerMThomasMAlbertiKGMMBiochemical measures in a population-based study: effect of fasting duration and time of dayAnn Clin Biochem200239Pt 549350112227856
- SmithLEShenWPerruzziCRegulation of vascular endothelial growth factor-dependent retinal NV by insulin-like growth factor-1 receptorNat Med19995121390139510581081
- HigginsRDYuKSandersRJNandgaonkarBNRotschildTRifkinDBDiltiazem reduces retinal NV in a mouse model of oxygen induced retinopathyCurr Eye Res1999181202710075199
- LangeCEhlkenCMartinGIntravitreal injection of the heparin analog 5-amino-2-naphthalenesulfonate reduces retinal NV in miceExp Eye Res200785332332717662276
- RitterMRBaninEMorenoSKAguilarEDorrellMIFriedlanderMMyeloid progenitors differentiate into microglia and promote vascular repair in a model of ischemic retinopathyJ Clin Invest2006116123266327617111048
- BaninEDorrellMIAguilarET2-TrpRS inhibits preretinal NV and enhances physiological vascular regrowth in OIR as assessed by a new method of quantificationInvest Ophthalmol Vis Sci20064752125213416639024
- PennJSGayCAComputerized digital image analysis of retinal vessel density: application to normoxic and hyperoxic rearing of the newborn ratExp Eye Res19925433293361521565
- DaviesMHStempelAJPowersMRMCP-1 deficiency delays regression of pathologic retinal NV in a model of ischemic retinopathyInvest Ophthalmol Vis Sci20084994195420218487365
- PaquesMGuyomardJLSimonuttiMPanretinal, high-resolution color photography of the mouse fundusInvest Ophthalmol Vis Sci20074862769277417525211
- FurtadoJMDaviesMHChoiDImaging retinal vascular changes in the mouse model of oxygen-induced retinopathyTransl Vis Sci Technol201212524049705
- Mezu-NdubuisiOJTengPWanekJIn vivo retinal vascular oxygen tension imaging and fluorescein angiography in the mouse model of oxygen-induced retinopathyInvest Ophthalmol Vis Sci201354106968697224052641
- IshikawaKYoshidaSKadotaKGene expression profile of hyperoxic and hypoxic retinas in a mouse model of oxygen-induced retinopathyInvest Ophthalmol Vis Sci2010518430720220049
- ZhuYNatoliRValterKStoneJDifferential gene expression in mouse retina related to regional differences in vulnerability to hyperoxiaMol Vis20101674075520454693
- MiyamotoNMandaiMTakagiHContrasting effect of estrogen on VEGF induction under different oxygen status and its role in murine ROPInvest Ophthalmol Vis Sci20024362007201412037012
- BrothwoodMWolkeDGamsuHBensonJCooperDPrognosis of the very low birthweight baby in relation to genderArch Dis Child19866165595642425752
- PhelpsDLBrownDRTungB28-day survival rates of 6676 neonates with birth weights of 1250 grams or lessPediatrics19918717171984621
- PalmerEAFlynnJTHardyRJIncidence and early course of retinopathy of prematurity. The Cryotherapy for Retinopathy of Prematurity Cooperative GroupOphthalmology19919811162816401800923
- YangMBDonovanEFWaggeJRRace, gender, and clinical risk index for babies (CRIB) score as predictors of severe retinopathy of prematurityJ AAPOS200610325326116814180
- FlowerRWMcLeodDSLuttyGAGoldbergBWajerSDPostnatal retinal vascular development of the puppyInvest Ophthalmol Vis Sci19852679579683159707
- ShaboALMaxwellDSInsulin-induced immunogenic retinopathy resembling the retinitis proliferans of diabetesTrans Sect Ophthalmol Am Acad Ophthalmol Otolaryngol1976813 Pt 1497508
- LangerHFChungK-JOrlovaVVComplement-mediated inhibition of NV reveals a point of convergence between innate immunity and angiogenesisBlood2010116224395440320625009
- IshidaSYamashiroKUsuiTLeukocytes mediate retinal vascular remodeling during development and vaso-obliteration in diseaseNat Med20039678178812730690
- VesseyKAWilkinson-BerkaJLFletcherELCharacterization of retinal function and glial cell response in a mouse model of oxygen-induced retinopathyJ Comp Neurol2011519350652721192081
- DorrellMIAguilarEJacobsonRMaintaining retinal astrocytes normalizes revascularization and prevents vascular pathology associated with oxygen-induced retinopathyGlia2010581435419544395
- LiuXWangDLiuYNeuronal-driven angiogenesis: role of NGF in retinal NV in an oxygen-induced retinopathy modelInvest Ophthalmol Vis Sci20105173749375720207957
- SaitoYGeisenPUppalAHartnettMEInhibition of NAD(P)H oxidase reduces apoptosis and avascular retina in an animal model of retinopathy of prematurityMol Vis20071384085317615545
- Wilkinson-BerkaJLDeliyantiDRanaINADPH oxidase, NOX1, mediates vascular injury in ischemic retinopathyAntioxid Redox Signal201420172726274024053718
- WangHZhangSXHartnettMESignaling pathways triggered by oxidative stress that mediate features of severe retinopathy of prematurityJAMA Ophthalmol20131311808523307212
- LiS-YFuZJLoACYHypoxia-induced oxidative stress in ischemic retinopathyOxid Med Cell Longev2012201242676923125893
- TanSMDeliyantiDFiggettWATaliaDMde HaanJBWilkinson-BerkaJLEbselen by modulating oxidative stress improves hypoxia-induced macroglial Müller cell and vascular injury in the retinaExp Eye Res20151361825912997
- RistoriCFilippiLDal MonteMRole of the adrenergic system in a mouse model of oxygen-induced retinopathy: antiangiogenic effects of beta-adrenoreceptor blockadeInvest Ophthalmol Vis Sci201152115517020739470
- MartiniDMonteMDRistoriCAntiangiogenic effects of β2-adrenergic receptor blockade in a mouse model of oxygen-induced retinopathyJ Neurochem201111961317132921988318
- GrossniklausHEKangSJBerglinLAnimal models of choroidal and retinal NVProg Retin Eye Res201029650051920488255
- CaoZJensenLDRouhiPHypoxia-induced retinopathy model in adult zebrafishNat Protoc20105121903191021127484
- CaoRJensenLDESöllIHauptmannGCaoYHypoxia-induced retinal angiogenesis in zebrafish as a model to study retinopathyPLoS One200837e274818648503