Abstract
Over the last several decades, a great number of advances have been made in the area of self-assembled supramolecules for regenerative medicine. Such advances have involved the design, preparation, and characterization of brand new self-assembled peptide nanomaterials for a variety of applications. Among all biomolecules considered for self-assembly applications, peptides have attracted a great deal of attention as building blocks for bottom-up fabrication, due to their versatility, ease of manufacturing, low costs, tunable structures, and versatile properties. Herein, some of the more exciting new designs of self-assembled peptides and their associated unique features are reviewed and several promising applications of how self-assembled peptides are advancing drug delivery, tissue engineering, antibacterial therapy, and biosensor device applications are highlighted.
Introduction
Grounded in nature, self-assembled molecules, involving lipids, peptides, proteins, sugars, and nucleic acids, are the fundamental building blocks of life as they comprise cell membranes, cell cytoskeletal structures, and extracellular matrices.Citation1–Citation3 In 1959, Feynman,Citation4 who described a process to form well-organized supramolecules by combining individual units through a bottom-up approach, first proposed the concept of self-assembled molecules. In the 1960s, LehnCitation5,Citation6 was the first one to introduce the term and concepts of supramolecular chemistry, and he had been awarded a Nobel Prize for his contribution to this area in 1987. Since then, efforts spanning a multitude of disciplines, involving mostly chemistry, physics, materials, mathematics, engineering, and biology, have contributed to this rapidly growing field to understand building block monomer structures, associated molecular assembly mechanisms, programmed architectures, controllable properties, and advanced functions.Citation1,Citation7
Just like higher hierarchical biomolecules in nature (such as proteins, DNA, and polysaccharides), most self-assembled molecules are formed by the interaction of individual monomers through weak, noncovalent interactions, including electrostatic interactions, hydrophobic interactions, hydrogen bonds, van der Waals interactions, and π–π stacking forces.Citation3,Citation8 Even though individual noncovalent forces are very weak (eg, hydrophobic forces <10 kcal/mol, electrostatic forces =1–20 kcal/mol, hydrogen bonds =2–30 kcal/mol, and π–π aromatic stacking =0–10 kcal/mol), the combination of several noncovalent forces together can generate very stable and well-organized structures.Citation8 There are several advantages of developing self-assembled materials through noncovalent bonds compared to covalent forces. For example, due to strong covalent bonds prevalent in materials such as polymers, covalent bonds (eg, C–C bond =86 kcal/mol, C–H bond =103 kcal/mol, C–O bond =81 kcal/mol) are not reversible without adding catalysts or conducting extensive material processing. Compared with covalent bonding, these noncovalent forces present in numerous self-assembled materials are reversible and dynamic, providing for advanced functions, such as high synthetic convergence, error correction, programmed design, and self-organization.Citation9
To maximize the formation of as many noncovalent forces as possible, the major component of self-assembled molecules has an amphiphilic structure with both hydrophobic and hydrophilic portions.Citation10 When responding to an aqueous environment, such molecules display hydrophobic or hydrophilic domains on the surface to match that of the solvent properties. Among all the self-assembling molecules designed today, peptides are particularly attractive building blocks in this respect since peptide sequences can be formulated to have both hydrophilic and hydrophobic domains in the same building block. Owing to such unique properties in self-assembled peptides, they have been used for numerous applications, including storing bioinformation via their various sequences, peptide folding, fast and easy synthesis, a variety of functionalization methods, and their programmed responses to external stimulations (such as pH value, ion concentration, and hydrophobicity).Citation8,Citation11
In terms of the higher hierarchical structure of self-assembled peptides, their stability depends on the monomer structure in terms of the length of the amino acid chain and the shape of the functional group.Citation12 At the same time, the external environment, including temperature, pH, ionic strength, and mechanical force, can affect or reverse the self-assembly process.Citation13 Therefore, based on these properties, scientists can design self-assembled molecules with an “intelligence” to be triggered by the changing environment, such as specific targeting, controlled release, and improved efficacy.Citation9
To synthesize peptides and their hybrid structures, there are three main methods: solid-phase chemical synthesis, protein engineering, and ring-opening polymerization strategy.Citation14 Generally, solid-phase peptide synthesis is used for short-to-medium-length peptide sequences of highly precise structures. Although the yield of solid-phase peptide synthesis is >98% per step, the synthetic limitation of this method is in the range of 70 amino acids. To synthesize a long peptide sequence (>50 amino acids) with a defined structure such as silk and collagen molecules, researchers can utilize protein engineering to control genetic expression in bacteria. For the large-scale production of polypeptides, it is recommended to use ring-opening polymerization, in which cyclic monomers are added to the chain end to form a long peptide. However, this method produces a peptide primary structure with a lower accuracy than that obtained during solid-phase synthesis.Citation14
In this review, we would like to introduce self-assembled nanostructures (nanospheres, nanotubes, nanofibers, and other ordered nanostructures) with linear peptide monomers (amphiphilic peptides, ionic-complementary peptides, etc) and nonlinear peptide monomers (cyclic peptides and hybrid peptides) as building blocks and discuss some relevant recent applications of such molecules involved during drug delivery, tissue engineering, antimicrobial control, and electronic devices ().
Figure 1 Schematic illustration of various nanostructures (nanosphere, nanotube, nanofiber, and ordered nanostructure) formed by self-assembling peptides (amphiphilic peptides, ionic-complementary peptides, cyclic peptides, and hybrid peptides) and their applications in tissue engineering, drug delivery, bioimaging, and biosensors.
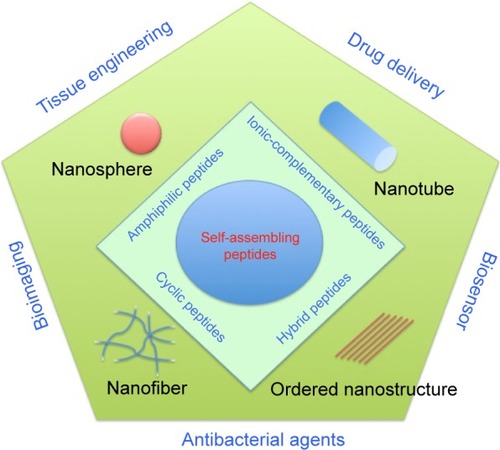
Self-assembled peptide types and structures
Amphiphilic peptides
Amphiphilic peptides involve pure linear peptides, ionic-complementary peptides, long-chain alkylated peptides, peptide phospholipids, and peptide-based block copolymers.Citation10
Inspired by lipid molecules in cell membranes, pure peptides with hydrophobic tails and hydrophilic heads can self-assemble into nanotubes, nanovesicles, and micelles, depending both on their chemical properties (eg, peptide sequences, charges, concentration, etc) and physical properties (eg, size, shape, etc).Citation15–Citation17 For the hydrophobic tail (which normally contains six residues), amino acids such as G, A, V, L, I, and F are good candidates; meanwhile, D, K, E, R, and H are used in the hydrophilic domain.Citation10,Citation18,Citation19 For example, similar to surfactants, lipid-like peptides, such as A6D, V6D, G4DD, G6DD, G8DD, A6K, and KA6 sequences, spontaneously form self-organized nanostructures (such as micelles, vesicles, and fibers) once reaching the critical aggregation concentration (CAC) (eg, a CAC of A6D ≈1.6 mM and a CAC of A6K ≈1.5 mM).Citation9,Citation20,Citation21 Moreover, due to their similarity to phospholipids, these peptides could stabilize membrane proteins, which denature and aggregate easily.
For example, Yeh et alCitation22 and Zhao et alCitation23 utilized lipid-like peptides (eg, A6D and I6K2) to stabilize the functional forms of membrane proteins Escherichia coli glycerol-3-phosphate dehydrogenase and G protein-coupled receptor bovine rhodopsin, respectively. Compared to the common surfactants (eg, n-dodecyl-β-d-maltoside and octyl-d-glucoside) used for protein stabilization, these peptides have a higher efficiency to stabilize membrane proteins.Citation23
Ionic-complementary self-assembling peptides
Inspired from the Z-DNA binding protein zuotin, in 1993, Zhang et alCitation24 discovered the first self-assembling peptide EAK16 (n-AEAEAKAKAEAEAKAK-c) that formed into nanofibers. Since then, the rapid development of this type of self-assembling peptide has fostered numerous applications, including three-dimensional (3D) cell culture, tissue engineering, regenerative medicine, and sensory devices.Citation25–Citation27 This peptide has one side group with charged side chains and another side group with hydrophobic chains. In water, the charged side is exposed on the outside and the hydrophobic side forms a double sheet inside the nanofiber.Citation24,Citation28 Meanwhile, the peptide sequences with periodically repeated positive and negative charges form the stable structure by ionic-complementary forces in a checkerboard-like pattern and then assemble typical beta-sheet structures and eventually form a hydrogel network of nanofibers.Citation13,Citation29
Owing to the combination of ionic force and hydrogen bonds inside the beta-sheet structures, these nanofibers are stable under a wide range of temperatures, pH values, and high concentrations of denaturing chemicals.Citation26 Since the hydrophobic interactions are not specific, these nanofibers may diffuse to minimize equilibrium energy.Citation13,Citation30
There are different types of ionic-complementary self-assembled peptides (): 1) −+−+−+−+ (eg, peptide RADA16-I: Ac-RADARADARADARADA); 2) −−++−−++ (eg, peptide RADA16-II: Ac-RARADADARARADADA); 3) −−−+++; and 4) −−−−++++.Citation27,Citation31,Citation32 Among these peptides, the RADA16-I peptide can promote cell growth and tissue regeneration; therefore, it has been commercialized as a product termed PuraMatrix.Citation26,Citation32
Figure 2 Different types of ionic-complementary peptides.
Notes: (A) The hydrophobic side of the peptide molecule is green, negative charges are red, and positive charges are blue. (a) The peptide structure has one hydrophobic side and one side with negative charges. (b) The peptide structure has one hydrophobic side and one side with positive charges. (c) The peptide structure has one hydrophobic side and one side with alternate positive and negative charges. (B) Four representative ionic peptides: RAD16-I, RAD16-II, EAK16-I, and EAK16-II. Reprinted from Nano Today, Volume 4, Edition 2, Yang Y, Khoe U, Wang X, Horii A, Yokoi H, Zhang S. Designer self-assembling peptide nanomaterials, pages 193–210, Copyright 2009 with permission from Elsevier.Citation27
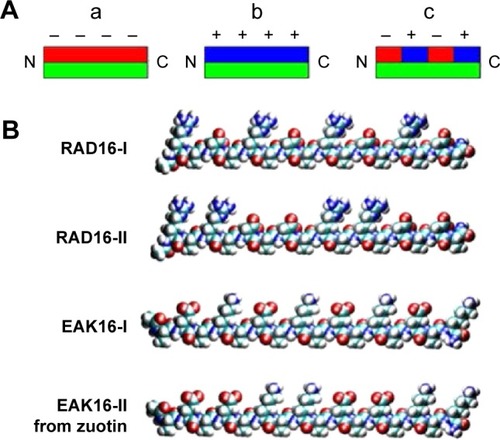
Once mixed with physiological fluids, amphiphilic peptides form a transparent hydrogel network within seconds. The hydrogel of these ionic-complementary self-assembled peptides is composed of 0.5%–1% peptides with 99.0%–99.5% water, and therefore, there are a large number of spaces between the nanofibers, which are enough for cells to grow and differentiate.Citation31,Citation33 When used in 3D cell culture systems, this type of the self-assembled peptide scaffold provides mechanical strength to encapsulate cells in a desired location; has excellent nutrient, growth factor, and oxygen diffusion for cell growth; and can release therapeutic agents in a controlled or programmed fashion.Citation34,Citation35 The ionic-complementary self-assembled peptides have been used to enhance various types of cell growth and differentiation, including bone, cartilage, vessel, heart, and neural systems.Citation34,Citation36 For example, the self-assembled peptide scaffolds made of RADA16-I and RADA16-II peptides have been used to enhance neural cell attachment, differentiation, and neurite outgrowth during in vitro studies, as well as to promote active synapse formation during in vivo studies.Citation33 For example, Gelain et al studied the 3D cultures of mouse neural stem cells using the self-assembling peptide RADA16 with the functionalized sequences derived from neural cell adhesion motifs, bone marrow homing proteins, and collagen molecules. After 7 days of culturing, the bone marrow homing peptides (SKPPGTSS and PFSSTKT)-conjugated RADA16 peptide scaffolds promoted the most neural stem cell growth and differentiation.Citation33
Long-chain alkylated peptides
Long-chain alkylated peptides are composed of hydrophilic peptide sequences and hydrophobic alkyl chains. The alkyl chain can be conjugated on both the N- and C-termini of the peptide sequences. A representative amphiphilic peptide molecule has four domains: hydrophobic tail, beta-sheet forming segment, charged groups, and bioactive epitopes ().Citation37 In the aqueous solution, individual alkylated peptides could aggregate together to form cylinder nanofibers ().Citation11 Since the most of bioactive epitopes are hydrophilic, these functionalized peptides can be exposed on the surface of nanofibers to interact with cellular receptors, which consequently influence cell signaling pathways, gene expression, and cell functions.Citation11,Citation37,Citation38
Figure 3 (A) Molecular structure of an alkylated peptide with four regions: hydrophobic tail, beta-sheet forming segment, charged groups, and bioactive epitope. Adapted from Cui H, Webber MJ, Stupp SI. Self-assembly of peptide amphiphiles: from molecules to nanostructures to biomaterials. Biopolymers. 2010;94(1):1–18. Copyright © 2010 Wiley Periodicals, Inc.Citation37 (B) Schematic illustration of an alkylated peptide (IKVAV) molecule and the nanofiber formed by IKVAV peptides. (C) TEM image of IKVAV nanofibers. From Silva GA, Czeisler C, Niece KL, et al. Selective differentiation of neural progenitor cells by high-epitope density nanofibers. Science. 2004;303(5662):1352–1355. Reprinted with permission from AAAS.Citation11
Abbreviation: TEM, transmission electron microscopy.
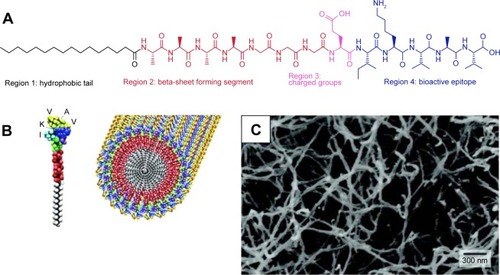
Yu et alCitation39 and Forns et alCitation40 demonstrated that the hydrocarbon chains can be used to stabilize peptide sequences and studied the folded structures. Self-assembled alkylated peptides have been utilized to functionalize carbon tubes via noncovalent bonds to enhance their solubility in water.Citation41
To mimic cell-membrane proteins, peptides have been modified with phospholipids to form peptide–phospholipid conjugates. For example, Musiol et alCitation42 have synthesized lipopeptides with the sequence derived from human prion proteins. HeLa cell experiments using confocal fluorescence microscopy proved that the self-assembling lipid peptide micelles were transported into cells very quickly.
Cyclic peptide
As early as 1974, De Santis et alCitation43 determined theoretically that cyclic peptides with alternating d- and l-amino acids could self-assemble into nanotubes. However, it was not until 1993 that Ghadiri et alCitation44 synthesized the first type of self-assembling cyclic peptide, cyclo-(l-Gln-d-Ala-l-Glu-d-Ala)2, based on De Santis’ theory.
The self-assembling cyclic peptides are composed of stacking cyclic peptide monomers with a flat conformation structure, in which the carbonyl and amino groups are pointed perpendicular to the ring and the side chains present a pseudo-equatorial outward-pointing orientation.Citation45,Citation46 Then the nanotubes were stabilized by hydrogen bonds between amide groups in neighboring peptide cycles (). Typical cyclic peptide sequences include alternating d,l-α-amino acids, β-amino acids; alternating α,β-amino acids; and alternating α,γ-amino acids, δ-amino acids, and oligoureas.Citation46–Citation49
Figure 4 Schematic illustration of the cyclic peptide molecule and the nanotubular structure provided by cyclic peptides.
Notes: Reprinted with permission from Macmillan Publishers Ltd: Nature. Fernandez-Lopez S, Kim HS, Choi EC, et al. Antibacterial agents based on the cyclic D,L-alpha-peptide architecture. 2001;412(6845):452–455.Citation45
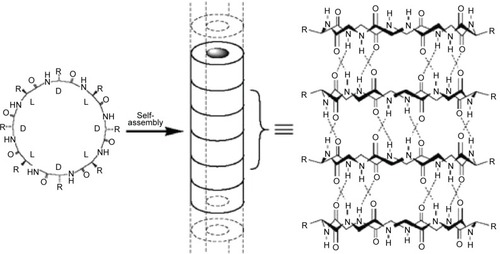
Compared to the other self-assembled peptide structures, cyclic peptide nanotubes have unique properties: 1) their precise diameter depends on the number of amino acids, chemical structure, and the side-chain size and 2) the functions of the nanotubes can be tailored by varying the side chains.Citation46 For example, when the number of amino acids in the cyclic peptide ring increases from 4 to 12, the internal diameter increases from 2 Å to 13 Å.Citation46
Applications in medicine
Drug delivery
The conventional administration of hydrophobic drugs suffers several disadvantages, including low water solubility, poor oral availability, quick biodegradation, nonspecific delivery, and serious side effects.Citation50,Citation51 Therefore, drug delivery systems could encapsulate these therapeutic agents to increase drug efficacy.Citation52 Similarly, delivery vehicles can also improve the efficacy of certain hydrophilic drugs, which have unspecific targeting or short half-lives.Citation53 In order to protect highly sensitive biomolecules (such as peptides, proteins, DNAs, and RNAs) from biodegradation and concentrate and retain them in the target organ, it is necessary to use a delivery vehicle, for which self-assembled peptides are a good option.Citation54,Citation55
For drug delivery applications, the basic requirements for self-assembled nanomaterials are biocompatibility, non-cytotoxicity, and biodegradablility.Citation56,Citation57 For drug loading and release, the nanocarriers should encapsulate molecules at a high concentration, protect them from dilution and degradation, and release them in a controlled and prolonged manner ().Citation58–Citation61 For advanced functions, the self-assembled systems should have domains specific for targeting or sensory capability to be utilized for the intended application.Citation56,Citation62
Figure 5 Schematic illustration of cell-membrane interactions and drug release of the drug-loaded self-assembling peptides.
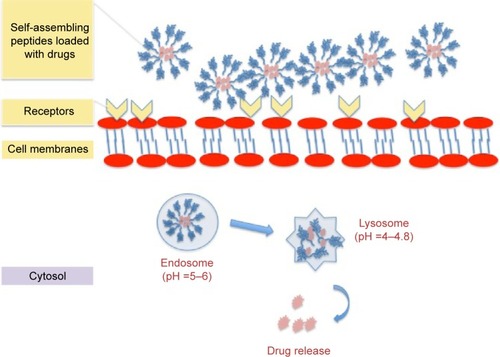
As an example of the aforementioned application, Webber et alCitation63 conjugated the anti-inflammatory drug dexamethasone with the peptide amphiphile (C16-V2A2E2) via a hydrazine linkage to achieve long-term drug release in both in vitro and in vivo tests. In vitro studies showed that dexamethasone could be released over several weeks at physiological pH and temperature. Furthermore, the peptide nanofibers were mixed with polystyrene microparticles and injected into mice, and finally, a luminescence assay and histological staining were used to evaluate the inflammation after 3 days or 9 days. The results demonstrated that the localized drug-loaded nanofiber gel could promote immune suppression.Citation63
Zhang et alCitation64 utilized the self-assembling Tat peptide to encapsulate the hydrophobic drug paclitaxel to facilitate drug delivery and efficacy. First, four sequences with different numbers of hydrophobic tails were tested, and only the four-tailed sequence qC8-Tat self-assembled into nanotubes under aqueous solutions at a pH of 7.4 (). After the coumarin-6-loaded nanofibers were delivered into KB-3-1 cervical cancer cells, confocal microscopy indicated that hydrophobic drugs were transported into cancer cells at high efficacy through the adsorptive-mediated pathway.Citation64
Figure 6 (A) Schematic illustration of paclitaxel-loaded Tat peptide nanofibers. (B) TEM image of paclitaxel-loaded Tat nanofibers in PBS. (C) Confocal image of the endocytosis pathway of drug release. (D) CD spectrum of the Tat peptide nanofibers. (E) KB-3-1 cervical cancer cell inhibition by the drug-loaded peptides. Reprinted with permission from Zhang P, Cheetham AG, Lin YA, Cui H. Self-assembled tat nanofibers as effective drug carrier and transporter. Acs Nano. 2013;7(7):5965–5977. Copyright 2013 American Chemical Society.Citation64
Abbreviations: TEM, transmission electron microscopy; PBS, phosphate-buffered saline; CD, circular dichroism.
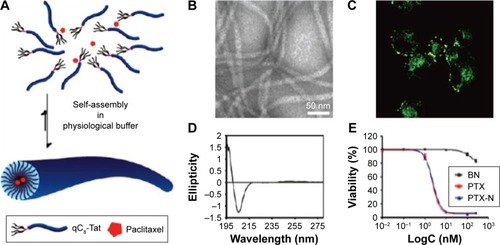
For controlled drug releases, peptide hydrogels could encapsulate small molecules or proteins such as enzymes and antibodies.Citation65,Citation66 The release profiles are determined not only by the molecule size, charge, and hydrophobicity but also by the characteristics of the peptide hydrogel, including peptide sequences, concentration, and the hydrogel pore size.Citation59
Tissue engineering
For tissue regeneration, self-assembled peptides with nanotubular or nanofibered structures could mimic the natural extracellular matrix of many tissues and localize drug release at the desired site.Citation11,Citation58,Citation67 Similar to the spherical shape of nanoparticles, nanotubes or fibers could not only display a high density of signals on the surface but also can carry multifunctional molecules to target specific cellular receptors, such as G protein-coupled receptors and kinase-linked receptors.Citation58,Citation68,Citation69 Different from micelles, nanofibers have a precise geometry that retains signals over long distances on the cell surface to benefit many applications in medicine that require directional growth, such as spinal cord regeneration, vessel growth, and cartilage and bone repair.Citation11,Citation29,Citation35,Citation70,Citation71 Moreover, several types of peptides could form a rigid network or filaments with good mechanical properties to serve as substrates or 3D scaffolds to support the growth, proliferation, differentiation, and function of cells.Citation34,Citation72–Citation74 To introduce some specific bioactive functions, it is generally a good idea to incorporate the functional peptide motifs on the C-termini of the peptide sequence with a GG spacer between the motifs and ionic-complementary sequence.Citation27 The functional motifs can then provide cells with more external stimuli than the 2D coating in terms of bioactive molecule concentration.Citation27,Citation75
Conventionally, a variety of polymers, such as poly(l-lactic acid) and poly(glycolic acid), and biopolymers, such as collagen and alginate, have been used as artificial scaffolds for various tissue engineering applications. Although these polymers are biodegradable and easy to fabricate, most of them lack bioactivity to direct cell growth and functions.Citation76 On the other hand, with their ability to self-assemble in the physiological environment, the peptide solution could be injected into the injured location and forms a scaffold to promote tissue regeneration.Citation34,Citation72,Citation77 With peptides derived from growth factor, nanofibers can expose these peptides on their surfaces to bind and activate cellular receptor for cell signaling and/or protein synthesis.Citation78,Citation79 Furthermore, the scaffolds composed of peptides can encapsulate stem cells and induce their differentiation into normal cells through bioactive peptides at a high density on the scaffold surface.Citation76,Citation78,Citation80
In a previous study, to improve cartilage regeneration, the hydrogels formed by the peptide KLD12 (KLDLKLDLKLDL) were cocultured with primary bovine chondrocytes, and the results showed that peptide hydrogel retained the chondrocyte phenotype to produce a cartilage-like extracellular matrix and type II collagen.Citation35 After 4 weeks of culturing, the material stiffness of the extracellular matrix increased, and therefore, it was suggested that the KLD12 self-assembling peptide scaffold was promising for cartilage tissue repair.Citation35
Moreover, Stroumpoulis et alCitation81 studied cell responses on vesicles formed by (C16)2-Glu-C2-GRGDSP and (C16)2-Glu-PEO-GRGDSP sequences. The sequence with PEO promoted the highest fibroblast cell adhesion and growth, which could be used to develop a membrane system to screen biological probes for cell adhesion and growth. Later on, Lin et al synthesized pH-sensitive ampiphilic peptides C16GSH and C16EOSH composed of histidine and serine with a single fatty acid tail and branched structure.Citation77 Using histidine as the switch, these peptides were viscoelastic liquids at a pH value <5.5. In a neutral solution, these peptides formed micelles. While in pH >6.5, the amphiphilic peptides assembled into nanofibers to form a hydrogel. Once cultured with cells, the hydrogel kept fibroblasts proliferating for 96 hours. This type of nanofibers is promising to develop a pH-sensitive cell scaffold to transfer the peptide solution into a hydrogel under physiological environment.Citation77
In addition, mimicking the ligand–receptor interactions of extracellular matrix components, Lee et alCitation82 synthesized amphiphilic peptides with sequences specifically binding to heparin sulfate. Then the hybrid scaffold localized with the bone morphogenetic protein-2 to modulate the ligand–receptor signaling to promote bone regeneration.
In our group, anticancer drugs (eg, curcumin) were encapsulated into the self-assembled peptide C18GR7RGDS to inhibit selectively bone cancer cells (MG-63 osteosarcoma).Citation83 Fourier transform infrared and X-ray diffraction analyses proved that curcumin was encapsulated in the hydrophobic core of peptide nanoparticles. The cell studies showed that self-assembled peptides significantly improved the delivery efficiency. Moreover, compared to healthy bone cells, curcumin-loaded peptide nanoparticles demonstrated selective cytoxicity against bone cancer cells.Citation83
Zhang et alCitation84 designed temperature-sensitive nanofibers (V3A3E3) to form aligned cellular wires. demonstrates that the amphiphilic peptides could form a string-like hydrogel in a phosphate-buffered saline solution or can be mixed with a CaCl2 solution after a temperature treatment (heated to 80°C for 30 minutes and then cooled down to 20°C). Accordingly, mechanical tests and scanning electron microscopy proved that the nanofibers organized along the length of the string. When incubated in the hydrogel, human mesenchymal stem cells grew both cell bodies and filopodia along the direction of the string formed by the peptides.Citation84
Figure 7 (A) After heating, amphiphilic peptides formed the hydrogel in a PBS solution. (B) After mixing with a CaCl2 solution, the peptide solution formed a string-like hydrogel. (C) Mesenchymal stem cells grew and differentiated at along the direction aligned with the hydrogel string formed from the peptides. (D) Fluorescence image of calcein-labeled human mesenchymal stem cells in the peptide hydrogel. Reprinted by permission from Macmillan Publishers Ltd: Nature Materials. Zhang S, Greenfield MA, Mata A, et al. A self-assembly pathway to aligned monodomain gels. 2010;9(7):594–601., Copyright 2010.Citation84
Abbreviation: PBS, phosphate-buffered saline.
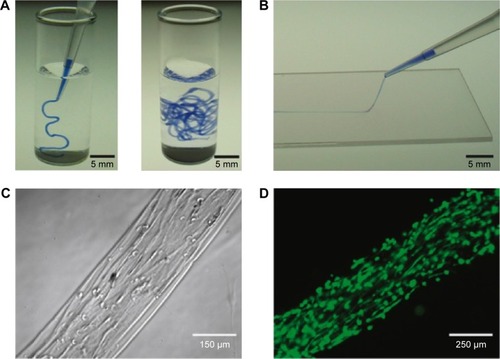
Antibacterial agents
Over the past 3 decades, the antibacterial effects of various bioactive peptides have attracted extensive attention.Citation85,Citation86 As a part of our innate defense systems, antimicrobial peptides were widely found in different types of organisms. With lengths of 10–40 amino acids, most antimicrobial peptides are capable of interacting with negatively charged bacterial membranes to show a broad spectrum of ability. The mechanism of antimicrobial peptides is not only determined by the size, hydrophobicity, amphipathicity, net charge, and secondary structure of the peptide sequence but also affected by their interaction with bacterial membranes.Citation87–Citation90
For example, Veiga et al synthesized an antibacterial hydrogel using arginine-rich self-assembled peptides with different arginine contents (two, four, six, or eight Arg residues in the sequence). They investigated the viability of E. coli and Staphylococcus aureus (105 CFU/dm2) on the peptide hydrogel surface with varying weight percentages (0.5, 1, 1.5, and 2) after 24 hours of culturing. Both increases in the number of arginine groups and the weight percent could enhance the antibacterial capability of the self-assembled hydrogels. For gels containing four, six, or eight arginine groups, both E. coli and S. aureus were completely inhibited after 24 hours, irrespective of the weight percentage of the peptides. However, the hydrogels with two arginine groups and containing 2 wt% peptides inhibited only 50% of E. coli growth. The antibacterial results indicated that the hydrogel formed by the self-assembled PEP6R peptides (VKVRVRVRVDPPTRVRVRVKV) had the greatest antibacterial ability with a low cytotoxicity toward human erythrocytes and mesenchymal stem cells.Citation86
Chu-Kung et alCitation91,Citation92 studied the effect of the length of fatty acids of peptides on antimicrobial properties. In their study, the peptides YGAAKKAAKAAKKAAKAA (AKK) and LKKLLKLLKLLKL (LKK) were conjugated with fatty acids, and the results indicated that the increased length of the fatty tails conjugated to AKK peptides enhanced interactions between the peptides and membranes, which consequently increased the antibacterial activity (for E. coli and S. epidermidis).Citation91 However, once conjugated with a longer fatty acid tail and peptides start to assemble into nanofibers, the antibacterial activity was reduced.
As another example on the use of self-assembled peptides for antibacterial applications, bone fracture healing, and antimicrobial applications, KLD12 peptides (KLDLKLDLKLDL) with variable numbers of arginine residues at the N-terminus have been synthesized to introduce the antimicrobial properties while maintaining the β-sheet and self-assembled structures.Citation93 The results of propidium iodide staining and scanning electron microscopy demonstrated that the increased arginine caused leaking of the E. coli membrane and death.
Chen et alCitation94 also assembled antimicrobial peptides (surfactin) on photoluminescent gold nanodots (SFT/DT-Au) to inhibit both normal and multidrug-resistant bacteria. In vitro studies indicated that the synergistic effect of peptides and gold dots enhanced anti-infection properties dramatically by the disruption of the membrane. Animal studies also demonstrated that SFT/DT-Au not only inhibited methicillin-resistant S. aureus infection but also promoted collagen production in wounded skin of rats infected by methicillin-resistant S. aureus.Citation94
In previous studies, cyclic peptide nanotubes were mainly used for antibacterial applications.Citation45,Citation95,Citation96 With stacking peptide cycles, nanotubes are able to form transmembrane pores to mimic ion channels in membranes.Citation45,Citation97,Citation98 Mechanistic studies showed that the antibacterial activity is due to the enhancement of the membrane permeability.Citation96,Citation97 Moreover, these cyclic peptides could also inhibit virus infections by blocking virus entry or endosome escape in mammalian cells. To inhibit methicillin-resistant bacteria, it is significant to choose a specific peptide sequence against bacterial selectively rather than mammalian cells.Citation99
Khalfa and TarekCitation98 simulated the interaction of self-assembling cyclic peptides with cytoplasmic membrane models using coarse-grained molecular dynamic simulations to study the mechanism of the antibacterial process. First, the cyclic peptide ([RRKWLWLW]) self-assembled on the top of the membrane formed by palmitoyl-oleoyl-phosphatidylethanolamine (POPE) and palmitoyl-oleoyl-phosphatidylglycerol (POPG) lipid bilayers. With the increased concentration of peptides on the surface, the lipids released from the POPE–POPG bilayers. Moreover, the mechanical properties of the membrane were affected by cyclic peptides. indicates that in the beginning of the interaction, cyclic peptides with a low concentration started to form short nanotubes and did not penetrate the membrane. At the 1/10 cyclic peptide/lipid ratio, lipid protrusion appeared. Once the cyclic peptide/lipid ratio increased to 1/5, the peptides aggregated with negative lipid molecules and caused large perturbations of the membrane.Citation98
Figure 8 CG-MD images of the interaction of self-assembling cyclic peptides ([RRKWLWLW]) with POPE–POPG lipid membranes.
Notes: (A) With a ratio of 1/22 CP/Lip, the upper image is the initial interaction at 0 ns and the lower image is the final simulation omitting the peptides at 165 ns. (B) With the ratio of 1/10 CP/Lip at 658 ns, the top image is the top view, the middle image is the side view of the initial interaction, and the lower image is the final simulation omitting the peptides. (C) With the ratio of 1/7 CP/Lip at 1.02 μs, the top image is the top view, the middle image is the side view of the initial interaction, and the lower image is the final simulation omitting the peptides. (D) With the ratio of 1/5 CP/Lip at 6.12 μs, the top image is the top view, the middle image is the side view of the initial interaction, and the lower image is the final simulation omitting the peptides. Reprinted with permission from Khalfa A, Tarek M. On the antibacterial action of cyclic peptides: insights from coarse-grained MD simulations. J Phys Chem. 2010;114(8):2676–2684. Copyright 2010 American Chemical Society.Citation98
Abbreviations: CG-MD, coarse-grained molecular dynamic; POPE, palmitoyl-oleoyl-phosphatidylethanolamine; POPG, palmitoyl-oleoyl-phosphatidylglycerol; CP/Lip, cyclic peptide/lipid.
![Figure 8 CG-MD images of the interaction of self-assembling cyclic peptides ([RRKWLWLW]) with POPE–POPG lipid membranes.Notes: (A) With a ratio of 1/22 CP/Lip, the upper image is the initial interaction at 0 ns and the lower image is the final simulation omitting the peptides at 165 ns. (B) With the ratio of 1/10 CP/Lip at 658 ns, the top image is the top view, the middle image is the side view of the initial interaction, and the lower image is the final simulation omitting the peptides. (C) With the ratio of 1/7 CP/Lip at 1.02 μs, the top image is the top view, the middle image is the side view of the initial interaction, and the lower image is the final simulation omitting the peptides. (D) With the ratio of 1/5 CP/Lip at 6.12 μs, the top image is the top view, the middle image is the side view of the initial interaction, and the lower image is the final simulation omitting the peptides. Reprinted with permission from Khalfa A, Tarek M. On the antibacterial action of cyclic peptides: insights from coarse-grained MD simulations. J Phys Chem. 2010;114(8):2676–2684. Copyright 2010 American Chemical Society.Citation98Abbreviations: CG-MD, coarse-grained molecular dynamic; POPE, palmitoyl-oleoyl-phosphatidylethanolamine; POPG, palmitoyl-oleoyl-phosphatidylglycerol; CP/Lip, cyclic peptide/lipid.](/cms/asset/29751a06-10a1-47ae-ba6c-b86996ad2261/dijn_a_117501_f0008_c.jpg)
Nanosensors
Once targeting biomolecules or metallic nanoparticles into self-assembled peptides were incorporated, peptide nanotubes could act as biosensors with enhanced sensitivity and selectivity for mineralization, imaging probes, and electronic devices.Citation100–Citation103
Inspired from peptide sequences with a biomineralized capability in nature, peptide nanotubes can be conjugated with these sequences (such as peptide amphiphiles containing the RGD sequence) to nucleate metal/semiconductor materials on the surface. Several groups have utilized peptide nanomaterials to control mineralization for bone tissue regeneration.Citation104,Citation105 This method could facilitate bone cell growth along the peptide fibers with a desired direction in the scale of nanometers.
For diagnostic imaging, peptide nanomaterials have been also conjugated with radiometals for in vivo tracking using the near-infrared fluorescenceCitation106 or MRI.Citation107,Citation108 Bull et al synthesized two Gd(III) conjugated amphiphilic peptides (DOTA-KK(K)K-LL-CCC-K-C16 and DOTA-KGRGDS(K)K-LLL-AAA-K-C16) to form spherical and tubular structures. Since the self-assembling structure could increase the molecular weight of Gd, the rotational correlation time and consequently the relaxivity were enhanced. MRI tests demonstrated that these Gd functionalized peptides could increase T1 relaxation time for medical applications.Citation108
With advantages of one-dimensional direction and a controllable size, peptide nanotubes are suitable to work as building blocks for nanoelectronic devices.Citation109 After nucleation, the electronic properties of the peptide nanotubes can be tuned by the size, shape, arrangement, and density of a mineralized coating. Additionally, the conditions (eg, pH, ion concentration, etc) of the extra environment also affected the mineralization process and the final conductivity.Citation109,Citation110 Previous studies utilized such nanotubes to modify membranes to detect the conductivity change from the antigen–antibody interactions.Citation111
For example, the self-assembled histidine-rich HGGGHGHGGGHG (HG12) peptides with linear or branched alkyl chains were labeled by pyrene to detect metal ions ().Citation112 Since the HG12 sequence bonded Cu2+ specifically, the fluorescence could be blocked. On the other hand, the Ag+ could “light up” the fluorescence response of the nanofibers with branched alkyl chains (). Moreover, the nanofibers could work as templates for Ag nanoparticles for cell imaging and antibacterial effects.Citation112 summarizes the highlighted medical applications of self-assembled peptides in this review.
Table 1 Highlighted medical applications of self-assembled peptide nanostructures
Figure 9 (A) The molecular structure of the pyrene-labeled peptide amphiphiles 1 and 2. (B) Schematic representation of the fluorescence light off and light up detections to copper and silver ions. Reproduced from Kim I, Jeong HH, Kim YJ, et al. A “light-up” 1D supramolecular nanoprobe for silver ions based on assembly of pyrene-labeled peptide amphiphiles: cell-imaging and antimicrobial activity. J Mat Chem. 2014;2(38):6478–6486, with permission of The Royal Society of Chemistry.Citation112
Abbreviation: PET, photoinduced electron transfer.
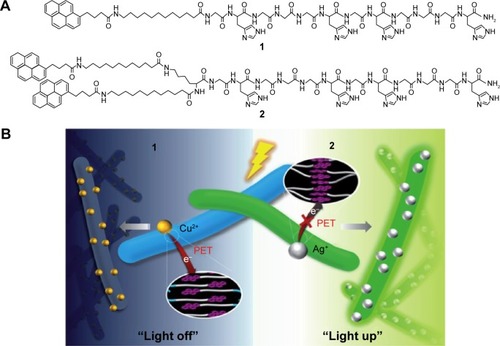
Future directions and conclusion
Based on noncovalent forces, self-assembled molecules could construct ordered structures of defined shapes, various properties, and multiple functions. Examples from recent studies have shown that self-assembled peptides with different structures have been used for a wide range of medical applications. However, in this field, scientists and researchers are still facing challenges to predict higher hierarchical structures, properties, and functions from the structure of the individual building molecule. With multidisciplinary efforts, self-assembled peptides will help us not only to study complex biological phenomena and create various applications but also to conquer the diseases and improve human health.
Acknowledgments
The authors would like to thank Wenzhou Institute of Biomaterials and Engineering and Northeastern University for funding.
Disclosure
The authors report no conflicts of interest in this work.
References
- WhitesidesGMBonchevaMBeyond molecules: self-assembly of mesoscopic and macroscopic componentsProc Natl Acad Sci U S A20029984769477411959929
- WhitesidesGMGrzybowskiBSelf-assembly at all scalesScience200229555642418242111923529
- TuRSTirrellMBottom-up design of biomimetic assembliesAdv Drug Deliv Rev200456111537156315350288
- FeynmanRPThere’s plenty of room at the bottomCaltech Eng Sci19592352236
- LehnJMSupramolecular chemistry – scope and perspectives molecules, supermolecules, and molecular devicesAngew Chem Int Ed Engl198827189112
- LehnJMPerspectives in chemistry-aspects of adaptive chemistry and materialsAngew Chem Int Ed Engl201554113276328925582911
- HagstromJESelf-assembling complexes for in vivo gene deliveryCurr Opin Mol Ther20002214314911249634
- ShiJFXuBNanoscale assemblies of small molecules control the fate of cellsNano Today201510561563026900396
- HosseinkhaniHHongPDYuDSSelf-assembled proteins and peptides for regenerative medicineChem Rev201311374837486123547530
- LowikDvan HestJCMPeptide based amphiphilesChem Soc Rev200433423424515103405
- SilvaGACzeislerCNieceKLSelective differentiation of neural progenitor cells by high-epitope density nanofibersScience200430356621352135514739465
- SchneiderJPPochanDJOzbasBRajagopalKPakstisLKretsingerJResponsive hydrogels from the intramolecular folding and self-assembly of a designed peptideJ Am Chem Soc200212450150301503712475347
- KimSKimJHLeeJSParkCBBeta-sheet-forming, self-assembled peptide nanomaterials towards optical, energy, and healthcare applicationsSmall201511303623364025929870
- KlokHAProtein-inspired materials: synthetic concepts and potential applicationsAngew Chem Int Ed200241915091513
- AntoniettiMForsterSVesicles and liposomes: a self-assembly principle beyond lipidsAdv Mater Deerfield2003151613231333
- HuangRLQiWSuRXZhaoJHeZMSolvent and surface controlled self-assembly of diphenylalanine peptide: from microtubes to nanofibersSoft Matter201171464186421
- LiuLBusuttilKZhangSThe role of self-assembling polypeptides in building nanomaterialsPhys Chem Chem Phys20111339174351744421818484
- CaplanMRSchwartzfarbEMZhangSGKammRDLauffenburgerDAControl of self-assembling oligopeptide matrix formation through systematic variation of amino acid sequenceBiomaterials200223121922711762841
- MariniDMHwangWLauffenburgerDAZhangSGKammRDLeft-handed helical ribbon intermediates in the self-assembly of a beta-sheet peptideNano Lett200224295299
- VautheySSantosoSGongHYWatsonNZhangSGMolecular self-assembly of surfactant-like peptides to form nanotubes and nanovesiclesProc Natl Acad Sci U S A20029985355536011929973
- von MaltzahnGVautheySSantosoSZhangSUPositively charged surfactant-like peptides self-assemble into nanostructuresLangmuir2003191043324337
- YehJIDuSCTortajadaAPauloJZhangSGPeptergents: peptide detergents that improve stability and functionality of a membrane protein, glycerol-3-phosphate dehydrogenaseBiochemistry20054451169121691916363804
- ZhaoXNagaiYReevesPJKileyPKhoranaHGZhangSDesigner short peptide surfactants stabilize G protein-coupled receptor bovine rhodopsinProc Natl Acad Sci U S A200610347177071771217098868
- ZhangSGHolmesTLockshinCRichASpontaneous assembly of a self-complementary oligopeptide to form a stable macroscopic membraneProc Natl Acad Sci U S A1993908333433387682699
- GelainFHoriiAZhangSDesigner self-assembling peptide scaffolds for 3-D tissue cell cultures and regenerative medicineMacromol Biosci20077554455117477441
- ZhaoXBPanFXuHMolecular self-assembly and applications of designer peptide amphiphilesChem Soc Rev20103993480349820498896
- YangYKhoeUWangXHoriiAYokoiHZhangSDesigner self-assembling peptide nanomaterialsNano Today200942193210
- ZhangSLockshinCCookRRichAUnusually stable beta-sheet formation in an ionic self-complementary oligopeptideBiopolymers19943456636728003624
- ZhangSHolmesTCDiPersioCMHynesROSuXRichASelf-complementary oligopeptide matrices support mammalian cell attachmentBiomaterials19951618138513938590765
- AltmanMLeePRichAZhangSGConformational behavior of ionic self-complementary peptidesProtein Science2000961095110510892803
- ZhangSGEmerging biological materials through molecular self-assemblyBiotechnol Adv2002205–632133914550019
- YokoiHKinoshitaTZhangSGDynamic reassembly of peptide RADA16 nanofiber scaffoldProc Natl Acad Sci U S A2005102248414841915939888
- GelainFBottaiDVescoviAZhangSDesigner self-assembling peptide nanofiber scaffolds for adult mouse neural stem cell 3-dimensional culturesPlos One200612e11917205123
- DavisMEMotionJPMNarmonevaDAInjectable self-assembling peptide nanofibers create intramyocardial microenvironments for endothelial cellsCirculation2005111444245015687132
- KisidayJJinMKurzBSelf-assembling peptide hydrogel fosters chondrocyte extracellular matrix production and cell division: Implications for cartilage tissue repairProc Natl Acad Sci U S A2002991599961000112119393
- WenYRoudebushSLBuckholtzGACoassembly of amphiphilic peptide EAK16-II with histidinylated analogues and implications for functionalization of beta-sheet fibrils in vivoBiomaterials201435195196520524680662
- CuiHWebberMJStuppSISelf-assembly of peptide amphiphiles: from molecules to nanostructures to biomaterialsBiopolymers201094111820091874
- NewcombCJSurSOrtonyJHCell death versus cell survival instructed by supramolecular cohesion of nanostructuresNat Commun20145332124531236
- YuYCTirrellMFieldsGBMinimal lipidation stabilizes protein-like molecular architectureJ Am Chem Soc19981203999799987
- FornsPLauer-FieldsJLGaoSFieldsGBInduction of protein-like molecular architecture by monoalkyl hydrocarbon chainsBiopolymers200054753154610984405
- ArnoldMSGulerMOHersamMCStuppSIEncapsulation of carbon nanotubes by self-assembling peptide amphiphilesLangmuir200521104705470916032892
- MusiolHJDongSLKaiserMToward semisynthetic lipoproteins by convergent strategies based on click and ligation chemistryChembiochem20056462562815723440
- De SantisPForniERizzoRConformational analysis of DNA-basic polypeptide complexes: possible models of nucleoprotamines and nucleohistonesBiopolymers19741323133264820064
- GhadiriMRGranjaJRMilliganRAMcReeDEKhazanovichNSelf-assembling organic nanotubes based on a cyclic peptide architectureNature199336664533243278247126
- Fernandez-LopezSKimHSChoiECAntibacterial agents based on the cyclic D,L-alpha-peptide architectureNature2001412684545245511473322
- ChapmanRDanialMKohMLJolliffeKAPerrierSDesign and properties of functional nanotubes from the self-assembly of cyclic peptide templatesChem Soc Rev201241186023604122875035
- IshiharaYKimuraSNanofiber formation of amphiphilic cyclic tri-beta-peptideJ Peptide Sci201016211011420063334
- GuerraABreaRJAmorinMCastedoLGranjaJRSelf-assembling properties of all gamma-cyclic peptides containing sugar amino acid residuesOrg Biomol Chem201210448762876623060041
- MontenegroJGhadiriMRGranjaJRIon channel models based on self-assembling cyclic peptide nanotubesAcc Chem Res201346122955296523898935
- LangerRDrug delivery and targetingNature199839266795109579855
- SuCWChiangCSLiWMHuSHChenSYMultifunctional nanocarriers for simultaneous encapsulation of hydrophobic and hydrophilic drugs in cancer treatmentNanomedicine20149101499151525253498
- RoslerAVandermeulenGWMKlokHAAdvanced drug delivery devices via self-assembly of amphiphilic block copolymersAdv Drug Deliv Rev20015319510811733119
- KopecekJSmart and genetically engineered biomaterials and drug delivery systemsEur J Pharm Sci200320111613678788
- MaurerNFenskeDBCullisPRDevelopments in liposomal drug delivery systemsExpert Opin Biol Ther20011692394711728226
- HuangRLQiWFengLBSuRXHeZMSelf-assembling peptide-polysaccharide hybrid hydrogel as a potential carrier for drug deliverySoft Matter201171362226230
- PawarRBen-AriADombAJProtein and peptide parental controlled deliveryExpert Opin Biol Ther2004481203121215268656
- CollinsLParkerALGehmanJDSelf-assembly of peptides into spherical nanoparticles for delivery of hydrophilic moieties to the cytosolACS Nano2010452856286420408581
- RajagopalKSchneiderJPSelf-assembling peptides and proteins for nanotechnological applicationsCurr Opin Struct Biol200414448048615313243
- XuXHLiYKLiHPSmart nanovehicles based on pH-triggered disassembly of supramolecular peptide-amphiphiles for efficient intracellular drug deliverySmall20141061133114024155260
- LinBFMissirlisDKrogstadDVTirrellMStructural effects and lipid membrane interactions of the pH-responsive GALA peptide with fatty acid acylationBiochemistry201251234658466822591394
- SantanaHAvilaCLCabreraIHow does growth hormone releasing hexapeptide self-assemble in nanotubes?Soft Matter201410469260926925325399
- BoekhovenJZhaRHTantakittiFAlginate-peptide amphiphile core-shell microparticles as a targeted drug delivery systemRSC Adv20155128753875625642326
- WebberMJMatsonJBTamboliVKStuppSIControlled release of dexamethasone from peptide nanofiber gels to modulate inflammatory responseBiomaterials201233286823683222748768
- ZhangPCheethamAGLinYACuiHSelf-assembled tat nanofibers as effective drug carrier and transporterAcs Nano2013775965597723758167
- PeppasNABuresPLeobandungWIchikawaHHydrogels in pharmaceutical formulationsEur J Pharmaceutics and Biopharm20005012746
- DavisMEHsiehPCHTakahashiTLocal myocardial insulin-like growth factor 1 (IGF-1) delivery with biotinylated peptide nanofibers improves cell therapy for myocardial infarctionProc Natl Acad Sci U S A2006103218155816016698918
- WanACAYingJYNanomaterials for in situ cell delivery and tissue regenerationAdv Drug Deliv Rev2010627–873174020156499
- LiYYuSMTargeting and mimicking collagens via triple helical peptide assemblyCurr Opin Chem Biol201317696897524210894
- AlsbaieeABeingessnerRLFenniriHSelf-assembled nanomaterials for tissue-engineering applicationsWebsterTJNanomedicine: Technologies and ApplicationsWoodhead Publishing LimitedCambridge, UK2012490533
- NahJWYuLHanSOAhnCHKimSWArtery wall binding peptide-poly (ethylene glycol)-grafted-poly(L-lysine)-based gene delivery to artery wall cellsJ Controll Release2002781–3273284
- SeminoCEKasaharaJHayashiYZhangSGEntrapment of migrating hippocampal neural cells in three-dimensional peptide nanofiber scaffoldTissue Eng2004103–464365515165480
- KretsingerJKHainesLAOzbasBPochanDJSchneiderJPCytocompatibility of self-assembled beta-hairpin peptide hydrogel surfacesBiomaterials200526255177518615792545
- KimSJKimJEKimSHTherapeutic effects of neuropeptide substance P coupled with self-assembled peptide nanofibers on the progression of osteoarthritis in a rat modelBiomaterials20167411913026454050
- KumadaYHammondNAZhangSGFunctionalized scaffolds of shorter self-assembling peptides containing MMP-2 cleavable motif promote fibroblast proliferation and significantly accelerate 3-D cell migration independent of scaffold stiffnessSoft Matter201062050735079
- HauserCAEZhangSDesigner self-assembling peptide nanofiber biological materialsChem Soc Rev20103982780279020520907
- ZhangZPHuJMaPXNanofiber-based delivery of bioactive agents and stem cells to bone sitesAdv Drug Deliv Rev201264121129114122579758
- LinBFMegleyKAViswanathanNpH-responsive branched peptide amphiphile hydrogel designed for applications in regenerative medicine with potential as injectable tissue scaffoldsJ Mater Chem201222371944719454
- KimJEKimSHJungYIn situ chondrogenic differentiation of bone marrow stromal cells in bioactive self-assembled peptide gelsJ Biosci Bioeng20151201919825540912
- WuECZhangSGHauserCAESelf-assembling peptides as cell-interactive scaffoldsAdv Funct Mater2012223456468
- ChengTYChenMHChangWHHuangMYWangTWNeural stem cells encapsulated in a functionalized self-assembling peptide hydrogel for brain tissue engineeringBiomaterials20133482005201623237515
- StroumpoulisDZhangHRubalcavaLGliemJTirrellMCell adhesion and growth to peptide-patterned supported lipid membranesLangmuir20072373849385617335250
- LeeSSHuangBJKaltzSRBone regeneration with low dose BMP-2 amplified by biomimetic supramolecular nanofibers within collagen scaffoldsBiomaterials201334245245923099062
- ChangRSunLWebsterTJSelective inhibition of MG-63 osteosarcoma cell proliferation induced by curcumin-loaded self-assembled arginine-rich-RGD nanospheresInt J Nanomedicine2015103351336526005346
- ZhangSGreenfieldMAMataAA self-assembly pathway to aligned monodomain gelsNat Mater20109759460120543836
- ChenCPanFZhangSAntibacterial activities of short designer peptides: a link between propensity for nanostructuring and capacity for membrane destabilizationBiomacromolecules201011240241120078032
- VeigaASSinthuvanichCGasparDFranquelimHGCastanhoMSchneiderJPArginine-rich self-assembling peptides as potent antibacterial gelsBiomaterials201233358907891622995710
- GalanthCAbbassiFLequinOMechanism of antibacterial action of dermaseptin B2: interplay between helix-hinge-helix structure and membrane curvature strainBiochemistry200948231332719113844
- ZelezetskyITossiAAlpha-helical antimicrobial peptides – using a sequence template to guide structure-activity relationship studiesBiochim Biophys Acta2006175891436144916678118
- BechingerBLohnerKDetergent-like actions of linear amphipathic cationic antimicrobial peptidesBiochimi Biophys Acta20061758915291539
- van KanEJMvan der BentADemelRAde KruijffBMembrane activity of the peptide antibiotic clavanin and the importance of its glycine residuesBiochemistry200140216398640511371202
- Chu-KungAFNguyenRBozzelliKNTirrellMChain length dependence of antimicrobial peptide-fatty acid conjugate activityJ Colloid Interface Sci2010345216016720185142
- Chu-KungAFBozzelliKNLockwoodNAHasemanJRMayoKHTirrellMVPromotion of peptide antimicrobial activity by fatty acid conjugationBioconjug Chem200415353053515149180
- TripathiJKPalSAwasthiBVariants of self-assembling peptide, KLD-12 that show both rapid fracture healing and antimicrobial propertiesBiomaterials2015569210325934283
- ChenWYChangHYLuJKSelf-assembly of antimicrobial peptides on gold nanodots: against multidrug-resistant bacteria and wound-healing applicationAdv Funct Mat2015254671897199
- BiondaNPitteloudJPCudicPCyclic lipodepsipeptides: a new class of antibacterial agents in the battle against resistant bacteriaFuture Med Chem20135111311133023859209
- DartoisVSanchez-QuesadaJCabezasESystemic antibacterial activity of novel synthetic cyclic peptidesAntimicrob Agents Chemother20054983302331016048940
- BreaRJVazquezMEMosqueraMCastedoLGranjaJRControlling multiple fluorescent signal output in cyclic peptide-based supramolecular systemsJ Am Chem Soc200712961653165717243683
- KhalfaATarekMOn the antibacterial action of cyclic peptides: insights from coarse-grained MD simulationsJ Phys Chem2010114826762684
- BiondaNStawikowskiMStawikowskaREffects of cyclic lipodepsipeptide structural modulation on stability, antibacterial activity, and human cell toxicityChemmedchem20127587188222392790
- WilliamsRJMartRJUlijnRVExploiting biocatalysis in peptide self-assemblyBiopolymers201094110711720091879
- MishraALooYHDengRHUltrasmall natural peptides self-assemble to strong temperature-resistant helical fibers in scaffolds suitable for tissue engineeringNano Today201163232239
- MishraAChanKHReithoferMRHauserCAEInfluence of metal salts on the hydrogelation properties of ultrashort aliphatic peptidesRSC Adv201332599859993
- RodriguezALParishCLNisbetDRWilliamsRJTuning the amino acid sequence of minimalist peptides to present biological signals via charge neutralised self assemblySoft Matter201391539153919
- HartgerinkJDBeniashEStuppSISelf-assembly and mineralization of peptide-amphiphile nanofibersScience200129455471684168811721046
- HuangZSargeantTDHulvatJFBioactive nanofibers instruct cells to proliferate and differentiate during enamel regenerationJ Bone Mineral Res2008231219952006
- TanisakaHKizaka-KondohSMakinoATanakaSHiraokaMKimuraSNear-infrared fluorescent labeled peptosome for application to cancer imagingBioconjug Chem200819110911718163535
- MoriscoAAccardoAGianolioETesauroDBenedettiEMorelliGMicelles derivatized with octreotide as potential target-selective contrast agents in MRIJ Peptide Sci200915324225019035577
- BullSRGulerMOBrasREMeadeTJStuppSISelf-assembled peptide amphiphile nanofibers conjugated to MRI contrast agentsNano Lett2005511415792402
- RechesMGazitECasting metal nanowires within discrete self-assembled peptide nanotubesScience2003300561962562712714741
- CavalliSAlbericioFKrosAAmphiphilic peptides and their cross-disciplinary role as building blocks for nanoscienceChem Soc Rev201039124126320023851
- KrolSMacrezRDocagneFTherapeutic benefits from nanoparticles: the potential significance of nanoscience in diseases with compromise to the blood brain barrierChem Rev201311331877190323157552
- KimIJeongHHKimYJA “light-up” 1D supramolecular nanoprobe for silver ions based on assembly of pyrene-labeled peptide amphiphiles: cell-imaging and antimicrobial activityJ Mat Chem201423864786486