Abstract
Introduction
Candida is the primary cause of invasive fungal disease, candidiasis, especially in developed nations. The increasing resistance observed in multiple antibiotics, coupled with the prolonged process of creating new antibiotics from the ground up, emphasizes the urgent requirement for innovative methods and new compounds to combat Candida infections. Employing a treatment strategy that combines antibiotics can improve efficacy, broaden the spectrum of targeted fungal, and reduce the chances of resistance emergence. This approach shows potential in tackling the escalating problem of antibiotic resistance. The objective of this research is to explore the potential synergistic effects of combining 3-hydrazinoquinoxaline-2-thiol and thymoquinone against a variety of Candida isolates. This investigation aims to offer an understanding of the collective antimicrobial action of these compounds.
Methods
Broth microdilution was utilized to assess the Minimum Inhibitory Concentrations (MICs) of 3-hydrazinoquinoxaline-2-thiol and thymoquinone for 22 clinical Candida isolates. Following this, a checkerboard assay was employed to analyze the interaction between 3-hydrazinoquinoxaline-2-thiol and thymoquinone, with a specific focus on the Fractional Inhibitory Concentration Index (FICI).
Results
The MICs of thymoquinone and 3-hydrazinoquinoxaline-2-thiol were determined for 22 clinical Candida strains, with thymoquinone exhibiting MICs ranging from 64 to 8 µg/mL, and 3-hydrazinoquinoxaline-2-thiol displaying MICs varying from 64 to 8 µg/mL. Notably, the combination of 3-hydrazinoquinoxaline-2-thiol and thymoquinone resulted in a synergistic effect, leading to a significant reduction in MICs, with reductions of up to 64-fold with FICI below 0.5 against tested strains.
Conclusion
The prospect of using 3-hydrazinoquinoxaline-2-thiol in combination with thymoquinone as an effective solution against Candida looks encouraging. Nevertheless, to validate its practical applicability, additional comprehensive testing and experiments are imperative.
Introduction
Fungi have become significant contributors to human diseases, particularly affecting the immunocompromised and individuals hospitalized with severe underlying conditions. The associated morbidity and mortality are considerable, highlighting the emergence of fungal diseases as crucial public health concerns.Citation1–3 Analyzing the patterns of Infectious disease mortality rates US, revealed a notable rise in mortality rate attributed to mycoses. Most of these mycosis deaths were linked to infections caused by Aspergillus, Cryptococcus and Candida species.Citation2 Fungal pathogens contribute to a minimum of Thirteen million infections and one and a half million fatalities worldwide annually, predominantly affecting individuals with compromised immune function.Citation4 Earlier records indicate that fungal infections led to almost seventy five thousand hospitalizations and an additional almost nine million outpatient visits in 2014. This resulted in healthcare costs of at least $7 billion.Citation5
Presently, the clinical use of antifungals is confined to four main classes: azoles, polyenes, echinocandins, and pyrimidine analogs. This limited array of antifungals raises concerns about the efficacy of treatments and raises the risk of fatal outcomes when the infecting organism develops resistance to 1 or more drugs. Diagnostic constraints exacerbate the issue, as various species may exhibit diverse resistance profiles. The rise of in the number of resistant strains, this includes strains that are resistant to several drugs, has been progressively documented in recent decades.Citation6–9 Apart from concerns regarding drug resistance, polyenes are recognized for their significant toxicity and possess a narrow therapeutic range. Both polyenes and echinocandins encounter constraints in terms of oral bioavailability, confining their application to intravenous administration and requiring prolonged hospitalization. Additionally, extended-spectrum triazoles, like posaconazole, encounter difficulties associated with variable bioavailability, immediate adverse reactions, and the emergence of resistance, collectively imposing limitations on their effective utilization.Citation10–12 While polyene has been proposed for topical use, its efficacy in treating fungal infections within the eye is hindered by its limited capacity to penetrate ocular tissues.Citation13 The challenge of drug resistance is exacerbated by the sluggish pace of drug discovery, which is impeded by factors such as high costs, stringent regulatory restrictions, and the rapid evolution of resistance mechanisms.Citation14 Therefore, it is crucial to investigate novel strategies in addressing antimicrobial resistance, and one of these approaches involves the use of antibiotic combination therapy.Citation14
Antibiotic combination therapy provides numerous advantages, including optimizing drug delivery to infection sites, improving bacterial clearance through the targeting of diverse mechanisms, preventing the emergence of resistance, and inhibiting toxin synthesis, ultimately reducing bacterial virulence.Citation15,Citation16 A highly successful antibiotic combination involves pairing a β-lactam antibiotic with β-lactamase blocker called clavulanic acid being a notable example. Clavulanic acid binds tightly to various bacterial β-lactamases, safeguarding the antibiotic from degradation. This combination, commercially known as Augmentin, enables the use of amoxicillin in treating bacterial infections resistant to β-lactam antibiotics.Citation17 Additionally, study has indicated that amphiphilic tobramycin and fluconazole exhibit a synergistic antifungal effect against various strains of C. albicans.Citation18 This motivates us to investigate the synergistic potential of combining antimicrobial drugs to enhance efficacy against drug-resistant Candida isolates.
Thymoquinone is a naturally occurring bioactive substance present in the seed of Nigella sativa, popularly recognized as black cumin or black seed.Citation19 Thymoquinone is renowned for its antioxidative and anti-inflammatory, anticancer and antibacterial activity.Citation20,Citation21 Thymoquinone has demonstrated antibacterial efficacy and has exhibited notable antifungal properties against Aspergillus niger and Candida albicans.Citation21 Research findings have unequivocally illustrated that derivatives of quinoxaline manifest an extensive and diverse range of antifungal effects.Citation22 Based on this assumption, we suggest that these derivatives of quinoxaline (3-hydrazinoquinoxaline-2-thiol) possess the capability to synergize with thymoquinone in combating various Candida species, with the inclusion of Candida albicans, Candida parapsilosis, Candida tropicalis, Candida krusei, and Candida glabrata particularly when utilized in combination therapy against a spectrum of clinical Candida strains. By building upon previous research findings, this approach introduces a promising strategy to tackle the complexities associated with Candida infections, potentially opening avenues for more efficacious treatment alternatives.
The primary objective for this investigation will be to assess the in vitro antimicrobial effectiveness of 3-hydrazinoquinoxaline-2-thiol in combination with thymoquinone across a diverse set of clinical Candida species. This study aims to elucidate the possible synergistic interaction among the 2 compounds and its influence on treatment efficacy. By doing so, it seeks to enhance our comprehension of varied strategies for addressing Candida infections.
Methods
Study Strain and Culture Condition
In this study, 22 Candida species were examined including Candida albicans (n= 14), Candida glabrata (n= 2), Candida tropicalis (n= 3), Candida krusei (n=2), Candida parapsilosis (n=1) from King Abdulaziz University Hospital of King Abdulaziz University in Jeddah, Saudi Arabia. These isolates were preserved in glycerol and stored at −80°C. Prior to testing, all isolates were cultured on blood agar (supplied by HiMedia, India). Cultivation took place overnight at 37°C under aerobic conditions. The identification and susceptibility testing of all isolates was carried out with the Vitek 2 system (bioMerieux, France) with the Candida card type AST-YS07, following the manufacturer’s guidelines. The Candida isolates utilized in our research were obtained as part of routine hospital procedures for microbial isolation. These isolates were collected solely for diagnostic purposes and were not associated with patient information such as age, risk factors, comorbidities, or nationality. As such, they were anonymized and did not require individual patient consent or ethical approval for their use in laboratory research.
Antimicrobial Agents
The drugs tested in this study, which specifically target Candida, included a 3-hydrazinoquinoxaline-2-thiol agent obtained through (Fluorochem Ltd., UK) and thymoquinone powder from Sigma. Both compounds were dissolved in the solvent dimethyl sulfoxide (DMSO). The original stock formula for both drugs is 10 mg/mL.
Broth Microdilution Assay
A broth microdilution test is carried out to determine antimicrobial susceptibility. A double serial dilution of the antimicrobials was prepared for this purpose and tested in Mueller Hinton Broth sourced from (Sigma-Aldrich, US). Then 100 µL of the previously prepared antimicrobial solution was added to each well of the 96-well flat plates (Italy). The density of the suspended inoculum was carefully calibrated to 0.5 McFarland with the aid of a Biosan densitometer DEN-1B suspension turbidity detector. Subsequently, 5 µL of the inoculum prepared was added to each well containing different concentrations of antimicrobial drugs. The 96 well plates were incubated overnight at 37°C. Antimicrobial sensitivity tests were performed in triplicate and the average values were calculated and recorded for further analysis. The MIC is defined as the minimum concentration of active agent that can suppress the detected level of growth of a pathogen. The MIC findings for the two antimicrobial drugs were determined using the broth microdilution method and analysed according to the guidelines of the Clinical and Laboratory Standards Institute (CLSI).Citation23–26
Checkerboard Assay
To evaluate the interactions between the antimicrobial agents, we used the checkerboard broth assay. In this procedure, a two-fold in-series dilution was made for each compound utilising MHB, and fifty μL of this dilution was added to 96-well flat plates (Italy Inc). The inoculum suspension density was accurately set to 0.5 McFarland by using a Biosan Densitometer DEN-1B suspension turbidimeter. Then 5 μL of the precipitated isolates were added carefully.Citation27
When determining the combination of compounds 3-hydrazinoquinoxaline-2-thiol and thymoquinone, the Fractional Inhibitory Concentration (FIC) was determined based on the formula FIC = MIC (3-hydrazinoquinoxaline-2-thiol in combination) / MIC (3-hydrazinoquinoxaline-2-thiol alone) + MIC (thymoquinone in combination) / MIC (thymoquinone alone). This calculation compares the MIC of the individual compounds when used in combination with those when used individually, which enables a comprehensive evaluation of the combined inhibitory effect.Citation28 The checkerboard test was performed in triplicate and the mean value were recorded.
Evaluation of Interactions of the Antimicrobial Agents
Conducting combination studies in a checkerboard experiment, the test mentioned above was employed for generating a matrix encompassing most of potential two drugs MICs within the specified concentration scope. The interaction between the two antimicrobials agents was defined quantitatively using the fractional inhibitory concentration index (FICI), estimated according to the following equation: FICI = [(MIC A in combination)/MIC A] + [(MIC B in combination)/MIC B].
The FICI results were evaluated as follows: less than or equal to 0.5 indicated synergy; > 0.5 but ≤ 1 signified an additive effect; > 1 but ≤ 2 suggested indifferences and values exceeding 2 indicated antagonism. In practical terms, synergy, as calculated in this manner, equates to a reduction of at minimum two dilution steps at the MIC of each agent when used in combination.Citation29
Result
The Assessment of the MICs for Both Thymoquinone and 3-Hydrazinoquinoxaline-2-Thiol
It is of utmost importance to ascertain the MICs of both thymoquinone and 3-hydrazinoquinoxaline-2-thiol prior to embarking on the checkerboard method. The MIC values for thymoquinone, delineated in , span from 8 to 64 µg/mL. Additionally, the MIC values for 3-hydrazinoquinoxaline-2-thiol, outlined in , vary from 8 to 64 µg/mL. With all this MIC data in hand, we carefully designed a checkerboard test where we mixed different amounts of thymoquinone and 3-hydrazinoquinoxaline-2-thiol. This intricate experimental design aims to scrutinize the potential synergistic or additive effects of the antibiotics against the prevailing resistant Candida strains. The insights gleaned from this assay will play a pivotal role in unravelling the intricate interactions between these agents when confronted with various clinical Candida species.
Table 1 MICs of Thymoquinone and 3-Hydrazinoquinoxaline-2-Thiol in μg/mL for Variety of Clinical Candida Species
3-Hydrazinoquinoxaline-2-Thiol Synergises Thymoquinone Against Different Candida Clinical Strains
A checkerboard test was conducted in order to assess the combination effects of 3-hydrazinoquinoxaline-2-thiol and thymoquinone on different Candida clinical strains. Thymoquinone, when added alone, proved ineffective in inhibiting Candida growth. However, a notable observation was noticed when thymoquinone was paired with 3-hydrazinoquinoxaline-2-thiol, the MICs of thymoquinone significantly reduced, reaching reductions up to 64 folds in certain strains. Likewise, the MICs of 3-hydrazinoquinoxaline-2-thiol demonstrated a significant decrease when it was combined with thymoquinone, with reductions of up to 64-fold in some strains. Moreover, we observed a reduction in Minimum Inhibitory Concentrations (MICs) by 4-fold and 16-fold against specific Candida strains when the combination of thymoquinone and 3-hydrazinoquinoxaline-2-thiol was employed, as noticed by the substantial decrease in MICs observed in our study ().
Figure 1 (A) Minimum inhibitory concentration (MIC) of 3-hydrazinoquinoxaline-2-thiol was 32 µg/mL and it was reduced to 8 µg/mL (4 fold) when it was combined with thymoquinone against C. albicans 2 (B) MIC of thymoquinone was 16 µg/mL and it was reduced to 1 µg/mL (16 fold) when it was combined with 3-hydrazinoquinoxaline-2-thiol against Candida 2. T represents thymoquinone and Q represents 3-hydrazinoquinoxaline-2-thiol. The experiment was performed in triplicate, and the average value was calculated.
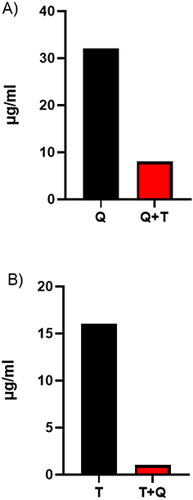
Even more noteworthy is the synergistic interaction observed in this combination against 22 diverse clinical Candida strains, indicated by consistently low FICI values less than 0.5 (). This compellingly indicates that 3-hydrazinoquinoxaline-2-thiol effectively enhances thymoquinone in the fight against Candida strains, presenting a promising path for enhanced therapy approaches ().
Table 2 The Interaction of 3-Hydrazinoquinoxaline-2-Thiol with Thymoquinone Against Diverse Candida Strains in vitro. Synergy is Considered When the Fractional Inhibitory Concentration Index (FICI) Falls Below 0.5
Discussion
Candida is a major cause of invasive fungal disease, candidiasis, especially in developed nations. It affects 2 to 14 people per 100,000 population and leads to over 250,000 cases of candidemia annually, resulting in around 50,000 deaths worldwide.Citation9,Citation30–32 This study demonstrates the combined effectiveness of thymoquinone and 3-hydrazinoquinoxaline-2-thiol derivatives against various Candida species. Each Candida strain showed different responses to these compounds. These findings not only enhance our understanding of Candida treatment but also suggest a strategy to combat antimicrobial resistance.
This study found that combining thymoquinone with 3-hydrazinoquinoxaline-2-thiol significantly reduced MICs, with up to a 64-fold decrease observed. The synergistic effect was consistent across various clinical Candida species, indicating a stronger antimicrobial effect compared to using each drug alone. Our study aligns with the findings of Dera et al 2021, which showed the efficacy of thymoquinone in synergy with gentamicin against Gram-positive Staphylococcus epidermidis (ATCC 12228).Citation33 Their investigation into the synergistic combination of thymoquinone supports our own research focused on thymoquinone with 3-hydrazinoquinoxaline-2-thiol against Candida strains. The observed synergism between thymoquinone and gentamicin emphasizes the potential broad-spectrum antimicrobial properties of thymoquinone and highlights its relevance in combating various bacterial strains, including those of Gram-positive bacteria.Citation33
The inclusion of a second antibiotic in the treatment protocol has the potential to override the limitations of the first antibiotic, as previously suggested.Citation34 Our research supports this concept and provides compelling evidence. When administered alone, thymoquinone failed to inhibit Candida growth at a lower MIC. However, when combined with 3-hydrazinoquinoxaline-2-thiol, it showed a novel ability to inhibit Candida growth at a lower MIC. This result is attributed to the enhanced interaction of thymoquinone and 3-hydrazinoquinoxaline-2-thiol due to synergy, and underscores the efficacy of combination therapy in managing the problems associated with candida infections.
Considering the toxicity potential related to very high concentrations thymoquinone and othanyer drug, using decreased concentration of each medicine in a synergistic way is proving to be a potential way to decrease risk of adverse effects.Citation35 This research may support this idea: while thymoquinone required 64 µg/mL to inhibit some Candida strains growth when used in isolation, a remarkable discovery was shown when combined with 3-hydrazinochinoxaline-2-thiol. In this combined treatment, only 4 µg/mL of thymoquinone per millilitre proved to be sufficient to inhibit the growth of the same Candida strain. This observation suggests that a comparable therapeutic effect may be reached with lower medicine concentration, potentially minimizing the risk of adverse effects. Nevertheless, further studies are urgently needed to validate and extend this promising result. Moreover, as thymoquinone efficient versus both Gram-positive and Gram-negative bacteria,Citation36,Citation37 its potential synergy with 3-hydrazinoquinoxaline-2-thiol goes beyond Candida and offers a wider scope of activities. The advantage of combination therapy is that it has a broader spectrum of activity against different organisms, thus extending its overall spectrum.Citation38 We believe that the antimicrobial combination therapy may provide a more extensive spectrum of coverage, but further tests are needed.
Our study showed that 3-hydrazinoquinoxaline-2-thiol can act synergistically with thymoquinone in the control of various clinical strains of Candida species, including Candida albicans, Candida parapsilosis, Candida glabrata, Candida krusei and Candida tropicalis. The observed enhanced effect among 3-hydrazinoxaline-2-thiol and thymoquinone seems to have been based on several mechanisms involving the inhibition of different signaling pathways. First and primarily thymoquinone exerts its antimicrobial effect against bacteria by causing irreversible damage to bacterial morphology, compromising cell membrane integrity, causing protein leakage, and disrupting intracellular proteins.Citation36 In the second place, 3-hydrazinoquinoxaline-2-thiol suppressed the synthesis of DNA.Citation39 Therefore, we posit that the synergistic outcome arises from concurrently targeting Candida through distinct pathways.
An possible alternative reason for the increased efficacy of the combination of thymoquinone and 3-hydrazinoquinoxaline-2-thiol versus several Candida species could be the higher generation of reactive oxygen species (ROS). These ROS disrupt cellular electron transport. This disruption leads to persistent oxidative stress, which leads to cell death. There is ample evidence that thymoquinone may stimulate the formation of ROS, that playing a decisive role in cell eradication of organism.Citation40 It is worth noting that, 3-hydrazinoquinoxaline-2-thiol is well recognised for its ability to generate reactive oxygen species.Citation41 On the basis of this, this study hypothesizes that the noticed synergy among thymoquinone and 3-hydrazinoquinoxalin-2-thiol could be related to overproduction of ROS. But, more tests and experiments are imperative to substantiate this hypothesis. More detailed studies are necessary to assess the underlying mechanisms of the interaction between 3-hydrazinoquinoxalin-2-thiol and thymoquinone.
Further investigations are essential to enhance our comprehension of the synergistic interplay among thymoquinone and 3-hydrazinoquinoxaline-2-thiol. This necessitates a multiple of important tests. Initially, a Time Kill assay is required to accurately assess the bactericidal effect of the proposed combination, ain further insight into its potential as a treatment strategy through time.Citation27 Additionally, a resistance test is crucial to evaluate if the Candida able to develop resistance against the combination of thymoquinone and 3-hydrazinoquinoxaline-2-thiol combination, ensuring its long-term effectiveness.Citation35,Citation42 The incorporation of an in vivo model is also important to evaluate the effect of the combination in a living organism, providing valuable information on its response and its interaction with Candida inside the body.Citation43,Citation44 Furthermore, a mechanistic study is required to delve into the underlying mechanisms of how these compounds work together against various clinical Candida strains, facilitating the development of additional effective combinations.
Conclusion
In summary, this research shows for the first evidence of the synergistic action among 3-hydrazinoquinoxaline-2-thiol and thymoquinone versus various Candida strains. The results suggest potential clinical application, but further research and trials are needed to optimize dosing, ensure safety, and assess efficacy in real-world settings.
Abbreviations
US, United States, MIC, minimum inhibitory concentration, FIC, Fractional inhibitory concentration, FICI, Fractional inhibitory concentration index, ROS, Reactive oxygen species, MHB, Muller-Hinton broth, C. albicans, Candida albicans, C. glabrata, Candida glabrata, C. parapsilosis, Candida parapsilosis, C. tropicalis, Candida tropicalis, C. krusei Candida krusei.
Disclosure
The authors of this scientific paper explicitly state that they have no discernible competing financial interests or personal affiliations that could be construed as having an impact on the findings presented in this manuscript. This transparent disclosure is offered to uphold the integrity and credibility of the scientific research documented herein.
Additional information
Funding
References
- Blumberg HM, Jarvis W, Soucie J, et al. Risk factors for candidal bloodstream infections in surgical intensive care unit patients: the NEMIS prospective multicenter study. Clin Infect Dis. 2001;33:177–186. doi:10.1086/321811
- Pfaller MA, Diekema DJ. Epidemiology of invasive candidiasis: a persistent public health problem. Clin Microbiol Rev. 2007;20:133–163. doi:10.1128/CMR.00029-06
- Rayens E, Norris KA, Cordero JCDSF. Mortality trends in risk conditions and invasive mycotic disease in the United States, 1999–2018. Clin Infect Dis. 2022;74:309–318. doi:10.1093/cid/ciab336
- Rayens E, Norris KA. Prevalence and healthcare burden of fungal infections in the United States, 2018. Open Forum Infect Dis. 2022;9. doi:10.1093/ofid/ofab593
- Benedict K, Jackson BR, Chiller T, B KD. Estimation of direct healthcare costs of fungal diseases in the United States. Clin Infect Dis. 2019;68:1791–1797. doi:10.1093/cid/ciy776
- Pfaller MA, Andes DR, Diekema DJ, et al. Epidemiology and outcomes of invasive candidiasis due to non-albicans species of Candida in 2496 patients: data from the prospective antifungal therapy (PATH) registry 2004–2008. PLoS One. 2014;9:e101510. doi:10.1371/journal.pone.0101510
- Pfaller MA, Messer SA, Woosley LN, Jones RN, Castanheira M. Echinocandin and triazole antifungal susceptibility profiles for clinical opportunistic yeast and mold isolates collected from 2010 to 2011: application of new CLSI clinical breakpoints and epidemiological cutoff values for characterization of geographic. J Clin Microbiol. 2013;51:2571–2581. doi:10.1128/JCM.00308-13
- Lockhart SR, Etienne KA, Vallabhaneni S, et al. Simultaneous emergence of multidrug-resistant candida auris on 3 continents confirmed by whole-genome sequencing and epidemiological analyses. Clin Infect Dis. 2017;64:134–140. doi:10.1093/cid/ciw691
- Ksiezopolska E, Gabaldón T. Evolutionary emergence of drug resistance in candida opportunistic pathogens. Genes. 2018;9:461. doi:10.3390/genes9090461
- Mota Fernandes C, Dasilva D, Haranahalli K, et al. The future of antifungal drug therapy: novel compounds and targets. Antimicrob Agents Chemother. 2021;65:1–13. doi:10.1128/AAC.01719-20
- Parente-Rocha JA, Bailão AM, Amaral AC, et al. Antifungal resistance, metabolic routes as drug targets, and new antifungal agents: an overview about endemic dimorphic fungi. Mediators Inflamm. 2017;2017:1–16. doi:10.1155/2017/9870679
- Miceli MH, Kauffman CA. Isavuconazole: a new broad-spectrum triazole antifungal agent. Clin Infect Dis. 2015;61:1558–1565. doi:10.1093/cid/civ571
- Nayak N. Fungal infections of the eye--laboratory diagnosis and treatment. Nepal Med Coll J. 2008;10:48–63.
- Boyd NK, Teng C, Frei CR. Brief overview of approaches and challenges in new antibiotic development: a focus on drug repurposing. Front Cell Infect Microbiol. 2021;11:1–12. doi:10.3389/fcimb.2021.684515
- Nguyen HM, Graber CJ. Limitations of antibiotic options for invasive infections caused by methicillin-resistant Staphylococcus aureus: is combination therapy the answer? J Antimicrob Chemother. 2009;65:24–36. doi:10.1093/jac/dkp377
- Stevens DL, Ma Y, Salmi D, et al. Impact of antibiotics on expression of Virulence-Associated Exotoxin genes in methicillin-sensitive and methicillin-resistant staphylococcus aureus. J Infect Dis. 2007;195:202–211. doi:10.1086/510396
- Miller LA, Ratnam K, Payne DJ. β - Lactamase-inhibitor combinations in the 21st century: current agents and new developments. Curr Opin Pharmacol. 2001;1:451–458. doi:10.1016/S1471-4892(01)00079-0
- Shrestha SK, Fosso MY, Garneau-Tsodikova S. A combination approach to treating fungal infections. Sci Rep. 2015;5:1–11. doi:10.1038/srep17070
- Amin B, Hosseinzadeh H. Black Cumin (Nigella sativa) and Its active constituent, thymoquinone: an overview on the analgesic and anti-inflammatory effects. Planta Med. 2016;82:8–16. doi:10.1055/s-0035-1557838
- Forouzanfar F, Fazly Bazzaz BS, Hosseinzadeh H. Black cumin (Nigella sativa) and its constituent (thymoquinone): a review on antimicrobial effects. IRAN J BASIC MED SCI. 2014;17:929–938.
- Almshawit H, Macreadie I. Fungicidal effect of thymoquinone involves generation of oxidative stress in Candida glabrata. Microbiol Res. 2017;195:81–88. doi:10.1016/j.micres.2016.11.008
- Elfadil A, et al. The wound healing potential of 2,3 dimethylquinoxaline hydrogel in rat excisional wound model. J Pharm Res Int. 2023;35:1–8. doi:10.9734/jpri/2023/v35i87339
- Tan CM, Therien AG, Lu J, et al. Restoring Methicillin-Resistant Staphylococcus aureus Susceptibility to β-Lactam Antibiotics. Sci Transl Med. 2012;4:126ra35. doi:10.1126/scitranslmed.3003592
- Mikkelsen K, Sirisarn W, Alharbi O, et al. The novel membrane-associated auxiliary factors AuxA and AuxB Modulate β -lactam Resistance in MRSA by stabilizing lipoteichoic acids. Int J Antimicrob Agents. 2021;57:106283. doi:10.1016/j.ijantimicag.2021.106283
- Clinical and Laboratory Standards Institute (CLSI). M07-A9: methods for dilution antimicrobial susceptibility tests for bacteria that grow aerobically; approved standard—Ninth edition; 2002. Available from: https://clsi.org/media/1928/m07ed11_sample.pdf. Accessed May 31, 2024.
- Elfadil A, Ibrahem K, Abdullah H, et al. Synergistic Activity of 3-Hydrazinoquinoxaline-2-thiol in combination with penicillin against MRSA. Infect Drug Resist. 2024;17:355–364. doi:10.2147/IDR.S448843
- Hu Y, Liu Y, Coates A. Azidothymidine produces synergistic activity in combination with colistin against antibiotic-resistant Enterobacteriaceae. Antimicrob Agents Chemother. 2019;63:1–11. doi:10.1128/AAC.01630-18
- Liu X, Pai PJ, Zhang W, et al. Proteomic response of methicillin- resistant S. aureus to a synergistic antibacterial drug combination: a novel erythromycin derivative and oxacillin. Sci Rep. 2016;25:1–12.
- Ryder NS, Leitner I. Synergistic interaction of terbinafine with triazoles or amphotericin B against Aspergillus species. Med Mycol. 2001;39:91–95. doi:10.1080/mmy.39.1.91.95
- Kullberg BJ, Arendrup MC. Invasive candidiasis. N Engl J Med. 2015;373:1445–1456. doi:10.1056/NEJMra1315399
- Bassetti M, Peghin M, Timsit JF. The current treatment landscape: candidiasis. J Antimicrob Chemother. 2016;71:ii13–ii22. doi:10.1093/jac/dkw392
- Morgan J, Meltzer MI, Plikaytis BD, et al. Excess mortality, hospital stay, and cost due to candidemia: a case-control study using data from population-based candidemia surveillance. Infect Control Hosp Epidemiol. 2005;26:540–547. doi:10.1086/502581
- Dera AA, Ahmad I, Rajagopalan P, et al. Synergistic efficacies of thymoquinone and standard antibiotics against multi-drug resistant isolates. Saudi Med J. 2021;42:196–204. doi:10.15537/smj.2021.2.25706
- Mulani MS, Kamble EE, Kumkar SN, Tawre MS, Pardesi KR. Emerging strategies to combat ESKAPE pathogens in the era of antimicrobial resistance: a review. Front Microbiol. 2019;10. doi:10.3389/fmicb.2019.00539
- Gonzales PR, Pesesky MW, Bouley R, et al. Synergistic, collaterally sensitive β-lactam combinations suppress resistance in MRSA. Nat Chem Biol. 2015;11:855–861. doi:10.1038/nchembio.1911
- Fan Q, Yuan Y, Jia H, et al. Antimicrobial and anti-biofilm activity of thymoquinone against Shigella flexneri. Appl. Microbiol. Biotechnol. 2021;105:4709–4718. doi:10.1007/s00253-021-11295-x
- Hariharan P, Paul-Satyaseela M, Gnanamani A. In vitro profiling of antimethicillin-resistant Staphylococcus aureus activity of thymoquinone against selected type and clinical strains. Lett Appl Microbiol. 2016;62:283–289. doi:10.1111/lam.12544
- Phillips IAN. Clinical uses and control of rifampicin and clindamycin. J Clin Pathol. 1971;24:410–418. doi:10.1136/jcp.24.5.410
- Cheng G, Sa W, Cao C, et al. Quinoxaline 1,4-di-N-oxides: biological activities and mechanisms of actions. Front Pharmacol. 2016;7:1–21. doi:10.3389/fphar.2016.00064
- Adegbeye MJ, Elghandour MMMY, Faniyi TO, et al. Antimicrobial and antihelminthic impacts of black cumin, pawpaw and mustard seeds in livestock production and health. Agrofor Syst. 2020;94:1255–1268. doi:10.1007/s10457-018-0337-0
- Chacón-Vargas KF, Andrade-Ochoa S, Nogueda-Torres B, et al. Isopropyl quinoxaline-7-carboxylate 1,4-di-N-oxide derivatives induce regulated necrosis-like cell death on Leishmania (Leishmania) mexicana. Parasitol Res. 2018;117:45–58. doi:10.1007/s00436-017-5635-3
- Rani R, Sharma D, Chaturvedi M, Parkash Yadav J. Antibacterial activity of twenty different endophytic fungi isolated from calotropis procera and time kill assay. Clin Microbiol Open Access. 2017;06. doi:10.4172/2327-5073.1000280
- Duan L, Zhang J, Chen Z, et al. Antibiotic combined with epitope-specific monoclonal antibody cocktail protects mice against bacteremia and acute pneumonia from methicillin-resistant staphylococcus aureus infection. J Inflamm Res. 2021;14:4267–4282. doi:10.2147/JIR.S325286
- Craig WA. Pharmacokinetic/pharmacodynamic parameters: rationale for antibacterial dosing of mice and men. Clin Infect Dis. 1998;26:1–12. doi:10.1086/516284