Abstract
Introduction
Patients who need to be readmitted to the hospital because of complications from infections or require long-term care and rehabilitation face substantial financial hardships. To ensure the safety of patients undergoing surgery, it is crucial to implement measures that prevent wound infections before and after the procedure. Antibacterial wound dressings are essential to prevent infections during surgical procedures. There are various types of antibacterial wound dressings available on the market, such as silver-based dressings, hydrocolloid dressings, polyhexamethylene biguanide, alginate dressings, collagen-based dressings, and iodine-based dressings.
Methods
We used each type (standard, knit, fibril, and non-woven) of a commercial brand of oxidized regenerated cellulose (ORC) called Regecel to test bacterial growth. The choice of antibacterial wound dressing depends on the type of wound being treated. Different bacterial strains require specific culture conditions to thrive and grow in laboratory settings. To obtain accurate and reliable results, it is vital to follow the precise culture conditions required for each bacterial strain.
Results
The evaluation of ORC highlighted its potential to inhibit bacterial growth, showing promising results against various bacterial strains and Candida albicans. Different variants of ORC, such as Regecel, have demonstrated impressive capacity to hinder the growth of 32 distinct bacterial strains, with inhibition rates ranging from 40–100%. These bacteria include methicillin-resistant Staphylococcus aureus (MRSA), vancomycin-resistant Enterococcus (VRE), and penicillin-resistant Streptococcus pneumoniae.
Conclusion
This study supports the usage and development of ORC (Regecel) as an innovative approach to treating bacterial infections.
Introduction
Surgical site infections (SSIs) impose a significant global financial burden on healthcare systems. The expenses for managing, treating, and controlling such infections are considerably high, and can range from thousands to millions of dollars in extreme cases. The Centers for Disease Control and Prevention (CDC) estimates that SSIs result in an additional $3.5–10 billion in US healthcare costs annually,Citation1 with the average cost of an SSI ranging from $20,739–29,659 per patient.Citation2
Patients who require readmission to the hospital due to infection-related complications or long-term care and rehabilitation face even greater financial burdens. To ensure the safety of patients undergoing surgery, it is essential to implement pre- and post-surgical wound infection prevention measures. Pre-surgical steps should be taken to ensure that both the surgical site and the patient’s general health are in optimal condition to prevent potential post-operative infections.Citation3
Wound dressings play a crucial role in the prevention of infections during surgical procedures. Antibacterial wound dressings are medical products that protect against a wide range of bacterial species and can reduce the risk of infection in post-operative patients. Currently, several types of antibacterial wound dressings are available on the market, and each type offers different features and benefits. These include silver-based, hydrocolloid, polyhexamethylene biguanide, alginate, collagen-based, and iodine-based dressings. The type of antibacterial wound dressing used depended on the type of wound being treated. Certain types of antibacterial wound dressings may be more appropriate than others for specific wound types.Citation4,Citation5
Recent advancements in wound healing include bioprinting, which involves the development of various bioprinted devices designed to match wound sites and accelerate skin regeneration. This includes techniques, polymer-based inks, and AI-assisted bioprinting, showcasing the potential for significant progress in this field.Citation6 In addition, electrospun nanofibrous materials have been developed to address wound healing issue by incorporating antibiotics, antimicrobial peptides, metals, and antibacterial polymers to enhance antibacterial activity.Citation7 Further, wound healing involves a bioactive microneedle patch that exhibits dual antibacterial and reactive oxygen species scavenging capabilities. This innovative product, named GMCM, combines a base material of biocompatible methacrylated gelatin (GelMA) with an AI-derived antimicrobial peptide (AMP) assembled with hollow MnO2 nanoparticles. The GMCM patch, when combined with low temperature photothermal therapy, showed significant potential in accelerating chronic wound healing, outperforming the product control in wound healing evaluations.Citation8 Extra, the development of alternative biocides such as photothermal therapy (PTT) has emerged as a promising approach, utilizing hyperthermia generated by photothermal agents (PTAs) under laser irradiation. Inorganic–organic hybrid nanomaterials, combining inorganic and organic elements, offer potential in PTT antibacterial applications by integrating advantages of both components while mitigating their inherent limitations.Citation9 Another approach is using hydrogel, GCM, as wound dressing loaded with melanin nanoparticles in a polysaccharide matrix for efficient healing of bacterially infected diabetic wounds. The hydrogel forms a protective barrier over the wound, halting bleeding and neutralizing inflammatory cytokines. It facilitates a transition from the inflammatory process to the wound healing phase due to its photothermal antimicrobial and reactive oxygen species scavenging properties. The three-dimensional network structure of the hydrogel enhances cell proliferation, migration, and angiogenesis, making it suitable for treating various bacteria-infected diabetic wounds.Citation10
Absorbable antibacterial hemostatic wound dressings are crucial for preventing surgical infections. They offer numerous benefits, such as reducing bacterial contamination and promoting the healing of surgical wounds. Moreover, they help control bleeding and decrease the risk of complications associated with post-operative infections. Adjunctive hemostats are classified into two groups based on their modes of action. The first group accelerates the natural coagulation process of the subject by providing a matrix, whereas the second group contains active biological components, such as thrombin and/or fibrinogen, which allow hemostasis to be achieved, regardless of the coagulation status of the subject. Adjunct topical absorbable hemostats aid in the coagulation cascade. Oxidized cellulose, oxidized regenerated cellulose (ORC), gelatin, collagen, chitin, chitosan, and polysaccharides are examples of products in this category.Citation11,Citation12
ORC is a hemostatic wound dressing used in medical practice since the 1960s.Citation13 ORC is a chemically modified form of cellulose that is particularly useful for controlling diffuse bleeding from broad surfaces.Citation13 The features of ORC include hemostatic properties, absorbency, and biocompatibility which enhance wound healing and management of skin wounds, However, disadvantages of ORC are cost and market availability. The traditional dressing such as Gauze and 3M Bandages possess different types of features which include versatility, moisture retention and low cost. While disadvantages include adherence to wound, and limited hemostatic properties.Citation14 Depending on the clinical need, bleeding site, and intensity, different types of ORC (standard, knit, fibril, and non-woven) can be used. ORC exerts mechanical hemostatic effects by swelling owing to blood absorption and activates coagulation on the collagen surface. In addition, the ORC has caustic hemostatic properties owing to its low pH, which imparts antibacterial properties. This makes it the preferred choice for gelatin foam in contaminated areas.Citation15
This study aimed to investigate the effectiveness of a particular commercial brand of ORC, namely REGECEL® (The National Medical Products Co. Ltd., Saudi Arabia), in fighting antibiotic-resistant microbes and nosocomial pathogens. The antibacterial activity of this product was assessed in vitro to determine its effectiveness against certain disease-causing bacteria.
Materials and Methods
This work was performed using commercial bacterial strains and no ethical approval was required.
Strains
The following microorganisms used in this study were obtained from the American Type Culture Collection (ATCC, Manassas, VA, USA): Pseudomonas aeruginosa BAA-1744, Enterobacter cloacae (ATCC 13047), Enterococcus faecalis (ATCC 51299), Pseudomonas aeruginosa (ATCC 9027), Klebsiella pneumoniae (ATCC 13883), Staphylococcus saprophyticus (ATCC 49907), Micrococcus luteus (ATCC 49732), Corynebacterium striatum (ATCC BAA-1293), Pseudomonas aeruginosa (ATCC 27853), Escherichia coli (ATCC 35218), Salmonella enteritidis (ATCC 13076), methicillin-resistant Staphylococcus aureus (MRSA; ATCC 43300), Streptococcus pneumoniae (ATCC 49136), Haemophilus influenzae (ATCC 10211), Enterococcus faecalis (ATCC 25212), Streptococcus agalactiae (group B) 12386, Haemophilus influenzae (ATCC 9007), Candida albicans (ATCC 10231), Moraxella catarrhalis (ATCC 8176), Neisseria meningitidis (ATCC 13090), Moraxella catarrhalis (ATCC 25238), Proteus vulgaris (ATCC 8427), Staphylococcus aureus (ATCC 25923), Escherichia coli (ATCC 25922), Streptococcus pyogenes–group A (ATCC 19615), Shigella sonnei (ATCC 25931), Enterobacter aerogenes (13048), Klebsiella pneumoniae (700603), Staphylococcus aureus (ATCC 29213), Staphylococcus epidermidis (ATCC 12228), Clostridium perfringens (ATCC 13124), penicillin-resistant Streptococcus pneumoniae (PRSP; clinical isolate), and vancomycin-resistant Enterococcus (VRE; clinical isolate).
Procedures
This study was conducted at the Microbiology Laboratory of King Khalid University Hospital between January 2023 and March 2023. To assess the effectiveness of different product variants of ORC (REGECEL®: REF# 248–0331/1, 248–027/0, 248–014/0, and 248–045/4; The National Medical Products Co. Ltd)., microbial challenge tests were conducted on 33 strains of microorganisms (). The cultures of test organisms were grown on a specific medium and incubated for 24 h at 30–35°C, except for organisms that required a different medium or temperature. The plates were exposed to four types of ORC products; standard, knit, fibril, and non-woven.
Table 1 Inhibition Percentages and CFUs for ORC. The Table Presents the Inhibition and CFUs (Colony-Forming Units) for 33 Bacterial Strains, as Well as C. Albicans. (A) CTL; (B) ORCK; (C) ORCS; (D) ORCF; (E) ORCN
Each of the four types of products were cut and weighed to 215 mg before being placed into a sterile 15 mL tube. The tubes were inoculated with 11 mL of tryptone soya broth and incubated at room temperature for 4–6 h. The medium was then transferred to a sterile tube and 100 µL of 0.5 MacFarland challenge microorganisms was added. After vortexing for 2 min, a 10-fold serial dilution was prepared and a 1:1000 dilution was used. A total of 100 µL was then inoculated onto Mueller-Hinton agar and incubated at 30–35°C with 5% CO2 for 24 h. Blood, MacConkey, and chocolate agar were used as standard culture conditions when needed. This procedure was performed in triplicate at 24-h intervals after the initial inoculation. A control microorganism for all tested strains was run alongside the ORC challenge tests. The resulting plates were read and the results were expressed as colony-forming units (CFU)/mL. This method was based on previous studies.Citation16,Citation17
Anaerobic bacteria were incubated in an anaerobic jar using an anaerobic kit. For fastidious organisms, chocolate agar was used instead of Mueller-Hinton agar.
Statistical Analysis
The mean CFU and standard deviation (SD) for each organism/variant were calculated using triplicate data and compared statistically to the control triplicate data using ANOVA, which was performed using GraphPad Prism 7 (GraphPad Software, Inc., La Jolla, CA, USA). All experiments were independently repeated in triplicate. Quantitative graphs were generated using GraphPad Prism version 5.03 (GraphPad Software Inc). The Statistical significance was set at p < 0.05.
Results
The results of the experiments comparing the ORC variants (standard, knit, fibril, and non-woven) with different organisms are presented in . These results were analyzed to determine the statistical significance of each variant compared to the positive controls, with the respective tables showing the statistical difference and indicating whether an ORC variant significantly affected the growth of a given organism (). The results indicated that some ORC variants had greater inhibitory activity against certain organisms than others while still being similarly effective as the positive controls. These findings suggest that certain ORC variants may be more suitable for controlling specific organisms in specific applications. Supplementary Figures 1–55 show examples of the different levels of inhibition, including complete, partial, and resistant growth.
Figure 1 Effects of ORC exposure on 12 strains. Quantitative graphs of 12 bacterial strains exposed to ORC agents including standard, knit, fibril, and non-woven. The controls were non-treated strains. The significance (P value) was referred as * ≤ 0.05, ** ≤ 0.01, and *** ≤ 0.001.
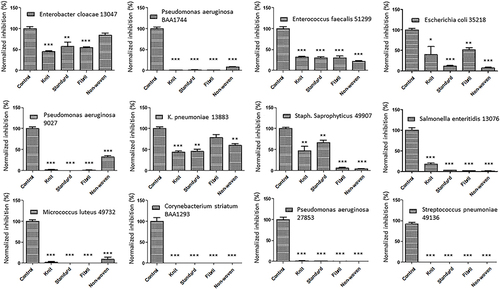
Figure 2 Effects of ORC exposure on 12 strains. Quantitative graphs of 12 bacterial strains exposed to ORC agents including standard, knit, fibril, and non-woven. The controls were non-treated strains. The significance (P value) was referred as ** ≤ 0.01, *** ≤ 0.001.
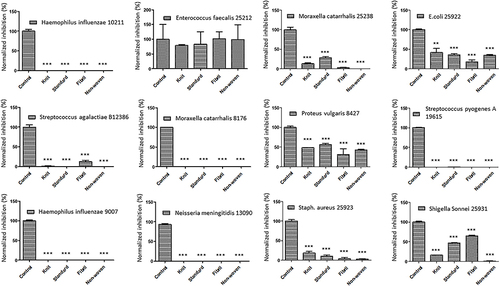
Figure 3 Effects of ORC exposure on 5 strains. Quantitative graphs of 5 bacterial strains exposed to ORC agents including standard, knit, fibril, and non-woven. The controls were non-treated strains. The significance (P value) was referred as * ≤ 0.05, ** ≤ 0.01, and *** ≤ 0.001.
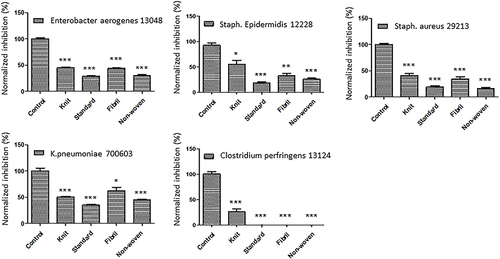
Figure 4 Effects of ORC exposure on four pathogens. Quantitative graphs of four major pathogens (MRSA, VRE, PRSP, and C. albicans) exposed to ORC agents including standard, knit, fibril, and non-woven. The controls were non-treated pathogens. The significance (P value) was referred as * ≤ 0.05, ** ≤ 0.01, and *** ≤ 0.001.
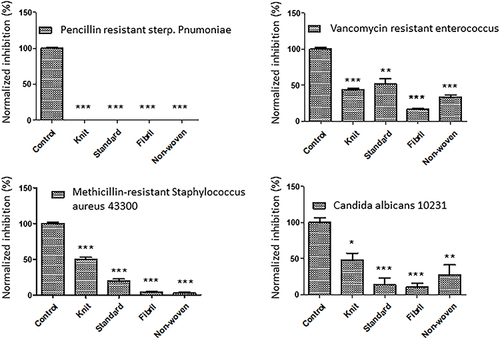
Different bacterial strains require specific culture conditions to grow and thrive in laboratory settings. For P. aeruginosa BAA1744, the use of nutrient or tryptic soy agar (TSA) incubated at 25–30°C are optimal conditions. K. pneumoniae 13883 can be grown on TSA at 35–37°C. C. striatum BAA1293 requires blood agar at 30–35°C with increased levels of CO2. H. influenzae 10211 can be grown on chocolate or blood agar supplemented with factors V (NAD) and X (hematin) at 35–37°C with increased levels of CO2. MRSA 43300 can be cultured on mannitol salt agar at 35–37°C. C. perfringens 13124 requires reinforced clostridial medium incubated at 35–37°C in anaerobic conditions. VRE and PRSP can be cultured on sheep blood or chocolate agar at 35–37°C with increased levels of CO2. It is essential to follow the specific culture conditions required for each bacterial strain to obtain accurate and reliable results.
Discussion
ORC variants showed remarkable ability to inhibit the growth of 32 different bacterial strains, ranging from 40–100% (). Thus, it is a highly effective antibacterial agent with broad-spectrum activity against a wide range of pathogens. The ability to inhibit bacterial growth at such a high rate is important for preventing the spread of infections caused by these bacteria. It is a promising drug with great potential for the treatment of bacterial infections. Further research is needed to determine its full range of capabilities and applications.
The 32 distinct bacterial strains mentioned, including Pseudomonas aeruginosa, Enterobacter cloacae, and MRSA, among others, are all significant in the context of wound healing. These bacteria are common pathogens that can infect wounds and delay the healing process. Their presence can lead to complications such as wound infection, sepsis, and even antibiotic resistance.Citation18–20
ORC variants have been shown to inhibit the growth of MRSA, VRE, PRSP, and C. albicans by 50–100% (). This is a significant finding because these strains are resistant to multiple antibiotics, making them difficult to treat. However, the ability of ORC to inhibit PRSP by 100% was even more impressive. These bacteria cause pneumonia, meningitis, and other serious infections, and penicillin-resistant strains are particularly difficult to treat. The ability of ORC to completely inhibit the growth of these bacteria suggests that it could be an effective treatment option for infections caused by these bacteria. Some studies have overridden blood brain barrier by encapsulating ORC drugs inside nanoparticles such as polycaprolactone bilayered Composite.Citation21 It worth noting that cellulose from ORC is a common biopolymer which can serve through encapsulation process as well.Citation22 These findings highlight the potential of ORC as a valuable tool in the fight against antibiotic-resistant bacterial infections.
In this study, ORC and its variants (standard, knit, fibril, and non-woven) were highly effective in the complete elimination of a wide range of pathogenic bacteria, including Haemophilus influenzae, Pseudomonas aeruginosa, Corynebacterium striatum, Neisseria meningitidis, Moraxella catarrhalis, Streptococcus pyogenes A, and PRSP (). These drugs have the unique ability to target biofilms, which are sticky matrices of bacteria that encase themselves in a protective layer to avoid destruction by antibiotics.
ORC drugs can penetrate biofilms and directly target bacterial cell walls, causing irreparable damage, and ultimately leading to complete bacterial elimination. Medical professionals worldwide have welcomed this groundbreaking solution because it provides a potential therapeutic approach for patients in whom traditional antibiotics have failed in the past. ORC drugs have the potential to revolutionize the treatment of bacterial infections, keeping us one step ahead in the battle against antibiotic-resistant bacteria.
Conclusion
The evaluation of ORC (Regecel) highlighted the potential of this drug to inhibit bacterial growth. The results indicated that 11 bacterial strains were almost completely inhibited in all variants (standard, knit, fibril, and non-woven), demonstrating the ability of ORC to effectively eliminate these strains. Additionally, 20 strains showed significant inhibition of all variants, further reinforcing the effectiveness of ORC in fighting bacterial infections. While only two strains showed non-significant inhibition in some of the variants, the overall results suggest that ORC has high potential for treating bacterial infections. Similarly, the ORC variants were superior against C. albicans growth. These findings support the development of ORC and encourage further research on the potential use of this drug in clinical settings. Overall, this study provides valuable insights into the potential of ORC as a new approach for treating bacterial infections. This approach requires validation through animal or in vivo studies to generate reliable preclinical outcomes.
Author Contributions
All authors made a significant contribution to the work reported, whether that is in the conception, study design, execution, acquisition of data, analysis and interpretation, or in all these areas; took part in drafting, revising or critically reviewing the article; gave final approval of the version to be published; have agreed on the journal to which the article has been submitted; and agree to be accountable for all aspects of the work.
Disclosure
The authors report no conflicts of interest in this work.
References
- Murphy LW. Preventing surgical site infections. AORN Journal. 2023;117(2):126–130. doi:10.1002/aorn.13868
- Ban K, Minei J, Laronga C, et al. American college of surgeons and surgical infection society: surgical site infection guidelines, 2016 update. J Am Coll Surgeons. 2016;224(1):59–74. doi:10.1016/j.jamcollsurg.2016.10.029
- Yao K, Bae L, Yew WP. Post-operative wound management. Aust Fam Physician. 2013;42(12):867–870.
- Zheng L, Li S, Luo J, Wang X. Latest advances on bacterial cellulose-based antibacterial materials as wound dressings. Front Bioeng Biotechnol. 2020;8:593768. doi:10.3389/fbioe.2020.593768
- Jones V, Grey JE, Harding KG. Wound dressings. BMJ. 2006;332(7544):777–780. doi:10.1136/bmj.332.7544.777
- Wang Z, Liang X, Wang G, Wang X, Chen Y. Emerging bioprinting for wound healing. Adv Mater. 2023;2304738. doi:10.1002/adma.202304738
- Wang Z, Hu W, Wang W, Xiao Y, Chen Y, Wang X. Antibacterial electrospun nanofibrous materials for wound healing. Advanced Fiber Mater. 2023;5(1):107–129. doi:10.1007/s42765-022-00223-x
- Wang G, Wang W, Chen Z, et al. Photothermal microneedle patch loaded with antimicrobial peptide/MnO2 hybrid nanoparticles for chronic wound healing. Chem Eng J. 2024;482:148938. doi:10.1016/j.cej.2024.148938
- Qi X, Xiang Y, Cai E, et al. Inorganic–organic hybrid nanomaterials for photothermal antibacterial therapy. Coord Chem Rev. 2023;496:215426. doi:10.1016/j.ccr.2023.215426
- Xiang Y, Qi X, Cai E, et al. Highly efficient bacteria-infected diabetic wound healing employing a melanin-reinforced biopolymer hydrogel. Chem Eng J. 2023;460:141852. doi:10.1016/j.cej.2023.141852
- Mohamad F, Alzahrani RR, Alsaadi A, et al. An explorative review on advanced approaches to overcome bacterial resistance by curbing bacterial biofilm formation. Infect Drug Resist. 2023;16:19–49. doi:10.2147/IDR.S380883
- Cziperle DJ. Avitene™ microfibrillar collagen hemostat for adjunctive hemostasis in surgical procedures: a systematic literature review. Med Devices. 2021;Volume 14:155–163. doi:10.2147/MDER.S298207
- Yu P, Zhong W. Hemostatic materials in wound care. Burns Trauma. 2021;9. doi:10.1093/burnst/tkab019
- Dhivya S, Padma VV, Santhini E. Wound dressings - a review. BioMedicine. 2015;5(4):22. doi:10.7603/s40681-015-0022-9
- Duenas-Garcia OF, Goldberg JM. Topical hemostatic agents in gynecologic surgery. Obstetrical Gynecol Surv. 2008;63(6):389–394. doi:10.1097/OGX.0b013e31816ff805
- Dineen P. The effect of oxidized regenerated cellulose on experimental infected splenotomies. J Surg Res. 1977;23(2):114–116. doi:10.1016/0022-4804(77)90198-6
- Moťková P, Brožková I, Vytřasová J, Kukla R. Antimicrobial effect of OKCEL® H-D prepared from oxidized cellulose. Folia Microbiologica. 2018;63(1):57–62. doi:10.1007/s12223-017-0534-7
- Bessa LJ, Fazii P, Di Giulio M, Cellini L. Bacterial isolates from infected wounds and their antibiotic susceptibility pattern: some remarks about wound infection. Int Wound J. 2015;12(1):47–52. doi:10.1111/iwj.12049
- Hariharan H, Matthew V, Fountain J, et al. Aerobic bacteria from mucous membranes, ear canals, and skin wounds of feral cats in Grenada, and the antimicrobial drug susceptibility of major isolates. Comp Immunol Microbiol Infect Dis. 2011;34(2):129–134. doi:10.1016/j.cimid.2010.05.001
- Malani PN, McNeil SA, Bradley SF, Kauffman CA. Candida albicans sternal wound infections: a chronic and recurrent complication of median sternotomy. Clinl Infect Dis. 2002;35(11):1316–1320. doi:10.1086/344192
- Sanpakitwattana A, Suvannapruk W, Chumnanvej S, Hemstapat R, Suwanprateeb J. Cefazolin loaded oxidized regenerated cellulose/polycaprolactone bilayered composite for use as potential antibacterial dural substitute. Polymers. 2022;14(20):4449. doi:10.3390/polym14204449
- Zamel D, Khan AU, Khan AN, et al. Regenerated Cellulose and Composites for Biomedical Applications. In: Regenerated Cellulose and Composites: Morphology-Property Relationship. Springer; 2023:265–311.