Abstract
Infectious diseases are known as one of the most life-threatening disabilities worldwide. Approximately 13 million deaths related to infectious diseases are reported each year. The only way to combat infectious diseases is by chemotherapy using antimicrobial agents and antibiotics. However, due to uncontrolled and unnecessary use of antibiotics in particular, surviving bacteria have evolved resistance against several antibiotics. Emergence of multidrug resistance in bacteria over the past several decades has resulted in one of the most important clinical health problems in modern medicine. For instance, approximately 440,000 new cases of multidrug-resistant tuberculosis are reported every year leading to the deaths of 150,000 people worldwide. Management of multidrug resistance requires understanding its molecular basis and the evolution and dissemination of resistance; development of new antibiotic compounds in place of traditional antibiotics; and innovative strategies for extending the life of antibiotic molecules. Researchers have begun to develop new antimicrobials for overcoming this important problem. Recently, platensimycin – isolated from extracts of Streptomyces platensis – and its analog platencin have been defined as promising agents for fighting multidrug resistance. In vitro and in vivo studies have shown that these new antimicrobials have great potential to inhibit methicillin-resistant Staphylococcus aureus, vancomycin-resistant enterococci, and penicillin-resistant Streptococcus pneumoniae by targeting type II fatty acid synthesis in bacteria. Showing strong efficacy without any observed in vivo toxicity increases the significance of these antimicrobial agents for their use in humans. However, at the present time, clinical trials are insufficient and require more research. The strong antibacterial efficacies of platensimycin and platencin may be established in clinical trials and their use in humans for coping with multidrug resistance may be allowed in the foreseeable future.
Introduction to managing drug resistance in the treatment of bacterial infections
Infectious diseases that are caused by bacteria, fungi, parasites, and viruses can arise from the invasion of a host cell by one of these pathogens. Infectious diseases are one of the most life-threatening groups of disabilities worldwide. According to World Health Organization data, approximately 13 million deaths related to infectious diseases have been reported worldwide. It is also known that infectious diseases cause 45% of all deaths in developing countries. At present, six groups of disabilities are considered the most lethal infectious diseases since they lead to 90% of total deaths related to infectious diseases: acute respiratory infections, acquired immunodeficiency syndrome, diarrheal diseases, tuberculosis (TB), malaria, and measles.Citation1,Citation2 Besides these illnesses, other tropical diseases, some sexually transmitted disabilities, and nosocomial infections can also threaten health of humans and cause death. The only way to combat infectious diseases is by chemotherapy using antimicrobial agents and antibiotics. Antimicrobial agents have been used for >70 years in the treatment of infectious diseases. These agents have great potential to reduce illnesses and treat affected patients. Since their first introduction, use of antibiotics has become widespread around the world because of their significant effectiveness on microbial agents.Citation3 However, due to their uncontrolled and unnecessary use, bacteria have evolved resistance against several antibiotics.Citation4 This situation has led to a reduction in effectiveness of conventional therapy (antibiotics). The development of resistance in bacteria is thought to be due to natural selection/adaptation. According to this mechanism, susceptible bacteria are inhibited and do not divide or die when exposed to an antimicrobial agent, while remaining resistant bacteria maintain viability. Resistant organisms multiply and spread to different geographic regions of the world. Moreover, resistant organisms can also transfer their resistant genes to susceptible individuals and make them more resistant to antimicrobial agents.Citation5,Citation6 Natural selection of resistant organisms occurs so rapidly in bacteria that some antibiotics have lost effectiveness in bacteria within a few months after its administration.Citation7
Currently, antibiotic resistance of microbial agents has become a serious global concern. For instance, about 440,000 new cases of multidrug-resistant (MDR) TB are reported every year, leading to the deaths of 150,000 people worldwide.Citation8 The prevalence of Staphylococcus aureus strains that are resistant to methicillin has reached high levels worldwide.Citation9 The number of methicillin-resistant S. aureus (MRSA) strains has increased at a rate of 40% in the last 10 years.Citation10 Since S. aureus is one of the most commonly seen causative agents of nosocomial infections, MRSA has recently become the most fatal bacteria in intensive care units of hospitals. Similarly, the rate of resistant Streptococcus pyogenes strains worldwide are 80% for erythromycin and 50% for penicillin, which are otherwise known as effective antibiotics.Citation11 Another big problem related to drug resistance has emerged for malaria. It is known that 30% of all people worldwide are at risk of malarial infection, and every year 1.5 million people infected with malaria die worldwide.Citation12 Moreover, this number is expected to increase in the future since Plasmodium spp. have evolved resistance against chloroquine, which had been the most effective drug used in treatment of this illness. All of these data reveal that drug resistance is one of the most important risks that threaten the health of the human population. In response, researchers have begun to develop new approaches to overcome this important problem. Among these approaches, combined use of conventional antibiotics composes one of the preliminary efforts. This application depends on enhancement of the mode of action mechanisms in addition to decreasing toxicity by using low dosages of different kinds of antibiotics with distinct effects on bacteria.Citation13,Citation14 Another approach is the use of plant derivatives, such as extracts and essential oils, and their applications combined with current antibiotics.Citation15,Citation16 On the other hand, some groups have focused on the molecular mechanisms of resistance and have targeted the prevention of the expression of resistance genes within bacteria.Citation17 There are also efforts directed toward the synthesis of new antibiotics and antimicrobial agents. Researchers are developing new drugs with different mechanisms of action that are expected to replace current antibiotics. One of these new synthesized antibiotics is platensimycin, with its promising antibacterial efficacy. This review considers the threat of drug resistance in the world in detail and then introduces effective mechanisms, pharmacologic properties, efficacy, and clinical trials of platensimycin for its use in the clinical setting.
Drug resistance in significant pathogens
Antimicrobial resistance of pathogens is increasing yearly. Recently, vancomycin-resistant Enterococcus, MDR TB, MRSA, and amantadine/rimantadine-resistant and oseltamivir-resistant influenza virus have been a serious public health threat.Citation18 Nearly every major pathogen has obtained resistance to a therapeutic agent. Resistant pathogens can lead to serious infections. They are managed with the few alternative drugs available for treatment. Streptococcus pneumoniae is an example of these criteria. In 2006, national surveys of invasive pneumococcal disease in the USA were 5,000 deaths and 41,400 cases. Meningitis caused 6.1% of cases, and bacteremia without focus caused 23.0%.Citation19 The case/fatality ratio may be higher than 25% for specific high-risk groups with meningitis and bacteremia despite proper treatment.Citation20 In 1994, drug-resistant S. pneumoniae became a nationwide notifiable disease in the USA.Citation21 In 2006, 21.6% of the resistant isolates were nonsusceptible to erythromycin, 22.7% were nonsusceptible to trimethoprim/sulfamethoxazole, and 25.6% were nonsusceptible to penicillin.Citation22 Chloroquine-resistant malaria is a worldwide problem of public health importance; 41.0% of the world’s population live in malaria-transmitted areas.Citation23 Every year, 350–500 million cases of malaria occur, and nearly one million people die.Citation24 Plasmodium falciparum resistance to chloroquine has been confirmed in Egypt, Central America west of the Panama Canal, Haiti, Dominican Republic, and other countries in the Middle East.Citation25 In some regions, most parasites (90%) may be resistant to chloroquine.Citation26 Contributors to the progression of parasitic resistance have been the limited number of attainable antimalarial drugs and defective dosing for malaria treatment.Citation27 TB’s public health implications have become more significant as the pathogen has become more and more resistant to antimicrobial therapy. In 2006, nearly 14,000 TB cases were examined in the USA.Citation28 The second most prevalently reported notifiable disease in the USA is TB. Gonorrhea is an additional example of a great antimicrobial resistance concern. In 2006, nearly 14% of isolates were tested resistant to fluoroquinolones, which was an increase from 2.2% in 2002.Citation29
Resistance of Gram-positive bacteria
Pneumococci
S. pneumoniae is the causative agent of diseases, such as pneumonia, meningitis, otitis media, and bacteremia. Infection of S. pneumoniae mainly results in higher death rates among younger, older, and immunocompromised people.Citation30 Resistance to penicillin in S. pneumoniae was first detected in 1967. Since then the penicillin-resistant strain of S. pneumoniae has become progressively prevalent worldwide.Citation31 Variants of the Spain23F-1 or Spain6B-2 clones were the predominant penicillin nonsusceptible S. pneumoniae (PNSP) isolated from 1994–1997 and remained so, but Taiwan19F-14 and Taiwan serotype 6B clones were disseminated in Hong Kong in 1999 and 2000.Citation32 The emergent clones are penicillin nonsusceptible. PNSP clones are generally resistant to tetracyclines, cotrimoxazole, chloramphenicol, and macrolides. Recent studies have indicated that the prevalence of PNSP between clinical isolates of S. pneumoniae is >40% in 16 of 60 countries observed, and <5% in only three of them.Citation33 An S. pneumoniae isolate is measured to lack susceptibility when penicillin’s minimal inhibitory concentration (MIC) is >0.06 mg/L,Citation34 and is related to a PNSP.
Enterococcus
Enterococci have stood out among Gram-positive bacteria because of their essential relevant resistance to multiple antimicrobials. In addition, they have the function to obtain or improve characteristics that render them resistant to even higher concentrations of several antibiotics.Citation35 The revelation of vancomycin resistance among strains of Enterococcus in the late 1980s was a crushing development.Citation35 Enterococci, especially Enterococcus faecalis, are one of the serious causative factors of infective endocarditis. Unluckily, vancomycin, penicillin, and other cell wall-active antimicrobials repress but do not destroy enterococci.Citation36 High-level streptomycin resistance (MIC 2000 μg/mL) in enterococci can result from adenylylation of aminoglycosides. Aminoglycoside-inactivating enzymes make it inactive against the ribosomal target.Citation37
High-level kanamycin resistance (MIC 2000 μg/mL) was already clearly typical among enterococci by the 1970s.Citation38 Therefore, for gentamicin, an MIC >500 μg/mL indicates high-level resistance. In the USA, it was found that 3.6% of enterococci isolated in hospitals with >500 patients were resistant to vancomycin, but only 1.8% in hospitals with <200 patients. Enterococcal vancomycin resistance in intensive care unit patients increased from 0.4% to 13.6% between 1989 and 1993. Most of the reports from the USA only mentioned vancomycin-resistant enterococci, as vancomycin remains their only commercially available glycopeptide.Citation39
MRSA
According to a USA study in 2007, 880,000 people are infected by MRSA every year and 2.4% of the patients stay in different hospital units annually. This type of infection also leads to six extra hospital days on average for patients. Therefore, MRSA infection results in $8 billion in total costs annually. Unfortunately, 5% of people with MRSA infection are dying and this number corresponds to 20,000–40,000 deaths per year.Citation40 Methicillin-resistant strains of S. aureus have the ability to grow in medium that includes methylpenicillin and derivatives, including oxacillin, nafcillin, and methicillin. This resistance is induced by expression of a modified penicillin-binding protein, PBP2a.Citation41 Methicillin-susceptible strains are repressed by oxacillin at concentrations of 4 μg/mL or methicillin at 8 μg/mL. MRSA grow in the presence of 16 μg/mL to >2,000 μg/mL of methicillin. Through the application of high antibiotic concentrations, S. aureus developed various resistance mechanisms against penicillin, including methicillin. Despite the fact that methicillin is resistant to hydrolysis by staphylococcal β-lactamase, S. aureus strains that produce large amounts of β-lactamase have been isolated.Citation42 These hyperproducers of β-lactamase tend to resist methicillin through limited hydrolysis of the antibiotic, resulting in a phenotype that, with respect to methicillin, is intermediate between susceptible and resistant.Citation42 There is an obvious need for more effective antibiotic therapy for MRSA infections. Reports describing treatment failure of vancomycin for MDR MRSA infections have raised concern for the emergence of strains of MRSA for which there will be no effective therapy.
Resistance of Gram-negative bacteria
Gram-negative bacteria are major causal agents of infections such as urinary tract infections, otitis media, sexually transmitted diseases, acute and chronic bronchitis, and community-acquired pneumonia. These diseases are frequently caused by Gram-negative organisms. Gramnegative microorganisms that are mostly seen in primary care units include Escherichia coli (urinary tract infection), Haemophilus influenzae (community-acquired pneumonia and bronchitis), Klebsiella pneumoniae (community-acquired pneumonia and urinary tract infection), Neisseria gonorrhoeae (gonorrhea), and Neisseria meningitidis (meningitis). With increasing antimicrobial use in society, a relative shortage of new antimicrobials with novel mechanisms of action and increases in outpatient therapy for many diseases have resistance issues in the ambulatory setting.
Recently, resistance to antimicrobial agents mostly used to treat Gram-negative pathogens has increased. Excessive use of antibiotics, uncontrolled infection, and plasmid-mediated resistance gene transfer all play important roles in this emerging threat. Reports of Gram-negative pathogens resistant to all commonly used antimicrobial agents have become progressively commonplace, both in the USA and abroad. One example of the genetic adaptability of these organisms, Pseudomonas aeruginosa often combines multiple mechanisms to result in broad-spectrum resistance.Citation43 It has been described as a public health crisis, but only few novel antimicrobial agents are in development for the treatment of MDR Gram-negative pathogens.Citation44 Efforts to preserve the antimicrobials are crucial to future success in treating Gram-negative infections.
Suitable diagnosis, liable antimicrobial use, and familiarity with local resistance patterns can help the clinician to treat patients. In the last few years, resistance to antimicrobial agents in the treatment of Gram-negative microorganisms has generally increased.
P. aeruginosa, Acinetobacter baumannii, and K. pneumoniae are among the most common bacteria, referred to as MDR bacteria. Excessive use of antimicrobials, careless infection control, and plasmid-mediated resistance gene transfer are playing important roles in this problem.Citation43
Resistance to β-lactam antibiotics
Bacterial resistance to β-lactam antibiotics and β-lactamase inhibitors is an important problem that decreases the clinical efficacy of drugs that are the cornerstone of antibiotics throughout the world. Gram-negative pathogens frequently synthesize new β-lactamases in order to respond to the efficacy of these kinds of antibiotics. More than 340 β-lactamase enzymes have been identified to date.Citation45 These drug inhibitory agents include class A SHV- and TEM-derived extended-spectrum β-lactamases (ESBLs). In addition to inhibitor-resistant enzymes, non-TEM, non-SHV class A ESBLs, and carbapenemases, class B metallo-β-lactamases and some of their new inhibitors, plasmid and chromosomally encoded class C enzymes, the OXA-type oxacillinases, ESBLs, and carbapenemases of class D are the other types of suppressing agents against β-lactam antibiotics.Citation46
The most common plasmid-mediated β-lactamase is TEM-1, which has been reported in approximately 75%–80% of plasmid-mediated β-lactamase resistance cases.Citation47–Citation50 The TEM enzyme was originally isolated from a single strain of E. coli. It was also isolated from blood culture in 1965.Citation51
The TEM-1 β-lactamase spread into the Enterobacteriaceae family within 10 years. A close relative of TEM-1, TEM-2, was recorded in P. aeruginosa in 1969. Furthermore, plasmids encoding the TEM-1 enzyme had spread in H. influenzae and N. gonorrhoeae in 1970.Citation52 Another common plasmid mediated β-lactamase was found in K. pneumoniae and E. coli and named as SHV-1 (for sulfhydryl variable). The SHV-1 β-lactamase is chromosomally encoded in most of the isolates of K. pneumoniae, and it is usually plasmid-mediated in E. coli.Citation53
One of the β-lactam agents, carbapenems, has an action against MDR Gram-negative bacteria. Imipenem, meropenem, and doripenem show high antibacterial activity against P. aeruginosa, but ertapenem does not show such efficacy.Citation54 In recent years, some Gram-negative bacteria have started to produce β-lactamase enzymes which degrade β-lactam rings and deactivate the efficacy of β-lactam antibiotics. It was recently demonstrated that Acinetobacter, Pseudomonas, and members of the Enterobacteriaceae family exhibited strong resistance to β-lactam.Citation55 B-lactamase production was also commonly seen in N. gonorrhoeae and this activity limited the usefulness of penicillin G for the treatment of N. gonorrhoeae.
Penicillin’s Gram-negative activity increases from penicillin to ampicillin and ticarcillin to piperacillin. Like penicillin, cephalosporins change their Gram-negative activity. First-generation agents like cefazolin act against pathogens such as E. coli and K. pneumoniae. Third-generation agents such as ceftazidime, cefotaxime, and ceftriaxone and fourth-generation agents such as cefepime show greater potency against these pathogen microorganisms.Citation44
Resistance to fluoroquinolones
MDR quinolones in Enterobacteriaceae has been rapidly rising all around the world.Citation56–Citation60
Resistance to fluoroquinolones has been shown to be frequently associated with alterations in gyrA.Citation61,Citation62 The mutations are localized at the 59 end of the gene (nucleotides 199–318 in the E. coli gene sequence), the quinolone resistance-determining region, near Tyr122, which binds transiently cleaved DNA.Citation63,Citation64
Genetic characterization of gyrA mutations associated with fluoroquinolone resistance in E. coli has been well defined by DNA sequence analysis of resistant strains of both clinical isolates and mutants selected in vitro.Citation64–Citation67 These mutations are most commonly found in the Ser83 and Asp87 codons and in the corresponding codon positions of the gyrA genes from several other organisms.Citation61,Citation62,Citation68,Citation69
The first plasmid-mediated quinolone-resistant protein Qnr was identified from urine in a K. pneumoniae isolate in 1994. It began a novel era in resistance to quinolones. Quinolones insert into bacteria through porins or directly through the lipid cytoplasmic membrane and target DNA topoisomerases.Citation60 Resistance to quinolones in Enterobacteriaceae most commonly occurs slowly as a result of mutation, usually accumulating in the genes encoding primarily DNA gyrase and also topoisomerase IV. Decreased permeability caused by changes in the amount of porins (in particular outer membrane protein F) or increased efflux in regulatory genes of chromosomally-encoded MDR by mutations may cause increments in quinolone resistance.Citation56,Citation70,Citation71
Fluoroquinolones have become well-used agents in ambulatory settings for a wide range of infections such as urinary tract infections, pneumonia and other respiratory infections, and gastrointestinal infections.
Recently, resistance to fluoroquinolones in Gram-negative bacteria has been increasing. Changes in DNA gyrase and topoisomerase IV – enzymes of bacteria that are targeted by fluoroquinolone – leads to mutational resistance. Furthermore, the presence of multidrug efflux pumps is a significant contributor to fluoroquinolone resistance. Multidrug efflux pumps remove the drug before its antimicrobial action can be achieved, seen in P. aeruginosa in particular.
Worldwide, there are several reported rates of fluoroquinolone resistance in both community-acquired and nosocomial pathogens. Many factors, such as patient characteristics, local epidemiological factors, antibiotic policies, inadequate or excessive usage, lower standards of living in developing countries, and lack of information may be responsible for such divergent data.Citation72
Multistep selection of resistant strains resulting from the association of different resistance mechanisms is responsible for the emergence of high-level resistance to fluoroquinolones. Thus, recognition of strains with low-level resistance is important in order to modify standard therapeutic regimens. As can be seen in vitro, selection of a resistant clone is more likely to occur with the use of less effective compounds. All resistant mutants selected with one compound display cross-resistance to the other compounds but at a variable MIC level. High-level resistance is threatening because such bacteria are already resistant to or have reduced susceptibility to new antibiotics. When resistant strains are first selected, they may spread in the community or in the hospital environment if they have epidemic potential (salmonellae and pneumococci) or if favorable conditions exist. Follow-up of fluoroquinolone resistance is compulsory and should be performed everywhere, including individual communities, so that infections can be better managed and the spread of resistant strains can be counteracted.Citation73
Resistance to aminoglycosides
There are three mechanisms of resistance to aminoglycosides:
Reduced uptake or decreased cell permeability. Reduced drug uptake, mostly seen in Pseudomonas spp. and other nonfermenting Gram-negative bacilli, is due to membrane impermeabilization, but the underlying molecular mechanisms are unknown.Citation74 Aerobic Gramnegative bacilli in general show a phenomenon of adaptive resistance.Citation75,Citation76
Altered ribosome binding sites. Mutations of aminogly-coside attachment may interfere with ribosomal binding. Resistance to streptomycin can occur by this mechanism if this agent binds to a single site on the 30S subunit of the ribosome. Resistance to other aminoglycosides by this mechanism is uncommon. Because they bind to multiple sites on both ribosomal subunits, high-level resistance cannot be selected by a single step.Citation77
Enzymatic modifications. This is the most common type. About 50 different enzymes have been identified,Citation78 and enzymatic modification leads to high-level resistance.Citation77 Encoding for aminoglycoside-modifying enzymes are generally found on plasmids and transposons. Because of multiple genes, most enzyme-mediated resistance occurs in Gram-negative bacilli.Citation79
There are three types of aminoglycoside-modifying enzymes:Citation78
N-acetyltransferases, which catalyze acetyl coenzyme A (CoA)-dependent acetylation of an amino group.
O-adenyltransferase, which catalyze adenosine triphosphate-dependent adenylylation of a hydroxyl group.
O-phosphotransferases, which catalyze adenosine triphosphate-dependent phosphorylation of a hydroxyl group.
For the treatment of Gram-negative infections, currently used agents include gentamicin, tobramycin, and amikacin. Their spectrum of activity includes Enterobacteriaceae, Acinetobacter spp., and P. aeruginosa. Intrinsic aminoglycoside resistance happens mainly via drug exclusion from the cell.
Gram-negative organisms and resistance
E. coli
E. coli is the most common Gram-negative pathogen encountered in clinical practice. E. coli, as one of the normal human gastrointestinal flora, is the primary cause of urinary tract infections in the outpatient setting. Infections caused by E. coli include uncomplicated urinary tract infections to hospital-acquired pneumonia and sepsis. Thus, antimicrobial resistance in E. coli is of major importance in both the ambulatory and hospitalized patient.
Recently, resistance to fluoroquinolones among clinical strains of E. coli has been rising. In 2004, according to the National Nosocomial Infections Surveillance project, about 10% of E. coli strains isolated from hospitalized patients were resistant to fluoroquinolones.Citation72,Citation79 Mostly, this resistance is due to either efflux pumps or target-site modification.
K. pneumoniae
K. pneumoniae is in contrast to E. coli, a constant producer of SHV-1. While ampicillin and amoxicillin could be expected to penetrate the cell wall of K. pneumoniae, they have no defense to β-lactamases, preventing therapy with these agents. The addition of a β-lactamase inhibitor should repair the effect of the penicillin agent, provided no ESBLs or other resistance mechanisms are present. The MDR profile of ESBL-producing Gram-negative bacteria reveals that this organism has broad-spectrum resistance from penicillin to aztreonam. Fluoroquinolone resistance in K. pneumoniae is similar to E. coli and other Enterobacteriaceae. Resistance most commonly occurs as a mutation of DNA gyrase and topoisomerase IV.
P. aeruginosa
P. aeruginosa is naturally resistant to many agents, either through β-lactamase production or through the exclusion of antimicrobial agents from the cell.Citation43 Mutational resistance has been increasing in frequency.
A. baumannii
Several studies show that carbapenem overuse affects the MDR profile of A. baumannii.Citation80–Citation82
Resistance of TB
TB is caused by Mycobacterium tuberculosis. While drug-resistant TB organisms are resistant to at least one first-line anti-TB drug, MDR TB is caused by isoniazid (INH) and rifampin (RIF) – the two most potent TB drugs. Both treating and curing drug-resistant TB is complicated. Inappropriate or inadequate management can threaten life.Citation83
Drug resistance is diagnosed by drug-susceptibility testing in the laboratory. However, since these tests can take several weeks, treatment could be started with an empirical treatment regimen based on expert advice, depending on the probability that the person has drug-resistant TB disease. When testing results are known, the treatment regimen should be adjusted according to the results. Patients should be monitored closely throughout treatment. Directly observed therapy should always be used in the treatment of drug-resistant TB to ensure adherence.
The problem of resistance of TB is very old. The first two drugs used in formal clinical trials, streptomycinCitation84,Citation85 and para-aminosalicylic acid,Citation86 were developed in the 1940s. In 1994, it was thought that 2.2 billion people were infected with M. tuberculosisCitation87 and the organism caused 1.6 million deaths. Drug resistance can be natural (primary) or acquired. If natural, the patient is infected with a resistant organism even though the patient has never received therapy. Acquired resistance means that the patient’s initially sensitive organism becomes resistant after using antimicrobials.
In 2005, the overall prevalence of primary resistance to INH was 7.3%, the overall prevalence of acquired resistance to INH was 14.6%, and the overall prevalence of primary MDR TB was 1% in the USA.Citation87 In 2006 in the USA, the prevalence of MDR TB was about 0.02% (17 cases). More than 80% of cases had no previous exposure to TB, and therefore considered to be primary MDR TB.Citation88
Spontaneous mutations in the chromosome can lead to drug resistance in M. tuberculosis. Mutations associated with RIF and fluoroquinolone resistance occur at about 10−8, mutations leading to INH, streptomycin, ethambutol, kanamycin, or para-aminosalicylic acid resistance occur at a frequency of approximately 10−6, and mutations leading to ethionamide, capreomycin, cycloserine, or thiacetazone resistance occur at a frequency of about 10−8.Citation89 These frequencies are important as resistance to a combination of antibiotics (eg, RIF with INH) would be expected to occur at a combined rate.Citation90,Citation91
In general, bacteria are resistant to both drugs. However, the possibility of cure using both drugs is high because INH would kill RIF-resistant bacteria and RIF would kill INH-resistant bacteria. RIF resistance would improve among the INH-resistant population at a frequency of 10−8. At first, the patient might improve while RIF kills the majority of the bacteria, but then strains resistant to both drugs would occur, and the patient would relapse. On the other hand, a clinician’s prescribing behavior may lead to drug resistance.
Treatment of drug-resistant TB
Standard short-course TB therapy is the best way to prevent MDR TB. Standard management is effective in patients with bacilli that are resistant to INH and/or streptomycin. Drug resistance should be suspected in a patient who presents these conditions:
Has had first therapy;
Is in an area where drug resistance is widespread;
Sputum smears are positive after 2 months of therapy;
Sputum smears are positive after 4 months of therapy;
The patient’s chest radiography is getting worse.
If the anti-TB drugs are insufficient, drug resistance can occur. Second-line agents must be employed when INH, RIF, pyrazinamide, or ethambutol cannot be used because of drug resistance.
Prevention of drug-resistant TB
Patients exposed to INH mono-resistant TB can be treated for 2 months with a combination of rifamycin and pyrazinamide.Citation92,Citation93 If the patient cannot tolerate pyrazinamide, a 4–6 month regimen of rifamycin alone is acceptable.
Patients exposed to RIF mono-resistant TB can be treated with an ordinary INH regimen.
The treatment and cure of MDR-TB cases is very difficult because there are no sufficient published data concerning the composition, duration, or effectiveness of treatment regimens for MDR-TB. Standard regimens that have been suggested include pyrazinamide and ethambutol or pyrazinamide and quinolone. These drugs are expected to treat active disease for 12 months in immunocompromised patients and ≥6 months in patients who are immunocompetent.Citation92
Finally, the success of therapy should be measurable with drug-susceptible TB. Patients should heal clinically and cultures should become negative. In addition, smears and cultures should be observed every 2 months for the next 2 years.
The challenges of MDR strains of bacteria and current management
The discovery of antibiotics was one of the major advancements in public health during the 20th Century.Citation94 During the early period of antibiotic usage, bacterial infections were considered defeated. Antibiotics provided prevention of potentially lethal infections.Citation95 However, the emergence of MDR bacteria in the last few decades is resulting in one of the most important clinical health problems in modern medicine.Citation96
Antibiotic resistance occurs by vertical transmission of the product of innate genetics and physiology of bacteria through species.Citation96 Resistance can also be transferred horizontally by plasmids or by chromosomally located conjugative transposons across species and genera.Citation97 This combinatorial genetic strategy has resulted in the accumulation of MDR phenotypes in many species of bacteria.Citation96 However, the emergence of MDR could not be adequately explained on the basis of random, independent mutational events.Citation98 Alteration or modification of the target of antibiotics, degradation of the antibiotic structure, and decreased permeability and energy-dependent (or active) efflux are some of the resistance action of bacteria.Citation95 Efflux-mediated resistance is mostly drug specific. On the other hand, some of the efflux systems accommodate multiple drugs and thus contribute significantly to bacterial intrinsic and acquired MDR.Citation95
MDR organisms, predominantly bacteria, are resistant to one or more classes of antimicrobial agents.Citation99 More strains of pathogens have become antibiotic resistant, and some have become resistant to many antibiotics and chemotherapeutic agents.Citation100 Even if the known MDR organisms were defined as resistant to at least three antibiotics belonging to three different families, these organisms are frequently resistant to most available antimicrobial agents.Citation101 It has been shown by different researchers that some strains of E. coli, K. pneumoniae, and A. baumannii are resistant to all antimicrobial agents, and Stenotrophomonas maltophilia, Burkholderia cepacia, and Ralstonia pickettii are intrinsically resistant to the broadest spectrum antimicrobial agents.Citation102 The increasing antimicrobial resistance of infectious agents is defined as one of the most important health threats worldwide.Citation103
It was estimated that at least 25,000 patients die each year from an infection caused by MDR bacteria in the European Union.Citation104 Antibiotic resistance is also a major public health issue in low and middle income countries.Citation105 The potential for transfer of drug resistance to human bacteria and MDR has become a worldwide concern.Citation98 Therefore, there is an urgent and critical need to develop new antibacterial agents with activity against MDR bacteria.Citation104
Managing MDR requires understanding of the molecular basis, evolution, and dissemination of resistance; development of new antibiotic compounds in place of traditional antibiotics; and innovative strategies for extending the life of antibiotic molecules.Citation96 One of the major problems facing the antibiotic drug discovery sector is the difficulty in identifying new druglike compounds with suitable antibacterial activity.Citation96
Over the last 50 years, medicinal chemists have been highly successful in developing new antibiotics, both natural and synthetic, such as fourth-generation β-lactam and third-generation macrolides. However, significantly new approaches and strategies for new molecules are needed.Citation106
Generally, antibiotics have been founded by testing natural products. Natural product antibiotic effects can be directly isolated from soil and marine microorganisms or antibiotic-producing organisms that can be modified genetically. Another approach is the diversion of natural metabolic pathways of antibiotic-producing organisms by introduction of substrate precursors into the fermentation system.Citation107 However, different approaches should be developed for these products to improve their spectrum, pharmacokinetics, human safety, and ability to cope with resistance.
Recently, an attempt to develop antibiotics with an effect on bacteria, viruses, fungi, and protozoa was based on nanotechnology. Nanoemulsions pass through pores of skin and hair follicles to the infected area, without harming the dermal cells, and disrupt the microorganism and decrease the risk of resistance against antibiotics.Citation108
The molecular basis of tolerance to antimicrobial agents should be examined in detail with gene knockouts, genomics, and proteomics. Combinations of antibacterial agents that prolong the life of these drugs can be used after a detailed performance study.Citation109 Even if research and development of antibacterial agents against infectious agents starts in the near future, the issue of antibiotic resistance is likely to continue to increase.Citation104 Side effects of newly developed antibiotics constitute another serious problem. Therefore, potential approaches should be to increase patient compliance, decrease the potential for resistance development, reduce drug costs, and increase efficacy, ultimately extending the life of these antimicrobial agents.Citation109
Platensimycin and platencin
Bacterial infections are increasingly defying antibiotics that are heavily used in hospitals.Citation110 For this reason, treatment costs and therapy failures are increasing with regard to the fight against infectious diseases. In particular, resistant Grampositive pathogens such as MSRA,Citation111,Citation112 vancomycin-resistant enterococci,Citation113 and penicillin-resistant S. pneumoniae are posing a serious threat.Citation114,Citation115
There are many approaches to developing an effective antibacterial agent that target an essential constituent or pathway in the infection agents. However, some of these attempts have been undesirable due to inefficient pharmacokinetics, drug resistance, and toxicity. Moreover, when drug research costs are considered, the problem is increasingly difficult to solve. On the other hand, initiatives about an encouragement program for governments to develop antimicrobial agents have been published.Citation116 As a result of these initiatives, considerable effort has been put into developing new techniques to improve antibiotics, for example the antisense ribonucleic acid (RNA) method has been developed for use in the discovery and improvement of antibiotics.Citation117–Citation121
In the antisense RNA method, drug targets are determined with the antisense RNA by decreasing expression levels of a certain protein which is thought to be effective in an essential pathway. Comparisons can then be made about the strain with the depleted protein. According to the test results, special drugs can be developed that target the protein of interest.Citation120 To demonstrate accuracy of the method, FabF (a β-ketoacyl-acyl carrier protein [ACP] synthase II) and related other targets were investigated.Citation122
It is expected that decreasing fabF transcript levels due to expression of antisense fabF transcript impairs the target proteins. When control organisms were compared to cells expressing fabF antisense RNA with zone of inhibition measurement, only FabF-inhibited cells indicated significant distinction from others.Citation122 As a result, researchers focused on the inhibition of the FabF pathway. Eventually, in 2006, Wang et al screened platensimycin as an inhibitor of the FabF/H pathway.Citation123–Citation126
Platensimycin was identified as a metabolite of a filamentous bacterium Streptomyces platensis MA7327 strain found in soil samples from Spain and South Africa in 2006.Citation122,Citation124,Citation126–Citation129 Platensimycin is a bacteriostatic drug that does not exhibit cross-resistance to Gram-positive bacteria with MIC values of 0.1–0.32 mg/mL, therefore making it a potentially useful antibiotic candidate.Citation130,Citation131 Platensimycin was efficiently used in a murine model of a common S. aureus infection and no toxicity was observed in these animals. In addition, lack of toxicity in HeLa cells with platensimycin and lack of antifungal activity are clear signs for the promising marked selectivity of platensimycin.Citation134,Citation135 Furthermore, platensimycin shows strong efficacy without any observed toxicity in vivo and it is nontoxic to humans. Its unique target is bacterial fatty acid synthesis (FAS).
Platensimycin blocks FabF and stops bacterial FAS selectively.Citation122,Citation123,Citation128,Citation132 Different procedures (reversephase high-performance liquid chromatography, mass spectrometry, two-dimensional nuclear magnetic resonance, and X-ray crystallographic analysis) were utilized to acquire the structure of platensimycin. Among them, X-ray crystallographic analysis presented absolute stereochemistry of the molecule.Citation124 Its molecular formula is C24H27NO7 and molecular weight is 441.47 g/mol. The structure of platensimycin consists of two distinct structural motifs that are joined by an amide bond.Citation125 Platensimycin is formed by two structures. One of these is an aromatic unit which has a hydrophobic characteristic and the other one is a tetracyclic lipophilic unit.Citation126,Citation131,Citation133 The aromatic and tetracyclic portions are joined by a flexible amide chain. The aromatic unit binds to FabF and penetrates into the catalytic site.Citation134
After discovery and patenting of platensimycin, researchers focused on the synthesis of platensimycinCitation125,Citation135–Citation138 and its analogs.Citation123,Citation139 These studies revealed that platensimycin, which had great potential to penetrate through bacterial cell membranes, demonstrated high antibacterial activity against Gram-positive bacterium including staphylococci and enterococci. Despite the promising efficacy of platensimycin, FASII is not a suitable antibiotic target for Gram-positive pathogens as exogenous fatty acids fully bypass inhibition of the FAS pathway in both in vivo and in vitro conditions.
Platensimycin is not effective against Gram-negative bacteria.Citation123,Citation131 This is due to the extrusion mechanism of Gramnegative bacteria. This mechanism pumps platensimycin out of the cell membrane in Gram-negative bacteria. It demonstrates that platensimycin can inhibit Gram-negative bacteria when this pump mechanism is disabled due to mutations. For example, E. coli is an extrusion-negative mutant bacterium and platensimycin is effective against these bacteria.Citation123,Citation140,Citation141
The Merck team recently described their finding of platencin as an antibiotic with an analogous structure and profile to platensimycin.Citation128,Citation142 Platencin is actually an analog of platensimycin. It also demonstrates antibacterial activity against Gram-positive bacteria (25% of the MIC 0.5–32 mg/mL) through the inhibition of FAS. In vitro studies have shown that it is active against vancomycin-resistant enterococci, MRSA, and both linezolid- and macrolide-resistant pathogens.Citation123,Citation128,Citation131
This antibiotic is an agent for another rising drug applicant due to its in vivo capability and lack of toxic side effects. Platencin is produced by the S. platensis strain MA7339. S. platensis was discovered in a soil sample collected from Spain. The similar whole-cell differential assay was used. The molecular formula of platencin is C24H25NO6 and its molecular weight is 425.47 g/mol, marginally smaller than platensimycin. It is comprised of a tetracyclic unit lacking the ether ring (thus different from the pentacyclic ketolide of platensimycin). The tetracyclic unit is connected to an aminobenzoic acid moiety by an amide bond.Citation142 Platensimycin inhibits the elongation-condensing enzyme FabF. In contrast to platensimycin, platencin is a binary inhibitor of FabF and the condensing enzyme FabH. The fact that platencin fundamentally targets two enzymes in the FAS pathway may assist in reducing the potential for resistance advancement against the drug.
MIC rates of platencin against a group of organisms were nearly the same, but it showed lower activity in the existence of serum. It also demonstrated acceptable antibacterial activity in vivo. The most critical conflict was the enzyme inhibition profile of platencin. It determined a dual activity against FabF and FabH. Capability against FabF (half maximal inhibitory concentration [IC50] = 0.113 μM) was about six times less than platensimycin while inhibition of FabH (IC50 = 16.2 μM) was four times more intense. By themselves these IC50 rates are not remarkable, but in combination these two antibiotic candidates have a synergistic impact on suppressing bacterial cell growth.Citation128,Citation142
Jayasuriya et al used docking assays to demonstrate these potency differences. It is tempting to visualize the design that maximizes inhibition of both these enzymes to exploit the synergistic effect on antibacterial activity.Citation142
Pharmacology of platensimycin and platencin
Platensimycin belongs to a unique structural class of natural antibiotics and has no cross-resistance to other classes of antibiotic-resistant bacteria. MIC values of platensimycin are 0.5 μg/mL against S. aureus (MRSA) and S. aureus (MRSA, macrolide), 1 μg/mL against S. aureus (MRSA, linezolid), 0.5 μg/mL against S. aureus (vancomycin-intermediate S. aureus, vancomycin), 0.1 μg/mL against Enterococcus faecium (vancomycin-resistant enterococci), and >64 μg/mL against E. coli (wild-type).Citation123 Molecular weight of platensimycin is 441.47 g/mol (). Platencin (molecular weight 425.2 g/mol) is another novel natural product that has similar structure and profile to platensimycin (). MIC values of platencin against vancomycin-resistance E. faecium and efflux-negative E. coli are <0.06 μg/mL and 2 μg/mL, respectively. Platencin does not exhibit cross-resistance to MRSA.
Figure 1 (A) two-dimensional and (B) three-dimensional molecular structure of platensimycin (molecular weight 441.47 g/mol).
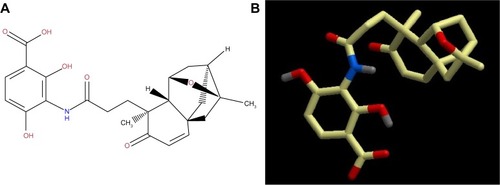
Figure 2 (A) two-dimensional and (B) three-dimensional molecular structure of platencin (molecular weight 425.2 g/mol).
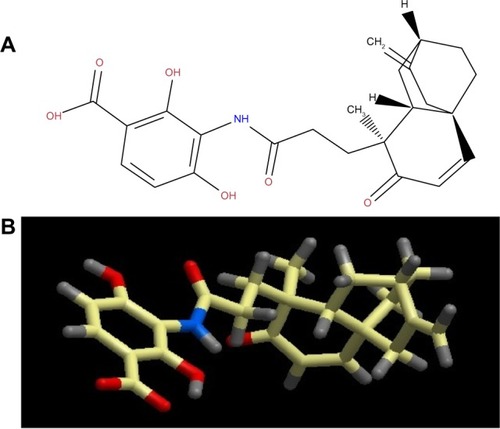
Both platensimycin and platencin have different ways of killing Gram-positive pathogens, including MDR strains of staphylococci and enterococci. It is known that most antibiotics target one of four essential bacterial processes including cell wall biosynthesis, nucleic acid biosynthesis, protein synthesis, and folic acid biosynthesis.Citation143 Platensimycin shows antibacterial activity by targeting FASII. FASII is present in prokaryotes, plants, fungi, and parasites, as well as in mitochondria. In animals, as well as yeast and some fungi, these same reactions occur in FASI. FASI is less efficient than FASII.Citation144 The ACP is a key component in type II systems and carries the fatty acid intermediates from enzyme to enzyme through the cytosol. Usually, C16–C18 fatty acids are the principal end products of the pathway; in case of bacteria, the pathway is more complicated and consists of FASI and FASII. The first enzyme of the fatty acid biosynthesis is the acetyl-CoA carboxylase (AccABCD) that transfers acetyl-CoA to malonyl-CoA, which subsequently interacts with ACP by malonyl-CoA–ACP transacylase (FabD) to form malonyl-ACP. The original fatty acid cycle is initiated by the condensation of malonyl-ACP with acetyl-CoA by the β-ketoacyl-ACP synthase III (FabH) to form β-ketobutyryl-ACP and carbon dioxide. The following steps are repeated until the desired chain length of the fatty acid is achieved: the first is the nicotinamide adenine dinucleotide phosphate-dependent reduction of the keto group to the hydroxyl group to give a β-hydroxyacyl-ACP intermediate by the p-ketoacyl-ACP reductase (FabG). In the next step, water is removed by either a FabA or FabZ isoform of the β-hydroxyacyl-ACP dehydratase to give a trans-2-enoyl-ACP. The third step involves the reduction of the produced double bond by the nicotinamide adenine dinucleotide-dependent trans-2-enoyl-ACP reductase I (FabI) to form an acyl-ACP. The formed acyl-ACP is then condensed with a new malonyl-ACP by either β-ketoacyl-ACP synthase I or II (FabB and FabF) to start a subsequent cycle.Citation145 So far, the only enzyme of this pathway successfully inhibited by antibiotics (INH and triclosan) in clinical use is FabI. Further inhibitors of enzymes in the fatty acid biosynthesis have been elucidated, among them two natural inhibitors of the condensing enzymes, thiolactomycin (FabH, FabB/F) and cerulenin.Citation146,Citation147 According to studies, new antibiotics must be developed for the inhibition of FASII. Fatty acids have to be synthesized for bacterial viability. If fatty acids are not synthesized in bacteria, bacteria will not build a cell wall. Platensimycin prohibits the pathway of FASII by specifically inhibiting β-ketoacyl-ACP synthase II (FabF).Citation124 Platencin is less active than platensimycin against FabF. However, it is more active than platensimycin against FabH.Citation130
Pharmacokinetics
Application of pharmacokinetic principles allows the relationship between dose and antibiotic concentration to be described. This is one important component of the relationship between dosage regimen and time course of effect. The existence of the second component is the concentration–effect relationship (pharmacodynamics). Therefore, by predicting the time course of antibiotic concentrations, one important step towards predicting the time course of antibiotic effect has been made. If the same dosage regimen of an antibiotic is given to different patients, there is true between-subject variability in the observed antibiotic concentrations. A reason for high between-subject variability may be that patients differ in their ability to absorb or eliminate an antibiotic. By including the variability of the pharmacokinetic parameters into a pharmacokinetic model, one can predict drug effects more precisely and optimize dosage regimens for the patient population.Citation148 Platensimycin and platencin are promising antibiotics against important infection agents. However, their pharmacokinetic and safety profiles in humans are still not fully understood. In a mouse study, test animals were infected with S. aureus by intravenous injection with continuous infusion of platensimycin. As a result, the infection rate decreased approximately 40% in the kidney. Moreover, no toxic effect was detected when different concentrations of platensimycin were applied to the test animals. Platensimycin shows low toxicity in vitro in HeLa cells (IC50 >1000 μg/mL).Citation123 According to all reported studies, platensimycin and platencin are promising antibiotics. Therefore, studies should be conducted on pharmacokinetics.
Analogs/derivatives of platensimycin and platencin
Many chemists, biochemists, and pharmacologists have tried to synthesize analogs/derivatives of platensimycin and platencin because of their strong antibacterial properties. One study has demonstrated that 7-phenylplatensimycin and 11-methyl-7-phenylplatensimycin are more potent analogs of platensimycin by carbonyl ylide cycloaddition strategy, and that they showed higher activity than platensimycin against MRSA and vancomycin-insensitive S. aureus.Citation149 Barykina et al have synthesized nor-platencin, which is four to 16 times less active than platencin against several resistant strains of S. aureus, macrolide-resistant E. faecalis, and vancomycin-resistant E. faecium.Citation150 In another study, Patra et al synthesized and characterized four new ferrocene-containing bioorganometallics inspired by the antibiotic platensimycin lead structure. 3-(4,4-diferrocenoylpentanamido)-2,4-dihydroxybenzoic acid; 3-(4,4-diferrocenoylbutanamido)-2,4-dihydroxybenzoic acid; 3-(4-[acetylferrocene] butanamide)-2,4-dihydroxybenzoic acid; and 3-(4-ferrocenoylbutanamido)-2,4-dihydroxybenzoic acid were found to be inactive against Gram-negative E. coli and P. aeruginosa. However, among all the new compounds tested, 3-(4-[acetylferrocenoyl]butanamido)-2,4-dihydroxybenzoic acid, bearing a ferrocenyl 1,3-diketone functionality, inhibits S. aureus growth selectively at an MIC value of 128 μg/mL.Citation151 Wang et al have synthesized and tested biological activity of oxazinidinyl platensimycin, the methyl ester analog, and the simplified oxazinidinyl analog. Although its structure mimics that of platensimycin, oxazinidinyl platensimycin is two orders of magnitude less potent.Citation152 Another study by Wang et al synthesized dialkylamino-2,4-dihydroxybenzoic acids as analogs of platensimycin and platencin.Citation153
Patient-focused perspectives related to the use of platensimycin and platencin
Today, the bacterial resistance against existing antibiotics is well known and it has been a major issue for the last few decades.Citation154 There are many chemicals that are lethal for bacteria, but they have the same effect on the host cells and hence cannot be used to cure bacterial infection. Thus, an attack on bacterial metabolic pathways selectively in the presence of a host is an important step in solving the problem. After a search for new antibiotics for fighting against MDR strains, two novel antibiotics, platensimycin and platencin, have been discovered. The advantage of these antibiotics is that they inhibit the bacterial fatty acid biosynthesis, which is essential for the survival of bacteria. Their structures are related with slightly different mechanisms of action, and they target different enzymes in the bacterial fatty acid biosynthesis.Citation123,Citation155
Platensimycin has strong, broad-spectrum Gram-positive antibacterial activity and is selective for inhibiting cellular lipid biosynthesis. Wang et al showed that the antibacterial effect is via the selective targeting of FabF and FabB in the synthetic pathway of fatty acids.Citation123 The inhibitor with the most potential for the FabF/B enzymes is platensimycin, which shows in vivo efficacy with no observed toxicity.Citation123 Both platensimycin and platencin show in vivo activity, but the usefulness of this target in Gram-positive organisms is doubtful due to the ability of exogenous unsaturated fatty acids to remediate FASII inhibition.Citation156 It has been shown that platensimycin is active against M. tuberculosis through the inhibition of mycolic acid synthesis, and that it inhibits both KasA and KasB enzymes.Citation157 It also provides a new lead for anti-TB drug design.Citation156 Modifying either the aromatic portion or the ketolide domain of platensimycin and platencin has produced many analogs recently.Citation158–Citation164 However, none of them have better activity than platensimycin and platencin.Citation155 Thus, to improve their poor pharmacokinetic properties, several congeners of these products have been discovered from the fermentation of broth but, again, none of them have exhibited better activity.Citation165–Citation168 For a major breakthrough in antibiotic research, platensimycin and platencin may still provide many derivative compounds, which are waiting to be discovered.Citation155
The preclinical trials of these new antibiotics are targeted to combat MRSA in a mouse model.Citation123 It has been reported that platensimycin is also a potent and highly selective inhibitor for mammalian FAS for the treatment of diabetes and related metabolic disorders in animal models. In particular, platensimycin inhibits FAS, but not the sterol synthesis in rat primary hepatocytes. It also preferentially concentrates in liver when administered orally to mice and potently inhibits hepatic de novo lipogenesis, increases glucose oxidation, and reduces fatty acid oxidation. In chronic applications, the platensimycin led to a reduction in liver triglyceride levels and improved insulin sensitivity in db/+ mice fed a high-fructose diet. The ambient glucose levels in db/db mice were also reduced with use of platensimycin.Citation169
Platensimycin is not ready for clinical use. The in vivo activity has been established in a mouse model, but to maintain efficacy, continuous delivery is necessary. The pharmacokinetic profile for platensimycin is poor, and needs improvement before clinical use.Citation170
Disclosure
The authors report no conflicts of interest in this work.
References
- World Health OrganizationThe Global Burden of disease: 2004 UpdateGenevaWorld Health Organization2008 Available from: http://www.who.int/healthinfo/global_burden_disease/GBD_report_2004update_full.pdf
- World Health OrganizationGlobal Health Risks: mortality and burden of disease attributable to selected major risksGenevaWorld Health Organization2009 Available from: http://www.who.int/healthinfo/global_burden_disease/GlobalHealthRisks_report_full.pdf
- ZinnerSHAntibiotic use: present and futureNew Microbiol200730332132517802919
- CunhaBAAntibiotic resistanceMed Clin North Am20008461407142911155850
- MacleanRCHallARPerronGGBucklingAThe evolution of antibiotic resistance: insight into the roles of molecular mechanisms of resistance and treatment contextDiscov Med2010105111211820807471
- LevySBMarshallBAntibacterial resistance worldwide: causes, challenges and responsesNat Med200410Suppl 12S122S12915577930
- BushKAntibacterial drug discovery in the 21st centuryClin Microbiol Infect200410Suppl 4101715522035
- World Health Organization2011/2012 Tuberculosis Global FactsGenevaWorld Health Organization2011 Available from: http://www.who.int/tb/publications/2011/factsheet_tb_2011.pdfAccessed April 16, 2013
- StyersDSheehanDJHoganPSahmDFLaboratory-based surveillance of current antimicrobial resistance patterns and trends among Staphylococcus aureus: 2005 status in the United StatesAnn Clin Microbiol Antimicrob20065216469106
- BorgMAde KrakerMSciclunaEPrevalence of methicillin-resistant Staphylococcus aureus (MRSA) in invasive isolates from southern and eastern Mediterranean countriesJ Antimicrob Chemother20076061310131517913724
- SibandaTOkohAIThe challenges of overcoming antibiotic resistance: plant extracts as potential sources of antimicrobial and resistance modifying agentsAfr J Biotechnol200762528862896
- GarciaLSMalariaClin Lab Med20103019312920513543
- WrightGDQ&A: antibiotic resistance: where does it come from and what can we do about it?BMC Biol2010812320887638
- RahalJJNovel antibiotic combinations against infections with almost completely resistant Pseudomonas aeruginosa and Acinetobacter speciesClin Infect Dis200643Suppl 2S95S9916894522
- StefanovicODStanojevicDDComicLRSynergistic antibacterial activity of Salvia officinalis and Cichorium intybus extracts and antibioticsActa Pol Pharm201269345746322594260
- KangHKKimHYChaJDSynergistic effects between silibinin and antibiotics on methicillin-resistant Staphylococcus aureus isolated from clinical specimensBiotechnol J20116111397140821491604
- TanYTTillettDJMcKayIAMolecular strategies for overcoming antibiotic resistance in bacteriaMol Med Today200068309314 Swedish10904248
- DeydeVMXuXBrightRASurveillance of resistance to adamantanes among influenza A(H3N2) and A(H1N1) viruses isolated worldwideJ Infect Dis20071962249257 French17570112
- Centers of Disease Control and PreventionActive Bacterial Core Surveillance (ABCs) Report: Emerging Infections Program Network, Streptococcus pneumoniae, 2007Atlanta, GACenters for Disease Control and Prevention2008 Available from: http://www.cdc.gov/abcs/reports-findings/survreports/spneu07.pdfAccessed April 16, 2013
- LaurichesseHGrimaudOWaightPJohnsonAPGeorgeRCMillerEPneumococcal bacteraemia and meningitis in England and Wales, 1993 to 1995Commun Dis Public Health19981122279718833
- Council of State and Territorial EpidemiologistsNational Surveillance for Drug-Resistant Streptococcus Pneumoniae (DRSP) Invasive Disease. CSTE Position Statement 1994–10Atlanta, GACouncil of State and Territorial Epidemiologists1994
- Centers for Disease Control and Prevention2007Extensively drug resistant tuberculosis – United States, 1993–2006Morb Mortal Wkly Rep56250253
- World Health OrganizationWorld malaria situation in 1994. Part I. Population at riskWkly Epidemiol Rec199772362692749293226
- GuinovartCNaviaMMTannerMAlonsoPLMalaria: burden of diseaseCurr Mol Med20066213714016515506
- ArguinPMKozarskyPEReedCCenters for Disease Control and PreventionCDC Health Information for International Travel 2008St Louis, MOMosby2007
- BjorkmanAPhillips-HowardPAThe epidemiology of drug-resistant malariaTrans R Soc Trop Med Hyg19908421771802202097
- KrogstadDJMalaria as a reemerging diseaseEpidemiol Rev199618177898877332
- PetoHMPrattRHHarringtonTALoBuePAArmstrongLREpidemiology of extrapulmonary tuberculosis in the United States, 1993–2006Clin Infect Dis200949913501357 Swedish19793000
- WangFDChenYYChenTLLiuCYRisk factors and mortality in patients with nosocomial Staphylococcus aureus bacteremiaAm J Infect Control200836211812218313513
- FeldmanCKlugmanKPneumococcal infectionsCurr Opin Infect Dis1997102109115
- DowsonCGCoffeyTJSprattBGOrigin and molecular epidemiology of penicillin-binding-protein-mediated resistance to β-lactam antibioticsTrends Microbiol19942103613667850202
- IpMLyonDJYungRWTsangLChengAFIntroduction of new clones of penicillin-nonsusceptible Streptococcus pneumoniae in Hong KongJ Clin Microbiol20024041522152511923387
- DoernGVPfallerMAKuglerKFreemanJJonesRNPrevalence of antimicrobial resistance among respiratory tract isolates of Streptococcus pneumoniae in North America: 1997 results from the SENTRY antimicrobial surveillance programClin Infect Dis19982747647709798031
- HiramatsuKAritakaNHanakiHDissemination in Japanese hospitals of strains of Staphylococcus aureus heterogeneously resistant to vancomycinLancet1997350909216701673 Finnish9400512
- EliopoulosGMAntibiotic resistance in Enterococcus species: an updateCurr Clin Top Infect Dis19961621518714247
- StorchGAKrogstadDJAntibiotic-induced lysis of enterococciJ Clin Invest19816836396456792220
- KrogstadDJKorfhagenTRMoelleringRCJrWennerstenCSwartzMNAminoglycoside-inactivating enzymes in clinical isolates of Streptococcus faecalis. An explanation for resistance to antibiotic synergismJ Clin Invest197862248048697312
- MoelleringRCJrKorzeniowskiOMSandeMAWennerstenCBSpecies-specific resistance to antimicrobial synergism in Streptococcus faecium and Streptococcus faecalisJ Infect Dis19791402203208 Swedish113469
- Centers for Disease Control and PreventionNosocomial enterococci resistant to vancomycin – United States, 1989–1993MMWR Morb Mortal Wkly Rep199342305975998336690
- KlevensRMMorrisonMANadleJInvasive methicillin-resistant Staphylococcus aureus infections in the United StatesJAMA20072981517631771 French17940231
- HartmanBJTomaszALow-affinity penicillin-binding protein associated with β-lactam resistance in Staphylococcus aureusJ Bacteriol198415825135166563036
- McDougalLKThornsberryCThe role of β-lactamase in staphylococcal resistance to penicillinase-resistant penicillins and cephalosporinsJ Clin Microbiol19862358328393011847
- LivermoreDMMultiple mechanisms of antimicrobial resistance in Pseudomonas aeruginosa: our worst nightmare?Clin Infect Dis200234563464011823954
- AndesDCraigWACephalosporinsMandellGLBennettJEDolinRPrinciples and Practice of Infectious DiseasesPhiladelphia, PAElsevier2005294311 French
- ShahAAHasanFAhmedSHameedACharacteristics, epidemiology and clinical importance of emerging strains of Gram-negative bacilli producing extended-spectrum β-lactamasesRes Microbiol2004155640942115249058
- HelfandMSBonomoRAB-lactamases: a survey of protein diversityCurr Drug Targets Infect Disord20033192312570729
- MatthewMPlasmid-mediated β-lactamases of Gram-negative bacteria: properties and distributionJ Antimicrob Chemother197954349358385575
- PerilliMSegatoreBde MassisMRTEM-72, a new extended-spectrum β-lactamase detected in Proteus mirabilis and Morganella morganii in ItalyAntimicrob Agents Chemother20004492537253910952610
- RosenauACattierBGoussetNHarriauPPhilipponAQuentinRCapnocytophaga ochracea: characterization of a plasmid-encoded extended-spectrum TEM-17 β-lactamase in the phylum Flavobacter-bacteroidesAntimicrob Agents Chemother200044376076210681352
- RoyCFozASeguraCTiradoMFosterCReigRPlasmid-determined β-lactamases identified in a group of 204 ampicillin-resistant EnterobacteriaceaeJ Antimicrob Chemother19831255075106608515
- DattaNKontomichalouPPenicillinase synthesis controlled by infectious R factors in EnterobacteriaceaeNature196520850072392415326330
- SirotDSirotJLabiaRTransferable resistance to third-generation cephalosporins in clinical isolates of Klebsiella pneumoniae: identification of CTX-1, a novel β-lactamaseJ Antimicrob Chemother1987203323334 Finnish3316146
- De ChampsCSauvantMPChanalCProspective survey of colonization and infection caused by expanded-spectrum-β-lactamase-producing members of the family Enterobacteriaceae in an intensive care unitJ Clin Microbiol1989271228872890 French2592552
- ChambersHFOther β-lactam antibioticsMandellGLBennettJEDolinRPrinciples and Practice of Infectious DiseasesPhiladelphia, PAElsevier2005311318
- LivermoreDMWoodfordNThe β-lactamase threat in Enterobacteriaceae, Pseudomonas and AcinetobacterTrends Microbiol20064941342016876996
- RuizJMechanisms of resistance to quinolones: target alterations, decreased accumulation and DNA gyrase protectionJ Antimicrob Chemother20035151109111712697644
- BurmanLGApparent absence of transferable resistance to nalidixic acid in pathogenic Gram-negative bacteriaJ Antimicrob Chemother197735509516332684
- MunshiMHSackDAHaiderKAhmedZURahamanMMMorshedMGPlasmid-mediated resistance to nalidixic acid in Shigella dysenteriae type 1Lancet1987285564194212887725
- Martinez-MartinezLPascualAJacobyGAQuinolone resistance from a transferable plasmidLancet199835191057977999519952
- DrlicaKZhaoXDNA gyrase, topoisomerase IV, and the 4-quinolonesMicrobiol Mol Biol Rev19976133773929293187
- DeguchiTYasudaMNakanoMQuinolone-resistant Neisseria gonorrhoeae: correlation of alterations in the gyrA subunit of DNA gyrase and the parC subunit of topoisomerase IV with antimicrobial susceptibility profilesAntimicrob Agents Chemother1996404102010238849219
- GeorgiouMMunozRRomanFCiprofloxacin-resistant Haemophilus influenzae strains possess mutations in analogous positions of gyrA and parCAntimicrob Agents Chemother199640717411744 French8807076
- HorowitzDSWangJCMapping the active site tyrosine of Escherichia coli DNA gyraseJ Biol Chem198726211533953443031051
- Moniot-VilleNGuibertJMoreauNAcarJFCollatzEGutmannLMechanisms of quinolone resistance in a clinical isolate of Escherichia coli highly resistant to fluoroquinolones but susceptible to nalidixic acidAntimicrob Agents Chemother19913535195232039202
- ConradSOethingerMKaifelKKlotzGMarreRKernWVGyrA mutations in high-level fluoroquinolone-resistant clinical isolates of Escherichia coliJ Antimicrob Chemother19963834434558889719
- HeisigPTschornyRCharacterization of fluoroquinolone-resistant mutants of Escherichia coli selected in vitroAntimicrob Agents Chemother1994386128412918092826
- VilaJRuizJMarcoFAssociation between double mutation in gyrA gene of ciprofloxacin-resistant clinical isolates of Escherichia coli and MICsAntimicrob Agents Chemother1994381024772479 Portuguese7840592
- TankovicJPerichonBDuvalJCourvalinPContribution of mutations in gyrA and parC genes to fluoroquinolone resistance of mutants of Streptococcus pneumoniae obtained in vivo and in vitroAntimicrob Agents Chemother19964011250525108913454
- WangYHuangWMTaylorDECloning and nucleotide sequence of the Campylobacter jejuni gyrA gene and characterization of quinolone resistance mutationsAntimicrob Agents Chemother19933734574638384814
- HooperDCMechanisms of action and resistance of older and newer fluoroquinolonesClin Infect Dis200031Suppl 2S24S2810984324
- HawkeyPMMechanisms of quinolone action and microbial responseJ Antimicrob Chemother200351Suppl 1293512702701
- HooperDCMechanisms of fluoroquinolone resistanceDrug Resist Updat199921385511504468
- AcarJFGoldsteinFWTrends in bacterial resistance to fluoroquinolonesClin Infect Dis199724Suppl 1S67S738994781
- ChambersHFSandeMAAntimicrobial agents: the aminoglycosidesHardmanJGLimbirdLEThe Pharmacological Basis of TherapeuticsNew York, NYMcGraw-Hill199511031121
- KarlowskyJAHobanDJZelenitskySAZhanelGGAltered denA and anr gene expression in aminoglycoside adaptive resistance in Pseudomonas aeruginosaJ Antimicrob Chemother19974033713769338489
- XiongYQCaillonJKerguerisMFAdaptive resistance of Pseudomonas aeruginosa induced by aminoglycosides and killing kinetics in a rabbit endocarditis modelAntimicrob Agents Chemother1997414823826 French9087497
- KucersACroweSGraysonMLHoyJThe Use of Antibiotics: A Clinical Review of Antibacterial, Antifungal, and Antiviral DrugsOxfordButterworth-Heinemann1997
- DaviesJWrightGDBacterial resistance to aminoglycoside antibioticsTrends Microbiol199752342409211644
- GilbertDAminoglycosidesMandellGLBennettJEDolinRPrinciples and Practice of Infectious DiseasesPhiladelphia, PAElsevier2000307355
- National Nosocomial Infections Surveillance SystemNational Nosocomial Infections Surveillance (NNIS) System Report, data summary from Jan 1992 through Jun 2004, issued Oct 2004Am J Infect Control2004328470185 French15573054
- RahalJJUrbanCSegal-MaurerSNosocomial antibiotic resistance in multiple Gram-negative species: experience at one hospital with squeezing the resistance balloon at multiple sitesClin Infect Dis200234449950311797177
- ManikalVMLandmanDSaurinaGOydnaELalHQualeJEndemic carbapenem-resistant Acinetobacter species in Brooklyn, New York: citywide prevalence, interinstitutional spread, and relation to antibiotic usageClin Infect Dis200031110110610913404
- BlumbergHMBurmanWJChaissonREAmerican Thoracic Society/Centers for Disease Control and Prevention/Infectious Diseases Society of America: treatment of tuberculosisAm J Respir Crit Care Med20031674603662 French12588714
- World Health OrganizationAnti-Tuberculosis Drug Resistance in the WorldGenevaWorld Health Organization2008 Available from: http://whqlibdoc.who.int/hq/2008/WHO_HTM_TB_2008.394_eng.pdfAccessed April 16, 2013
- Centers for Disease Control and PreventionEmergence of Mycobacterium tuberculosis with extensive resistance to second-line drugs – worldwide, 2000–2004MMWR Morb Mortal Wkly Rep2006551130130516557213
- ShimaoTDrug resistance in tuberculosis controlTubercle198768Suppl 25183120383
- World Health OrganizationTB: A Global Emergency. WHO Report on the TB EpidemicGenevaWorld Health Organization1994 Available from: http://whqlibdoc.who.int/hq/1994/WHO_TB_94.177.pdfAccessed April 16, 2013
- SchatzABugleEWaksmanSAStreptomycin, a substance exhibiting antibiotic activity against Gram-positive and Gram-negative bacteriaExp Biol Med19445516669 Italian
- LehmannJPara-aminosalicylic acid in the treatment of tuberculosisLancet1946163841521008766
- BlochABSimonePMMcCrayECastroKGPreventing multidrug-resistant tuberculosisJAMA19962756487489 Italian8627974
- FriedenTRSterlingTRMunsiffSSWattCJDyeCTuberculosisLancet2003362938788789913678977
- Centers for Disease Control and PreventionPrevention and treatment of tuberculosis among patients infected with human immunodeficiency virus: principles of therapy and revised recommendationsMMWR Recomm Rep199847RR–20158
- Targeted tuberculin testing and treatment of latent tuberculosis infection. This official statement of the American Thoracic Society was adopted by the ATS Board of Directors, Jul 1999. This is a Joint Statement of the American Thoracic Society (ATS) and the Centers for Disease Control and Prevention (CDC). This statement was endorsed by the Council of the Infectious Diseases Society of America (IDSA), Sep 1999, and the sections of this statementAm J Respir Crit Care Med20001614 Pt 2S221S24710764341
- BowlerPGWelsbySTowersVMultidrug-resistant organisms, wounds and topical antimicrobial protectionInt Wound J20129438739622640181
- KumarASchweizerHPBacterial resistance to antibiotics: active efflux and reduced uptakeAdv Drug Deliv Rev200557101486151315939505
- WrightGDSutherlandADNew strategies for combating multidrug-resistant bacteriaTrends Mol Med200713626026717493872
- NeuHCThe crisis in antibiotic resistanceScience19922575073106410731509257
- WaxRGLewisKSalyersAATaberHBacterial Resistance to AntimicrobialsBoca Raton, FLCRC Press2008
- BoichevaMLazarovaGRachkovaKRukanovaDTenevaMDzenevaHBacterial pathogens with multidrug resistance. Etiological structure of infections in ICU, Umbal-Ead Stara ZagoraTrakia Journal of Sciences20108Suppl 2165170
- NikaidoHMultidrug resistance in bacteriaAnnu Rev Biochem200978119146 Italian19231985
- SiegelJDRhinehartEJacksonMChiarelloLManagement of multidrug-resistant organisms in health care settings, 2006Am J Infect Control20073510 Suppl 2S165S19318068814
- AlekshunMNLevySBMolecular mechanisms of antibacterial multidrug resistanceCell200712861037105017382878
- Amato-GauciAAmmonAThe First European Communicable Disease Epidemiological ReportStockholmEuropean Centre for Disease Prevention and Control2007 Available from: http://www.ecdc.europa.eu/en/publications/Publications/0706_SUR_First_%20Annual_Epidemiological_Report_2007.pdfAccessed April 16, 2013
- Freire-MoranLAronssonBManzCCritical shortage of new antibiotics in development against multidrug-resistant bacteria – time to react is nowDrug Resist Updat201114211812421435939
- ZaidiAKHuskinsWCThaverDBhuttaZAAbbasZGoldmannDAHospital-acquired neonatal infections in developing countriesLancet2005365946511751188 German15794973
- Committee on New Directions in the Study of Antimicrobial TherapeuticsTreating Infectious Diseases in a Microbial World: Report of Two Workshops on Novel Antimicrobial TherapeuticsWashington, DCNational Academies Press2006
- GootzTDDiscovery and development of new antimicrobial agentsClin Microbiol Rev19903113312404566
- AkyarISearching for Antibiotics Against Super Bug BacteriaAcibadem University Health Sciences Journal2010126267
- CoatesAHuYBaxRPageCThe future challenges facing the development of new antimicrobial drugsNat Rev Drug Discov200211189591012415249
- PearsonH“Superbug” hurdles key drug barrierNature2002418689746912152043
- BarrettJFMRSA: status and prospects for therapy? An evaluation of key papers on the topic of MRSA and antibiotic resistanceExpert Opin Ther Targets20048651551915584858
- SalgadoCDFarrBMCalfeeDPCommunity-acquired methicillin-resistant Staphylococcus aureus: a meta-analysis of prevalence and risk factorsClin Infect Dis2003362131139 Portuguese12522744
- DeshpandeLMFritscheTRMoetGJBiedenbachDJJonesRNAntimicrobial resistance and molecular epidemiology of vancomycin-resistant enterococci from North America and Europe: a report from the SENTRY antimicrobial surveillance programDiagn Microbiol Infect Dis2007658216317017368801
- GordonKABiedenbachDJJonesRNComparison of Streptococcus pneumoniae and Haemophilus influenzae susceptibilities from community-acquired respiratory tract infections and hospitalized patients with pneumonia: five-year results for the SENTRY Antimicrobial Surveillance ProgramDiagn Microbiol Infect Dis200346428528912944021
- WhitneyCGFarleyMMHadlerJIncreasing prevalence of multidrug-resistant Streptococcus pneumoniae in the United StatesN Engl J Med2000343261917192411136262
- TalbotGHBradleyJEdwardsJEJrGilbertDScheldMBartlettJGBad bugs need drugs: an update on the development pipeline from the Antimicrobial Availability Task Force of the Infectious Diseases Society of AmericaClin Infect Dis200642565766816447111
- JiYZhangBVanSFIdentification of critical staphylococcal genes using conditional phenotypes generated by antisense RNAScience2001293553822662269 Dutch11567142
- HutchisonCAPetersonSNGillSRGlobal transposon mutagenesis and a minimal Mycoplasma genomeScience199928654472165216910591650
- AkerleyBJRubinEJNovickVLAmayaKJudsonNMekalanosJJA genome-scale analysis for identification of genes required for growth or survival of Haemophilus influenzaeProc Natl Acad Sci U S A200299296697111805338
- ForsythRAHaselbeckRJOhlsenKLA genome-wide strategy for the identification of essential genes in Staphylococcus aureusMol Microbiol20024361387140011952893
- KobayashiKEhrlichSAlbertiniAEssential Bacillus subtilis genesProc Natl Acad Sci U S A2003100846784683 Italian12682299
- YoungKJayasuriyaHOndeykaJGDiscovery of FabH/FabF inhibitors from natural productsAntimicrob Agents Chemother200650251952616436705
- WangJSoissonSMYoungKPlatensimycin is a selective FabF inhibitor with potent antibiotic propertiesNature2006441709135836116710421
- SinghSBJayasuriyaHOndeykaJGIsolation, structure, and absolute stereochemistry of platensimycin, a broad spectrum antibiotic discovered using an antisense differential sensitivity strategyJ Am Chem Soc200612836119161192016953632
- SinghSBHerathKBWangJTsouNBallRGChemistry of platensimycinTetrahedron Lett20074854295433
- HerathKBAttygalleABSinghSBBiosynthetic studies of platensimycinJ Am Chem Soc200712950154221542318034483
- ArnaudCAntibiotic halts lipid synthesisChem Eng News2006847
- WangJKodaliSLeeSHDiscovery of platencin, a dual FabF and FabH inhibitor with in vivo antibiotic propertiesProc Natl Acad Sci2007104187612761617456595
- BartonSNew antibiotic on the horizon?Nat Rev Drug Discov20065539539
- SinghSBYoungKNew antibiotic structures from fermentationsExpert Opin Ther Pat2010201359137120653459
- HabichDvon NussbaumFPlatensimycin, a new antibiotic and “superbug challenger” from natureChem Med Chem20061995195416952137
- NicolaouKCStepanAFListerTDesign, synthesis, and biological evaluation of platensimycin analogues with varying degrees of molecular complexityJ Am Chem Soc200813039131101311918771264
- HolzgrabeUDingermannTZundorfIAn old pathway to new antibiotics – platensimycin. Alte wege zu neuen antibiotika – platensimycinPharm Unserer Zeit2006355388389 German17009781
- PriceACChoiKHHeathRJLiZWhiteSWRockCOInhibition of β-ketoacyl-acyl carrier protein synthases by thiolactomycin and cerulenin. Structure and mechanismJ Biol Chem200127696551655911050088
- NicolaouKCLiAEdmondsDJTotal synthesis of platensimycinAngew Chem Int Ed Engl200645427086709017013803
- KaliappanKPRavikumarVAn expedient enantioselective strategy for the oxatetracyclic core of platensimycinOrg Lett20079122417241917489601
- ZouYChenCHTaylorCDFoxmanBMSniderBBFormal synthesis of (+/−)-platensimycinOrg Lett2007991825182817407302
- LiPPayetteJNYamamotoHEnantioselective route to platensimycin: an intramolecular Robinson annulation approachJ Am Chem Soc2007129319534953517630748
- NicolaouKCListerTDentonRMMonteroAEdmondsDJAdamantaplatensimycin: a bioactive analogue of platensimycinAngew Chem Int Ed Engl200746254712471417514775
- PoteraCNovel pentacyclic from soils blocks bacterial fatty acid synthesisMicrobe20061350351
- ManallackDCrosbyIKhakhamYCapuanoBPlatensimycin: a promising antimicrobial targeting fatty acid synthesisCurr Med Chem200815770571018336284
- JayasuriyaHHerathKBZhangCIsolation and structure of platencin: a FabH and FabF dual inhibitor with potent broad-spectrum antibiotic activityAngew Chem Int Ed Engl200746254684468817487925
- PearsonHAntibiotic faces uncertain futureNature2006441709126026116710378
- ChanDIVogelHJCurrent understanding of fatty acid biosynthesis and the acyl carrier proteinBiochem J2010430111920662770
- HeathRJWhiteSWRockCOLipid biosynthesis as a target for antibacterial agentsProg Lipid Res200140646749711591436
- CampbellJWCronanJEJrBacterial fatty acid biosynthesis: targets for antibacterial drug discoveryAnnu Rev Microbiol20015530533211544358
- MiyakawaSSuzukiKNotoTHaradaYOkazakiHThiolactomycin, a new antibiotic. IV Biological properties and chemotherapeutic activity in miceJ Antibiot (Tokyo)19823544114197096196
- HolfordNHSheinerLBKinetics of pharmacologic responsePharmacol Ther19821621431666752972
- JangKPKimCHNaSW7-phenylplatensimycin and 11-methyl-7-phenylplatensimycin: more potent analogs of platensimycinBioorg Med Chem Lett20102072156215820207542
- BarykinaOVRossiKLRybakMJSniderBBSynthesis and antibacterial properties of (−)-nor-platencinOrg Lett200911225334533719845392
- PatraMGasserGWenzelMMerzKBandowJEMetzler-NolteNSynthesis and biological evaluation of ferrocene-containing bioorganometallics inspired by the antibiotic platensimycin lead structureOrganometallics20102943124319
- WangJLeeVSintimHOEfforts towards the identification of simpler platensimycin analogues – the total synthesis of oxazinidinyl platensimycinChemistry200915122747275019212990
- WangJSintimHODialkylamino-2,4-dihydroxybenzoic acids as easily synthesized analogues of platensimycin and platencin with comparable antibacterial propertiesChemistry201117123352335721322068
- CohenRApproaches to reduce antibiotic resistance in the communityPediatr Infect Dis J2006251097798017006310
- PalanichamyKKaliappanKPDiscovery and syntheses of “superbug challengers” – platensimycin and platencinChem Asian J20105466870320209576
- SilverLLChallenges of antibacterial discoveryClin Microbiol Rev20112417110921233508
- BrownAKTaylorRCBhattAFuttererKBesraGSPlatensimycin activity against mycobacterial β-ketoacyl-ACP synthasesPLoS One200947e630619609444
- NicolaouKCTriaGSEdmondsDJTotal synthesis of platencinAngew Chem Int Ed Engl20084791780178318246566
- HayashidaJRawalVHTotal synthesis of (+/–)-platencinAngew Chem Int Ed Engl200847234373437618454442
- TiefenbacherKMulzerJSynthesis of platensimycin. Synthese von platensimycinAngew Chem20081201425822590 German
- YunSYZhengJCLeeDConcise synthesis of the tricyclic core of platencinAngew Chem Int Ed Engl200847336201620318548466
- WaalboerDCJSchaapmanMCvan DelftFLRutjesFPHigh-pressure entry into platencinAngew Chem Int Ed Engl200847356576657818666193
- NicolaouKCTohQYChenDYAn expedient asymmetric synthesis of platencinJ Am Chem Soc200813034112921129318665593
- AustinKABanwellMGWillisACA formal total synthesis of platencinOrg Lett200810204465446818798641
- JayasuriyaHHerathKBOndeykaJGStructure of homoplatensimide A: a potential key biosynthetic intermediate of platensimycin isolated from Streptomyces platensisTetrahedron Lett2008492236483651
- HerathKBZhangCJayasuriyaHStructure and semisynthesis of platensimide A, produced by Streptomyces platensisOrg Lett20081091699170218393511
- ZhangCOndeykaJZinkDLBurgessBWangJSinghSBIsolation, structure and fatty acid synthesis inhibitory activities of platensimycin B1–B3 from Streptomyces platensisChem Commun (Camb)2008405034503618931777
- SinghSBJayasuriyaHHerathKBIsolation, enzyme-bound structure, and activity of platensimycin A1 from Streptomyces platensisTetrahedron Lett2009503751825185
- WuMSinghSBWangJAntidiabetic and antisteatotic effects of the selective fatty acid synthase (FAS) inhibitor platensimycin in mouse models of diabetesProc Natl Acad Sci U S A2011108135378538321389266
- SkoldOAntibiotics and Antibiotic ResistanceHoboken, NJJohn Wiley & Sons2011