Abstract
To address the limitations of traditional drug delivery, TiO2 nanotubes (TNTs) are recognized as a promising material for localized drug delivery systems. With regard to the excellent biocompatibility and physicochemical properties, TNTs prepared by a facile electrochemical anodizing process have been used to fabricate new drug-releasing implants for localized drug delivery. This review discusses the development of TNTs applied in localized drug delivery systems, focusing on several approaches to control drug release, including the regulation of the dimensions of TNTs, modification of internal chemical characteristics, adjusting pore openings by biopolymer coatings, and employing polymeric micelles as drug nanocarriers. Furthermore, rational strategies on external conditions-triggered stimuli-responsive drug release for localized drug delivery systems are highlighted. Finally, the review concludes with the recent advances on TNTs for controlled drug delivery and corresponding prospects in the future.
Introduction
To address the limitations of conventional drug therapies related to restricted drug solubility, short circulating time, lack of selectivity, side effects, and unfavorable pharmacodynamics, considerable studies have been carried out in past years toward the development of more efficient drug delivery systems.Citation1–Citation4 Approximately 90% of drugs that are used currently on the market are hydrophobic and poorly water-insoluble, and this restricts their pharmacological efficiency and particularly challenges the systemic delivery route.Citation5,Citation6 Another inherent limitation of the therapies is that various drugs are inactivated or removed by the gastrointestinal system and other body organs including kidney and liver, and only <1% of administered drug molecules reach the site of interest, where higher dosages of drugs are required to achieve an optimized local concentration.Citation7,Citation8 Therefore, innovative drug delivery approaches are urgently needed to address the disadvantages of traditional drug delivery.
The inherent limitations of conventional therapies could be addressed on the basis of developing more efficient and rational drug delivery systems, in which two concepts involved in targeting drug delivery and localized drug delivery systems are verified to be the most perspective strategies. The utilization of nanotechnology to medicine is an emerging field with significant potential for localized drug delivery systems. Nanotechnology has boosted the development of various new nanomaterials and drug carriers for drug release applications, including polymer micelles, liposomes vesicle, multifunctional dendritic polymers, nanocapsules, nanospheres, TiO2 nanotubes (TNTs), and so on,Citation7,Citation9–Citation12 as shown in . For these nanoporous or nanotube carriers, a special niche in drug delivery technology has been guaranteed to correspond with them because of their simple preparation, controlled nanoporous or nanotube formation, mechanical rigidity, chemical resistivity, high loading capability, high surface area, and so on.Citation13–Citation21 TNT arrays formed on Ti surface by a facile electrochemical anodizing process are recognized as a desirable candidate to promote clinical therapeutic effect of medical implants.Citation22–Citation25
This paper aimed at reviewing TNTs used as carriers for controlled drug release and compiling the most recent advances on TNT-based drug-releasing implants for localized and smart drug delivery applications. Various methods designed to control sustained drug release from TNT implants are discussed, which include controlling TNT morphologies and chemical modification. Additionally, some advanced strategies on externally triggered stimuli-responsive drug release are discussed, and these sources hold significant potential of producing alternative drug release pathways that could overcome the limitations of the traditional diffusion mechanism. Finally, this review concludes a general overview on the future trends, challenges, and the prospective outlook for the interesting and promising research field.
Development of TNTs by electrochemical anodization
With the development of TNTs constructed by electrochemical anodizing, more and more attention is paid to achieve higher nanotube growth rates, improve controllable dimensions and nanotubes ordering.Citation19–Citation31 In order to improve the technology of TNT fabrication, various electrochemical protocols were addressed to support it, which involve aqueous and organic electrolytes with different chemical compositions and electrochemical conditions. The electrochemical anodization process is carried out usually in electrolytes containing some fluoride ions to fabricate TNT layers. In electrolytes with acid, salt, or alkali solution, short tube lengths were formed (from 400 nm to 2 μm) during anodization process, and these TNT arrays lacked organized structure and uniformity, which are described as the first generation of TNTs.Citation32–Citation35 Then TNTs of longer lengths (5–7 μm) were fabricated in a pH-controlled electrolyte containing small amounts of fluoride ions, which are considered as the second generation of TNTs.Citation36–Citation39 The third generation of TNT arrays with further longer length (1,000 μm) was fabricated in non-aqueous polar organic electrolytes in the presence of fluoride species by electrochemical anodization.Citation40–Citation43 Recently, non-fluoride-based synthesis of TNTs has been reported, which are addressed as the fourth generation, showing significant improvement in the growth rate and the quality of fabricated TNTs.Citation44–Citation46
For TNT arrays formed in fluoride-based electrolyte, an appropriate external voltage and low electrolyte acidity are needed for yielding TNT layers and growing long tubes. At a controlled anodization voltage of 80–120 V, electrochemical anodization of Ti results in vertically oriented and hexagonally closely packed TNT structures with high growth rate, optimal geometry, and stable mechanical characteristics.Citation1,Citation7 In addition, some other TiO2 morphologies were reported, such as nanopore branched, bamboo-type nanotubes, inner tubes, spongy, nanolace, and multilayer nanotubes were fabricated by controlling the voltage during the process of nanotube preparation.Citation47–Citation50 Thereby the study of TNT fabrication is a dynamic and active research area, and the development of novel strategies and synthesis methods is expected to be extended in the future.
Strategies to control drug delivery from TNTs
Drug delivery from nanotubes is dependent on the diffusion process when TNTs are implanted into the host body with physiological milieu. This diffusion process can be defined by Fick’s first law, which is influenced by various factors such as molecular size of the drugs, charge, dissolution rate and diffusion coefficient, dimensions of nanotubes, charge and surface chemistry, and interfacial interaction of drug molecules and TNT surface.Citation51–Citation55 Depending on these conditions, different drug release profiles were obtained and different strategies have been implemented into TNT-based systems in order to provide a controlled drug release for a broad range of clinical therapies. It is known that different drug release strategies need to be considered for different therapies, thus TNT-based drug-releasing systems must be designed with flexible drug release capabilities and optimized parameters in order to fulfill the requirements of different therapies. It is worthwhile stressing that zero-order type release is the most satisfactory release strategy for drug-releasing implants, which results in the drug being released at a uniform and constant rate independent of concentration and time.Citation56 Considering that, increasing studies are focused on exploring different strategies in TNT-based drug-releasing implants in order to design and advance their drug-releasing performances for specific drugs and therapies. A schematic diagram summarizing these strategies aimed at controlling the release of drugs from TNTs is presented in . In this schematic diagram, a single nanotube was subjected to various modifications for controlling drug release, including A) structural modifications of diameter and length of TNTs, B) surface modifications, C) adjusting pore openings of TNTs with polymer deposition, D) biodegradable polymer coatings, E) polymeric micelles as drug nanocarriers, and F) stimulated drug release strategies by external sources.
Figure 2 Strategies for controlling drug release from TNTs.
Notes: (A) Controlling the nanotube diameters and length; (B) surface chemistry (hydrophobic, hydrophilic, charged); (C) tuning nanotube opening by plasma polymerization; (D) degradation of dip-coated polymer film closing nanotubes (PLGA or chitosan); (E) using drug nanocarriers (micelles) for multidrug delivery; (F) delayed/sequential drug release of drugs/drug carriers. External field triggered drug release using (G) temperature, (H) magnetic field, (I) ultrasound, (J) light, and (K) radiofrequency with gold nanoparticles. Only single nanotube structure is shown to present an array of TNTs.
Abbreviations: APTES, 3-aminopropyl triethoxysilane; PLGA, poly (lactic-co-glycolic acid); TNT, TiO2 nanotube; d, diameter; l, length; 2-phos, 2-carboxyethyl-phosphonic acid; 16-phos, 16-phosphono-hexadecanoic acid; PFPTES, penta-fluorophenyldimethylchlorosilan; PNIPAAm, poly (N-isopropylacrylamide).
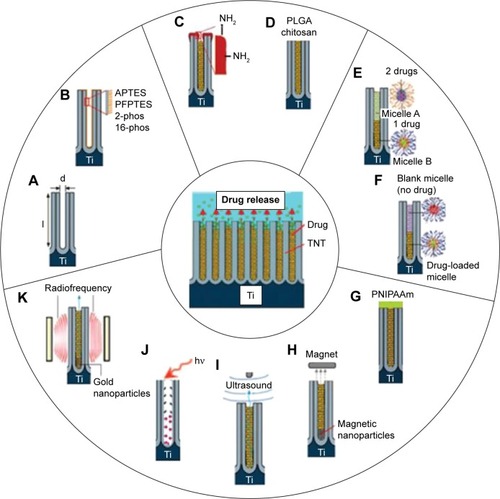
Dimensions of TNTs and surface functionalization
According to Fick’s law, the diffusion rate of drug molecules from nanopores depends on their dimensions as these confined nanochannels can be controlled precisely at nanoscale.Citation57,Citation58 To investigate the influence of pore size on the drug release profiles, studies from several groups confirmed that the drug release is considerably related to the dimensions of TNTs.Citation59–Citation62 Aw et al studied the relationship between the length of the TNTs and anodization time, and their results demonstrated that length of TNTs is controlled by anodization time and that the drug release time was extended with an increase in the nanotube length based on drug loaded in TNT implants of different nanotube lengths (25–100 μm) and a fixed pore diameter of 110 nm.Citation2 The drug release from TNTs can be extended by shrinking their lengths and diameters, which results from the fact that drug molecules encapsulated deeper inside TNTs needed a longer time to diffuse out of nanotubes because of the capillary force. In addition, Hamlekhan et al studied that anodization condition (voltage and duration) influences the release profiles of TNT groups based on the dimensions of TNTs influenced by anodization conditions.Citation59 As demonstrated by various studies, length and diameter of TNTs are increased with the increasing anodization voltage. Moreover, the amount of drug loaded in TNTs increases as the anodization duration is increased based on comparing the profiles with the TNT dimensions specified in all TNT groups, as presented in .Citation59
Figure 3 Concentration of drug released from TNTs anodized at (A) 60 V, (B) 70 V, (C) 90 V, and (D) 120 V.
Notes: The area of less than 30 min corresponds to active release stage. During this stage, most of the loaded drug is released from nanotubes into aqueous environment. Some groups of TNTs release the overall amount of the loaded drug in less than 15 min, while the other groups prolong release to about 1 h (marked by vertical dash line). Error bars show variance for n=3. Copyright IOP Publishing. Reproduced with permission. All rights reserved. Hamlekhan A, Sinha-Ray S, Takoudis C, et al. Fabrication of drug eluting implants: study of drug release mechanism from titanium dioxide nanotubes. J Phys D Appl Phys. 2015;48:275401. Published 10 June 2015.Citation59
Abbreviations: h, hours; min, minutes; d, days; TNT, TiO2 nanotube.
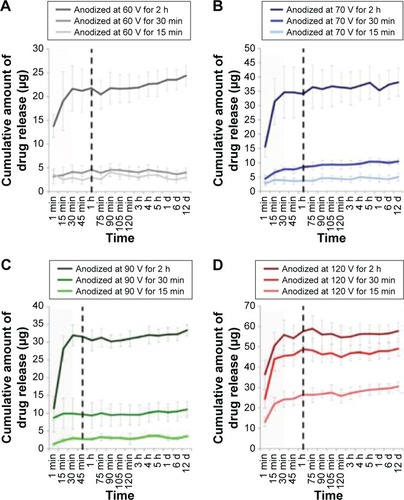
The next strategy for controlling drug release from TNTs was based on the surface functionalization of the nanotubes. The aim of this strategy is to dynamically change the interaction between drug molecules and inner walls of the nanotubes for altering the drug release kinetics. This approach was previously demonstrated on porous silica particles and was successfully translated into TNTs by using polymers and self-assembled monolayers with excellent stability and flexibility for surface modification.Citation63–Citation69 Organic silanes (ie, 3-aminopropyl triethoxysilane [APTES], penta-fluorophenyldimethylchlorosilan [PFPTES]) and phosphonic acids (ie, 2-carboxyethyl-phosphonic acid and 16-phosphono-hexadecanoic acid) were used to modify TNTs, which demonstrate that this approach could change drug loading and release characteristics of TNTs for hydrophilic and hydrophobic drugs, as shown in .Citation2 It is worthwhile stressing that APTES holds the silane group hydrophilic that can impart different properties onto the surface of TNTs.Citation70,Citation71 Moreover, APTES protonates can give similar properties of drugs in phosphate-buffered saline (PBS) with neutral pH.
Figure 4 Schemes showing the concept of chemical modification.
Notes: (A) Modification on TNTs by phosphonic acid using 2-carboxyethyl-phosphonic acid (2-phos) and 16-phosphono-hexadecanoic acid (16-phos); (B) drug release from 2-phos, 16-phos-modified TNTs and the control sample (unmodified, bare TNTs). Reproduced from Aw MS, Kurian M, Losic D. Non-eroding drug-releasing implants with ordered nanoporous and nanotubular structures: concepts for controlling drug release. Biomater Sci. 2014;2:10–34, with permission of The Royal Society of Chemistry, http://dx.doi.org/10.1039/C3BM60196J.Citation2
Abbreviations: h, hours; TNT, TiO2 nanotube.
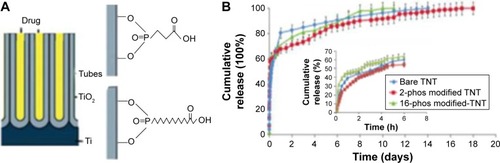
Based on the results presented above, it is demonstrated that drug loading and releasing features are significantly influenced by surface charge and chemical and interfacial properties. Specific surface modification strategy is useful for rational designing implants with splendid properties for optimized application, whereas this strategy is still limited to achieve a sustained release of drugs from TNTs for a longer duration.
Plasma polymerized biopolymer coating modifications
In order to overcome the problem that a long and sustained drug release cannot be realized by surface modification of TNTs, a new strategy using plasma polymer coatings on the top surface of TNTs to reduce the opening of nanopores, which confirmed that drugs release from TNTs is possible to follow the zero-order release kinetics.Citation72–Citation76 Various drugs with different size and properties have been successfully performed on TNTs based on this concept, and these drugs include anti-inflammatory drugs, anticancer drugs, functionalized metal oxide nanospheres, bovine serum albumin, and bone morphogenetic proteins (BMPs) drug micelles.Citation73,Citation77,Citation78 It is worthwhile noticing that plasma deposition method holds a capability of modifying the top surface of TNT layers, and the modified TNTs are imparted to desirable properties including antibacterial activity, implant rejection, anti-biofouling, and better integration to reduce sensing capabilities.Citation72 With the plasma deposition method being widely used in medical application, the disadvantages of this technology are also presented, such as the calibration requirement of plasma conditions, its high cost, and some unavailable technical knowledge.
Considering these limitations of the plasma deposition, a significantly simpler method with low cost was explored based on coating TNT opening.Citation60,Citation79 Poly (lactic-co-glycolic acid) (PLGA) and chitosan were selected for dip-coating, which result from two polymers with biocompatible and biodegradable possibilities and are known to have some beneficial properties including antibacterial and osseo-integration and are also used for drug delivery systems. PLGA or chitosan was coated on drug-loaded TNTs by dip-coating for controlling drug release and improving antibacterial and bone integration of TNTs, as schematically shown in .Citation80
Figure 5 Schematic diagram of TNTs implants loaded with drugs where the nanotubes were covered with ultrathin film of biodegradable polymer (PLGA or chitosan) using a simple dip-coating process.
Notes: Reprinted from Acta Biomater, Volume 8, Gulati K, Ramakrishnan S, Aw MS, Atkins GJ, Findlay DM, Losic D. Biocompatible polymer coating of titania nanotube arrays for improved drug elution and osteoblast adhesion, pages 449–456, Copyright 2012, with permission from Elsevier.Citation80
Abbreviations: PLGA, poly (lactic-co-glycolic acid); TNT, TiO2 nanotube.
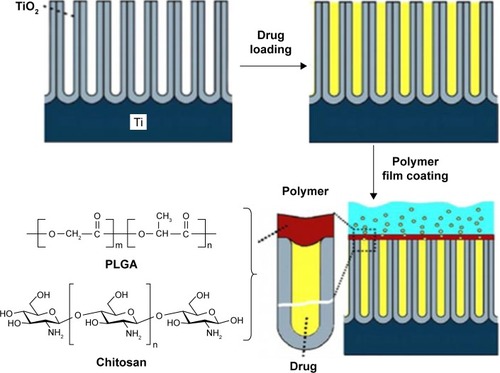
Significant changes in drug release profiles were observed because of coating a polymer film on openings of the nanotubes as shown in .Citation80 From the results, it was demonstrated that drug release profiles were significantly influenced by polymers’ chemical composition and the thickness of polymer layer. Meanwhile, the burst release occurred at the first 6 hours is presented in release process regardless of a polymer film exited or not, which can be explained that the initial release is from drug loaded on TNTs’ top surface, and the high concentration gradient through nanotubes allows drugs’ rapid release at the beginning of release duration. In addition, it was also concluded that TNT arrays coated with a thin PLGA polymer layer shows an extended release duration with a higher level of burst release and that a thin chitosan layer coated on TNTs could provide a shorter release duration with a lower level of burst release.
Figure 6 Comparative drug release graphs of anti-inflammatory drug (indomethacin) from polymer-coated TNTs/Ti.
Notes: (A, B) Overall and burst drug release from uncoated TNTs/Ti and TNTs/Ti coated with thin and thick chitosan layers; (C, D) overall and burst drug release from uncoated TNTs/Ti and TNTs/Ti coated with thin and thick PLGA layers. Reprinted from Acta Biomater, Volume 8, Gulati K, Ramakrishnan S, Aw MS, Atkins GJ, Findlay DM, Losic D. Biocompatible polymer coating of titania nanotube arrays for improved drug elution and osteoblast adhesion, pages 449–456, Copyright 2012, with permission from Elsevier.Citation80
Abbreviations: min, minutes; PLGA, poly (lactic-co-glycolic acid); TNT, TiO2 nanotube.
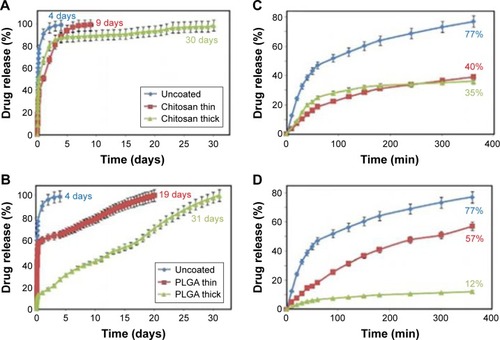
Form these results, it was demonstrated that the drug release can extend to several months with zero-ordered kinetics by controlling the thickness of the biopolymer film coated on TNTs. This design of TNT implants is focused on its local drug delivery with several weeks releasing, which has been performed by a study based on post-surgical implant surgeries, and its result indicates that systemically delivered gentamicin has fewer side effects in promoting bone healing.Citation81 The adhesion and proliferation of human osteoblastic cells on TNT implants were investigated to confirm polymer-coated TNTs, especially chitosan coating could enhance osteoblast integration to implants.Citation2 TNTs with dip-coating modification are believed to be achievable in a clinical environment because of their facile fabrication process and no specific technology requirements.
Advanced TNT drug systems with multi-drug and stimulated drug release
Multi-drug delivery with micelles as drug carriers from TNTs
Considering the treatment of some complex diseases that require more than one kind of drug, a new concept of using polymeric micelles for loading drugs was addressed, especially multi-drug nanocarriers were integrated into TNTs for designing implants with advanced multi-drug releasing. This advanced drug release strategy holds the capability of delivering several drugs for a pre-programmed time to permit a later release, as schematically presented in .Citation58 As the most desired candidates for this concept, hydrophobic and hydrophilic properties of drug carriers provide a desired structure of polymer layers in TNTs without inter-mixing, and a unique successive release pattern from TNTs can be achieved based on this multiple-drug delivery system. In terms of drug release profiles based on TNTs loaded with two layers drug carries, shows the sequential releasing of regular micelles (d-α-tocopheryl polyethylene glycol 1000 succina [TPGS]) encapsulating two hydrophobic drugs (indomethacin and itraconazole) loaded at the top, followed by the releasing of inverted micelles (PEGylated phospholipids [DGP]) encapsulating hydrophilic drugs (gentamicin) loaded at the bottom.Citation58
Figure 7 Scheme depicting the concept for controlling multiple drug release from TNTs.
Notes: (A) TNTs loaded with two types of polymer micelles, a regular micelle (TPGS) encapsulated with hydrophobic and an inverted micelle (DGP 2000) encapsulated with hydrophilic drug; (B) scheme of sequential drug release with layered drug carriers with details of two-step drug release in (C) and (D); (E) sequential and multiple release of drug carriers loaded with three drugs from TNTs. Reproduced from Aw MS, Addai-Mensah J, Losic D. A multi-drug delivery system with sequential release using titania nanotube arrays. Chem Commun. 2012;48:3348–3350, with permission of The Royal Society of Chemistry, http://dx.doi.org/10.1039/C2CC17690D.Citation58
Abbreviations: TNT, TiO2 nanotube; TPGS, d-α-tocopheryl polyethylene glycol 1000 succina; DGP, PEGylated phospholipids.
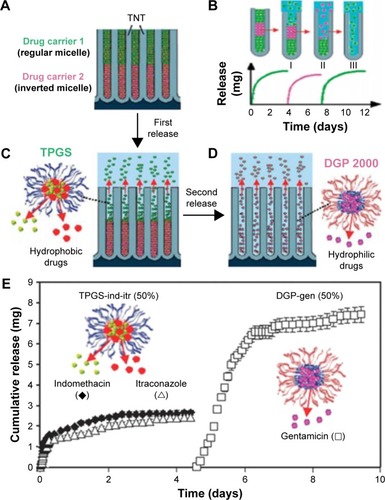
Compared with conventional drug carriers, polymeric micelles can enhance drug delivery system because of the prolonged therapeutic effects of drugs in targeted organs or tissues.Citation82 Considering the candidates of polymers, poly(ethylene oxide) (PEO)-poly(propylene oxide) (PPO)-PEO consisted of hydrophilic polar head (PEO) and copolymers-lipophilic alkyl tail (PPO) was used as drug carriers due to its organic chains with different number of tri-block monomers.Citation83 TPGS, a vitamin E derivative, can significantly improve drug encapsulation efficiency and increase the bioavailability of anticancer drugs, thus was selected as a drug carrier.Citation84,Citation85 Moreover, the PEGylated phospholipids (DGP 2000 and 5000) are also used in drug delivery system in broad commercial applications.Citation86
The multi-drug delivery system used in various local drug therapies presents sequential release of drug carriers by varying types of drug-loaded carriers and positions of carries in TNTs. Release profiles of this multi-drug delivery system can be controlled by adjusting the length and pore diameters of TNTs, surface properties of micelles and their loading conditions. Furthermore, this multi-drug delivery system fully satisfies complex requirements for bone therapies required over long periods to prevent inflammation and improve implant integration.
Stimulated drug delivery from TNTs with external trigger
Extended drug release for long-term therapies are not satisfied in critical situations such as unexpected onset of inflammation, sudden viral attack, osteomyelitis, and so on, where high concentrations of drug are immediately required. To settle these emergency conditions, a concept of stimulated drug delivery system with external trigger based on TNTs is put forward to achieve therapeutic efficacy.Citation7 Extensive investigations were explored to increase the treatment effect in the required time period, which sometimes needed high dosage with precise schedule or only for a short period to treat urgent diseases.Citation87,Citation88 For satisfying the demand of delivering pre-determined amount of drugs required by pharmacokinetic parameters, several concepts are reported, including magnetic, ultrasound, and voltage.
Magnetic-sensitive drug delivery
A concept of drug encapsulated in nanomagnetic structures was proposed, which focused on designing triggered drug delivery systems because the nanomagnetic structures possess exciting possibilities for magnetic field triggered drug release. Regarding this concept, Shrestha et al reported on using TNTs filled with magnetic nanoparticles (MNPs) in order to achieve magnetic- and photocatalytic-guided release of drugs.Citation89 In this study, a model drug was attached to magnetic TNTs by using a silane coupling agent as a cross-linker, then ultraviolet (UV) irradiation was provided for inducing chain scission of this agent monolayer attached to TNTs, thus the release of the fluorescent molecule takes place at the anchoring siloxane groups as schematically outlined in . It was demonstrated by the phenomenon that the release of the fluorescent marker into the electrolyte was clearly visible after few seconds of UV irradiation imparted to dye-functionalized TNTs, as presented in .Citation89 From these results, it is shown that the approach reported here can realize a temporally and spatially photoinduced drug release based on magnetic-sensitive drug delivery system.
Figure 8 Schematic representation of the model drug release from TNTs.
Notes: (A) The release principle of active molecules (model drug) from the functionalized magnetic TNTs upon irradiation with UV light. The release of the fluorescent dye into the surrounding system by the dye-functionalized magnetic TNTs with UV light was “off” (B) and “on” (C). The movement of the tube layers in water was guided by a permanent magnet underneath the petri dish. Reproduced from Shrestha NK, Macak JM, Schmidt-Stein F, et al. Magnetically guided titania nanotubes for site-selective photocatalysis and drug release. Angew Chem Int Edit. 2009;48:969–972. Copyright © 2009 WILEY-VCH Verlag GmbH & Co. KGaA, Weinheim.Citation89
Abbreviations: TNT, TiO2 nanotube; UV, ultraviolet.
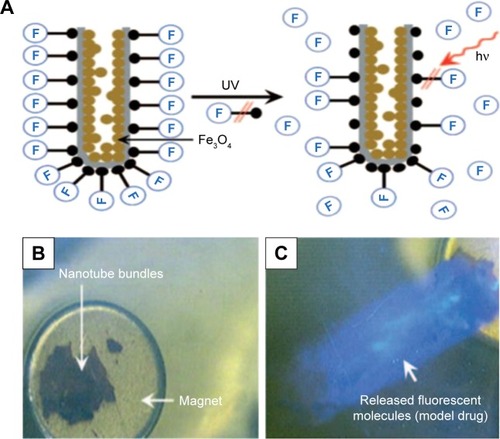
In addition, a new concept was addressed, aiming to design drug-releasing implants being assisted by MNPs loaded inside TNTs.Citation90 In this study, iron oxide MNPs (DOPA-Fe3O4) with dopamine modification was used for improving the biocompatibility of the MNPs and their loading inside the TNTs. Considering drug carriers, three types of amphiphilic micelles including Pluronic F127, TPGS, and PEO-PPO-PEO were explored to study the concept of magnetic-sensitive drug delivery system. For the drug-release profiles, it is confirmed that cumulative release of the three drug carriers reaches ~100% within 1–1.5 hours under the application of the magnetic field.Citation90 Although this strategy also presents some limitations of uncontrolled release triggered by external magnetic fields from the environment, it is still particularly valuable for drug-releasing implants in orthopedics and bone surgery where on-demand release is required under emergency situation.
Ultrasound-sensitive drug delivery
In order to overcome the drawbacks of magnetic field-stimulated release, the drug-releasing system based on ultrasound-mediated drug and nanocarrier release from TNTs was explored. Aw et al reported the application of local ultrasonic external field for triggering drug release from TNTs.Citation91 It is the first time that ultrasound-mediated drug micelles are selected for local drug delivery system, and shows this concept of using ultrasonic waves (USW) as a trigger combined with TNT implants. For controlling drug-micelles release from TNTs, several USW parameters were explored, including pulse length, amplitude, pulsation time, and power intensity. The USW power intensity controlled by various distance between probe and sample has a significant effect on the profile of drug release from TNTs as shown in . In this work, drug release profiles varies as the distance between the probe and sample is changed, for example, when the distance is set as 2.0, 1.5, 1.0, and 0.5 cm, it corresponds to the power of 25, 50, 75, and 100 W, as presented in . It is indicated that the distance between the probe and sample is shorter, the USW power intensity is greater, and the force of the impact becomes stronger. These effects may result from the fact the wave energy could propagate directly without much hindrance in the medium.
Figure 9 Ultrasound-stimulated drug release from TNTs.
Notes: (A) Drug delivery based on TNTs arrays as drug–drug carrier releasing platform and polymeric micelles as drug carriers; (B) effects of the distance between the probe and sample in buffer (2.0, 1.5, 1.0, and 0.5 cm) which corresponds to power measurement of 25, 50, 75, and 100 W, respectively: drug release profile; (C) release duration (min) as a function of Sonotrode distance from the sample. Reprinted from International Journal of Pharmaceutics, Volume 443, Aw MS, Losic D. Ultrasound enhanced release of therapeutics from drug-releasing implants based on titania nanotube arrays, pages 154–162, Copyright 2013, with permission from Elsevier.Citation91
Abbreviations: TNT, TiO2 nanotube; min, minutes; PBS, phosphate-buffered saline; TPGS, d-α-tocopheryl polyethylene glycol 1000 succina.
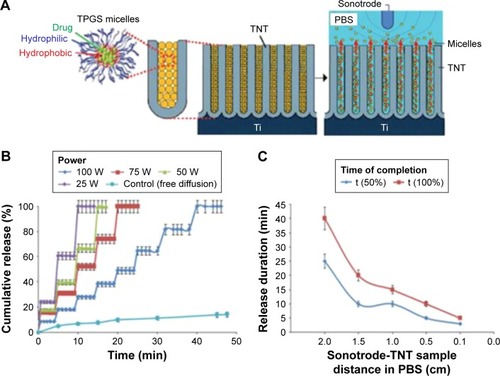
With regard to the mechanism of drug-micelles release from TNTs by USW, it is likely involved that a combination of thermal and cavitation processes caused by mechanical vibration result from forces produced by the ultrasound waves in interaction with buffer and TNT implants. The application of this strategy can be involved in bone therapies and local delivery systems including stents or brain drug delivery. However, more ex vivo or in vivo studies based on various drugs loaded inside drug-released TNT implants are required to demonstrate the feasibility of this concept.
Voltage-sensitive drug delivery
Among various stimuli-responsive drug delivery system approaches, the voltage-sensitive release is another attractive strategy for its beneficial properties. Impartation of voltage could induce the chain scission based on TNTs grafted with octadecylphosphonic acid for wettability or attached to an enzyme of horseradish peroxidase, as reported by Song et al.Citation92 In this study, a reaction of OH with terephthalic acid (TA) to form 2-hydroxyterephthalic acid (TAOH) results in a blue fluorescence at different voltages for identical duration as shown in .Citation92 The results indicate that the strong blue fluorescence is visible when TNTs are held at 5 V, whereas virtually no fluorescence can be seen at 1.5 V or no voltage. For these reasons, it is possible that generated valence-band holes can react with their environment in a similar manner as photogenerated holes in TNTs at a potential of 5 V. schematically shows voltage-induced OH⋅radical formation and payload release, which demonstrates voltage-induced pseudo photocatalytic processes, and in particular the valence band ionization mechanism may result in the chain scission reactions from TNTs.
Figure 10 Radical mechanism.
Notes: (A) Fluorescence testing of radical formation by reaction of terephthalic acid with anatase TNTs before voltage application and after 1.5 V and 5 V; (B) reaction scheme for voltage-induced OH⋅radical formation and payload release. Reproduced from Song YY, Roy P, Paramasivam I, Schmuki P. Voltage-induced payload release and wettability control on TiO2 and TiO2 nanotubes. Angew Chem Int Edit. 2010;49:351–354, Copyright © 2010 WILEY-VCH Verlag GmbH & Co. KGaA, Weinheim.Citation92
Abbreviations: TNT, TiO2 nanotube; ocp, open circuit potential.
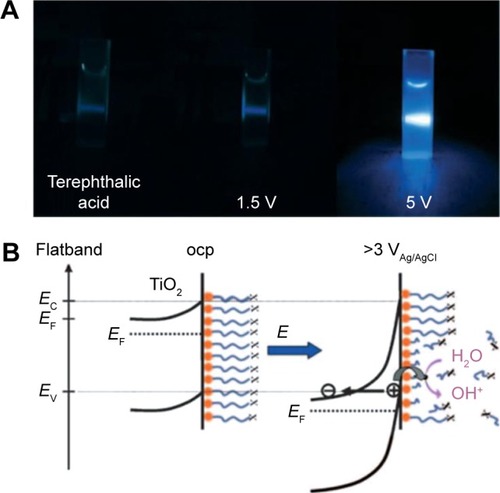
In addition, Sirivisoot et al reported an approach that was used to trigger drug release by an electrical field. In their study, drugs were encapsulated into multi-walled carbon nanotubes (MWCNTs) grown out of TNTs, where drugs release from TNTs under the control of electrical field.Citation93,Citation94 Their research achievement could be applied on treating bone repair and other more serious bone diseases. Furthermore, Sirivisoot et al carried out an experiment by doping polypyrrole with antibiotics (penicillin and streptomycin) and an anti-inflammatory drug (dexamethasone); their loading by electrodeposition inside MWCNTs grown on TNTs was considered as the further advancement of voltage-sensitive drug delivery.Citation95,Citation96 Although the development of this strategy is in its primary stage, it has an enormous potential for advanced developments in drug-released TNT implants.
Ex vivo and in vivo studies of drug release characteristics
Most of the aforementioned studies on drug release therapies of TNTs were performed through in vitro experiments using PBS as eluting medium. This situation is significantly different from real clinical circumstances that possess the real bone tissues and real biological environment, thereby many challenges are presented for in vivo applications, especially for how to accurately monitor the distribution of drug molecules from TNTs to the bone tissue.Citation7 For this challenge, implantable TNT-Ti wires were selected to be inserted into the bone for providing extended drug release as reported by Aw et al.Citation97 In this study, a TNT-Ti wire was inserted into a hole drilled through each bone core, fitting tightly into the bone core’s center, as shown in , and trabecular bone core with TNT-Ti wire inside the bone chamber was connected to perfusion pump that provides culture media to keep bone cells alive as shown in .Citation97 Bioluminescence images were captured at different times for the 5-day experiment on drug-release studies as presented in .Citation97 From these results, it is demonstrated that the model drug’s concentration in 3D bone matrix is increasing and changes in the concentration exist across all directions from TNT-Ti implants.Citation97 In brief, the TNT-Ti wire can be considered as a safe drug-releasing implant used in the local drug delivery system for bone therapies such as bone infection, bone inflammation, and even bone cancer.
Figure 11 Ex vivo study of transport of drug in bone released from TNTs wire implant.
Notes: (A) Photo of trabecular bone with embedded TNTs/Ti wire implant loaded with drug at the center of the bone core (side view). (B) Trabecular bone core with TNTs/Ti wire inside the bone chamber connected to perfusion pump that provides culture media to keep bone cells alive. Bioluminescence images of bone with inserted drug-releasing implant (based on a Ti wire with TNTs on the surface) for local drug delivery: distribution of released model drug (rhodamine B) taken at (C) 1, (D) 4, (E) 24, and (F) 120 h using the Xenogen IVIS® 100 (Caliper Life Sciences, Inc, Hopkinton, MA) in vivo imaging system. Adapted with permission of Dove Medical Press, from Characterization of drug-release kinetics in trabecular bone from titania nanotube implants, Aw MS, Khalid KA, Gulati K, et al. 2012;7:4883–4892; permission conveyed through Copyright Clearance Center, Inc.Citation97
Abbreviations: h, hours; TNT, TiO2 nanotube.
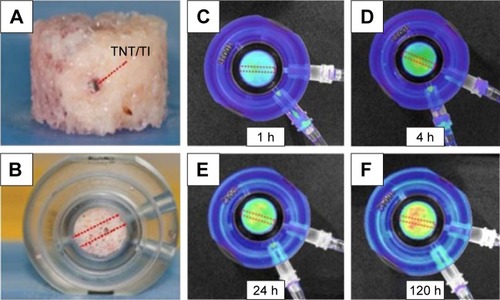
A suitable in vivo performance must be provided before any biomaterial is used in a real clinical application, thus TNTs have to integrate within the bone tissue and survive the stresses experienced during surgical insertion inside the animal model. As described in the previous section, von Wilmowsky et al used pigs for studying the in vivo performance of TNT-Ti implants.Citation98 In this study, investigations on peri-implant bone formation, bone–implant contact, and immunohistochemistry were performed for evaluating the effects of these implants, demonstrating that TNT coatings can enhance osteoblast functions, suppress shearing forces caused by implant insertion, and promote bone formation when compared to commercially available pure Ti implants. Another study reported by Park et al demonstrated that bone–implant contact results showed the capability of improving osseointegration for protein-loaded TNT implants based on loading fibroblast growth factor and human fibronectin fragment (hFNIII9–10) fusion protein inside TNTs on Ti implants which were inserted in rabbit tibia, followed up for 3 months.Citation99 It is worthwhile stressing that these experiments were carried out over a period of 2–3 months, and more studies should be carried out to investigate longer healing periods of TNTs implants for clinical therapies.
Apparently, these studies help establishing future databases consisting of detailed information on the degree of toxicity on the nanoscale, which would help to clarify the division of toxic effects of nanoscale materials, including TNTs. Furthermore, extensive studies on the interaction between cells/tissues from different organs and parts of the body with TNTs are also required.Citation100
Conclusion and future perspectives
Recent advances of drug-releasing TNT implants are reviewed in this work, and it is indicated that the application of TNTs is a promising alternative to develop various localized drug delivery systems that possess the capability to overcome limitations of systemic drug therapies. TNTs present beneficial properties for drug delivery application, including controllable nanotube dimensions, tunable geometries and surface chemistry, high surface area, high and versatile drug-loading capacity for several drugs, ability to modulate drug release kinetics, and so forth.
In this review, it is confirmed that TNT implants have a significant potential in clinical therapeutics, and capabilities of this implant can be realized by tuning their drug-releasing characteristics and providing multi-drug release of different drugs in different fashions. These approaches aim to optimize drug dosage, release rate, and time needed for a broad range of specific therapies, which have been presented in detail in this review. For these purposes, several strategies including magnetic, electromagnetic, and ultrasonic were used as triggers to release drugs from TNTs, which present outstanding features offering great perspectives and opportunities for TNT applications. Although still at initial stage, these external stimulus strategies are considered as promising applications in drug-releasing implants for developing smart clinical therapies.
Regarding the excellent biocompatibility of TNTs, numerous studies based on cells, ex vivo or in vivo animal models have been performed to prove their excellent biocompatibility. It is indicated that long-term toxicity assay and tolerability studies are needed to be performed on animals to evaluate the safety of blank TNTs and drug-loaded TNTs before proceeding with human clinical trials, thereby more in vivo studies are urgently required before these localized drug delivery systems can be applied in clinical trials.
Acknowledgments
The authors thank the National Natural Science Foundation of China (21501127; 51502185), Natural Science Foundation of the Jiangsu Higher Education Institutions of People’s Republic of China (15KJB430025), and Wenzhou government’s start-up fund (WIBEZD2014005-01; WIBEZD2014005-04). They also acknowledge the funds from the project of the Priority Academic Program Development of Jiangsu Higher Education Institutions (PAPD) and Project for Jiangsu Scientific and Technological Innovation Team (2013).
Disclosure
The authors report no conflicts of interest in this work.
References
- LosicDSimovicSSelf-ordered nanopore and nanotube platforms for drug delivery applicationsExpert Opin Drug Deliv200961363138119860534
- AwMSKurianMLosicDNon-eroding drug-releasing implants with ordered nanoporous and nanotubular structures: concepts for controlling drug releaseBiomater Sci201421034
- MainardesRMSilvaLPDrug delivery systems: past, present, and futureCurr Drug Targets2004544945515216911
- FahrALiuXDrug delivery strategies for poorly water-soluble drugsExpert Opin Drug Deliv2007440341617683253
- WolinskyJBColsonYLGrinstaffMWLocal drug delivery strategies for cancer treatment: gels, nanoparticles, polymeric films, rods, and wafersJ Control Release2012159142622154931
- PrakashSMalhotraMShaoWTomaro-DuchesneauCAbbasiSPolymeric nanohybrids and functionalized carbon nanotubes as drug delivery carriers for cancer therapyAdv Drug Delivery Rev20116313401351
- LosicDAwMSSantosAGulatiKBarianaMTitania nanotube arrays for local drug delivery: recent advances and perspectivesExpert Opin Drug Deliv20151210312725376706
- SantosAAwMSBarianaMKumeriaTWangYLosicDDrug-releasing implants: current progress, challenges and perspectivesJ Mater Chem B2014261576182
- VanDMcGuireTLangerRSmall scale systems for in vivo drug deliveryNat Biotechnol2003211184119114520404
- DuncanRNanomedicine gets clinicalNanotoday200581617
- AmijiMMNanotechnology for targeted drug and gene deliveryNanomedicine20062299300
- KayserOLemkeATrejoNHThe impact of nanobiotechnology on the development of new drug delivery systemsCurr Pharm Biotechno2005635
- WangSOrdered mesoporous materials for drug deliveryMicropor Mesopor Mat200911719
- WangGOtuonyeANBlairEADentonKTaoZAsefaTFunctionalized mesoporous materials for adsorption and release of different drug molecules: a comparative studyJ Solid State Chem200918216491660
- SonSJBaiXLeeSBInorganic hollow nanoparticles and nanotubes in nanomedicine: part 1. Drug/gene delivery applicationsDrug Discov Today20071265065617706547
- BiancoAKostarelosKPratoMApplications of carbon nanotubes in drug deliveryCurr Opin Chem Biol2005967467916233988
- KlumppCKostarelosKPratoMBiancoAFunctionalized carbon nanotubes as emerging nanovectors for the delivery of therapeuticsBiochim Biophys Acta2006175840441216307724
- LaiYKLinLXPanFBioinspired patterning with extreme wettability contrast on TiO2 nanotube array surface: a versatile platform for biomedical applicationsSmall201392945295323420792
- MartinCRKohliPThe emerging field of nanotube biotechnologyNat Rev Drug Discov20032293712509757
- SonSJBaiXNanAGhandehariHLeeSBTemplate synthesis of multifunctional nanotubes for controlled releaseJ Control Release200611414315216870299
- GeMZCaoCYLiSHIn-situ plasmonic Ag nanoparticles anchored TiO2 nanotube arrays as visible-light-driven photocatalysts for enhanced water splittingNanoscale201685226523426878901
- GulatiKKantKFindlayDLosicDPeriodically tailored titania nanotubes for enhanced drug loading and releasing performancesJ Mater Chem B2015325532559
- GeMZCaoCYHuangJYSynthesis, modification and photo/photoelectrocatalytic degradation applications of TiO2 nanotube arrays: a reviewNanotechnol Rev2016575112
- MoonKSBaeJMJinSOhSInfrared-mediated drug elution activity of gold nanorod-grafted TiO2 nanotubesJ Nanomater20142014750813
- SongYYSchmidt-SteinFBauerSSchmukiPAmphiphilic TiO2 nanotube arrays: an actively controllable drug delivery systemJ Am Chem Soc20091314230423219317500
- LaiYKHuangJYCuiZQRecent advances in TiO2-based nanostructures surfaces with controllable wettability and adhesionSmall2016122203222426695122
- GeMZCaoCYHuangJYA review of one-dimensional TiO2 nanostructures for environmental and energy applicationsJ Mater Chem A2016467726801
- ZhangYYJiangZLHuangJYTitanate and titania nanostructured materials for environmental and energy applications: a reviewRSC Adv201557947979510
- GeMZLiQSCaoCYOne-dimensional TiO2 nanotube photocatalysts for solar water splittingAdvanced Science20163 article 1600152
- JiaHKerrLLKinetics of drug release from drug carrier of polymer/TiO2 nanotubes composite-pH dependent studyJ Appl Polym Sci201513241750
- LiHQLaiYKHuangJYMultifunctional wettability patterns prepared by laser processing on superhydrophobic TiO2 nanostructured surfacesJournal of Materials Chemistry B20153342347
- ZwillingVDarque-CerettiEBoutry-ForveilleADavidDPerrinMYAucouturierMStructure and physicochemistry of anodic oxide films on titanium and TA6V alloySurf Interface Anal199927629637
- GrimesCASynthesis and application of highly ordered arrays of TiO2 nanotubesJ Mater Chem20071714511457
- MorGKVargheseOKPauloseMShankarKGrimesCAA review on highly ordered, vertically oriented TiO2 nanotube arrays: fabrication, material properties, and solar energy applicationsSol Energ Mat Sol C20069020112075
- LaiYKGaoXFZhuangHFHuangJYLinCJJiangLDesigning superhydrophobic nanostructures with tunable water adhesionAdv Mater20092137993803
- CaiQPauloseMVargheseOKGrimesCAThe effect of electrolyte composition on the fabrication of selforganized titanium oxide nanotube arrays by anodic oxidationJ Mater Res200520230236
- CheongYLYamFKNgSWHassanZNgSSLowIMFabrication of titanium dioxide nanotubes in fluoride-free electrolyte via rapid breakdown anodizationJ Porous Mat20152214371444
- PrakasamHEShankarKPauloseMVargheseOKGrimesAA new benchmark for TiO2 nanotube array growth by anodizationJ Phys Chem Lett200711172357241
- SyrekKKapusta-KolodziejJJaroszMSulkaGDEffect of electrolyte agitation on anodic titanium dioxide (ATO) growth and its photoelectrochemical propertiesElectrochim Acta2015180801810
- GeMCaoCLiSEnhanced photocatalytic performances of n-TiO2 nanotubes by uniform creation of p-n heterojunctions with p-Bi2O3 quantum dotsNanoscale20157115521156026084384
- LaiYPanFXuCFuchsHChiLIn situ surface-modification-induced superhydrophobic patterns with reversible wettability and adhesionAdv Mater2013251682168623208816
- RoyPBergerSSchmukiPTiO2 nanotubes: synthesis and applicationsAngew Chem Int Edit20115029042939
- PauloseMShankarKYoriyaSAnodic growth of highly ordered TiO2 nanotube arrays to 134 μm in lengthJ Phys Chem B2006110161791618416913737
- PauloseMPengLLPopatKCFabrication of mechanically robust, large area, polycrystalline nanotubular/porous TiO2 membranesJ Memb Sci2008319199205
- ZhangYFuWYangHSynthesis and characterization of TiO2 nanotubes for humidity sensingAppl Surf Sci200825455455547
- AllamNKGrimesCAFormation of vertically oriented TiO2 nanotube arrays using a fluoride free HCl aqueous electrolyteJ Phys Chem Lett20071111302813032
- BauerSKleberSSchmukiPTiO2 nanotubes: tailoring the geometry in H3PO4/HF electrolytesElectrochem Commun2006813211325
- AlbuSPKimDSchmukiPGrowth of aligned TiO2 bamboo-type nanotubes and highly ordered nanolaceAngew Chem Int Edit20084719161919
- AlbuSPGhicovAAldabergenovaSFormation of double-walled TiO2 nanotubes and robust anatase membranesAdv Mater20082041354139
- ChenBLuKHierarchically branched titania nanotubes with tailored diameters and branch numbersLangmuir2012282937294322188171
- JiaHKerrLLSustained ibuprofen release using composite poly (lactic-co-glycolic acid)/yitanium dioxide nanotubes from Ti implant surfaceJ Pharm Sci20131022341234823657983
- BrammerKSKimHNohKHighly bioactive 8 nm hydrothermal TiO2 nanotubes elicit enhanced bone cell responseAdv Eng Mater201113B88B94
- SimchiATamjidEPishbinFBoccacciniARRecent progress in inorganic and composite coatings with bactericidal capability for orthopaedic applicationsNanomedicine20117223921050895
- AninweneGEYaoCWebsterTJEnhanced osteoblast adhesion to drug-coated anodized nanotubular titanium surfacesInt J Nanomed20083257264
- MosekeCHageFVorndranEGbureckUTiO2 nanotube arrays deposited on Ti substrate by anodic oxidation and their potential as a long-term drug delivery system for antimicrobial agentsAppl Surf Sci201225853995404
- TicuE-LVercaigne-MarkoDFroidevauxRHumaAArtenieVGuillochonDUse of a protease-modified-alumina complex to design a continuous stirred tank reactor for producing bioactive hydrolysatesProcess Biochem20054028412848
- AwMSAddai-MensahJLosicDPolymer micelles for delayed release of therapeutics from drug-releasing surfaces with nanotubular structuresMacromol Biosci2012121048105222821826
- AwMSAddai-MensahJLosicDA multi-drug delivery system with sequential release using titania nanotube arraysChem Commun20124833483350
- HamlekhanASinha-RaySTakoudisCFabrication of drug eluting implants: study of drug release mechanism from titanium dioxide nanotubesJ Phys D Appl Phys201548275401
- AwMSGulatiKLosicDControlling drug release from titania nanotube arrays using polymer nanocarriers and biopolymer coatingBiomater Nanobiotech20112477484
- CaiKYJiangFLuoZChenXYTemperature-responsive controlled drug delivery system based on titanium nanotubesAdv Eng Mater201012B565B570
- ÇalışkanNBayramCErdalEKarahaliloğluZDenkbasEBTitania nanotubes with adjustable dimensions for drug reservoir sites and enhanced cell adhesionMater Sci Eng C201435100105
- WenLXDingHMWangJXChenJFPorous hollow silica nanoparticles as carriers for controlled delivery of ibuprofen to small intestineJ Nanosci Nanotechnol200663139314417048529
- AjamiEAguey-ZinsouKFFunctionalization of electropolished titanium surfaces with silane-based self-assembled monolayers and their application in drug deliveryJ Colloid Interface Sci201238525826722858398
- SlowingIIVivero-EscotoJLWuCWLinVSYMesoporous silica nanoparticles as controlled release drug delivery and gene transfection carriersAdv Drug Delivery Rev20086012781288
- LinCXQiaoSZYuCZIsmadjiSLuGQPeriodic mesoporous silica and organosilica with controlled morphologies as carriers for drug releaseMicropor Mesopor Mat2009117213219
- Gary-BoboMHocineOBrevetDCancer therapy improvement with mesoporous silica nanoparticles combining targeting, drug delivery and PDTInt J Pharm201242350951522178618
- LinaCXYuanPYuaCZQiaoSZLuGQCooperative self-assembly of silica-based mesostructures templated by cationic fluorocarbon/hydrocarbon mixed-surfactantsMicropor Mesopor Mat2009126253261
- AnglinaEJChengLYFreemanbWRSailorMJPorous silicon in drug delivery devices and materialsAdv Drug Delivery Rev20086012661277
- KämmererPWGabrielMAl-NawasBScholzTKirchmaierCMKleinMOEarly implant healing: promotion of platelet activation and cytokine release by topographical, chemical and biomimetical titanium surface modifications in vitroClin Oral Implants Res20122350451021435015
- WilliamsEHDavydovAVMotayedAImmobilization of streptavidin on 4H-SiC for biosensor developmentAppl Surf Sci201225860566063
- LosicDColeMADollmannBVasilevKGriesserHJSurface modification of nanoporous alumina membranes by plasma polymerizationNanotechnology20081924570421825829
- SimovicSLosicDVasilevKControlled drug release from porous materials by plasma polymer depositionChem Commun20104613171319
- SimovicSLosicDVasilevKControlled release from porous platformsPharm Technol2011356871
- NohKBrammerKSChoiCKimSHFrandsenCJJinSA new nano-platform for drug release via nanotubular aluminum oxideJ Biomate Nanobiotechnol20112226233
- SchmidGMaterials in nanoporous aluminaJ Mater Chem20021212311238
- NairLSLaurencinCTPolymers as biomaterials for tissue engineering and controlled drug deliveryAdv Biochem Eng Biotechnol2006102479017089786
- PeerDKarpJMHongSFarokhzadOCMargalitRLangerRNanocarriers as an emerging platform for cancer therapyNat Nanotechnol2007275176018654426
- GulatiKAtkinsGJFindlayDMLosicDNano-engineered titanium for enhanced bone therapyProc SPIE2013881216
- GulatiKRamakrishnanSAwMSAtkinsGJFindlayDMLosicDBiocompatible polymer coating of titania nanotube arrays for improved drug elution and osteoblast adhesionActa Biomater2012844945621930254
- AwMSSimovicSAddai-MensahJLosicDPolymeric micelles in porous and nanotubular implants as a new system for extended delivery of poorly soluble drugsJ Mater Chem20112170827089
- RapoportNPittWGSunHNelsonJLDrug delivery in polymeric micelles: from in vitro to in vivoJ Control Release200391859512932640
- LiuLYongK-TRoyIBioconjugated pluronic triblock-copolymer micelle-encapsulated quantum dots for targeted imaging of cancer: in vitro and in vivo studiesTheranostics2012270571322896772
- MuthuMSKulkarniSALiuYFengS-SDevelopment of docetaxel-loaded vitamin E TPGS micelles: formulation optimization, effects on brain cancer cells and biodistribution in ratsNanomedicine2012735336422329606
- XuWLingPZhangTPolymeric micelles, a promising drug delivery system to enhance bioavailability of poorly water-soluble drugsJ Drug Deliv2013201334031523936656
- SawantRMHurleyJPSalmasoS“SMART” drug delivery systems: double-targeted pH-responsive pharmaceutical nanocarriersBioconjug Chem20061794394916848401
- SavonittoSUrbanoMDCaraccioloMUrgent surgery in patients with a recently implanted coronary drug-eluting stent: a phase II study of “bridging” antiplatelet therapy with tirofiban during temporary withdrawal of clopidogrelBr J Anaesth201010428529120047898
- HoHHLauTWLeungFTseH-FSiuC-WPeri-operative management of anti-platelet agents and anti-thrombotic agents in geriatric patients undergoing semi-urgent hip fracture surgeryOsteoporosis Int201021573577
- ShresthaNKMacakJMSchmidt-SteinFMagnetically guided titania nanotubes for site-selective photocatalysis and drug releaseAngew Chem Int Edit200948969972
- AwMSAddai-MensahJLosicDMagnetic-responsive delivery of drug carriers using titania nanotube arraysJ Mater Chem20122265616563
- AwMSLosicDUltrasound enhanced release of therapeutics from drug-releasing implants based on titania nanotube arraysInt J Pharm201344315416223313837
- SongYYRoyPParamasivamISchmukiPVoltage-induced payload release and wettability control on TiO2 and TiO2 nanotubesAngew Chem Int Edit201049351354
- SirivisootSParetaRAWebsterTJA conductive nanostructured polymer electrodeposited on titanium as a controllable, local drug delivery platformJ Biomed Mater Res A201199A586597
- SirivisootSParetaRWebsterTJElectrically controlled drug release from nanostructured polypyrrole coated on titaniumNanotechnology20112208510121242621
- SirivisootSYaoCXiaoXSheldonBWWebsterTJGreater osteoblast functions on multiwalled carbon nanotubes grown from anodized nanotubular titanium for orthopedic applicationsNanotechnology200718365102
- SirivisootSWebsterTJMultiwalled carbon nanotubes enhance electrochemical properties of titanium to determine in situ bone formationNanotechnology20081921232131
- AwMSKhalidKAGulatiKCharacterization of drug-release kinetics in trabecular bone from titania nanotube implantsInt J Nanomed2012748834892
- von WilmowskyCBauerSLutzRIn vivo evaluation of anodic TiO2 nanotubes: an experimental study in the pigJ Biomed Mater Res B200989B165171
- ParkJMKoakJYJangJHHanCHKimSKHeoSJOsseointegration of anodized titanium implants coated with fibroblast growth factor-fibronectin (FGF-FN) fusion proteinInt J Oral Maxillofac Implants20062185986617190295
- LiXWangLFanYFengQCuiFBiocompatibility and toxicity of nanoparticles and nanotubesJ Nanomater20122012548389