Abstract
Objective
Sarcopenia is a universal problem in elderly individuals. The molecular regulatory mechanisms in sarcopenia are not well understood. In the present study, we explored a possible molecular mechanism involved in the pathogenesis of sarcopenia.
Methods
Differentially expressed genes (DEGs) were identified using the Gene Expression Omnibus (GEO) database. Signaling pathways related to these DEGs were identified by gene set enrichment analysis (GSEA). Pearson correlation was calculated for all the pairwise comparisons of gene expression values between coding genes and DEGs. Interactions between the proteins encoded by the DEGs were identified using the STRING database. Gene ontology (GO) and Kyoto Encyclopedia of Genes and Genomes (KEGG) biological pathway analyses were performed to predict the functions of the DEGs.
Results
Three differentially expressed miRNAs and 5 differentially expressed mRNAs were identified in association with DEGs. We found that miRNA-1245a expression in patients with sarcopenia was higher than that in healthy controls. The GSEA showed that many pathways, such as the JAK-STAT signaling pathway and pathways related to glioma, gap junctions, and regulation of the actin cytoskeleton, were enriched in the high-miRNA-1245a-expression group. A total of 127 miRNA-1245a-related mRNAs were identified. The GO and KEGG analyses revealed that miRNA-1245a had a strong effect on a number of fundamental biological processes, such as kinase activity, that are related to the development of sarcopenia.
Conclusion
Our analyses indicate that miRNA-1245a may be a potential key molecule in the diagnosis and treatment of sarcopenia, which provides a basis for the research of miRNA in sarcopenia.
Introduction
Due to the aging of the global population, age-related diseases, such as sarcopenia, are becoming a great concern worldwide. The overall prevalence of sarcopenia is 10% in the 60–69-year-old population, and as people age, the incidence can exceed 40% percent in people over 80 years old.Citation1,Citation2 More than 200 million people worldwide are expected to suffer from sarcopenia by 2050.Citation3 The European Working Group on Sarcopenia in Older People (EWGSOP) recommended using the presence of low muscle mass and muscle strength or physical performance to develop a practical clinical definition. Sarcopenia is an age-related syndrome characterized by progressive degeneration of skeletal muscle mass and functional decline, and it is a major contributor to the risk of physical disability, poor quality of life and premature death.Citation3 As a major cause of frailty, disability and mortality in the elderly, sarcopenia has recently received considerable attention.Citation4,Citation5 The potential molecular mechanisms of sarcopenia are not completely understood but appear to involve multiple pathways associated with a decline in skeletal muscle mass and function, including inflammation, protein synthesis and degradation, autophagy, impaired satellite cell activation, and mitochondrial dysfunction.Citation6,Citation7 Patients with sarcopenia often have metabolic diseases such as diabetes mellitus, hyperlipidemia and hypertension.Citation8 Considering the increase of life expectancy and the growing elderly population, a steady increase in sarcopenia in the upcoming decades will become a heavy financial burden on families, society and the health care system. Many efforts have been suggested to clarify the molecular mechanisms of sarcopenia for the purpose of developing efficient prevention strategies and appropriate treatment measures. Hence, much effort has been focused on identifying and characterizing the many pathways and mechanisms underlying sarcopenia. MicroRNAs (miRNAs) are critical regulators involved in nearly all cellular processes through the post-transcriptional regulation of gene expression. miRNAs play key roles in satellite cells regulation, myogenesis induction, muscle protein homeostasis, neurodegeneration, and fat infiltration.Citation9 Notably, their expression profiles change with age.Citation10 miRNAs were associated with many signaling pathways, such as TGF-β/myostatin/BMP and PI3K/AKT/mTOR, which were shown to contribute to sarcopenia development.Citation9
In the present study, RNA expression profile datasets were downloaded from the Gene Expression Omnibus (GEO) database to identify differentially expressed genes (DEGs) in patients with sarcopenia by comprehensively applying multiple bioinformatics analysis tools. The results of the gene expression analysis provide in-depth insights into the cellular and molecular mechanisms related to the development of sarcopenia.
Methods
Microarray Data and Data Preprocessing
Gene expression profiles of GSE111006 (with 32 samples), GSE111010 (with 23 samples), and GSE111016 (with 40 samples) were obtained from the GEO database. All datasets were based on the GPL16791 platform. depicted the workflow of this study. Raw data on RNA expression were obtained, from which reads per million data were extracted. A log2 transformation was applied to the data and normalized by the median intensity.
Identification of DEGs
The “limma” R package was used to perform DEG analysis by comparing samples obtained from sarcopenia and healthy control groups. The Benjamini-Hochberg method was used to adjust the raw p-values to the false discovery rate (FDR).Citation11 DEGs were identified based on the criteria of |log2FC|>1 and FDR < 0.25.
Screening of mRNAs Related to DEGs
The “limma” R package was applied to calculate the Pearson correlation between the mRNAs and DEGs. An mRNA with a |correlation coefficient| > 0.6 and P < 0.01 was considered to be a DEG-related mRNA. Interactions between the proteins encoded by the DEGs were identified using the STRING database (http://string-db.org).
Gene Set Enrichment Analysis (GSEA)
GSEA was used to interpret gene expression data by using GSEA software 4.1 from the Broad Institute.Citation12 The normalized enrichment score (NES) was calculated for each gene set. Significant KEGG enrichment was determined with the criteria set as a normal P-value <0.01 and FDR <0.25.
Gene Ontology (GO) and Kyoto Encyclopedia of Genes and Genomes (KEGG) Pathway Enrichment Analyses
GO and KEGG enrichment analyses were performed to reveal the functions and pathways of DEGs, respectively, using the “clusterProfiler” R package.
Statistical Analysis
All statistical analyses were performed using R software (version 4.0.2). The Wilcoxon signed-rank test was performed to determine the difference in the expression of genes. P < 0.05 was considered to be statistically significant.
Results
Differentially Expressed Genes in Sarcopenia
The first step, which was to select and retrieve all appropriate datasets, identified the differentially expressed genes (DEGs) in skeletal muscle samples obtained from patients with sarcopenia and healthy controls. The t test in the “limma” package was used to identify the DEGs, and volcano plots were generated to visualize the DEGs (). In total, 3 differentially expressed miRNAs and 5 differentially expressed mRNAs were identified. We found that miRNA-1245a expression in the patients with sarcopenia was higher than that in the healthy controls. A heat map and a violin plot showing the expression levels of the DEGs are shown in and .
Figure 2 Analysis of differentially expressed genes (DEGs). (A) Volcano plot of the DEGs identified by integrated analysis of the GEO datasets (red dot represents upregulated gene, blue dot represents down-regulated gene). A cutoff of a 1-fold change and FDR of <0.25 were used as the threshold values to determine DEG significance. (B) The heat map shows the expression of DEGs in sarcopenia patients compared to controls (red bars represent sarcopenia population, blue bars represent healthy population). (C) Violin plot analysis was performed to compare the expression of DEGs (red represents sarcopenia population, blue represents healthy population).
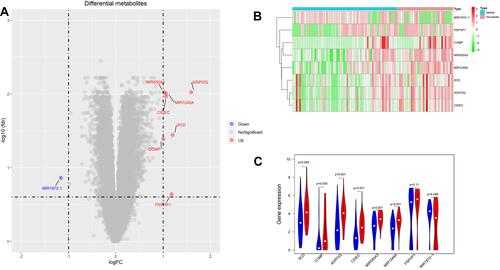
GSEA
Patients were divided into groups of high- and low-expression miRNA-1245a to elucidate the molecular mechanism critical for the development of sarcopenia. Finally, the outcome of GSEA showed that the KEGG pathways enriched in the high-expression group included the JAK-STAT signaling pathway and pathways related to glioma, gap junctions, and regulation of the actin cytoskeleton ().
Identification and Enrichment Analysis of miRNA-1245a-Related Genes
We also identified miRNA-1245a-related genes by Pearson correlation analysis. There were a total of 28 miRNA-1245a-related genes that were contained in the PPI network (). The GO analysis showed that these genes were enriched in extrinsic components of the membrane, kinase regulator activity, and regulation of MAP kinase activity (). The KEGG analysis showed that the miRNA-1245a-related genes were mainly related to the chemokine signaling pathway and Fc gamma R-mediated phagocytosis ().
Discussion
To identify more useful biomarkers in sarcopenia, bioinformatics methods were used with three profile datasets (GSE111006, GSE111010 and GSE1111016). Thirty-three sarcopenia patients and sixty-two healthy controls were enrolled in the present research. We found a total of 3 changed miRNAs, including 2 upregulated and 1 downregulated miRNAs in the patients with sarcopenia. Only miR-1245a was used to analysis as a target biomarker for sarcopenia rather than another miRNA in our study because few miRNA-related genes were identified except for miRNA-1245a by Pearson correlation analysis (data not shown). A large number of miRNAs are important regulators of age-related muscle atrophy. A more comprehensive understanding of the roles of miRNAs in sarcopenia as a whole will continue to evolve. miR-550a-3p can negatively regulate the expression of ERK1 and ERK2.Citation13 Upregulation of miR-550a-3p in sarcopenia may lead to the downregulation of the ERK1/2 signaling pathway. A previous study showed that Hachimijiogan promoted the proliferation of C2C12 skeletal muscle cells through the ERK1/2 signaling pathway without activation of the Akt signaling pathway.Citation14 In addition, a study showed that undercarboxylated osteocalcin enhanced myogenic differentiation via activation of the GPRC6A-ERK1/2 signaling pathway.Citation15 Exercise training was previously shown to ameliorate the aging-related muscle changes in microstructure, mitochondria, and performance through the activation of the ERK1/2 signaling pathway.Citation16 Therefore, in this study, we inferred that the upregulation of miRNA-550a-3p might promote the development of sarcopenia by downregulating the ERK1/2 signaling pathway. To date, no information about the biological role of miRNA-1972-1 has been reported.
It was previously shown that the expression level of miRNA-1245a was downregulated after chronic resistance exercise (RE).Citation17 However, the role of miRNA-1245a in muscle adaptation to RE training was not elucidated. Our results revealed that KEGG pathways enriched in the group with high expression of miRNA-1245a included the JAK-STAT signaling pathway and pathways related to glioma, gap junctions, and regulation of the actin cytoskeleton, which might help explain the underlying role of miRNA-1245a. Interestingly, a previous study showed that differentially methylated genes were mainly associated with the regulation of the actin cytoskeleton, energy metabolism, and muscle function in older women with sarcopenia.Citation18
The activity of muscle satellite cells (SCs) was shown to contribute to skeletal muscle regeneration and the maintenance of muscle mass.Citation19 Human aging is associated with susceptibility to muscle inflammation, which contributes to the impaired regenerative capacity of skeletal muscle.Citation20 Inhibition of JAK-STAT signaling was previously shown to result in a marked enhancement of muscle repair.Citation21 A study showed elevated p38αβ MAPK activity in freshly isolated aged SCs compared to that in young SCs.Citation22 Partial inhibition of p38αβ MAPK signaling was shown to restore the generation of Pax7+ cells and increase the self-renewal of aged SCs.Citation22 Activin A was shown to induce skeletal muscle catabolism through a p38 MAPK-independent mechanism.Citation23 Hyperhomocysteinemia was shown to inhibit the regenerative capacity of SCs through p38 MAPK signaling.Citation22
Certain limitations of these studies should be considered. First, a small number of DEGs were identified in our analysis. In addition, a previous study showed eight miRs to be upregulated in cancer cachexia, with FDRs ranging from 0.21 to 0.22;Citation24 this result may have been related to the selection of muscle tissues from elderly individuals as a control. If we had chosen to use data from the Genotype-Tissue Expression (GETx) database as a control, more than 1000 DEGs may have been identified. Moreover, more differentially expressed miRNAs were found between old and young skeletal muscle samples in a study by Zheng et al.Citation25 Second, information on characteristics of the patients from which samples were obtained, such as insulin resistance, obesity, diabetes, high blood pressure, and lipid abnormalities, was not provided, which prevented us from further analyzing whether these factors played roles in miRNA-1245a expression. Third, the three GEO profile datasets were composed of samples with a variety of ethnic backgrounds. Forth, “in silico analysis” is within a matter of speculation. Therefore, more studies are warranted to perform further analysis, and further in vitro and in vivo studies are required to confirm our findings.
With aging, dysregulation of miRNA-1245a in muscle is closely associated with sarcopenia, suggesting that miRNA-1245a may be a viable diagnostic and treatment biomarker for sarcopenia.
Ethics Statement
Ethical review and approval was not required for the study on human participants in accordance with the local legislation and institutional requirements. Written informed consent for participation was not required for this study in accordance with the national legislation and the institutional requirements.
Author Contributions
All authors made a significant contribution to the work reported, whether that is in the conception, study design, execution, acquisition of data, analysis and interpretation, or in all these areas; took part in drafting, revising or critically reviewing the article; gave final approval of the version to be published; have agreed on the journal to which the article has been submitted; and agree to be accountable for all aspects of the work.
Acknowledgment
Thanks are due to Dr. Jing Wang (ORCID 0000-0001-9981-5530) for assistance with the analysis of data.
Disclosure
The authors report no conflicts of interest in this work.
Additional information
Funding
References
- Shafiee G, Keshtkar A, Soltani A, et al. Prevalence of sarcopenia in the world: a systematic review and meta- analysis of general population studies. J Diabetes Metab Disord. 2017;16:21. doi:10.1186/s40200-017-0302-x
- Melton LJ 3rd, Khosla S, Crowson CS, et al. Epidemiology of sarcopenia. J Am Geriatr Soc. 2000;48(6):625–630. doi:10.1111/j.1532-5415.2000.tb04719.x
- Cruz-Jentoft AJ, Baeyens JP, Bauer JM, et al. Sarcopenia: European consensus on definition and diagnosis: report of the European Working Group on Sarcopenia in Older People. Age Ageing. 2010;39(4):412–423. doi:10.1093/ageing/afq034
- Björkman M, Jyväkorpi SK, Strandberg TE, et al. Sarcopenia indicators as predictors of functional decline and need for care among older people. J Nutr Health Aging. 2019;23(10):916–922. doi:10.1007/s12603-019-1280-0
- Yang M, Liu Y, Zuo Y, et al. Sarcopenia for predicting falls and hospitalization in community-dwelling older adults: EWGSOP versus EWGSOP2. Sci Rep. 2019;9(1):17636. doi:10.1038/s41598-019-53522-6
- Rong S, Wang L, Peng Z, et al. The mechanisms and treatments for sarcopenia: could exosomes be a perspective research strategy in the future? J Cachexia Sarcopenia Muscle. 2020;11(2):348–365. doi:10.1002/jcsm.12536
- Fochi S, Giuriato G, De Simone T, et al. Regulation of microRNAs in satellite cell renewal, muscle function, sarcopenia and the role of exercise. Int J Mol Sci. 2020;21(18):6732. doi:10.3390/ijms21186732
- Richter-Stretton GL, Fenning AS, Vella RK. Skeletal muscle - A bystander or influencer of metabolic syndrome? Diabetes Metab Syndr. 2020;14(5):867–875. doi:10.1016/j.dsx.2020.06.006
- Yin J, Qian Z, Chen Y, et al. MicroRNA regulatory networks in the pathogenesis of sarcopenia. J Cell Mol Med. 2020;24(9):4900–4912. doi:10.1111/jcmm.15197
- Gullett JM, Chen Z, O’Shea A, et al. MicroRNA predicts cognitive performance in healthy older adults. Neurobiol Aging. 2020;95:186–194. doi:10.1016/j.neurobiolaging.2020.07.023
- Korthauer K, Kimes PK, Duvallet C, et al. A practical guide to methods controlling false discoveries in computational biology. Genome Biol. 2019;20(1):118. doi:10.1186/s13059-019-1716-1
- Subramanian A, Tamayo P, Mootha VK, et al. Gene set enrichment analysis: a knowledge-based approach for interpreting genome-wide expression profiles. Proc Natl Acad Sci U S A. 2005;102(43):15545–15550. doi:10.1073/pnas.0506580102
- Wan C, Wen J, Huang Y, et al. Microarray analysis of differentially expressed microRNAs in myelodysplastic syndromes. Medicine. 2020;99(27):e20904. doi:10.1097/MD.0000000000020904
- Takeda T, Tsuiji K, Li B, et al. Proliferative effect of Hachimijiogan, a Japanese herbal medicine, in C2C12 skeletal muscle cells. Clin Interv Aging. 2015;10:445–451. doi:10.2147/CIA.S75945
- Liu S, Gao F, Wen L, et al. Osteocalcin Induces Proliferation via Positive Activation of the PI3K/Akt, P38 MAPK Pathways and Promotes Differentiation Through Activation of the GPRC6A-ERK1/2 Pathway in C2C12 Myoblast Cells. Cell Physiol Biochem. 2017;43(3):1100–1112. doi:10.1159/000481752
- Inoue A, Cheng XW, Huang Z, et al. Exercise restores muscle stem cell mobilization, regenerative capacity and muscle metabolic alterations via adiponectin/AdipoR1 activation in SAMP10 mice. J Cachexia Sarcopenia Muscle. 2017;8(3):370–385. doi:10.1002/jcsm.12166
- Ogasawara R, Akimoto T, Umeno T, et al. MicroRNA expression profiling in skeletal muscle reveals different regulatory patterns in high and low responders to resistance training. Physiol Genomics. 2016;48(4):320–324. doi:10.1152/physiolgenomics.00124.2015
- He L, Khanal P, Morse CI, et al. Differentially methylated gene patterns between age-matched sarcopenic and non-sarcopenic women. J Cachexia Sarcopenia Muscle. 2019;10(6):1295–1306. doi:10.1002/jcsm.12478
- Bellamy LM, Joanisse S, Grubb A, et al. The acute satellite cell response and skeletal muscle hypertrophy following resistance training. PLoS One. 2014;9(10):e109739. doi:10.1371/journal.pone.0109739
- Merritt EK, Stec MJ, Thalacker-Mercer A, et al. Heightened muscle inflammation susceptibility may impair regenerative capacity in aging humans. J Appl Physiol. 2013;115(6):937–948. doi:10.1152/japplphysiol.00019.2013
- Price FD, von Maltzahn J, Bentzinger CF, et al. Inhibition of JAK-STAT signaling stimulates adult satellite cell function. Nat Med. 2014;20(10):1174–1181. doi:10.1038/nm.3655
- Bernet JD, Doles JD, Hall JK, et al. p38 MAPK signaling underlies a cell-autonomous loss of stem cell self-renewal in skeletal muscle of aged mice. Nat Med. 2014;20(3):265–271. doi:10.1038/nm.3465
- Ding H, Zhang G, Sin KW, et al. Activin A induces skeletal muscle catabolism via p38β mitogen-activated protein kinase. J Cachexia Sarcopenia Muscle. 2017;8(2):202–212. doi:10.1002/jcsm.12145
- Narasimhan A, Ghosh S, Stretch C, et al. Small RNAome profiling from human skeletal muscle: novel miRNAs and their targets associated with cancer cachexia. J Cachexia Sarcopenia Muscle. 2017;8(3):405–416. doi:10.1002/jcsm.12168
- Zheng Y, Kong J, Li Q, et al. Role of miRNAs in skeletal muscle aging. Clin Interv Aging. 2018;13:2407–2419. doi:10.2147/CIA.S169202