Abstract
Despite widespread use and prospective biomedical applications of copper nanoparticles (Cu NPs), their biosafety issues and kinetics remain unclear. Thus, the aim of this study was to compare the detailed in vivo toxicity of Cu NPs and cupric ions (CuCl2; Cu ions) after a single oral dose. We determined the physicochemical characteristics of Cu NPs, including morphology, hydrodynamic size, zeta potential, and dissolution in gastric (pH 1.5), vehicle (pH 6.5), and intestinal (pH 7.8) conditions. We also evaluated the kinetics of Cu following a single equivalent dose (500 mg/kg) of Cu NPs and Cu ions. Cu NPs had highest dissolution (84.5%) only in gastric conditions when compared with complete dissolution of Cu ions under various physiological milieus. Kinetic analysis revealed that highest Cu levels in blood and tested organs of Cu NP-treated rats were 15%–25% lower than that of Cu ions. Similar to the case of Cu ions, Cu levels in the tested organs (especially liver, kidney, and spleen) of Cu NP-treated rats increased significantly when compared with the vehicle control. However, delay in reaching the highest level and biopersistence of Cu were observed in the blood and tested organs of Cu NP-treated rats compared with Cu ions. Extremely high levels of Cu in feces indicated that unabsorbed Cu NPs or absorbed Cu ions were predominantly eliminated through liver/feces. Cu NPs exerted apparent toxicological effects at higher dose levels compared with Cu ions and showed sex-dependent differences in mortality, biochemistry, and histopathology. Liver, kidney, and spleen were the major organs affected by Cu NPs. Collectively, the toxicity and kinetics of Cu NPs are most likely influenced by the release of Cu dissociated from Cu NPs under physiological conditions.
Introduction
In recent years, scientific research on nanoparticles (NPs) has shown a tremendous and rapid growth owing to their wide applications in various fields, including electronics, cosmetics, food industry, and medicine.Citation1–Citation3 The use of fabricated NPs has great potential in a wide range of applications, since their distinct physicochemical characteristics can be utilized in an application-specific manner by modifying their size, surface properties, and shape.Citation4 However, their unique physicochemical properties may affect their toxicological behavior in vivo by facilitating cellular uptake and translocation of the particles in the body.Citation5,Citation6 Furthermore, the physiological milieu influences the interaction between biological systems and NPs and can determine the fate and biosafety of NPs.Citation7 Currently, with the widespread use of NPs, health risks and environmental effects related to NP exposure have become of great concern.Citation8 In addition, the applications of NPs have been limited by lack of verification of biosafety.Citation9–Citation11 Thus, a detailed evaluation of their potential toxicity in vivo must precede any prospective applications.Citation12
Copper (Cu) is an essential element required for normal physiological functions in humans and animals, including hemoglobin formation, drug metabolism, carbohydrate metabolism, catecholamine biosynthesis, and antioxidant defense mechanism.Citation13,Citation14 However, when the intake of Cu exceeds the range of biological tolerance, it can cause adverse effects, including hemolysis, gastrointestinal distress, and liver and kidney damage.Citation15,Citation16 Recent toxicological investigations of NPs have revealed that they tend to exhibit quite different toxicological effects in vivo compared with larger particles of the same chemical composition.Citation17–Citation19 Copper nanoparticles (Cu NPs) and their oxidized form, copper oxide (CuO) NPs, are widely used as additives in livestock and poultry feed, polymers/plastics, and lubricants for metallic coating.Citation20 The excellent thermophysical properties of Cu NPs raise their value for applications in the field of electronics technology, such as semiconductors, electronic chips, and heat transfer nanofluids.Citation20,Citation21 In addition, synthesis of Cu NPs is cost effective, and the synthesized Cu NPs display antimicrobial activity against a wide range of pathogenic microorganisms. Such advantages of NPs have put them in the spotlight as an alternative antimicrobial agent in many biomedical applications.Citation22–Citation24 Despite the great potential of Cu NPs in biomedical applications, toxicity studies of Cu NPs and CuO NPs have been mostly limited to in vitro studies. Cu NPs exhibit cytotoxic effects on various cell lines.Citation25–Citation31 Additionally, CuO NPs have high cytotoxicity compared with conventional metal oxide NPs and carbon nanotubes.Citation32 Although there are increasing numbers of in vitro studies, only few reports investigated the in vivo toxicity of Cu NPs.Citation17,Citation33–Citation35 The acute toxicity study of Cu NPs and Cu ions has been previously conducted in mice.Citation17 The kinetics were evaluated limitedly on serum, kidney, and urine for 72 hours after exposure.Citation35 Despite these, limited information concerning the toxicity and kinetics was available. Furthermore, there was no information on biopersistence and excretion data of Cu NPs for long periods after exposure. For their practical applications, it is highly desirable to make a detailed and systematic study on the toxicity of Cu NPs and their kinetics in vivo. In this study, we conducted a detailed toxicity study and analyzed the biodistribution in a variety of other organs, including liver, brain, lung, heart, and spleen for 4 weeks, after single exposure. Combined with toxicity data, kinetics data for long term can provide valuable information about biopersistence/clearance and lead to better understanding of toxicity profiles of Cu NPs.
Herein, we determined the morphological and physico-chemical properties of Cu NPs. Compared with other routes of exposure, gastrointestinal route has potential for wide exposure of the public to higher dose of NPs due to more frequent ingestion via water, food, cosmetics, and drugs.Citation36,Citation37 Thus, we investigated the in vivo toxicity and kinetics of Cu NPs following oral exposure. To evaluate their in vivo toxicity, we performed a single oral dose toxicity study of Cu NPs in rats by evaluating mortality, biochemical parameters, and histopathology. In addition, we conducted a comparative toxicity study with their ionic form copper(II) chloride (CuCl2, Cu ions) to investigate whether in vivo toxicity of Cu NPs is influenced by the release of Cu dissociated from Cu NPs in physiological conditions when administered orally. In vivo kinetic study of nanoparticulate form and ionic forms of Cu was conducted to better understand the correlation between in vivo toxicity and their fate in biological systems, which can provide information regarding the biocompatibility of Cu NPs for their further application.
Materials and methods
Test chemicals and treatment
Cu NPs (CAS no 7440-50-8; 99.8% purity) were purchased from Skyspring Nanomaterials (Houston, TX, USA). The average particle size measured by transmission electron microscopy (TEM) and specified by manufacturer was 25 nm. Copper(II) chloride (CAS no 10125-13-0; CuCl2, >99.9% purity, molecular weight: 134.45 g/mol) purchased from Sigma-Aldrich Co. (St Louis, MO, USA) used as Cu ions. Hydroxypropylmethylcellulose (HPMC) (Sigma-Aldrich Co.) was used as a suspending vehicle. All other chemicals were of the highest grade commercially available. Cu NPs and Cu ions were dispersed into 1% HPMC solution (w/v) with Milli-Q water. The suspensions containing Cu NPs were prepared with ultrasonic dispersion (VCX130; Vibra Cell Sonics & Materials, Newtown, CT, USA) on ice for 20 minutes (130 W, 20 kHz, pulse 59/1) and vibrated for 2 minutes. To ensure that did not aggregate before administration, the time interval from preparation to oral gavage was limited to <20 minutes.
Physicochemical characterization of Cu NPs
Primary particle size and morphology were measured using TEM (JEM-2100F; JEOL, Tokyo, Japan) at an accelerating voltage of 150 kV and scanning electron microscopy (SEM; Zeiss EVO-MA10; Carl Zeiss Meditec AG, Jena, Germany) at an accelerating voltage of 15 kV. Samples for TEM analysis were prepared using a drop of solution of suspended NPs on carbon-coated nickel grids. The films on the TEM grids were allowed to dry before measurement. Samples for SEM analysis were dispersed onto double-sided adhesive carbon tape in an aluminum SEM stub and then dusted to release loose particles. The purity of NP powder was determined by energy-dispersive X-ray spectroscopy (JEM-2100F TEM equipped with X-MaxN 150 mm2 silicon drift detector; Oxford Instruments, Abingdon, UK). Hydrodynamic size and zeta potential of NPs were measured using ELS-8000 (Otsuka Electronics, Tokyo, Japan). Briefly, the optimal concentration of Cu NPs for measurement was determined by serial dilution under following conditions: temperature 25°C, refractive index 1.33, scattering angle 172°, and laser wavelength 633 nm. The zeta potential of NPs was measured under acidic conditions (artificial gastric fluid [AGF], pH 1.5) to simulate acidic gastric environment, vehicle conditions (pH 6.5), and basic conditions (deionized water, pH 7.8) to simulate the environment in the small intestine. Specific surface area of Cu NP powder was measured by the nitrogen absorption methods based on the multipoint Brunauer–Emmett–Teller method (ASAP2020; Micromeritics, Norcross, GA, USA). The levels of endotoxin in NP suspension were determined using a Pierce LAL Chromogenic Endotoxin Quantitation Kit (Thermo Fisher Scientific, Waltham, MA, USA).
Dissolution of Cu NPs and Cu ions in physiological conditions
The dissolution of Cu NPs and Cu ions was determined using above three pH conditions (AGF, vehicle, and deionized water) after 24 hours incubation. AGF was prepared according to a method described previously.Citation38 Briefly, 2.0 g NaCl and 3.2 g pepsin (Sigma-Aldrich Co.) were dissolved in 1 L of deionized water, and then the pH was adjusted to 1.5 using 2 N HCl (Sigma-Aldrich Co.). A total of 5 mg/mL of Cu NPs and Cu ions were incubated in the above solutions for 24 hours. After incubation, NP-free supernatants were collected by three repeated centrifugation at 15,000× g for 30 minutes. Supernatant samples were weighed ~1 g in 55 mL microwave digestion vessel and digested with 10 mL of concentrated nitric acid and 1 mL of 30% H2O2 overnight. It was heated in a microwave digestion system (ETHOS One; Milestone, Sorisole, Italy). Microwave digestion system condition was 40°C for 1 minute, 100°C for 20 minutes, 170°C for 2 hours to remove the remaining nitric acid and then allowed to cool. After samples were completely digested and colorless, the remaining solutions were diluted with 2% nitric acid. The degree of ionization was evaluated by determining Cu63, and their interference species (PO2, TiO, ArNa, etc) were excluded by methane gas. Cu analysis of each sample was carried out using inductively coupled plasma mass spectrometry (ICP-MS; NexION 300X; PerkinElmer Inc., Waltham, MA, USA). Cu standard solutions for ICP-MS calibration were prepared at concentrations of 5 ng/g, 10 ng/g, 50 ng/g, and 100 ng/g.
Experimental animals and environmental conditions
Sprague-Dawley rats were obtained from a specific pathogen-free colony at Samtako Co. (Osan, Republic of Korea). Forty-five rats (8 weeks old) of each sex were used for the in vivo toxicity study, and 45 male rats aged 7 weeks were used for the kinetic study. The animals were acclimated for 1 week before starting the experiments. Two rats were housed in each stainless wire mesh cage in a room maintained at a temperature of 23°C±3°C and a relative humidity of 50%±10% with artificial lighting from 08:00 to 20:00 and with 13–18 air changes per hour. Rats were provided tap water that had been sterilized by ultraviolet irradiation and commercial rodent chow (Samyang Feed, Wonju, Korea) ad libitum. The protocols for animals’ study were approved by the Institutional Animal Care and Use Committee of Chonnam National University (approval number: CNU IACUC-YB-2013-60), and the animals were cared for in accordance with the Guidelines for Animal Experiments of Chonnam National University.
Comparative single oral dose toxicity study
This study was conducted in compliance with the Test Guidelines of the Organization for Economic Cooperation and Development TG420 and 423.Citation39,Citation40 After a 7-day quarantine and acclimatization period, 45 healthy animals of each sex at 9 weeks of age were randomly assigned to nine experimental groups: four treatment groups receiving 312 mg/kg, 625 mg/kg, 1,250 mg/kg, and 2,500 mg/kg of Cu NPs, respectively; four treatment groups receiving 156 mg/kg, 312 mg/kg, 625 mg/kg, and 1,250 mg/kg of Cu ions, respectively; and one control group receiving 1% HPMC vehicle alone. The dose levels of Cu NPs were selected based on the results of a preliminary study. Six rats of each sex were assigned two groups (three males and three females per group) and were administered 1,000 mg/kg and 5,000 mg/kg of Cu NPs. All rats showed severe toxic symptoms at 5,000 mg/kg, whereas no treatment-related effects were found at 1,000 mg/kg. Thus, we selected 2,500 mg/kg as the highest dose level of Cu NPs. To compare their toxicity, dose levels of Cu ions were selected as overlapping with dose levels of Cu NPs. Each group consisted of five rats of each sex. The application volume (10 mL/kg body weight) was calculated based on the most recently recorded body weight of individual animals. Control rats received an equivalent volume of 1% HPMC vehicle alone. Median lethal dose (LD50) values of Cu NPs and Cu ions were calculated using Miller and Tainter’s Probit method.Citation41
Each animal was observed twice daily throughout test period for any clinical signs of toxicity or mortality. The body weight of each rat was measured on days 0, 1, 3, 7, and 14. At the scheduled necropsy, blood samples were collected from the vena cava under carbon dioxide (CO2) anesthesia. Collected blood samples were placed into tubes for serum separation and incubated at room temperature within 90 minutes. Serum samples were collected by centrifugation at 3,000 rpm for 10 minutes. Serum samples were evaluated using a blood chemistry autoanalyzer (Drichem 4000i; Fujifilm, Tokyo, Japan) to determine the following: aspartate aminotransferase (AST), alanine aminotransferase (ALT), alkaline phosphatase, blood urea nitrogen, creatinine, creatine phosphokinase (CPK), total bilirubin, total cholesterol (TCHO), total protein, albumin, lactate dehydrogenase (LDH), triglyceride (TG), sodium (Na), potassium (K), and chloride (Cl). After collecting blood samples, rats were sacrificed by exsanguination from the abdominal aorta and vena cava. All organs were removed, weighed, and examined for macroscopically visible lesions. The following organs were weighed: brain, thymus, heart, lung, liver, spleen, kidneys, adrenal glands, testes, seminal vesicles, prostates, epididymides, and ovaries. Relative weights were calculated based on the organ-to-body weight ratio. Histopathological evaluation was performed by fixing tissue in a 10% neutral-buffered formalin solution. The tissues were stained with hematoxylin and eosin for microscopic examination. All observations were made manually in a totally blinded manner using a light microscope with ×5, ×10, ×20, and ×40 objective lenses and a ×100 oil immersion lens.
In vivo absorption, biodistribution, and excretion study
After a 7-day quarantine and acclimatization period, 45 healthy male rats aged 8 weeks were randomly assigned to 15 groups (three rats per group). Seven treatment groups received a single oral dose of Cu NPs at 500 mg/kg, another seven treatment groups received a single oral dose of Cu ions at 500 mg/kg, and a vehicle control group received 1% HPMC alone. In the single oral dose toxicity study, Cu ions at 625 mg/kg caused several clinical signs, including hematuria, paleness, piloerection, diarrhea, and death. Therefore, we selected 500 mg/kg of Cu NPs and Cu ions to evaluate the absorption, distribution, and excretion. Three animals in each group were sacrificed at 0 hour (control), 12 hours, 24 hours, 48 hours, 72 hours, 1 week, 2 weeks, and 4 weeks after treatment. Absorption of Cu in Cu NP- and Cu ion-treated rats was determined from blood samples. To evaluate tissue distribution, tissue samples from the liver, kidneys, spleen, lung, heart, and brain were obtained and weighed. Excretion of Cu was measured using urine and feces samples. To collect urine and feces samples, three animals per groups were assigned to a metabolic cage for 3 hours before necropsy. The weighed blood, urine (~1 g), and tissues (~0.1 g) were digested with concentrated nitric acid overnight, and all samples were analyzed for elemental Cu and ICP-MS as described previously.
Statistical analysis
The numerical data were presented as mean ± SD. All statistical comparisons were analyzed by one-way analysis of variance followed by Dunnett’s multiple comparison test. A P-value of <0.05 was considered as statistically significant. Statistical analyses were performed using the GraphPad InStat Version 3.0 (GraphPad Software, Inc., La Jolla, CA, USA).
Results
Physicochemical characterization of Cu NPs
The morphology of Cu NPs was generally spherical shape confirmed by TEM and SEM analyses (). The size distribution of Cu NPs was characterized by TEM and dynamic light scattering. The average size of Cu NPs stated by the manufacturer was 25 nm, but the actual size was 32.7±10.45 nm (300 counts) (). Dynamic light scattering measurements showed that Cu NPs were prone to aggregation/agglomeration and formed larger size of NPs in vehicle (pH 6.5) and simulated intestinal conditions (pH 7.8) with a hydrodynamic diameter of 516.4±116.9 nm and 334.8±128.26 nm, respectively ( and ). However, the hydrodynamic diameter in simulated acidic conditions (pH 1.5) was not determined. Cu NPs powder consisted of Cu 98.15%, silicon 1.52%, and iron 0.33% as determined using energy-dispersive X-ray spectroscopy on the same images of TEM (data not shown). The specific surface area of Cu NPs was 14.7 m2/g as determined using Brunauer–Emmett–Teller methods. The zeta potential of the Cu NPs was 15.5±0.82 mV at pH 1.5, 1.32±1.2 mV at pH 6.5, and −6.2±0.2 mV at pH 7.8 (). The endotoxin levels of NP suspensions were not detected (data not shown).
Table 1 Physiochemical characterization of Cu NPs
Figure 1 Morphology and size distribution of Cu NPs.
Notes: (A and B) TEM and (C and D) SEM images of Cu NPs. Size distribution of (E) actual size and (F) hydrodynamic size of Cu NPs (in deionized water, pH 7.8) is determined by TEM and DLS methods, respectively.
Abbreviations: Cu NPs, copper nanoparticles; DLS, dynamic light scattering; SEM, scanning electron microscopy; TEM, transmission electron microscopy.
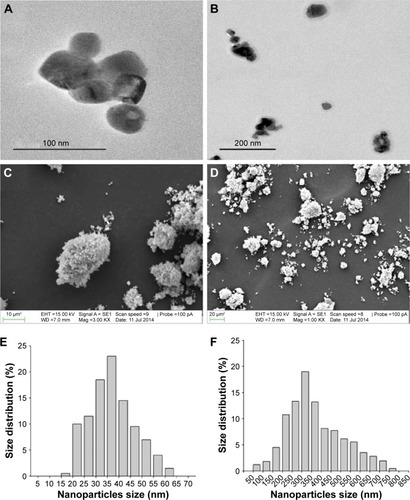
Dissolution of Cu NPs and Cu ions in gastric, vehicle, and intestinal conditions
The dissolution of Cu NPs and Cu ions in biological conditions was confirmed by ICP-MS method. Cu NPs displayed minimal solubility in vehicle (pH 6.5) or basic (pH 7.8) conditions simulated intestinal environments. However, Cu NPs were dissolved mostly in acidic conditions (pH 1.5) with a solubility of 84.5% (). In contrast, Cu ions were dissolved completely in all three conditions within 5 minutes.
Figure 2 Dissolution of Cu NPs in biological conditions and systemic absorption of Cu from Cu NPs and Cu ions.
Notes: (A) Dissolution ratios of Cu NPs and Cu ions in artificial gastric fluid (pH 1.5), vehicle (pH 6.5), and deionized water (pH 7.8) conditions, which simulated in vivo physiological conditions. Percentage of dissolution measured using ICP-MS after 24 hours incubation. (B) Absorption of Cu from Cu NP- and Cu ion-treated rats is measured at 0 hour (control), 12 hours, 24 hours, 48 hours, 72 hours, 1 week, 2 weeks, and 4 weeks after a single oral dose of 500 mg/kg each. Values are presented as mean ± SD (n=3). *P<0.05, **P<0.01 vs vehicle control. Cu ions, copper(II) chloride.
Abbreviations: AGF, artificial gastric fluid; DW, deionized water; Cu NPs, copper nanoparticles; HPMC, hydroxypropylmethylcellulose; ICP-MS, inductively coupled plasma mass spectrometry; SD, standard deviation.
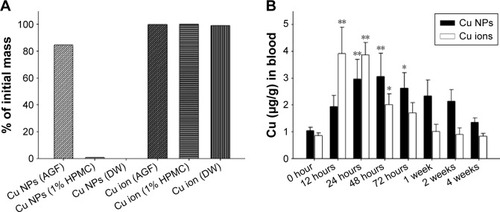
In vivo kinetics of Cu NPs and Cu ions
The in vivo kinetics of Cu NPs (500 mg/kg) and Cu ions (500 mg/kg, equivalent to 236 mg/kg based on Cu mass) were assessed using ICP-MS after oral administration, and then animals were sacrificed after 12 hours, 24 hours, 48 hours, 72 hours, 1 week, 2 weeks, and 4 weeks. The Cu content in the blood from Cu NP-treated groups was 51% lower (1.94 µg/g) than the highest level (3.91 µg/g) in Cu ion-treated rats at 12 hours (). The Cu NP-treated rats showed a highest level of the blood Cu (3.06 µg/g) at 48 hours and a prolonged blood circulation time even at 4 weeks. The tissue distribution patterns of Cu also showed a similar trend as observed for blood Cu levels in Cu NP- and Cu ion-treated rats. As shown in , Cu NPs showed a gradual increase in Cu levels and peaked at 48 hours or 72 hours in tested organs. Cu NP-treated rats displayed high values of Cu levels in the liver (516.5 µg/g), kidneys (54.7 µg/g), heart (47.6 µg/g), and spleen (38.1 µg/g). After reaching a peak, Cu levels gradually decreased from these organs. In contrast, Cu ions displayed a rapid increase in concentration within 12 hours and reached higher Cu values at 24 hours in the tested organs than those of Cu NPs. The Cu concentration in the tested organs from Cu ion-treated rats also showed a gradual decrease after reaching the highest level, but Cu levels in liver sharply decreased. Cu levels in the urine of Cu NP-treated rats were lower (1.42 µg/g at 72 hours) but gradually increased, relative to Cu ion-treated rats, which exhibited a sharp increase in Cu concentration and a twofold higher Cu content (2.82 µg/g at 24 hours) (). In contrast with urine excretion, Cu NP-treated rats showed 2.5-fold higher content of Cu in the excreted feces (23,667 µg/g at 24 hours) (), whereas less Cu content was excreted in the feces of Cu ion-treated rats (9,315 µg/g at 48 hours).
Figure 3 Biodistribution of Cu from Cu NPs and Cu ions.
Notes: Cu levels in (A) liver, (B) kidney, (C) spleen, (D) heart, (E) lung, and (F) brain in the Cu NP- and Cu ion-treated rats are determined at 0 hour (control), 12 hours, 24 hours, 48 hours, 72 hours, 1 week, 2 weeks, and 4 weeks after a single oral dose of 500 mg/kg each. Values are presented as mean ± SD (n=3). *P<0.05, **P<0.01 vs vehicle control. Cu ions, copper(II) chloride.
Abbreviations: Cu NPs, copper nanoparticles; SD, standard deviation.
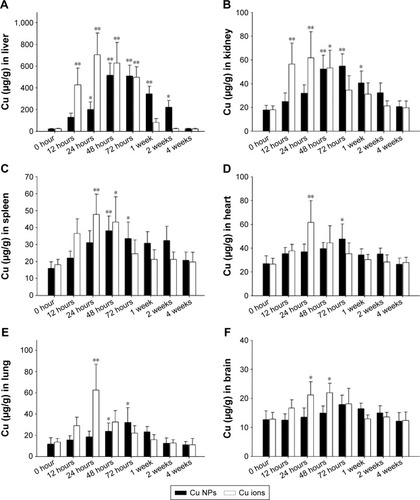
Figure 4 Excretion of Cu from Cu NPs and Cu ions.
Notes: Cu levels in (A) urine and (B) feces of Cu NP- and Cu ion-treated rats are measured at 0 hour (control), 12 hours, 24 hours, 48 hours, 72 hours, 1 week, 2 weeks, and 4 weeks after a single oral dose of 500 mg/kg each. Values are presented as mean ± SD (n=3). *P<0.05, **P<0.01 vs vehicle control. Cu ions, copper(II) chloride.
Abbreviations: Cu NPs, copper nanoparticles; SD, standard deviation.
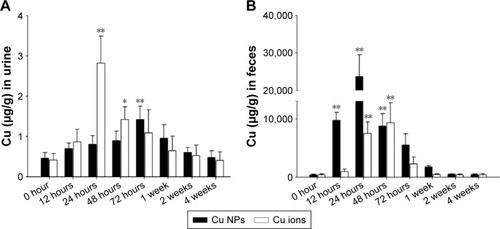
In vivo toxicity of Cu NPs and Cu ions
Mortality, body weight changes, and clinical signs
In this study, the in vivo toxicity of Cu NPs was assessed at multiple dose levels (312–2,500 mg/kg) following oral administration. Their toxicity was compared with that of Cu ions at 156–1,250 mg/kg. The LD50 value of Cu NPs (1,344 mg/kg) in male rats was 2.1-fold higher than that of Cu ions in male rats (640 mg/kg). In female rats, the oral LD50 value of Cu NPs (2,411 mg/kg) was 4.2-fold higher than that of Cu ions (571 mg/kg) based on mortality (survival rate) (). In addition, Cu NP-treated male rats showed more severe clinical signs, less body weight gain, and lower LD50 value than those of female rats, but no sex differences were observed in Cu ion-treated groups in this study. A significant suppression in the body weight gain was observed in the 1,250 mg/kg group of male rats and the 2,500 mg/kg group of female rats treated with Cu NPs, while rats treated with Cu ions showed a slight/significant suppression in the body weight gain at ≥312 mg/kg for both sexes (). Treatment-related clinical signs, including soft feces, diarrhea, piloerection, hematuria, depression, paleness, prone position, nasal discharge, and death, were observed during the test period (data not shown). The rats treated with Cu NPs showed these signs at ≥1,250 mg/kg, whereas Cu ion-treated rats showed similar toxic signs at ≥625 mg/kg of both sexes from test day 0 through day 10.
Figure 5 Mortality and body weight changes of Cu NP- and Cu ion-treated rats.
Notes: (A and B) Survival rate and (E and F) body weight changes of male and female rats, respectively, treated with single oral dose of Cu NPs (312–2,500 mg/kg). (C and D) Survival rate and (G and H) body weight changes of male and female rats, respectively, treated with Cu ions (156–1,250 mg/kg). Values are presented as mean ± SD. *P<0.05, **P<0.01 vs vehicle control. Cu ions, copper(II) chloride.
Abbreviations: Cu NPs, copper nanoparticles; LD50, median lethal dose; SD, standard deviation.
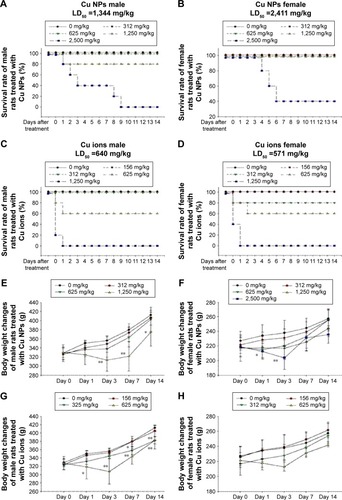
Histopathology
The histopathological changes were observed in the liver, kidneys, and spleen of male rats treated with Cu NPs at 1,250 mg/kg and female rats treated with Cu NPs at 2,500 mg/kg. These findings were also observed in both sexes of the rats treated with Cu ions at 625 mg/kg. Cu NP-treated rats showed mild inflammatory cell infiltration and dilated sinusoids in the liver (), mild-to-moderate degree of inflammatory cell infiltration, hyaline cast/cell debris in tubules, dilated tubules, atrophy of glomeruli in the kidneys, and multinucleated cells in the spleen. The incidence and severity of these findings in male rats were higher than those in female rats. These histopathological changes were also observed in the Cu ion-treated rats, except for the formation of multinucleated cells in the spleen.
Figure 6 Histopathological results from the liver, kidney, and spleen of rats treated with Cu NPs and Cu ions.
Notes: The liver from rats treated with Cu NPs (males for 1,250 mg/kg and females for 2,500 mg/kg) shows mild inflammatory cell infiltration (closed arrows), vacuolation (open arrows), and sinusoid dilation (open arrowheads). The rats treated with Cu ions show similar histopathological alterations at 625 mg/kg in both sexes (×200). The histological changes of kidneys from rats treated with Cu NPs include inflammatory cell infiltration (closed arrows), hyaline cast (closed arrowheads) and cell debris (asterisks) in tubules, dilated tubules (open arrowheads), and atrophy of glomeruli (open arrows). Kidneys treated with Cu ions exhibit mild dilated tubules and cast in tubules (cortex, ×100; medulla, ×200). The spleen of rats treated with Cu NPs shows mild decrease in cellularity in white pulp and increase in multinucleated giant cells (megakaryocytes; open arrows). Spleen of rats treated with Cu ions has no histological changes (×100). Hematoxylin and eosin stain. Scale bar =50 µm. Cu ions, copper(II) chloride.
Abbreviation: Cu NPs, copper nanoparticles.
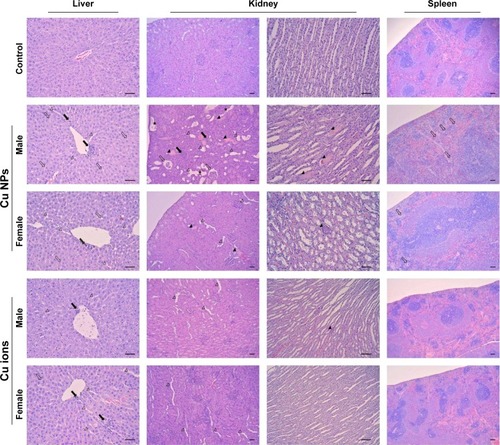
Serum biochemistry
The serum biochemistry results for Cu NP- and Cu ion-treated rats are presented in and . In the Cu NP-treated rats, the serum levels of AST, ALT, blood urea nitrogen, TCHO, and LDH in male rats and CPK and LDH in female rats increased significantly, while TG, Na, and Cl levels of male rats decreased significantly in the 1,250 mg/kg group when compared with those of the control group. A significant increase in the levels of AST, ALT, CPK, TCHO, total protein, and LDH and a significant decrease in the levels of TG, K, and Cl were also observed in female rats in the 2,500 mg/kg group (). In the Cu ion-treated rats, CPK and LDH levels increased significantly, while TG levels decreased significantly in male rats in the 312 mg/kg group when compared with those of the control group. The serum levels of AST, alkaline phosphatase, CPK, and LDH in male rats and CPK and LDH levels in female rats increased significantly, whereas the TG levels in male rats decreased significantly in the 625 mg/kg group when compared with those of the control group ().
Figure 7 Serum biochemical changes of Cu NP-treated rats at dose levels of 0 mg/kg, 312 mg/kg, 625 mg/kg, 1,250 mg/kg, and 2,500 mg/kg.
Notes: The bar graphs present serum biochemical changes of Cu NP (A–O)-treated male and female rats. Values are presented as mean ± SD. *P<0.05, **P<0.01 vs vehicle control.
Abbreviations: ALB, albumin; ALP, alkaline phosphatase; ALT, alanine aminotransferase; AST, aspartate aminotransferase; BUN, blood urea nitrogen; CPK, creatine phosphokinase; CRE, creatinine; Cu NPs, copper nanoparticles; LDH, lactate dehydrogenase; TBIL, total bilirubin; TCHO, total cholesterol; TG, triglyceride; TP, total protein; SD, standard deviation.
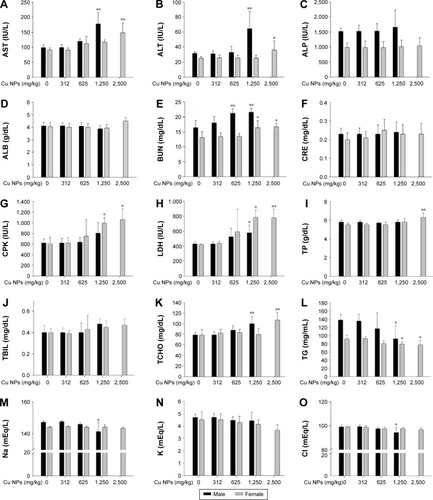
Figure 8 Serum biochemical changes of Cu ion-treated rats at dose levels of 0 mg/kg, 156 mg/kg, 312 mg/kg, and 625 mg/kg.
Notes: The bar graphs show serum biochemical changes of Cu ion (A–O)-treated male and female rats. Values are presented as mean ± SD. *P<0.05, **P<0.01 vs vehicle control. Cu ions, copper(II) chloride.
Abbreviations: ALB, albumin; ALP, alkaline phosphatase; ALT, alanine aminotransferase; AST, aspartate aminotransferase; BUN, blood urea nitrogen; CPK, creatine phosphokinase; CRE, creatinine; LDH, lactate dehydrogenase; TBIL, total bilirubin; TCHO, total cholesterol; TG, triglyceride; TP, total protein; SD, standard deviation.
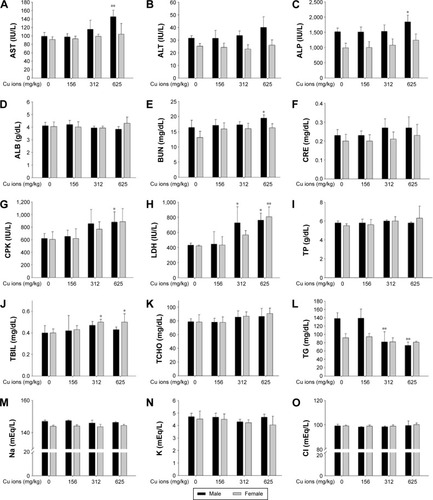
Organ weights
Organ weights obtained in the study for male and female rats treated with Cu NPs are presented in . In male rats, the relative weight of seminal vesicles in the 625 mg/kg group decreased significantly when compared with that of control group. The absolute and relative weights of the kidneys and the relative weights of the liver, lung, and testes in the 1,250 mg/kg group increased significantly, whereas the absolute and relative weights of seminal vesicles and the absolute weights of the heart, thymus, and epididymides decreased significantly when compared with those of the control group. In female rats, the absolute and relative weights of thymus in the group treated with Cu NPs at 2,500 mg/kg decreased significantly, whereas the relative kidney weight increased significantly when compared with that of the control group. The organ weight changes of rats in the Cu ion-treated groups are presented in . The relative weights of kidneys in male rats in the group treated with Cu ions at 625 mg/kg increased significantly when compared with that of the control group. In female rats, the absolute and relative weights of the spleen in the group treated with Cu ions at 625 mg/kg decreased significantly, whereas the relative weights of thymus and kidneys increased significantly when compared with those of the control group.
Discussion
Nanomaterials have recently received increasing attention because of their distinctive physicochemical properties and great prospects for use in a wide range of applications. However, their unique physicochemical, electrical, optical, and biological properties may dominate their toxicological behavior in vivo.Citation4,Citation42 In addition, physiological milieus influence the interaction of biological systems with NPs and can determine the fate and biosafety of NPs.Citation7 Thus, comprehensive characterization of the NPs in physiological conditions is a fundamental step prior to assessing the in vivo toxicity of Cu NPs. The hydrodynamic diameter and zeta potential of Cu NPs indicated that Cu NPs have a tendency to aggregate/agglomerate in vehicle (pH 6.5) and simulated intestinal (pH 7.8) conditions. However, their hydrodynamic diameter in simulated acidic conditions (pH 1.5) could not be determined, since Cu NPs rapidly dissolved in acidic conditions and the particle number of Cu NPs was not enough to determine the hydrodynamic diameter by dynamic light scattering analysis. The current paradigm for toxicity of metal nanomaterials is the release of toxic ions when thermodynamic properties of a material favor particle dissolution in a suspending medium or biological milieu.Citation43,Citation44 The dissolution of NPs and release of potential toxic ions are dependent on pH, composition, and exposure duration.Citation45 The dissolution in gastrointestinal fluids may help predict the uptake and blood concentrations.Citation46 It has been reported that Cu NPs or CuO NPs have high solubility in acidic milieu (pH 5.5) with high positive zeta potential.Citation25,Citation35 Thus, the high dissolution and zeta potential of Cu NPs in acidic conditions reflect a considerable interaction of Cu NPs within acidic milieu simulating the stomach.
In vivo biodistribution of Cu NPs can provide essential information regarding their absorption, accumulation sites, and clearance. Although the kinetics in serum, kidney, and urine of Cu NPs or Cu ions in mice have been reported,Citation35 there were limited data for biokinetics after Cu NPs on Cu ions exposure. Thus, we explored the further evaluation of the tissue biodistribution in the liver, spleen, lung, brain, and heart for 4-week period after single oral exposure. In the multistep process of NPs’ uptake, the physicochemical features of NPs (particle size, surface area, aggregation state, surface charge, etc) are likely to influence the degree of absorption and effects of administered NPs.Citation47,Citation48 The rate of dissolution also affects the absorption and biological response of NPs.Citation46 Comparative kinetic study of zinc oxide (ZnO) and titanium dioxide (TiO2) NPs revealed that the higher absorption and more extensive organ distribution of ZnO NPs might be due to their higher dissolution rate in acidic gastric fluid than TiO2 NPs, which have minimal dissolution in acidic milieu.Citation49 It has been reported that Cu levels in serum and kidney of nano-Cu (75 mg/kg)-treated mice are elevated at 24 hours and remain at the higher level until 72 hours when compared to equivalent Cu mass of ion-Cu (147.6 mg/kg, equivalent 70 mg/kg on Cu mass).Citation35 Considering the Cu mass of Cu ions (236 mg/kg, 47.2% of 500 mg/kg) used in this study, the absorbed Cu levels of Cu NP-treated groups showed slow increase and low uptake when compared with those of Cu ion-treated rats. Tissue distribution of Cu was similar to the absorption rate of Cu from Cu NPs and Cu ions. The highest levels of Cu in tested organs of Cu NP-treated rats were 15%–25% lower than those of Cu ions. The complete dissolution of Cu ions in vehicle (pH 6.5) suggested that dissociated Cu ions in suspension were readily absorbed from gastrointestinal tract. Eventually, Cu level in the blood and tested organs of Cu ions rapidly reached the highest level, which was much higher than that of Cu NPs at early time points. Cu NPs can react dramatically with hydrogen ions (H+) of gastric juice and transform into ionic states.Citation17,Citation35 Because the pH of gastric fluid is between 1.5 and 2.0, the NPs may be dissolved in the stomach, and the dissociated Cu ions are able to be absorbed into the systemic circulation following oral administration. However, Cu NPs were scarcely dissolved and easily aggregated/agglomerated with low zeta potential in vehicle solution, which affected the dissolution of Cu NPs in gastric environment when administered orally. In addition, high dose of NPs increases the potential for aggregation, subsequently decreasing the absorption of NPs from gastrointestinal tract.Citation46 Thus, aggregation of NPs in gastrointestinal tract can lead to decreased absorption.Citation50,Citation51 In view of this point, low and delayed uptake of Cu dissociated from Cu NPs may be due to increased aggregation and subsequent decreased dissolution of Cu NPs in physiological milieu that attributed to slow increase and less accumulation of Cu in organs. However, further studies will be necessary to investigate whether Cu NPs are absorbed as an entity under intestinal conditions or whether dissolved Cu ions are redeposited in the tissues.
Ingested Cu ions are mainly metabolized in the liver, and the major excretory route is liver/bile.Citation52,Citation53 As described earlier, Cu NPs react with H+ of gastric juice and can be transformed into ionic sates when they are administered orally.Citation17 After gastric emptying, the dissociation of Cu NPs is inhibited in basic milieu of small intestine, and then unabsorbed NPs are excreted in feces.Citation35 Thus, our findings suggest that most of the absorbed Cu dissociated from Cu NPs or unabsorbed Cu NPs is mainly excreted through feces, while a small amount of Cu NPs is eliminated through urine.
The oral LD50 value of nano-Cu (23.5 nm) is determined as 413 mg/kg, which is 3.8-fold higher than that of ion-Cu (110 mg/kg) in mice.Citation17 We confirmed that Cu NPs exerted lower toxic responses compared with Cu ions. The toxic responses of Cu NPs also showed clear sex-related difference at dose levels that produced changes in serum biochemistry, histology, and organ weights. As the males can secrete more H+ in stomach, more nano-Cu could be transformed into Cu ions in the male mice than in female mice. Thus, male mice exhibited more severe toxic symptoms than female mice after exposure to the same mass of nano-Cu.Citation17 These findings suggested that toxic effects of Cu NPs could be attributed to their transformation into ionic Cu states and Cu NPs were more easily transformed into Cu ions in male rats than in female rats. Thus, the different dissolution of Cu NPs and Cu ions in physiological conditions can probably explain the differences in in vivo toxicity profiles between Cu NPs and Cu ions. However, further study is necessary to determine the exact mechanism of sex-related difference in toxicity of Cu NPs.
Single or short-term exposure to Cu NPs can cause morphological changes in the liver, kidneys, and spleen with various histopathological alterations.Citation17,Citation33 Repeated oral administration of Cu NPs to rats at 200 mg/kg/d for 5 days caused necrosis of hepatocytes and renal proximal tubules accompanied with hepatic and renal dysfunction.Citation33,Citation34 In this study, a single oral dose of Cu NPs at 1,250 mg/kg in male rats and 2,500 mg/kg in female rats caused significant adverse effects on the histology of kidneys, liver, and spleen but not heart and lung. These histopathological changes were well supported by organ weight changes, including increased liver and kidney weights and decreased spleen weight. Additionally, the increment or decrement in serum biochemical levels exhibited a clear dose–response relationship and was consistent with the damage of kidney and liver. These changes were also observed in Cu ion-treated rats at ≥312 mg/kg in both sexes. Given that the accumulated Cu in organs may act as toxic reservoirs, these findings indicated that major organs affected by the Cu NPs in rats were liver, kidneys, and spleen, which were well correlated with the biodistribution of Cu. Collectively, these results support the hypothesis that toxicity profiles and kinetics of Cu NPs are closely associated with their dissolution rate in the physiological milieu, which involved a clear sex difference and less toxic response for Cu NPs compared to Cu ions.
Conclusion
Physicochemical analysis suggests that Cu NPs in vehicle or simulated intestinal milieu show a tendency of aggregation/agglomeration with low solubility. However, Cu NPs are dissolved in acidic conditions with high positive charge but less dissolution compared with Cu ions. These findings imply that interaction between physicochemical characters of NPs and biological system may affect their toxicity and behavior in vivo. Kinetic study demonstrates that Cu levels in blood and tested organs from Cu NPs show delayed increase and lower increase than that observed for Cu ions. However, absorbed or accumulated Cu from Cu NPs exhibits slower decrease in blood and tested organs than Cu ions. Unabsorbed Cu NPs or absorbed Cu dissociated from Cu NPs was primarily eliminated through feces. In vivo toxicity of Cu NPs occurs at much higher doses than that of Cu ions to elicit similar response with obvious sex-related differences. Furthermore, Cu NPs cause systemic toxic effects with functional and morphological changes in the liver, kidneys, and spleen. Collectively, by comparison with their ionic form, in vivo toxicity of Cu NPs is most likely correlated with their solubility in the physiological milieu and their biodistribution.
Disclosure
The authors report no conflicts of interest in this work.
Acknowledgments
This research was supported by Basic Science Research Program through the National Research Foundation of Korea funded by the Ministry of Education, Science and Technology (NRF-2013R1A1A2010835). The animal experiment in this study was supported by the Animal Medical Institute of Chonnam National University.
Supplementary materials
Table S1 Absolute and relative organ weights in rats treated with Cu NPs
Table S2 Absolute and relative organ weights in rats treated with Cu ions
References
- BhattacharyaSAlkharfyKMJanardhananRMukhopadhyayDNanomedicine: pharmacological perspectivesNanotechnol Rev201213235253
- BouwmeesterHDekkersSNoordamMYReview of health safety aspects of nanotechnologies in food productionRegul Toxicol Pharmacol2009531526219027049
- SuhWHSuslickKSStuckyGDSuhYHNanotechnology, nanotoxicology, and neuroscienceProg Neurobiol200987313317018926873
- AillonKLXieYEl-GendyNBerklandCJForrestMLEffects of nanomaterials physicochemical properties on in vivo toxicityAdv Drug Deliv Rev200961645746619386275
- KangMLimCHHanJHComparison of toxicity and deposition of nano-sized carbon black aerosol prepared with or without dispersing sonicationToxicol Res201329212112724278638
- NelAXiaTMadlerLLiNToxic potential of materials at the nano-levelScience2006311576162262716456071
- YallapuMMChauhanNOthmanSFImplications of protein corona on physico-chemical and biological properties of magnetic nanoparticlesBiomaterials20154611225678111
- JohnsonBMFraiettaJAGraciasDTAcute exposure to ZnO nanoparticles induces autophagic immune cell deathNanotoxicology20159673774825378273
- BormPJARobbinsDHauboldSThe potential risks of nanomaterials: a review carried out for ECETOCPart Fibre Toxicol200631116907977
- GaldieroSFalangaAVitielloMCantisaniMMarraVGaldieroMSilver nanoparticles as potential antiviral agentsMolecules201116108894891822024958
- OberdörsterGStoneVDonaldsonKToxicology of nanoparticles: a historical perspectiveNanotoxicology200711225
- JangGHHwangMPKimSYJangHSLeeKYA systematic in-vivo toxicity evaluation of nanophosphor particles via zebrafish modelsBiomaterials201435144044924094937
- TurnlundJRCopperShilsMEOlsonJAShikeMRossACModern Nutrition in Health and DiseaseBaltimore, MDLippincott Williams and Wilkins1999241252
- UauyROlivaresMGonzalezMEssentiality of copper in humansAm J Clin Nutr199867suppl 5S952S959
- BjörnPZHermannHDMaxLHeideSBarabaraKGHartmutDEpidemiological investigation on chronic copper toxicity to children exposed via the public drinking water supplySci Total Environ20033021–312714412526904
- GalhardiCMDinizYSFaineLAToxicity of copper intake: lipid profile, oxidative stress and susceptibility to renal dysfunctionFood Chem Toxicol200442122053206015500942
- ChenZMengHXingGMAcute toxicological effects of copper nanoparticles in vivoToxicol Lett2006163210912016289865
- DonaldsonKLiXYMacNWUltrafine (nanometer) particle mediated lung injuryJ Aerosol Sci1998295–6553560
- WarheitDBLaurenceBRReedKLRoachDHReynoldsGWebbTRComparative pulmonary toxicity assessment of single-wall carbon nanotubes in ratsToxicol Sci200477111712514514968
- Ebrahimnia-BajestanENiazmandHDuangthongsukWWongwisesSNumerical investigation of effective parameters in convective heat transfer of nanofluids flowing under a laminar flow regimeInt J Heat Mass Transf20115419–2043764388
- BondarenkoOJugansonKIvaskAKasemetsKMortimerMKahruAToxicity of Ag, CuO and ZnO nanoparticles to selected environmentally relevant test organisms and mammalian cells in vitro: a critical reviewArch Toxicol20138771181120023728526
- DasRGangSNathSSBhattacharjeeRLinoleic acid capped copper nanoparticles for antibacterial activityJ Bionanosci2010418286
- Esteban-TejedaLMalpartidaFEsteban-CubilloAPecharromanCMoyaJSAntibacterial and antifungal activity of a soda-lime glass containing copper nanoparticlesNanotechnology2009205050570119907067
- IngleAPDuranNRaiMBioactivity, mechanism of action, and cytotoxicity of copper-based nanoparticles: a reviewAppl Microbiol Biotechnol20149831001100924305741
- ChoWSDuffinRPolandCADifferential pro-inflammatory effects of metal oxide nanoparticles and their soluble ions in vitro and in vivo; zinc and copper nanoparticles, but not their ions, recruit eosinophils to the lungsNanotoxicology201261223521332300
- FahmyBCormierSACopper oxide nanoparticles induce oxidative stress and cytotoxicity in airway epithelial cellsToxicol In Vitro20092371365137119699289
- KahruASavolainenKPotential hazard of nanoparticles: from properties to biological and environmental effectsToxicology20102692–3899120176073
- PrabhuBMAliSFMurdockRCHussainSMSrivatsanMCopper nanoparticles exert size and concentration dependent toxicity on somatosensory neurons of ratNanotoxicology20104215016020543894
- RodheYSkoglundSWallinderIOPotacovaZMollerLCopper-based nanoparticles induce high toxicity in leukemic HL60 cellsToxicol In Vitro20152971711171926028147
- SiddiquiMAAlhadlaqHAAhmadJAl-KhedhairyAAMusarratJAhamedMCopper oxide nanoparticles induced mitochondria mediated apoptosis in human hepatocarcinoma cellsPLoS One201388e6953423940521
- XuJLiZXuPXiaoLYangZNanosized copper oxide induces apoptosis through oxidative stress in podocytesArch Toxicol20138761067107322903339
- KarlssonHLGustafssonJCronholmPMollerLSize-dependent toxicity of metal oxide particles – a comparison between nano- and micrometer sizeToxicol Lett2009188211211819446243
- LeiRHWuCQYangBHIntegrated metabolomic analysis of the nano-sized copper particle-induced hepatotoxicity and nephrotoxicity in rats: a rapid in vivo screening method for nanotoxicityToxicol Appl Pharmacol2008232229230118706438
- MannaPGhoshMGhoshJDasJSilPCContribution of nano-copper particles to in vivo liver dysfunction and cellular damage: role of IκBα/NF-κB, MAPKs and mitochondrial signalNanotoxicology20126112121319953
- MengHChenZXingGMUltrahigh reactivity provokes nanotoxicity: explanation of oral toxicity of nano-copper particlesToxicol Lett20071751–310211018024012
- ChunALWill the public swallow nanofood?Nat Nanotechnol200941279079119966821
- RashidiLKhosravi-DaraniKThe applications of nanotechnology in food industryCrit Rev Food Sci Nutr201151872373021838555
- WursterDEBurkeGMBergMJVeng-PedersenPSchotteliusDDPhenobarbital adsorption from simulated intestinal fluid, U.S.P., and simulated gastric fluid, U.S.P., by two activated charcoalsPharm Res1988531831863244631
- Organization for Economic Cooperation and Development [webpage on the Internet]Acute Oral Toxicity–Acute Toxic Class Method. Test Guideline No. 4232001 Available from: https://ntp.niehs.nih.gov/iccvam/suppdocs/feddocs/oecd/oecd_gl423.pdfAccessed February 5, 2016
- Organization for Economic Cooperation and Development [webpage on the Internet]Acute Oral Toxicity–Fixed Dose Procedure. Test Guideline No. 4202001 Available from: https://ntp.niehs.nih.gov/iccvam/suppdocs/feddocs/oecd/oecd_gl420.pdfAccessed February 5, 2016
- MillerLCTainterMLEstimation of the ED50 and its error by means of logarithmic-probit graph paperProc Soc Exp Biol Med1944572261264
- LanoneSBoczkowskiJBiomedical applications and potential health risks of nanomaterials: molecular mechanismsCurr Mol Med20066665166317022735
- FranklinNMRogersNJApteSCBatleyGEGaddGECaseyPSComparative toxicity of nanoparticulate ZnO, bulk ZnO, and ZnCl2 to a freshwater microalga (Pseudokirchneriella subcapitata): the importance of particle solubilityEnviron Sci Technol200741248484849018200883
- XiaTKovochichMLiongMComparison of the mechanism of toxicity of zinc oxide and cerium oxide nanoparticles based on dissolution and oxidative stress propertiesACS Nano20082102121213419206459
- XieYWilliamsNGTolicAAerosolized ZnO nanoparticles induce toxicity in alveolar type II epithelial cells at the air-liquid interfaceToxicol Sci2012125245046121964423
- BerginILWitzmannFANanoparticle toxicity by the gastrointestinal route: evidence and knowledge gapsInt J Biomed Nanosci Nanotechnol201331–2163210
- OberdörsterGMaynardADonaldsonKILSI Research Foundation/Risk Science Institute Nanomaterial Toxicity Screening Working GroupPrinciples for characterizing the potential human health effects from exposure to nanomaterials: elements of a screening strategyPart Fibre Toxciol200528
- AbbottLCMaynardADExposure assessment approaches for engineered nanomaterialsRisk Anal201030111634164420626687
- ChoWSKangBCLeeJKJeongJCheJHSeokSHComparative absorption, distribution, and excretion of titanium dioxide and zinc oxide nanoparticles after repeated oral administrationPart Fibre Toxicol201310923531334
- LoeschnerKHadrupNQvortrupKDistribution of silver in rats following 28 days of repeated oral exposure to silver nanoparticles or silver acetatePart Fibre Toxicol201181821631937
- LimCHHanJHChoHWKangMGStudies on the toxicity and distribution of indium compounds according to particle size in Sprague-Dawley ratsToxicol Res2014301556324795801
- TaoTYLiuFLKlompLWijmengaCGitlinDJThe copper toxicosis gene product Murrl directly interacts with the Wilson disease proteinJ Biol Chem200427843415934159612968035
- TurnlundJRHuman whole-body copper metabolismAm J Clin Nutr1998675960964