Abstract
Purpose
Microbial coinfections in COVID-19 patients carry a risk of poor outcomes. This study aimed to characterize the clinical and microbiological profiles of coinfections in patients with COVID-19.
Methods
A retrospective review of the clinical and laboratory records of COVID-19 patients with laboratory-confirmed infections with bacteria, fungi, and viruses was conducted. Only adult COVID-19 patients hospitalized at participating health-care facilities between February 1 and July 31, 2020 were included. Data were collected from the centralized electronic system of Dubai Health Authority hospitals and Sheikh Khalifa General Hospital Umm Al Quwain.
Results
Of 29,802 patients hospitalized with COVID-19, 392 (1.3%) had laboratory-confirmed coinfections. The mean age of patients with coinfections was 49.3±12.5 years, and a majority were male (n=330 of 392, 84.2%). Mean interval to commencement of empirical antibiotics was 1.2±3.6) days postadmission, with ceftriaxone, azithromycin, and piperacillin–tazobactam the most commonly used. Median interval between admission and first positive culture (mostly from blood, endotracheal aspirates, and urine specimens) was 15 (IQR 8–25) days. Pseudomonas aeruginosa, Klebsiella pneumoniae, and Escherichia coli were predominant in first positive cultures, with increased occurrence of Stenotrophomonas maltophilia, methicillin-resistant Staphylococcus aureus, Acinetobacter baumannii, Candida auris, and Candida parapsilosis in subsequent cultures. The top three Gram-positive organisms were Staphylococcus epidermidis, Enterococcus faecalis, and Staphylococcus aureus. There was variability in levels of sensitivity to antibiotics and isolates harboring mecA, ESBL, AmpC, and carbapenemase-resistance genes were prevalent. A total of 130 (33.2%) patients died, predominantly those in the intensive-care unit undergoing mechanical ventilation or extracorporeal membrane oxygenation.
Conclusion
Despite the low occurrence of coinfections among patients with COVID-19 in our setting, clinical outcomes remained poor. Predominance of Gram-negative pathogens, emergence of Candida species, and prevalence of isolates harboring drug-resistance genes are of concern.
Introduction
COVID-19 is a viral respiratory illness caused by severe acute respiratory syndrome coronavirus 2 (SARS-CoV2), which was first identified in Wuhan, China in December 2019. The outbreak was declared a pandemic by the World Health Organization on March 11, 2020. Although a majority of patients with COVID-19 have mild symptoms, some experience a severe clinical course characterized by multisystemic and life-threatening manifestations, with pneumonia and acute respiratory distress as prominent features.Citation1,Citation2 The presence of comorbidities, such as hypertension, diabetes, and obesity, as well as old age, have been linked with increased COVID-19 severity and mortality.Citation1
Microbial coinfections have been postulated to negatively impact host immunofunction and response to antibacterial therapy, which may contribute to poor patient outcomes.Citation3 Indeed, bacterial coinfection in viral pneumonia is a recognized predictor of mortality.Citation4,Citation5 During previous coronavirus outbreaks (SARS-CoV and Middle East respiratory syndrome coronavirus) coinfections with respiratory pathogens, including influenza, human metapneumovirus, Chlamydophila pneumoniae, and Mycoplasma pneumoniae, were reported.Citation3,Citation6–Citation8 In the ongoing COVID-19 pandemic, viral, bacterial, and fungal coinfections have been reported among patients with COVID-19.Citation9,Citation10 In a systematic review evaluating the burden of coinfections in patients with COVID-19, it was shown that 7% of patients hospitalized with COVID-19 had bacterial coinfections, increasing to 14% in studies that included only intensive-care unit (ICU) patients.Citation10 In addition, bacterial coinfection was a predictor of mortality.Citation10
Frequent use of empirical antibiotics in patients hospitalized with COVID-19 poses a risk of selection for antibiotic-resistant strains.Citation11 Additionally, coinfections with multidrug-resistant pathogens could contribute to prolonged duration of hospitalization, increased treatment costs, and poor clinical outcomes in patients with COVID-19. Current reports on coinfections in COVID-19 patients do not provide the full microbiological profiles of the pathogens identified.Citation9,Citation10 Therefore, to address this gap in the literature, this study was carried out to describe the prevalence and outcomes of coinfections in patients with COVID-19 and characterize the spectrum of causative agents and their antimicrobial-susceptibility profiles.
Methods
Study Design and Sites
This was a retrospective-cohort observational study of adult patients hospitalized with confirmed COVID-19 infections at participating centers between February 1 and July 31, 2020. A positive COVID-19 case was defined as person with a confirmed positive result on a nasopharyngeal swab tested using RT-PCR testing for SARS-CoV2. The study was carried out in the Dubai and Umm Al Quwain emirates of the United Arab Emirates. Data were collected from the centralized electronic system that covers all Dubai Health Authority hospitals and from Sheikh Khalifa General Hospital, which is the main COVID-19 facility in Umm Al Quwain. Only adult patients hospitalized with COVID-19 were included, and patients under the age of 18 years and pregnant women were excluded. Ethics approval for the study was obtained from the Dubai Scientific Research Ethics Committee (DSREC-06/2020_24) and Emirates Institutional Review Board for COVID-19 Research (DOH/CVDC/2020/1359). A waiver for patient consent was given by the ethics committee, as this was a retrospective analysis of data already on the electronic medical records. Patient-data confidentiality was maintained, and the study was carried out in compliance with the Declaration of Helsinki.
Data Collection
Clinical and microbiological data of eligible patients were extracted from medical and laboratory electronic systems. These included underlying comorbidities, duration of hospitalization, COVID-19–specific and other anti-infective therapy, clinical progression, including ICU admission and ventilation, and outcomes. From the microbiology-laboratory records, we obtained information on specimen types and microbiological investigation carried out, pathogens identified, antimicrobial-susceptibility profiles, and resistance genes. Positive cultures from specimens obtained for screening purposes (eg, methicillin-resistant Staphylococcus aureus screening) or those reported as contaminants (eg, from blood or urine cultures) were excluded. Microbiological investigations and reporting in the diagnostic laboratories were carried out using standard protocols in keeping with Clinical and Laboratory Standards Institute guidelines.Citation12 BioFire FilmArray (BioMérieux, Marcy l’Etoile, France) respiratory-panel and pneumonia-panel assays were carried out in accordance with manufacturer protocols.
Statistical Analysis
Data were entered and analysed using SPSS 24. Descriptive statistics for categorical variables are presented as numbers and percentages and continuous variables as means ± SD or medians and IQRs.
Results
During the study period, 29,802 patients with COVID-19 were hospitalized across participating centers. We identified 392 patients (1.3%) with laboratory-confirmed coinfections. The mean age of patients with coinfections was 49.3±12.5 years, and a majority were male (330 of 392, 84.2%). The predominant comorbidities identified were diabetes (117 of 392, 29.8%), hypertension (95 of 392, 24.2%), and asthma and cardiac disease (18 of 392, 4.6% each). A majority of patients received lopinavir–ritonavir (n=153) and favipiravir (n=111) as initial drugs for SARS-CoV2, while 68 received hydroxychloroquine. Of the 392 patients with coinfections, 130 (33.2%) died, predominantly those who required ICU care, mechanical ventilation, or extracorporeal membrane oxygenation (). The demographic and clinical profile of patients with coinfections is shown in .
Table 1 Demographic and clinical profile of COVID-19 patients with coinfections
The median duration of hospitalization was 21 (IQR 12–37) days. Mean interval between hospitalization and commencement of antibiotics was 1.2±3.6 days. The most common antibiotics administered were ceftriaxone (n=136), azithromycin (n=74), and piperacillin–tazobactam (n=41). The BioFire FilmArray respiratory panel using nasopharyngeal swab specimens was carried out on admission for 81 patients, of whom only two were positive (one each for rhinovirus and parainfluenza virus). The pneumonia panel was carried out using lower respiratory tract specimens for 48 patients, and this was done within 96 hours of admission for all patients, except two, who had the test done on days 13 and 14 of hospitalization. A majority of the tested patients (34 of 48, 62.5%) were positive, with the predominant organism being Haemophilus influenzae, followed by S. aureus. Fifteen patients had single-organism infections, predominantly H. influenzae (n=12, ) while eight of the 13 patients with two-organism infections and all six patients with three-organism infections harbored H. influenzae ().
Table 2 Respiratory pathogens detected in patients positive for more than one organism on the pneumonia panel
Figure 1 Distribution of respiratory pathogens among patients with a single organism detected on pneumonia panel.
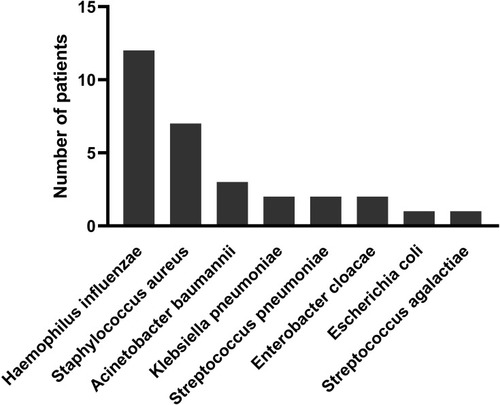
All 392 patients had laboratory-confirmed coinfections based on positive microbiological cultures. A majority (221 of 392) had single microbiological culture results. There were 171 patients considered to have superinfections, as they had more than one positive-culture result (range two to six) based on isolates from different body sites during their hospitalization. Median interval between admission and first positive-culture report was 15 (IQR 8–25) days. A majority of microbial cultures were identified from blood and central-line cultures, followed by endotracheal aspirates and urine (). When we looked specifically at first positive-culture results, the most commonly identified organisms were Gram-negative pathogens, namely Pseudomonas aeruginosa, Klebsiella pneumoniae, and Escherichia coli. Among the Gram-positive organisms, Staphylococcus epidermidis, Enterococcus faecalis, and S. aureus were predominant, while Candida albicans and Candida tropicalis were the most common fungal pathogens. shows the top 20 microorganisms identified from first positive cultures (Supplementary Figure 1 shows all organisms identified). However, when all available positive microbial cultures from the entire period of hospitalization were considered, increased prevalence was found for Stenotrophomonas maltophilia, methicillin-resistant S. aureus, and Acinetobacter baumannii, and the emergence of Candida auris and Candida parapsilosis among the top 20 organisms was observed (). A summary of the distribution of sensitivity to antibiotics tested is shown in . There was variability in sensitivity for antibiotics, with a trend toward resistance. For the commonly administered antibiotics, susceptibility testing was done infrequently for ceftriaxone (n=8), and only 40% of the 75 isolates tested for piperacillin–tazobactam were sensitive. There were 41 S. aureus isolates positive for the mecA gene while 65 E. coli and 39 K. pneumoniae isolates were identified as ESBL producers. There were 33 AmpC-producer isolates, comprised of E. coli (n=11), Serratia spp. (n=9), Enterobacter cloacae (n=8), Klebsiella spp. (n=4), and Morganella morganii (n=1). A majority of the 52 carbapenem-resistant isolates identified were P. aeruginosa (n=48), and the rest were K. pneumoniae (n=4).
Table 3 Distribution of antimicrobial susceptibility in positive cultures
Discussion
Bacterial coinfections occurring in patients with viral lower respiratory tract infections are frequently associated with significant morbidity and mortality. Based on this premise and experience from previous influenza pandemics, the use of empirical antibiotics in SARS-CoV2–infected patients was instituted in the ongoing COVID-19 pandemic.Citation13 However, the pattern of coinfections in patients infected with SARS-CoV2 and the microbiological profile of the spectrum of causative agents remains a crucial knowledge gap in the literature. Specifically, we identified the absence of relevant epidemiological data from our region on the pattern of coinfections among COVID-19 patients, and this is the first study focusing on this coinfection pattern.
The findings from this study indicated that there is a low incidence of laboratory-confirmed coinfections among hospitalized COVID-19 patients in our setting. We also found that clinical outcomes worsened if patients were in ICUs requiring high-level clinical care. In a report from Iran, a 12.4% occurrence of bacterial infections was reported among 340 patients with COVID-19.Citation14 In a large systematic review of 30 studies, bacterial coinfection was reported in 7% of patients hospitalised with COVID-19, which increased to 14% when only ICU patients were reported.Citation10 Similarly, a review of nine studies from China and the US showed that among 806 patients with COVID-19, 62 (8%) developed bacterial and fungal coinfections.Citation15 Among 338 patients hospitalized with COVID-19 in New York, a 6% rate of bacteremia was found,Citation16 whilst Zhou et al reported a 15% rate of bacterial coinfection, with 96% mortality.Citation17 However, these studies did not indicate if these were nosocomial infections nor did they provide information on how many of these patients required critical care. In contrast to these reports, our findings showed much lower rates of coinfections in patients hospitalized with COVID-19. Our finding of worsened clinical outcomes among those in ICUs requiring high-level clinical care is in keeping with the higher incidence of sepsis and mortality reported among COVID-19 patients in these units.Citation18–Citation20
Findings from the few patients with molecular screening suggested that viral coinfection and specifically Middle East respiratory syndrome coronavirus was not detected. This is similar to a report from Saudi Arabia.Citation21 Furthermore, based on the median interval between admission and first positive-culture report, we surmise that a majority of our patients had nosocomial infections, which is of significance, as initiation of empirical antimicrobial therapy using broad-spectrum antibiotics was commenced very early. This is in keeping with previously reported work that showed that bacterial coinfection was rarely documented in COVID-19 patients at admission and suggests that careful review of routine initiation of empirical antibiotics in COVID-19 patients is warranted.Citation22
Our findings indicate that Gram-negative pathogens maintained an overall predominance as causative agents of initial and subsequent infections. This finding of a predominance of Gram-negative bacteria is in keeping with a pattern reported in a systematic review of 30 studies reporting coinfections in 3,834 patients with COVID-19.Citation22 Although there was variability in antimicrobial resistance, the bacterial isolates showed a higher trend of antibiotic resistance than the fungal isolates, which had high sensitivity to the antifungal tested. Additionally, commonly used empirical antibiotics were infrequently tested or exhibited low susceptibility profiles. Our findings also indicate that carriage of mecA, ESBL, AmpC, and carbapenemase-resistance genes were prevalent among the isolates identified. This is in keeping with the high occurrence of isolates harboring these resistance genes in our setting.Citation23–Citation25 As such, it is important that this molecular epidemiological pattern be factored into the selection of empirical antibiotics.
The emergence of fungal agents, especially C. auris and C. parapsilosis, during subsequent cultures is of concern. Although other reports have identified COVID-associated pulmonary aspergillosis as a frequent coinfection in critically ill patients, our findings did not reflect this.Citation26 However, as higher occurrence of poor clinical progression and mortality has been reported for COVID-19 patients with fungal coinfections, this finding remains worrisome.Citation26–Citation30 We hypothesize that the increment in fungal infections was most likely due to the acquisition of these pathogens in the hospital setting and the higher susceptibility of the patients, given their treatment with broad-spectrum antibiotics and immunosuppressive therapies.Citation27 This could also explain why some of our patients subsequently had multiple organisms cultured from different body sites during the clinical course of their illness.
A limitation of this study is that data of patients hospitalized with COVID-19 infection who did not develop coinfections was not obtained. We recommend that future work include such data to enable comparative analysis.
Conclusion
This is the first descriptive report on coinfections among patients hospitalized with COVID-19 in our setting. Although the occurrence of these coinfections was low, patients demonstrated a tendency toward poor clinical outcomes. Additionally, the predominance of Gram-negative pathogens, emergence of Candida infections, and presence of bacterial isolates harboring drug-resistant genes are of concern. We recommend that clinicians remain mindful of these factors in their selection of empirical antimicrobial agents. Furthermore, utilization of rapid bacteriological testing approaches coupled with antimicrobial-stewardship programs to ensure judicious use of antibiotics in the context of COVID-19 is crucial.
Disclosure
The authors report no conflicts of interest in this work.
References
- Huang C, Wang Y, Li X, et al. Clinical features of patients infected with 2019 novel coronavirus in Wuhan, China. Lancet. 2020;395(10223):497–506. doi:10.1016/S0140-6736(20)30183-531986264
- Uddin M, Mustafa F, Rizvi TA, et al. SARS-CoV-2/COVID-19: viral genomics, epidemiology, vaccines, and therapeutic interventions. Viruses. 2020;12(5):526. doi:10.3390/v12050526
- Li XX, Zhou XN. Co-infection of tuberculosis and parasitic diseases in humans: a systematic review. Parasit Vectors. 2013;6(1):79. doi:10.1186/1756-3305-6-7923522098
- Guo L, Wei D, Zhang X, et al. Clinical features predicting mortality risk in patients with viral pneumonia: the mulbsta score. Front Microbiol. 2019;10:2752. doi:10.3389/fmicb.2019.0275231849894
- Mirzaei R, Goodarzi P, Asadi M, et al. Bacterial co-infections with SARS-CoV-2. IUBMB Life. 2020;72(10):2097–2111. doi:10.1002/iub.235632770825
- Alfaraj SH, Al-Tawfiq JA, Altuwaijri TA, Memish ZA. Middle east respiratory syndrome coronavirus and pulmonary tuberculosis coinfection: implications for infection control. Intervirology. 2017;60(1–2):53–55. doi:10.1159/00047790828683463
- Alfaraj SH, Al-Tawfiq JA, Alzahrani NA, Altwaijri TA, Memish ZA. The impact of co-infection of influenza a virus on the severity of Middle East respiratory syndrome coronavirus. J Infect. 2017;74(5):521–523. doi:10.1016/j.jinf.2017.02.00128189714
- Lee N, Chan PK, Yu IT, et al. Co-circulation of human metapneumovirus and SARS-associated coronavirus during a major nosocomial SARS outbreak in Hong Kong. J Clin Virol. 2007;40(4):333–337. doi:10.1016/j.jcv.2007.08.01517936066
- Lai CC, Wang CY, Hsueh PR. Co-infections among patients with COVID-19: the need for combination therapy with non-anti-SARS-CoV-2 agents? J Microbiol Immunol Infect. 2020;53(4):505–512. doi:10.1016/j.jmii.2020.05.01332482366
- Lansbury L, Lim B, Baskaran V, Lim WS. Co-infections in people with COVID-19: a systematic review and meta-analysis. J Infect. 2020;81(2):266–275. doi:10.1016/j.jinf.2020.05.04632473235
- Langford BJ, So M, Raybardhan S, et al. Antibiotic prescribing in patients with COVID-19: rapid review and meta-analysis. Clin Microbiol Infect. 2021;27(4):520–531. doi:10.1016/j.cmi.2020.12.01833418017
- Institute CaLS. M100 Performance Standard for Antimicrobial Susceptibility Testing. 2019.
- Alhazzani W, Moller MH, Arabi YM, et al. Surviving sepsis campaign: guidelines on the management of critically ill adults with coronavirus disease 2019 (COVID-19). Crit Care Med. 2020;48(6):e440–e469. doi:10.1097/CCM.000000000000436332224769
- Mahmoudi H. Bacterial co-infections and antibiotic resistance in patients with COVID-19. GMS Hyg Infect Control. 2020;15:Doc35.33391970
- Rawson TM, Moore LSP, Zhu N, et al. Bacterial and fungal coinfection in individuals with coronavirus: a rapid review to support COVID-19 antimicrobial prescribing. Clin Infect Dis. 2020;71(9):2459–2468. doi:10.1093/cid/ciaa53032358954
- Goyal P, Choi JJ, Pinheiro LC, et al. Clinical characteristics of COVID-19 in New York City. N Engl J Med. 2020;382(24):2372–2374. doi:10.1056/NEJMc201041932302078
- Zhou F, Yu T, Du R, et al. Clinical course and risk factors for mortality of adult inpatients with COVID-19 in Wuhan, China: a Retrospective Cohort Study. Lancet. 2020;395(10229):1054–1062. doi:10.1016/S0140-6736(20)30566-332171076
- King CS, Sahjwani D, Brown AW, et al. Outcomes of mechanically ventilated patients with COVID-19 associated respiratory failure. PLoS One. 2020;15(11):e0242651. doi:10.1371/journal.pone.024265133227024
- MacLaren G, Combes A, Brodie D. What’s new in ECMO for COVID-19? Intensive Care Med. 2021;47(1):107–109. doi:10.1007/s00134-020-06284-z33180168
- Roedl K, Jarczak D, Thasler L, et al. Mechanical ventilation and mortality among 223 critically ill patients with coronavirus disease 2019: a Multicentric Study in Germany. Aust Crit Care. 2021;34(2):167–175. doi:10.1016/j.aucc.2020.10.00933250401
- Ebrahim SH. Lack of MERS-CoV co-infection among hospitalized COVID-19 patients in Saudi Arabia. J Epidemiol Glob Health. 2020;10(3):191. doi:10.2991/jegh.k.200819.00132954705
- Karaba SM, Jones G, Helsel T, et al. Prevalence of co-infection at the time of hospital admission in COVID-19 patients, a Multicenter Study. Open Forum Infect Dis. 2021;8(1):ofaa578. doi:10.1093/ofid/ofaa57833447639
- Al Jalaf M, Fadali H, Alanee R, et al. Methicillin resistant Staphylococcus aureus in emergency department patients in the United Arab Emirates. BMC Emerg Med. 2018;18(1):12. doi:10.1186/s12873-018-0164-729764376
- Moubareck CA, Mouftah SF, Pal T, et al. Clonal emergence of Klebsiella pneumoniae ST14 co-producing OXA-48-type and NDM carbapenemases with high rate of colistin resistance in Dubai, United Arab Emirates. Int J Antimicrob Agents. 2018;52(1):90–95. doi:10.1016/j.ijantimicag.2018.03.00329530587
- Senok A, Nassar R, Celiloglu H, et al. Genotyping of methicillin resistant Staphylococcus aureus from the United Arab Emirates. Sci Rep. 2020;10(1):18551. doi:10.1038/s41598-020-75565-w33122734
- Velez Pintado M, Camiro-Zuniga A, Aguilar Soto M, et al. COVID-19-associated invasive pulmonary aspergillosis in a tertiary care center in Mexico City. Med Mycol. 2021. doi:10.1093/mmy/myab009
- Antinori S, Bonazzetti C, Gubertini G, et al. Tocilizumab for cytokine storm syndrome in COVID-19 pneumonia: an increased risk for candidemia? Autoimmun Rev. 2020;19(7):102564. doi:10.1016/j.autrev.2020.10256432376396
- Chowdhary A, Tarai B, Singh A, Sharma A. Multidrug-resistant candida auris infections in critically ill coronavirus disease patients, India, April–July 2020. Emerg Infect Dis. 2020;26(11):2694–2696. doi:10.3201/eid2611.20350432852265
- Mastrangelo A, Germinario BN, Ferrante M, et al. Candidemia in COVID-19 patients: incidence and characteristics in a prospective cohort compared to historical non-COVID-19 controls. Clin Infect Dis. 2020. doi:10.1093/cid/ciaa1594
- Rodriguez JY, Le Pape P, Lopez O, Esquea K, Labiosa AL, Alvarez-Moreno C. Candida auris: a latent threat to critically ill patients with COVID-19. Clin Infect Dis. 2020. doi:10.1093/cid/ciaa1595