Abstract
The percentages of organisms exhibiting antimicrobial resistance, especially resistance to multiple antibiotics, are incessantly increasing. Studies investigated that many bacteria are being resistant to ciprofloxacin. This review addresses the current knowledge on nano-based ciprofloxacin delivery approaches to improve its effectiveness and overcome the resistance issues. Ciprofloxacin delivery can be modified by encapsulating with or incorporating in different polymeric nanoparticles such as chitosan, PLGA, albumin, arginine, and other organic and inorganic nanostructure systems. Most of these nano-approaches are promising as an alternative strategy to improve the therapeutic effectiveness of ciprofloxacin in the future.
Introduction
Antibiotics are considered the most important advances in the history of modern medicine due to their phenomenal impact on reducing human morbidity, mortality, and economic losses. However, bacteria that challenge not only single but multiple antibiotics have become increasingly common through time. Antimicrobial resistance (AMR) is now the most important challenge in the fight against infectious diseases which is associated with inappropriate use and abuse of the drugs.Citation1 The number of microbial strains resisting, the geographical coverage, and the extent of resistance toward multiple antibiotics are accelerating. Many drugs with known susceptibility to antibiotics therapy are now returning in new habits as resistant to those therapies.Citation2
Quinolones are among great achievements in the antibiotic discovery. The first groups possessing a narrow range of action and unfavorable drug kinetics rose in the early 1960s. Through time, new quinolones have been developed with improved efficacy and spectrum. Nowadays, they are being widely prescribed for a variety of infectious diseases even though AMR is becoming a tackle against their effectiveness.Citation3 They are known to act by inhibiting type 2 bacterial DNA topoisomerases, DNA gyrase, and topoisomerase IV which are the commonest areas of quinolone resistance now. They are classified with generations as first generation (nalidixic acid), second generation (norfloxacin, ciprofloxacin, ofloxacin, enoxacin, lomefloxacin), third generation (moxifloxacin, levofloxacin, gatifloxacin, sparfloxacin), and fourth generation (trovafloxacin). The first generation was effective against Gram-negative bacteria excluding Pseudomonas species but the spectrum broadens toward the new generations.Citation4 Ciprofloxacin is used for the treatment of a wide range of infections, such as complicated urinary tract infections, typhoid, bone or joint infections, shigellosis, cystic fibrosis, chronic suppurative otitis media, prostatitis, and epididymo-orchitis. It is one of the topmost selling antibiotics being available at a cheaper cost and with different drug delivery systems like: oral tablets, eye drops, suspensions, ointments, infusion, in-situ gel, hydrogels, films, microbeads, elastic liposomes, floating matrix tablets, ocuserts, and niosomal cream.Citation5
Even though a substantial and momentary reduction of infections caused by resistant bacteria was attained by quinolones, concerns about treatment failure and bacterial resistance appeared shortly after their innovation.Citation6,Citation7 Alarms regarding the suitable use of fluoroquinolones and the quality of their pharmaceutically equivalent preparations have been knocking after the therapeutic failures and amplified bacterial resistance were reported.Citation4 Onwards, measures become in need to combat this resistance risk and get the most out of their effectiveness. Research and feedback mechanisms, antimicrobial stewardship programs (ASP), susceptibility testing, and targeted clinical response are among the approaches toward fighting AMR. The development of new antibiotics and discovery of novel drug delivery systems should also be considered. To be successful, determined efforts of all members of the society are necessary.Citation8 With the growth of innovation and technology, there has been much interest in possible alternative antimicrobial therapies through nanotechnology. The scenarios for conjugates or combinations of nanomaterials (NMs) and the conventional antibiotic drugs for targeting and combating microbial resistance are in a careful optimism.Citation9 Nanomaterials can carry or transport antimicrobials; deliver them into the target, or ultimately act synergistically by their inherent antimicrobial activity. Moreover, nanoparticles (NPs) may contest the mechanisms of bacterial drug resistance with different mechanisms. They can inhibit some important bacterial processes like biofilm formation.Citation10 In this review, the different NMs and associated nanotechnology-based ciprofloxacin delivery approaches against resistant microbes have been discussed.
Resistance Challenges of the Conventional Ciprofloxacin Delivery
Ciprofloxacin-Resistant Problematic Groups and Challenges
Ciprofloxacin has been shown to be active against various Gram-positive and Gram-negative bacterial isolates such as: E. coli, H. influenzae, other H. spp., N. gonorrhoeae, N. meningitides, Moraxella catarrhalis, K. pneumoniae, Legionella pneumophila, Moraxella catarrhalis, Proteus mirabilis, and P. aeruginosa, methicillin-susceptible S. aureus (MSSA), S. pneumoniae, S. epidermidis, E. faecalis, and S. pyogenes both in vitro and in vivo.Citation4 It has been used successfully since 1987 and included in the WHO’s essential medicines list.Citation11 However, an increasing proportion of bacterial clinical isolates are being resistant to ciprofloxacinCitation6 that induces higher rates of hospitalization, greater length of hospital stay, and higher rates of illness and death.Citation12
Ciprofloxacin replaced aminoglycosides (due to their resistance) as the drug of choice against keratitis caused by P. aeruginosa. However, due to its overuse, there has been a considerable increase in ciprofloxacin resistance among P. aeruginosa isolates (from <1% in 1994, to 4% in 1998, finally to 29% in 2003, and exhibit multidrug-resistant (MDR) character now). Fluoroquinolones were recommended as a drug of choice to treat gonococcal infections in 1993. In 1997, the first resistant strains were reported in Hong Kong and Philippines. In 2004, fluoroquinolone was no longer recommended for treatment due to the increased resistance and declined sensitivity of the causative agent. As a result, cephalosporins were replaced in the place of fluoroquinolones for treating gonococcal infections. summarizes the review by Ahmad et al on ciprofloxacin-resistant problematic groups of bacteria.Citation13
Table 1 Ciprofloxacin-Resistant Problematic Bacterial Groups
Mechanism for Development of Resistance
Ciprofloxacin resistance in the majority of bacterial isolates is from chromosomal mutations and/or reduced drug accumulation due to overexpression of efflux pump systems.Citation14 The efflux pump system reduces the intracellular concentrations to sub-lethal levels, while point mutations reduce drug binding to specific enzymatic sites, decreasing activity. In this regard, fluoroquinolones are prevented from binding to the enzymes involved in DNA replication, including DNA gyrase and DNA topoisomerase IV.Citation12 Genetic mutations take the vast proportion of bacterial resistances. GyrAB and parCE are target genes of P. aeruginosa for ciprofloxacin action and their mutation reduced the affinity of DNA gyrase or topoisomerase for the drug.Citation11 In Egypt, double mutations (1 gyrA/1 parC) and three mutations (2 gyrA/1 parC) were found in different bacterial species like E. coli and K. pneumoniae. Plasmid-mediated quinolone resistance is also the other genetic related resistance for ciprofloxacin. Under this group, genes like aac(6′)-Ib-cr of E. coli, qnrB/aac(6′)-Ib-cr and qnrS of K. pneumoniae, qnrB of P. aeruginosa, and qnrS of A. baumannii have been reported.Citation14 Ciprofloxacin resistance toward S. aureus is due to: 1) point mutations on encoding the subunits of topoisomerase IV and DNA gyrase; and 2) efflux by the membrane-associated protein which actively transports fluoroquinolones out of the bacterial cell.Citation15 Mutation appears to be the only mechanism to acquire resistance in M. tuberculosis and some P. aeruginosa infections. P. aeruginosa contains 12 efflux pumps and four of these are known to efflux fluoroquinolones. E. coli resistance to ciprofloxacin, both in vivo and in vitro, requires mutation.Citation16,Citation17 Fluoroquinolone resistance to Salmonella isolates includes mutations, active efflux, and plasmid-mediated protection of target topoisomerases. The acrB, soxS, marA, ramA, and rob were characterized as ciprofloxacin-resistant Salmonella enterica serotype Enteritidis mutant genes.Citation18 Whereas, resistance to N. gonorrhoeae, Campylobacter spp., and Salmonella can be chromosomally as well as plasmid-mediated and bacterial biofilm formation.Citation13
Factors Associated with Ciprofloxacin Resistance
Indiscriminate use in routine clinical practices, unnecessary consumption during viral infections, and drug overuse are the determinant factors for ciprofloxacin and other antibiotics resistance. Clinically, three major factors are associated with increasing resistance to fluoroquinolones: 1) under-dosing, ie, use of a slightly potent drug whose MIC is scarcely reached in serum or infected tissues; 2) overuse or misuse of drugs that encourage resistant mutants; and 3) failure to timely identify and reply to changes in microbial susceptibilities.Citation12 Prior use history and higher age, high intake of pork and chicken, as well as the concomitant prescription of calcium supplements or proton pump inhibitors with fluoroquinolones were reported as contributing factors for resistance to ciprofloxacin in UTIs.Citation7 The accessibility and consumption of inferior-standard and counterfeit ciprofloxacin formulations, especially in the developing countries, is another factor which has contributed to the increased risk of therapeutic failure and drug resistance. The overuse or misuse of ciprofloxacin is likely to be due to widespread availability of generic versions of ciprofloxacin.Citation4
Strategies to Minimize or Counter Ciprofloxacin Resistance
Historically, several approaches have been employed to address AMR. These include: 1) the use of a newer more potent antimicrobials; 2) continuing to use older agents, preserving the activity of the new drugs; 3) antibiotic cycling or rotation, with a predefined period, usually 3 months; 4) combination therapy; and 5) pharmacokinetic and pharmacodynamic data-based prescription have been exploited for years. Evidence-based antimicrobial selection must be implemented by clinicians.Citation12 Loading ciprofloxacin with novel approaches to target the site of infection and use of combination therapies with other anti-Pseudomonas agents are also recommended as possible complementary strategies.Citation11 Reserpine, a well-known mammalian MDR pump inhibitor, has resulted in suppression of resistance in S. aureus and S. pneumoniae strains when used in combination with ciprofloxacin. Boeravinone B 66, an inhibitor of the S. aureus multidrug efflux pump NorA, was recently shown to act synergistically with ciprofloxacin to inhibit biofilm formation by S. aureus.Citation13 New drug development alone is now becoming insufficient for safeguarding the advancement in medicine due to the failure of addressing limitations associated with poor pharmacokinetics, incomplete targeting, and toxicity. Nowadays, the application of nanostructures that have intrinsic antimicrobial potential or nanocarriers that can modify the transport and delivery of the antibiotic have been presented as new strategies and defense mechanisms against MDR infectious organisms.Citation9,Citation19 Application of nanotechnology in the pharmaceutical field offers new advancements for developing novel formulations from the diverse types of nanoparticles (NPs) considering their different sizes and shapes with their versatile antimicrobial properties. These NPs can bring a hopeful solution as they can directly target the bacteria by themselves or can act as drug carrier systems.Citation10
Nanomaterials: Alternative Delivery Systems for AMR
Nanomaterial Preparation and Mechanisms in Countering AMR
Nanotechnology is an innovative science that utilizes NMs with dimensions from 1–1000 nm. Several NMs are already used as broad-scale antimicrobial agents and carriers of antimicrobial agents in consumer products.Citation9 However, the practical applicability of different nanocarriers has been limited due to their insufficient biocompatibility, lower sensitivity (with respect to temperature and pH), and lack of complete biodegradability.Citation20 Antibiotic delivery with NPs results in improved bioavailability by enhancing aqueous solubility, increasing resistance time in the body, and targeting specific site of action.Citation21 Although the nanotechnology-associated high costs make the conventional therapy preferable, these platforms might be of utmost importance with their impact on cell wall permeability, efflux activity, reactive species formation, and metabolism and reproduction inhibition.Citation10
NMs can be incidental (byproduct of industrial and natural processes), engineered, or natural which may be produced from living organisms and plants.Citation22 They are prepared and characterized for their properties in different ways. Metallic NPs are mainly synthesized by either reducing the particle size by mechanical or chemical methods or by green synthesis using bacteria, plant extract, and fungus. Polymeric NPs are prepared by cross linking, polymerization methods, and polymer precipitation methods. states the different parameters for characterization of NPs and instruments used for these characterizations.Citation23 Representative examples for different sources of the nanomaterials used for ciprofloxacin delivery and the characterization done in the articles included in this review are listed in . NP-based drug delivery systems introduce a wide range of therapeutics, by either binding the drug to their large surface area or carrying it to the site of infection effectively, safely, and with a controlled rate of targeted delivery. They are capable of disrupting bacterial membranes and hindering biofilm formation, thus reducing the survival of the microorganism. This way, NPs provide an alternative strategy with potential to use both antibiotic-free and antibiotic-coated approaches.Citation10 Nanomaterial antibiotic functionalization includes use of polymers, dendrimers, and inorganic NPs with antibiotic molecules attached to their surfaces. This antibiotic nanomaterial functionalization results in improved effectiveness due to: 1) improved drug-delivery features, better than the conventional antibiotic alone; and 2) interesting synergistic effects. NMs themselves can damage the cell membrane, generate reactive oxygen species, and release toxic metals causing intrinsic antimicrobial effect. Rather than focusing upon particular biochemical processes as traditional antibiotics, they are likely to disrupt multiple cellular processes in a less specific fashion.Citation9
Table 2 Different Parameters for Characterization of Nanoparticles
Table 3 Sources of Nanomaterials for Ciprofloxacin Delivery
Nanotoxicity, Resistance, and Regulatory Aspects
Besides their anticipated benefits, prolonged exposure to NMs might cause nanotoxicity and thus, possible jeopardies for human health and the ecosystems. Proteins and other biomolecules from the body and the environment could rapidly bind to NMs, resulting in the formation of protein/biomolecule corona that can affect NM’s nature of interaction with the body system. Systematized knowledge on NMs toxicity is required for their rational design with reduced potential toxicity.Citation33 NPs that are inhaled or extracted from drug–NP formulations can effortlessly reach the bloodstream and then the liver, heart, or blood cells. Many of them seem to be nontoxic and others have positive health effects. The engineered, smallest size of NPs can facilitate the translocation of active chemical species from barriers of the skin, lung, body tissues, and organs. Thus, irreversible oxidative stress, organelle damage, asthma, and cancer can be caused by NPs depending on their composition. The generation of neoantigens that cause possible organ enlargement and dysfunction are common chronic toxic effects of NPs.Citation22
Even though there are not well established reports regarding the mechanism and intensity of nano-resistance to microorganisms, there are some predictions and pre-concerns. Potential novel antifungal metal-based NPs, such as ZnO, Ag, or CuO, showed fungal resistance mediated by biomolecule coronas acquired in pathophysiological and ecological environments.Citation34 The great diversity of antimicrobial mechanisms exerted by NPs suggests that bacteria are unlikely to generate resistance to NPs. However, bacterial resistance is dreaded with the mechanisms not yet been investigated more. There are predicted intrinsic and extrinsic mechanisms for NP microbial resistance. The intrinsic resistance mechanisms can include outer membrane permeability, multidrug resistance efflux pumps, downregulation of genes, and chromosomal resistance genes. In the other way, the extrinsic mechanisms are in the form of adaptive mutations, point mutations, and plasmids with resistance genes. It is important to study all the aforementioned resistance mechanisms to understand and prevent complex resistance challenges.Citation35,Citation36
In spite of the great hope on nanomedicine and its pharmaceutical applications, there is currently very little regulation that guides the area. However, the research community is calling repeatedly for providing legal certainty to the manufacturers, policymakers, healthcare providers, and the general public so as to minimize challenges in funding, research, and development of works on NMs that reduce public acceptance and perception.Citation37 Regulations via legislation, laws, and rules have been implemented by several government organizations including the USA and the European Union (EU) to minimize or avoid risks associated with NMs. However, there is no specific international regulation, no internationally agreed upon protocols or legal definitions for production, handling or labeling, testing toxicity, and evaluating the environmental impact of NPs. Extensive research in the field of nanotoxicology and strict regulation by government agencies is essential to identify and avoid toxic NPs.Citation22
Nanotechnology-Based Approaches for Ciprofloxacin Delivery
Nanoparticles of Chitosan-Loaded Ciprofloxacin
Chitosan, the amino polysaccharide copolymer of 1,4-d-glucosamine and N-acetyl glucosamine is derived from chitin by alkaline or enzymatic deacetylation. It is used for encapsulation of many antibiotics for their nano-delivery.Citation38 The minimum inhibitory concentration (MIC) of ciprofloxacin-loaded chitosan NPs was demonstrated to be 50% lower than that of ciprofloxacin HCl alone against E. coli and S. aureus. The loading resulted in increased drug penetration into the bacterial cell improving antibacterial activity with reduced dose.Citation24 Another similar study indicated uppermost ciprofloxacin antibacterial activity against E. coli and MRSA with a significant (85.6%) decrease in MIC, enhanced drug delivery, increased drug penetration, and controlled release.Citation25 Ciprofloxacinchitosan microparticles for local drug delivery to the lungs against pneumonia causing agents, namely E. coli, P. aeruginosa, and S. aureus demonstrated good loading efficiency, enhanced localized effects, better escaping of the body’s local defense system, improved antibacterial effects, and less cytotoxicity to human lung epithelial cells.Citation39
In vitro evaluation of sustained ciprofloxacin release from chitosan/hydroxyapatite hydrogel nanocomposites exhibited a sustained antibacterial activity against S. aureus and E. coli through a diffusion process promising for designing prolonged release drug delivery systems.Citation40 Other in vitro release data of ciprofloxacin with chitosan–alginate systems promised an alternative system for the sustained delivery of ciprofloxacin.Citation19 A study on chitosan bandages loaded with ciprofloxacin for polymicrobial wound infections demonstrated good flexibility, tensile strength, porosity, and wound exudate absorption. The bandage released ciprofloxacin for up to 14 days in a sustained manner and showed a significant antimicrobial activity both in vitro and in vivo toward poly-microbial cultures of C. albicans, E. coli, and S. aureus.Citation41 The other important investigation of chitosan–ciprofloxacin NPs is targeting. A study conducted on the ciprofloxacin capsule system encapsulated by chitosan and dextran sulfate biopolymers demonstrated improved targeting of the Salmonella containing vacuoles to kill the pathogen so that complete cure can be achieved without any relapse.Citation42 In another study, ciprofloxacin was loaded into chitosan/heparin NPs to target enteropathogenic bacteria. The synergistic effect reduced the MIC of free ciprofloxacin against E. coli by half and a targeted killing of 60% load within 30 min.Citation43
Ciprofloxacin-Loaded PLGA/PCL Nanoparticles
The polymeric NPs of poly(d,l-lactide-coglycolide) (PLGA) and poly(ε-caprolactone) (PCL) have shown significant therapeutic potential to achieve controlled drug delivery. An investigation on the incorporation of ciprofloxacin encapsulated PLGA and PCL NPs on tissue-engineered scaffolds resulted in high encapsulation efficiency, better stability, predictable controlled release, and targeting the site of action. Additionally, both the encapsulated NPs were safer and effective against S. aureus and P. aeruginosa compared to the free drug.Citation29 Ciprofloxacin-loaded PLGA electrospun fibers also demonstrated effective antibacterial properties against P. aeruginosa, S. aureus, and S. epidermidis.Citation44 Türeli et al studied the encapsulation efficiency, release pattern, cytotoxicity, and antibacterial activity of ciprofloxacin-loaded PLGA NPs. The result revealed high drug loading, promising penetration into the mucus where the bacteria reside, controlled release, sustained local drug concentration, and enhanced anti-P. aeruginosa activity with reduced dose, thus reduced side effects.Citation45 NP parameter optimization enabled ciprofloxacin-loaded PLGA NP preparations with pre-defined quality to fulfill the requirements of local drug delivery under cystic fibrosis disease conditions.Citation46 Similarly, a study on ciprofloxacin-loaded PLGA NPs for eradicating P. aeruginosa biofilm showed a steady release of the antibiotic and the repeated application of the NPs for 3 days resulted in biofilm reduction by 95%.Citation47 Evaluation of the efficacy of sustained release PLGA micro- and nanoparticles containing ciprofloxacin against bacterial biofilms demonstrated a sustained release over 6 days to effectively eradicate culturable S. aureus. The formulation might be a valuable alternative for the treatment of biofilms by achieving high local and sustained drug concentrations while minimizing systemic adverse effects and improving patient compliance.Citation48
Liposomal and Micellar NPs for Ciprofloxacin Delivery
The efficacy of liposomal antibiotics has been shown in numerous in vivo studies to be clearly superior to that of the free antibiotics. But, formulation factors such as liposome’s membrane rigidity, surface charge, size, and polymer hydrophobicity must be controlled and optimized.Citation49 Liposomal ciprofloxacin formulations have been developed for enhancing lung residence time reducing the burden of inhaled antimicrobial therapy from multiple daily administrations due to rapid absorptive clearance of antibiotics from the lungs.Citation50 In a study comparing the release of ciprofloxacin from the liposomal formulation and a solution formulation for inhaled drug delivery, the liposomal formulation provided zero-order release kinetics that prolonged residence time in the lungs.Citation51 Liposomal ciprofloxacin administered intravenously and intraperitoneally to mice infected with intracellular S. typhimurium demonstrated an increased plasma habitation time and concentration of drug in the liver, spleen, lungs, and kidneys. Besides, an intratracheal administration increased pulmonary drug retention. It has also an extended survival and can reduce the number of bacteria in the liver and spleen compared with the free drug.Citation52 Mannosylated liposomes loaded with ciprofloxacin were investigated for targeting against intracellular infections of the respiratory system. Upon pulmonary administration on rats, they demonstrated an efficient macrophage targeting.Citation53
Ciprofloxacin-loaded liposome against Salmonella Dublin demonstrated greater stability and antimicrobial properties than free ciprofloxacin. It helps the drug to be accumulated in the spleen and liver persisting for 48 h after the final administration.Citation54 PEGylated phosphatidylcholine-rich liposomal nanovesicles loaded with ciprofloxacin for ameliorating infectious pneumonia led to the eradication of intracellular MRSA with a 3.2-fold increase of pulmonary ciprofloxacin accumulation after IV injection of the lipid nanovesicles.Citation55 Inhaled liposomal ciprofloxacin NPs were developed to overcome the rapid clearance of antibiotics from the lungs. They successfully controlled the release of ciprofloxacin and showed enhanced antibacterial activity against P. aeruginosa supporting the potential of using inhaled liposomal ciprofloxacin for respiratory infections.Citation56 Current Phase-II clinical studies demonstrated a dual release profile (a rapid bactericidal effect followed by a sustained release from liposomes). The clinical study reports indicated that they are well tolerated with no serious adverse effects, and they significantly reduced pseudomonal bacterial load.Citation57
Enhanced localization of ciprofloxacin was achieved by actively loading it into photoactivatable liposomes that contain small amounts of porphyrin-phospholipid. High drug encapsulation and significant light-induced drug release was achieved which established the feasibility of remote loading antibiotics into photoactivatable liposomes promising for enhanced localized antibiotic therapy.Citation32 Ciprofloxacin efficiently inhibits the growth of M. avium in vitro in a murine macrophage-like cell line using negatively charged liposomes and in vivo using specific stealth liposomes in a mouse model of tuberculosis infection.Citation58 Ciprofloxacin-loaded liposomes coated with muco-adhesive biocompatible chitosan (chitosomes) resulted in a more pronounced effect against both Gram-positive and Gram-negative bacteria with a diffusional drug release mechanism. In addition, chitosan coating improved the ocular permeation of ciprofloxacin HCl where bacterial conjunctivitis symptoms were controlled by the prolonged release.Citation59
Polymeric micelles entrap hydrophilic and hydrophobic compounds and charged macromolecules through electrostatic, hydrophobic, and hydrogen bonding interactions.Citation60 The micellar ciprofloxacin treatment in P. aeruginosa infected mice showed on-demand release of ciprofloxacin with reduced bacterial load and alveolar injury.Citation61 In an investigation to enhance ocular delivery of ciprofloxacin, micellar formulations had been used to compare them with commercially available ciprofloxacin eye drops in rabbit’s eye. Pharmacokinetic parameters were determined and the investigated micellar formulations showed a significant improvement in the rate and extent of absorption with prolonged effect.Citation62 Zadeh et al also confirmed that polymeric micelles with proper particle sizes below 190 nm demonstrated improved gastro-intestinal solubility, intestinal membrane permeability, and loading efficiency.Citation63 A study on ciprofloxacin-loaded core-shell micellar nanospheres incorporated into a conventional, transparent contact lens provided a sustained, effective, and new drug delivery platform for treating ocular disorders. Nanosphere/ciprofloxacin incorporated into contact lenses () was tested for growth inhibition of S. aureus and P. aeruginosa and the result demonstrated that polymerized contact lenses were transparent and effective against the bacteria.Citation64
Figure 1 Micellar nanosphere encapsulated ciprofloxacin incorporated into contact lens
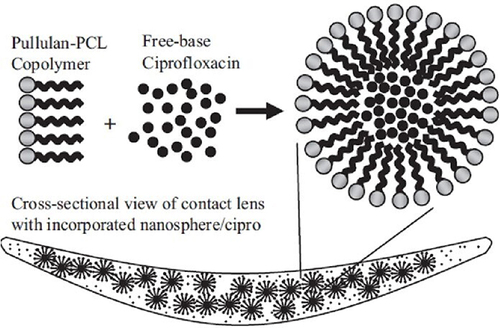
Other Polymer-Based Nanocarriers for Ciprofloxacin Delivery
Albumin, as a polymer abundant in the human body, has favorable properties such as biodegradability, biocompatibility, nontoxicity, low cost, and high ability to bind to a variety of drugs. Ciprofloxacin-loaded human serum albumin (HSA) NPs showed a significant antibacterial effect with greater susceptibility to bacteria which was resistant to all antibiotic groups with sustained release, increased serum half-life, reduced drug toxicity and side effects, and increased susceptibility or reduced resistance.Citation26 In another study, ciprofloxacin entrapped in an arginine-based nanocarrier system developed on a mesoporous silica NP (Arg-MSN) showed gradual drug release, two-fold higher in vitro antibacterial activity against Salmonella, targeting at the intra-vacuolar Salmonella, and localized delivery of the antibiotic. In addition, an increased reactive nitrogen species upon Arg-MSN treatment in the infected cells was observed. The coordinated effect of improved antibiotic delivery, intracellular targeting, and production of reactive nitrogen species resulted in an enhanced antibacterial activity (). This can be an attractive antibiotic carrier system for clearing intravacuolar infections.Citation27
Figure 2 Arginine grafted mesoporous silica nanoparticle targeting intracellular Salmonella.
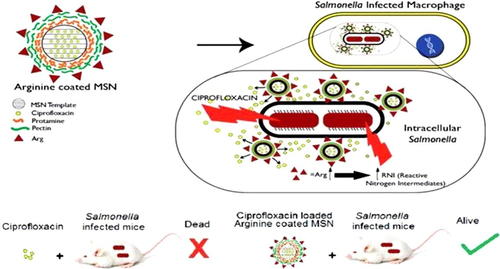
The antibacterial effect of ciprofloxacin was also enhanced by encapsulating the drug in carboxymethyl tamarind kernel polysaccharide (CMTKP). Ciprofloxacin-loaded CMTKP NPs revealed higher antibacterial activity and lowest cytotoxicity than ciprofloxacin alone.Citation30 Ciprofloxacin-loaded poly-isobutyl cyanoacrylate polymeric NPs demonstrated improved pharmacokinetic properties and augmented antibacterial activity against Mycobacterium avium.Citation65 Bioactive materials like hydroxyapatite (HA) in combination with antibiotics are widely developed for the treatment of osteomyelitis which play a dual role: as bone substitutes at the affected site and as local drug delivery systems for antibiotic delivery. The in situ loading of ciprofloxacin onto HA NPs provided sustained and prolonged release of ciprofloxacin with synergistic antibacterial activity against S. aureus and E. coli that causes osteomyelitis which can suggest the synthesized ciprofloxacin-loaded HA NPs as potential candidates for the treatment of osteomyelitis.Citation66 Use of ciprofloxacin during tissue engineering practices can be enhanced by applying it as ciprofloxacin-loaded polymeric NP incorporated electrospun fiber.Citation29
Ciprofloxacin-Conjugated Metallic Nanoparticles
NPs of silver (Ag), gold (Au), Zinc (Zn), copper (Cu), and inorganic carriers such as silica and alumina serve as novel carriers for pharmaceutical formulations. Their synergistic antibacterial activity with their bio-modification property with several functional groups such as amines, disulfides, and thiols is a considerable promising advantage.Citation67 Metallic NPs can tackle bacterial threats passively through prolonged drug retention at the specific site, or actively through surface conjugation with active molecules that can bind to a certain target.Citation10
Graphene oxide-coated Ag NPs demonstrated an effective encapsulation of ciprofloxacin with a controlled release and a synergistic antibiotic activity against selected bacterial strains of S. aureus, B. subtilis, E. coli, and P. aeruginosa.Citation68 In an investigation of Ag-ciprofloxacin nanoformulation against Pseudomonas keratitis, a synergistic antibacterial effect, decreased ciprofloxacin dose, and decreased genetic mutations for decreasing in the emergence of resistant strains were the main findings.Citation69 Functionalization with ciprofloxacin after a one year aging period of synthesized Ag NPs facilitated the penetration of Ag ions into the bacterial cell wall which resulted in a significant improvement of antibacterial activity against both Gram-positive and Gram-negative bacteria.Citation70 In another study, ciprofloxacin conjugated to biogenic Au NPs demonstrated enhanced bactericidal activity against Gram-negative and Gram-positive bacteria.Citation71 Ciprofloxacin, adsorbed in Au NPs, showed a characteristic release over an extended period of time.Citation72 Ciprofloxacin capping with metallic (Ag and Au) NPs under optimized conditions significantly implicated an improved solubility, pharmacokinetics, bioavailability, and a significant antibacterial activity.Citation73
Banoee et al investigated that the antibacterial activity of ciprofloxacin against S. aureus and E. coli increased in the presence of ZnO NPs. The study predicted that ZnO NPs may interfere with the efflux pumping activity which helps for restoring ciprofloxacin action by increasing its absorption into the cell.Citation74 In another similar study, only the low concentrations of ciprofloxacin-coated ZnO NPs (equivalent to the sub-minimum inhibitory concentrations of pure ciprofloxacin) demonstrated a considerably enhanced (4- to 32-fold) antibacterial activity. This result is of particular value, as using biocompatible ZnO NPs in combination therapy can lower amounts of antibiotics needed.Citation75 A review report by Allahverdiyev et al stated that ciprofloxacin with ZnO NPs showed increased inhibition zone areas against S. aureus and E. coli. The effect of ZnO is proposed to be interference with the pumping activity of NorA protein, possiblly binding on the nitogen, florine, and carboxilic ends of the ciprofloxacin structure ().Citation76
Figure 3 Ciprofloxacin-loaded lipid-core nanocapsules and their effects.Citation31
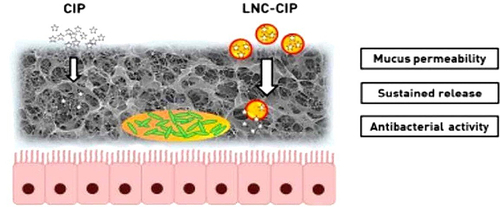
Metal oxide NPs are already applied as an antibacterial additive in various products. Nanostructured MgO is a low cost, easy to manipulate, and biocompatible metal oxide with intrinsic antibacterial activity. Mixed biphasic ZnMgO NPs showed a high antibacterial efficiency. Zn0.15Mg0.85O combined with ciprofloxacin potentiated antibiotic activity of ciprofloxacin against B. subtilis and E. coli.Citation77 Metal oxides with magnetic drug delivery properties, like iron oxide, improve the ability to define specific locations increasing the target and reducing the nontarget drug concentration. Ciprofloxacin-loaded CoFe2O4 led to magnetic targeting of bacteria, a controlled manner drug release, and a 2-fold increase in the inhibition zone.Citation68,Citation78 A study of ciprofloxacin-loaded CaCO3 NPs showed slower in vitro release of 12 h and a preserved antimicrobial effect against S. aureus after 2 days of incubation.Citation28
Ciprofloxacin-Loaded Solid Lipid Nanocarriers
Encapsulation and drug delivery with lipid nanocarriers prevents antibiotic drug therapies suffering from issues of over dosage, associated side effects, poor patient compliance, and development of resistances.Citation20 While poor membrane transport limits the potency of many antibiotics, drug-loaded NP vehicles can enter host cells via endocytosis. Besides, the carrying potentials of multiple drug combinations offer highly complex and unpredictable antimicrobial actions where the bacteria cannot develop resistance.Citation10,Citation79 The possible improvement in the antimicrobial therapy of ciprofloxacin-loaded lipid NPs is due to the enhanced intracellular delivery of the poorly-cell-penetrating antibiotics. The NPs are ingested by the phagocytic system, involve macrophage activation, and increase the immune response of the host.Citation80 Solid lipid nanoparticles (SLNPs) represent a superior alternative to other nanocarriers due to their high entrapment efficiency, ease of scale up, stability during storage, protection of labile drugs against degradation, improved tissue tolerance, less stringent regulatory requirements owing to the use of physiological lipids, and delivery by almost all routes.Citation81,Citation82
Ciprofloxacin-loaded SLNP formulations were subjected to an in vitro microbiological assay to verify the effect of nanoencapsulation on the cell growth inhibitory activity against S. aureus and P. aeruginosa. The result showed a more efficacious delivery inside bacterial cells and a higher stability of the encapsulated drug.Citation83 SLNPs are effective against skin infections as they tend to adhere to the skin surface and continuously release drugs over long periods of time in the stratum corneum. Ciprofloxacin-loaded SLNPs exhibit the prolonged release of drugs, especially in the skin and oral infections. A comparative study of ciprofloxacin-loaded protein, lipid, and chitosan NPs showed that SLNs were found to produce controlled release with the smallest size than others with the potential to act as long-acting antibiotic carriers in various regional and systemic infectious states.Citation84 Ghaffari et al demonstrated that ciprofloxacin release from SLNPs is more rapid with a significant burst effect followed by a sustained release that suggested that SLNPs can act as promising carriers for sustained ciprofloxacin release.Citation19
Ciprofloxacin release from SLNPs containing stearic acid displayed strongest burst effect with rapid release rate.Citation85 In an investigation for a solution to overcome biological (mucus) barrier, ciprofloxacin was loaded into lipid-core nanocapsules (LNC) for high mucus permeability, sustained release, and antibacterial activity. It resulted in 50% increase on drug permeation through mucus. High encapsulation efficiency, improved apparent aqueous solubility, reduced dosing frequency and overall dose, biofilm preventing, improved mucus permeation, and protection of the drug from deactivation were the major observations of the study. With these combined advantages, ciprofloxacin-loaded LNC represented a promising drug delivery system of an improved antibiotic therapy in cystic fibrosis.Citation31
Conclusion
Targeting ciprofloxacin more precisely to the site of infection by using carriers, an adjuvant approach whereby ciprofloxacin is paired with compounds that potentiate its activity against biofilm cells, combination of materials that act synergistically with ciprofloxacin against microbes, and protection of the drug from deactivation or efflux have been demonstrated to combat resistance and enhance antibacterial activity. Ciprofloxacin–NP conjugation, ciprofloxacin nanoencapsulation, loading ciprofloxacin in liposomes, and polymeric nanoformations are among the different promising nanotechnology-based approaches to combat ciprofloxacin resistance. Highly reduced MIC and dosing, reduced side effects, enhanced stability, targeting and controlling release, synergistic effect, optimized pharmacokinetic profile, and minimized chance of resistance have been demonstrated from the different ciprofloxacin nano-delivery studies. Especially, the synergistic activity, biodegradability, biocompatibility, higher stability, simple synthesis pathway, high loading efficiency, and cost-effectiveness of polymeric NPs are very promising and interesting for further investigations and alternative-setting approaches.
Abbreviations
AMR, antimicrobial resistance; CMTKP, carboxymethyl tamarind kernel polysaccharide; HA, hydroxyapatite; LNC, lipid-core nanocapsules; MDR, multidrug resistant; MIC, minimum inhibitory concentration; MRSA, methicillin-resistant S. aureus; MSSA, methicillin-susceptible S. aureus; NMs, nanomaterials; NPs, nanoparticles; PCL, poly(ε-caprolactone); PLGA, poly(lactic-co-glycolic) acid; SLN, solid lipid nanocarrier; SLNPs, solid lipid nanoparticles; UTI, urinary tract infections.
Disclosure
The authors have declared that there is no competing interest.
Acknowledgments
Addis Ababa University is thankfully acknowledged for giving us an internet access.
Additional information
Funding
References
- World Heath Organization. Bibliography of scientific publications on antimicrobial resistance from South-East Asia Region, Printed in India. © World Health Organization; 2011.
- Gashe F, Mulisa E, Mekonnen M, Zeleke G. Antimicrobial resistance profile of different clinical isolates against third-generation cephalosporins. J Pharmaceut. 2018;2018:1–7. doi:10.1155/2018/5070742
- Pham TDM, Ziora ZM, Blaskovich MAT. Quinolone Antibiotics. Med Chem Commun. 2019;10(10):1719–1739. doi:10.1039/C9MD00120D
- Sharma D, Patel RP, Zaidi STR, Sarker MMR, Lean QY, Ming LC. Interplay of the quality of ciprofloxacin and antibiotic resistance in developing countries. Front Pharmacol. 2017;8:546. doi:10.3389/fphar.2017.00546
- Vidyavathi M, Srividya G. A review on ciprofloxacin: dosage form perspective. Int J Appl Pharmaceut. 2018;10(4):6. doi:10.22159/ijap.2018v10i4.25315
- Reis ACC, Santos SRS, Souza SC, Saldanha MG, Pitanga TN, Oliveira RR. Ciprofloxacin resistance pattern among bacteria isolated from patients with community-acquired urinary tract infection. Rev Inst Med Trop Sao Paulo. 2016;58:53. doi:10.1590/S1678-9946201658053
- Mulder M, de Jong JCK, Goessens WHF, et al. Risk factors for resistance to ciprofloxacin in community-acquired urinary tract infections due to Escherichia coli in an elderly population. J Antimicrob Chemother. 2017;72:281–289. doi:10.1093/jac/dkw399
- Lee C-R, Cho I, Jeong B, Lee S. Strategies to minimize antibiotic resistance. Int J Environ Res Public Health. 2013;10(9):4274–4305.
- Aruguete DM, Kim B, Hochella MF, et al. Antimicrobial nanotechnology: its potential for the effective management of microbial drug resistance and implications for research needs in microbial nanotoxicology. Environ Sci Processes Impacts. 2013;15(1):93–102.
- Baptista PV, McCusker MP, Carvalho A, et al. Nano-strategies to fight multidrug resistant bacteria — “A Battle of the Titans”. Front Microbiol. 2018;9:1441.
- Rehman A, Patrick WM, Lamont IL. Mechanisms of ciprofloxacin resistance in Pseudomonas aeruginosa: new approaches to an old problem. J Med Microbiol. 2019;68(1):1–10. doi:10.1099/jmm.0.000873
- Scheld WM. Maintaining fluoroquinolone class efficacy: review of influencing factors. Emerg Infect Dis. 2003;9(1). doi:10.3201/eid0901.020277
- Ahmad I, Ahmad S, Rumbaugh KP, Editors. Antibacterial Drug Discovery to Combat MDR. Singapore: © Springer Nature Singapore Pte Ltd; 2019.
- Hamed SM, Elkhatib WF, El-Mahallawy HA, Helmy MM, Ashour MS, Aboshanab KMA. Multiple mechanisms contributing to ciprofloxacin resistance among gram negative bacteria causing infections to cancer patients. Sci Rep. 2018;8(1):12268. doi:10.1038/s41598-018-30756-4
- Campion JJ, McNamara JJ, Evans ME. Evolution of ciprofloxacin-resistant Staphylococcus aureus in in vitro pharmacokinetic environments. Antimicrob Agents Chemother. 2004;48(12):4733–4744. doi:10.1128/AAC.48.12.4733-4744.2004
- Cirz RT, Romesberg FE. Induction and inhibition of ciprofloxacin resistance-conferring mutations in hypermutator bacteria. Antimicrob Agents Chemother. 2006;50(1):220–225. doi:10.1128/AAC.50.1.220-225.2006
- Zhao F, Yang H, Bi D, Khaledi A, Qiao M. A systematic review and meta-analysis of antibiotic resistance patterns, and the correlation between biofilm formation with virulence factors in uropathogenic E. coli isolated from urinary tract infections. Microb Pathog. 2020;144:104196. doi:10.1016/j.micpath.2020.104196
- O’Regan E, Quinn T, Frye JG, et al. Fitness costs and stability of a high-level ciprofloxacin resistance phenotype in Salmonella enterica serotype Enteritidis: reduced infectivity associated with decreased expression of Salmonella pathogenicity Island 1 genes. Antimicrob Agents Chemother. 2010;54(1):367–374. doi:10.1128/AAC.00801-09
- Ghaffari S, Varshosaz J, Haririan I, Khoshayand MR, Azarmi S, Gazori T. Ciprofloxacin loaded Alginate/Chitosan and solid lipid nanoparticles, preparation, and characterization. J Dispers Sci Technol. 2012;33(5):685–689. doi:10.1080/01932691.2011.579831
- Kumar S, Bhanjana G, Kumar A, Taneja K, Dilbaghi N, Kim K-H. Synthesis and optimization of ceftriaxone-loaded solid lipid nanocarriers. Chem Phys Lipids. 2016;200:126–132. doi:10.1016/j.chemphyslip.2016.09.002
- Bali GK, Singla S, Kashyap Y, et al. Preparation, physico-chemical characterization and pharmacodynamics of ceftriaxone loaded BSA nanoparticles. J Nanomed Nanotechnol. 2018;9:509. doi:10.4172/2157-7439.1000509
- Jaison J, Barhoum A, Chan YS, Dufresne A, Danquah MK. Review on nanoparticles and nanostructured materials: history, sources, toxicity and regulations. Beilstein J Nanotechnol. 2018;9:1050–1074. doi:10.3762/bjnano.9.98
- Patel S, Singh D, Srivastava S, et al. Nanoparticles as a platform for antimicrobial drug delivery. Adv Pharmacol Pharm. 2017;5(3):31–43. doi:10.13189/app.2017.050301
- Sobhani Z, Samani SM, Montaseri H, Khezri E. Nanoparticles of chitosan loaded ciprofloxacin: fabrication and antimicrobial activity. Adv Pharm Bull. 2017;7(3):427–432. doi:10.15171/apb.2017.051
- Marei N, Elwahy AHM, Salah TA, El Sherif Y, El-Samie EA. Enhanced antibacterial activity of Egyptian local insects’ chitosan-based nanoparticles loaded with ciprofloxacin-HCl. Biomac. 2019;126:262–272.
- Shafiei S, Hassanshahian M, Shakeri S, Hamayeli H. Evaluation the antibacterial activity of nanoantibiotics imipenem and ciprofloxacin loaded in human serum albumin against some antibiotic-resistant pathogenic bacteria. J Exper Nanosci. 2020;15(1):350–362. doi:10.1080/17458080.2020.1796978
- Mudakavi RJ, Vanamali S, Chakravortty D, Raichur AM. Development of arginine based nanocarriers for targeting and treatment of intracellular Salmonella. RSC Adv. 2017;7(12):7022. doi:10.1039/C6RA27868J
- Dizaj SM, Lotfipour F, Barzegar-Jalali M, Zarrintan M, Adibkia K. Ciprofloxacin HCl-loaded calcium carbonate nanoparticles: preparation, solid state characterization, and evaluation of antimicrobial effect against. Artif Cells Nanomed Biotechnol. 2017;45(3):535–543. doi:10.3109/21691401.2016.1161637
- Günday C, Anand S, Gencer HB, et al. Ciprofloxacin-loaded polymeric nanoparticles incorporated electrospun fibers for drug delivery in tissue engineering applications. Drug Deliv Transl Res. 2020;10(3):706–720. doi:10.1007/s13346-020-00736-1
- Dilbaghi N, Kaur H, Ahuja M, Arora P, Kumar S. Synthesis and evaluation of ciprofloxacin-loaded carboxymethyl tamarind kernel polysaccharide nanoparticles. J Exper Nanosci. 2014;9(10):1015–1025. doi:10.1080/17458080.2013.771244
- Torge A, Wagner S, Chaves PS, et al. Ciprofloxacin-loaded lipid-core nanocapsules as mucus penetrating drug delivery system intended for the treatment of bacterial infections in cystic fibrosis. Int J Pharm. 2017;527(1–2):92–102. doi:10.1016/j.ijpharm.2017.05.013
- Ghosh S, Qi R, Carter KA, Zhang G, Pfeifer BA, Lovell JF. Loading and releasing ciprofloxacin in photoactivatable liposomes. Biochem Eng J. 2019;141:43–48. doi:10.1016/j.bej.2018.10.008
- Westmeier D, Siemer S, Vallet C, et al. Boosting nanotoxicity to combat multidrug-resistant bacteria in pathophysiological environments. Nanoscale Adv. 2020;2(11):5428–5440. doi:10.1039/D0NA00644K
- Siemer S, Westmeier D, Vallet C, et al. Resistance to nano-based antifungals is mediated by biomolecule coronas. ACS Appl Mater Interfaces. 2019;11(1):104–114. doi:10.1021/acsami.8b12175
- Niño-Martínez N, Orozco NFS, Martínez-Castañón G-A, Méndez FT, Ruiz F. Molecular mechanisms of bacterial resistance to metal and metal oxide nanoparticles. Int J Mol Sci. 2019;20(11):2808. doi:10.3390/ijms20112808
- Salas-Orozco M, Niño-Martínez N, Martínez-Castañón G-A, et al. Mechanisms of resistance to silver nanoparticles in endodontic bacteria: a literature review. J Nanomater. 2019;2019. doi:10.1155/2019/7630316
- Foulkes R, Man E, Thind J, Yeung S, Joy A, Hoskins C. The regulation of nanomaterials and nanomedicines for clinical application: current and future perspectives. Biomater Sci. 2020;8(17):4653–4664. doi:10.1039/D0BM00558D
- Manimekalai P, Manavalan R. Selection of excipients for the formulation of Ceftriaxone sodium loaded chitosan nanoparticle through drug-excipient compatibility testing. Int J PharmTech Res. 2015;8(1):5–10.
- Kucukoglu V, Uzuner H, Kenar H, Karadenizli A. In vitro antibacterial activity of ciprofloxacin loaded chitosan microparticles and their effects on human lung epithelial cells. Int J Pharm. 2019;569:118578. doi:10.1016/j.ijpharm.2019.118578
- Mahdavinia GR, Karimi MH, Soltaniniya M, Massoumi B. In vitro evaluation of sustained ciprofloxacin release from κ-carrageenan-crosslinked chitosan/hydroxyapatite hydrogel nanocomposites. Int J Biol Macromol. 2019;126:443–453. doi:10.1016/j.ijbiomac.2018.12.240
- Raveendran NT, Mohandas A, Menon RR, Menon AS, Biswas R, Jayakumar R. Ciprofloxacin and fluconazole containing fibrin nanoparticles incorporated chitosan bandages for the treatment of polymicrobial wound infections. ACS Appl Bio Mater. 2018;2(1):243–254.
- Gnanadhas DP, Thomas MB, Elango M, Raichur AM, Chakravortty D. Chitosan-dextran sulphate nanocapsule drug delivery system as an effective therapeutic against intraphagosomal pathogen Salmonella. J Antimicrob Chemother. 2013;68(11):2576–2586. doi:10.1093/jac/dkt252
- Kumar GV, Su CH, Velusamy P. Ciprofloxacin loaded genipin cross-linked chitosan/heparin nanoparticles for drug delivery application. Mater Lett. 2016;180:119–122. doi:10.1016/j.matlet.2016.05.108
- Buck E, Maisuria V, Tufenkji N, Cerruti M. Antibacterial properties of PLGA electrospun scaffolds containing ciprofloxacin incorporated by blending or physisorption. ACS Appl Bio Mater. 2018;1(3):627–635. doi:10.1021/acsabm.8b00112
- Türeli NG, Torge A, Juntke J, et al. Ciprofloxacin-loaded PLGA nanoparticles against cystic fibrosis P. aeruginosa lung infections. Eur J Pharmaceut Biopharmaceut. 2017;17:363–371.
- Türeli NG, Türeli AE, Schneider M. Optimization of ciprofloxacin complex loaded PLGA nanoparticles for pulmonary treatment of cystic fibrosis infections: design of experiments approach. Int J Pharm. 2016;515(1–2):343–351.
- Baelo A, Levato R, Julián E, Crespo A, Astola J, Gavaldà J. Disassembling bacterial extracellular matrix with DNase-coated nanoparticles to enhance antibiotic delivery in biofilm infections. J Control Release. 2015;209:150–158. doi:10.1016/j.jconrel.2015.04.028
- Thomas N, Thorn C, Richter K, Thierry B, Prestidge C. Efficacy of poly-lactic-co-glycolic acid micro- and nanoparticles of ciprofloxacin against bacterial biofilms. J Pharm Sci. 2016;105:1–8.
- Hadinoto K, Cheow WS. Nano-antibiotics in chronic lung infection therapy against Pseudomonas aeruginosa. Colloids Surf B Biointerfaces. 2014;116:772–785. doi:10.1016/j.colsurfb.2014.02.032
- Cipolla D, Wu H, Eastman S, Redelmeier R, Gonda I, Chan H. Development and characterization of an in vitro release assay for liposomal ciprofloxacin for inhalation. J Pharm Sci. 2014;103(1):314–327. doi:10.1002/jps.23795
- Ong HX, Benaouda F, Traini D, et al. In vitro and ex vivo methods predict the enhanced lung residence time of liposomal ciprofloxacin formulations for nebulization. Eur J Pharmaceut Biopharmaceut. 2014;86(1):83–89. doi:10.1016/j.ejpb.2013.06.024
- Page-Clisson ME, Pinto-Alphandary H, Chachaty E, Couvreur P, Andremont A. Drug targeting by polyalkylcyanoacrylate nanoparticles is not efficient against persistent Salmonella. Pharm Res. 1998;15(4):544–549. doi:10.1023/A:1011921608964
- Chono S, Tanino T, Seki T, Morimoto K. Efficient drug targeting to rat alveolar macrophages by pulmonary administration of ciprofloxacin incorporated into mannosylated liposomes for treatment of respiratory intracellular parasitic infections. J Control Release. 2008;127(1):50–58. doi:10.1016/j.jconrel.2007.12.011
- Magallanes M, Dijkstra J, Fierer J. Liposome-incorporated ciprofloxacin in treatment of murine salmonellosis. Antimicrob Agents Chemother. 1993;37(11):2293–2297. doi:10.1128/AAC.37.11.2293
- Hsu CY, Sung CT, Aljuffali IA, Chen CH, Hu KY, Fang JY. Intravenous anti-MRSA phosphatiosomes mediate enhanced affinity to pulmonary surfactants for effective treatment of infectious pneumonia. Nanomed Nanotechnol Biol Med. 2018;14(2):215–225. doi:10.1016/j.nano.2017.10.006
- Ong HX, Traini D, Cipolla D, et al. Liposomal nanoparticles control the uptake of ciprofloxacin across respiratory epithelia. Pharm Res. 2012;29:3335–3346.
- Antoniu SA. Inhaled ciprofloxacin for chronic airways infections caused by Pseudomonas aeruginosa. Expert Rev Anti Infect Ther. 2012;10(12):1439–1446. doi:10.1586/eri.12.136
- Yanagihara K. Design of anti-bacterial drug and anti-mycobacterial drug for drug delivery system. Curr Pharm Des. 2002;8(6):475–482. doi:10.2174/1381612023395808
- Mehanna MM, Elmaradny HA, Samaha MW. Mucoadhesive liposomes as ocular delivery system: physical, microbiological, and in vivo assessment Mucoadhesive liposomes in ocular delivery. Drug Dev Ind Pharm. 2010;36(1):108–118. doi:10.3109/03639040903099751
- Zhang J, Ma PX. Host-guest interaction mediated polymeric core-shell assemblies: versatile nanocarriers for drug delivery. Angew Chem Int Ed. 2009;48(5):964–968. doi:10.1002/anie.200804135
- Chen M, Xie S, Wei J, Song X, Ding Z, Li X. Antibacterial micelles with vancomycin-mediated targeting and pH/lipase-triggered release of antibiotics. ACS Appl Mater Interfaces. 2018;10(43):36814–36823. doi:10.1021/acsami.8b16092
- Taha EI, Badran MM, El-Anazi MH, Bayomi MA, El-Bagory IM. Role of pluronic F127 micelles in enhancing ocular delivery of ciprofloxacin. J Mol Liq. 2014;199(2014):251–256. doi:10.1016/j.molliq.2014.09.021
- Zadeh M, Sharif B, Esfahani G, Salimi Y. Permeability of ciprofloxacin-loaded polymeric micelles including ginsenoside as P-glycoprotein inhibitor through a Caco-2 cells monolayer as an intestinal absorption model. Molecules. 2018;23(8):1904. doi:10.3390/molecules23081904
- Garhwal R, Shady SF, Ellis EJ, et al. Sustained ocular delivery of ciprofloxacin using nanospheres and conventional contact lens materials. IOVS. 2012;53(3):1341–1352.
- Fawaz F, Bonini F, Maugein J, Lagueny AM. Ciprofloxacin-loaded polyisobutylcyanoacrylate nanoparticles: pharmacokinetics and in vitro antimicrobial activity. Int J Pharm. 1998;168(2):255–259. doi:10.1016/S0378-5173(98)00116-1
- Kumar GS, Govindan R, Girija EK. In situ synthesis, characterization and in vitro studies of ciprofloxacin loaded hydroxyapatite nanoparticles for the treatment of osteomyelitis. J Mater Chem B. 2014;2(31):5052–5060. doi:10.1039/c4tb00339j
- Panda BP, Patnaik S, Maharana RL. Current trends in design and development of nanopharmaceutical dosage forms. Bioequiv Bioavailab Int J. 2017;1(1):000104.
- Kooti M, Sedeh AN, Motamedi H, Rezatofighi SE. Magnetic graphene oxide inlaid with silver nanoparticles as antibacterial and drug delivery composite. Appl Microbiol Biotechnol. 2018;102(8):3607–3621. doi:10.1007/s00253-018-8880-1
- Zavarshani M, Ahmadi M, Saei HD, Tehrani AA, Naghadeh BD. Comparison therapeutic effects of ciprofloxacin, silver nanoparticles and their combination in the treatment of Pseudomonas keratitis in rabbit: an experimental study. Iran J Pharmaceut Res. 2019;18(1):320–327.
- Mohsen E, El-Borady OM, Mohamed MB, Fahim IS. Synthesis and characterization of ciprofloxacin loaded silver nanoparticles and investigation of their antibacterial effect. J Radiat Res Appl Sci. 2020;13(1):416–425.
- Sreedharan SM, Singh R. Ciprofloxacin functionalized biogenic gold nanoflowers as nanoantibiotics against pathogenic bacterial strains. Int J Nanomedicine. 2019;14:9905–9916.
- Tom RT, Suryanarayanan V, Reddy PG, Baskaran S, Pradeep T. Ciprofloxacin-protected gold nanoparticles. Langmuir. 2004;20:1909–1914.
- Nisar M, Khan SA, Qayum M, et al. Robust synthesis of ciprofloxacin-capped metallic nanoparticles and their urease inhibitory assay. Molecules. 2016;21:411.
- Banoee M, Seif S, Nazari ZE, et al. ZnO nanoparticles enhanced antibacterial activity of ciprofloxacin against Staphylococcus aureus and Escherichia coli. J Biomed Mater Res B Appl Biomater. 2010;93:557.
- Seif S, Kazempour ZB, Pourmand MR, et al. Preparation of ciprofloxacin-coated zinc oxide nanoparticles and their antibacterial effects against clinical isolates of Staphylococcus aureus and Escherichia coli. Arzneimittelforschung. 2011;61(8):472–476.
- Allahverdiyev AM, Kon KV, Abamor ES, Bagirova M, Rafailovich M. Coping with antibiotic resistance: combining nanoparticles with antibiotics and other antimicrobial agents. Expert Rev Anti Infect Ther. 2012;9(11):1035–1052.
- Auger S, Henry C, Péchoux C, et al. Exploring multiple effects of Zn0.15Mg0.85O nanoparticles on Bacillus subtilis and macrophages. Sci Rep. 2018;8:12276.
- Rodrigues GR, López-Abarrategui C, de la Serna Gómez I, Dias SC, Otero-González AJ, Franco OL. Antimicrobial magnetic nanoparticles based-therapies for controlling infectious diseases. Int J Pharm. 2018;555:356–367.
- El-Salamouni NS, Farid RM, El-Kamel AH, El-Gamal SS. Effect of sterilization on the physical stability of brimonidine-loaded solid lipid nanoparticles and nanostructured lipid carriers. Int J Pharm. 2015;496(2):976–983.
- Zaki NM, Hafez MM. Enhanced antibacterial effect of ceftriaxone sodium-loaded chitosan nanoparticles against intracellular Salmonella typhimurium. AAPS PharmSciTech. 2012;13(2):411–421.
- Mehnert W. Solid lipid nanoparticles production, characterization and applications. Adv Drug Deliv Rev. 2001;47(2–3):165–196.
- Qu J, Zhang L, Chen Z, et al. Nanostructured lipid carriers, solid lipid nanoparticles, and polymeric nanoparticles: which kind of drug delivery system is better for glioblastoma chemotherapy? Drug Deliver. 2016;23(9):3408–3416.
- Pignatello R, Leonardi A, Fuochi V, Petronio G, Greco AS, Furneri PM. A method for efficient loading of ciprofloxacin hydrochloride in cationic solid lipid nanoparticles: formulation and microbiological evaluation. Nanomater. 2018;8:304.
- Jain D, Banerjee R. Comparison of ciprofloxacin HCl-loaded protein, lipid, and chitosan nanoparticles for drug delivery. J Biomed Mater Res B Appl Biomater. 2008;86B(1):105–112.
- Shazly GA. Ciprofloxacin controlled-solid lipid nanoparticles: characterization, in vitro release, and antibacterial activity assessment. Biomed Res Int. 2017;2017:2120734.