Abstract
As the number of commercial and consumer products containing engineered nanomaterials (ENMs) continually rises, the increased use and production of these ENMs presents an important toxicological concern. Although ENMs offer a number of advantages over traditional materials, their extremely small size and associated characteristics may also greatly enhance their toxic potentials. ENM exposure can occur in various consumer and industrial settings through inhalation, ingestion, or dermal routes. Although the importance of accurate ENM characterization, effective dosage metrics, and selection of appropriate cell or animal-based models are universally agreed upon as important factors in ENM research, at present, there is no “standardized” approach used to assess ENM toxicity in the research community. Of particular interest is occupational exposure to tungsten carbide cobalt (WC-Co) “dusts,” composed of nano- and micro-sized particles, in hard metal manufacturing facilities and mining and drilling industries. Inhalation of WC-Co dust is known to cause “hard metal lung disease” and an increased risk of lung cancer; however, the mechanisms underlying WC-Co toxicity, the inflammatory disease state and progression to cancer are poorly understood. Herein, a discussion of ENM toxicity is followed by a review of the known literature regarding the effects of WC-Co particle exposure. The risk of WC-Co exposure in occupational settings and the updates of in vitro and in vivo studies of both micro- and nano-WC-Co particles are discussed.
Engineered nanomaterials (ENMs), nanotoxicity, and means of exposure
Because of recent technological and manufacturing advancements, the production and use of ENMs has increased at a rapid pace. The term ENM broadly encompasses a number of nano-sized materials that vary in shape, such as nanotubes, nanowires, or nanoparticles (NPs), which are generally defined as “any material having at least one dimension smaller than 100 nm.”Citation1 Because of their extremely small size and high surface area, NPs offer a number of advantages over traditional “bulk” materials and are suitable for a wide variety of applications in consumer goods,Citation2,Citation3 medical devices and diagnostics,Citation4–Citation6 pharmaceutical products,Citation4,Citation7–Citation10 fuel additives,Citation11,Citation12 and other industrial uses.Citation13–Citation17 As a result, the number of manufactured goods containing NPs is continually rising; in 2006, manufacturers reported that NPs were incorporated in over 600 consumer products worth $50 billion in market value,Citation18 a number which has doubled to >1,600 NP-containing consumer products in 2013.Citation19
With the increased use of NPs in such diverse applications, a concomitant risk of exposure exists across consumer households and in commercial occupational settings. NPs exist in our daily environments and are often referred to as “contaminants” of important air, water, and soil resources.Citation20,Citation21 Because these NPs are all around us, exposure can occur through mechanisms such as inhalation, ingestion, and dermal exposure.Citation22 Additionally, emerging evidence suggests that humans may also be exposed to NPs internally, which may be generated in situ due to orthopedic surgical implant wear.Citation23,Citation24 These potential routes of NP exposure are summarized graphically in . Importantly, the route of exposure ultimately determines which body system or specific tissues the NPs interact with which in turn determines the effects of NP exposure such as toxicityCitation25,Citation26 or alterations in physiological function.Citation27
The effects of NP exposure can be divided into primary and secondary categories (), depending upon the extent of exposure. Primary effects resulting from direct cellular NP contact may include toxicity, oxidative stress, DNA damage, and inflammation.Citation1,Citation28,Citation29 Because of their small size, NPs may translocate through tissue barriers into the blood, where they can circulate and eventually deposit in other organs, thereby generating a secondary NP exposure. Secondary effects may include toxicity at the site of NP deposition, in organs such as the liver, spleen, or kidneys, stimulation of systemic inflammation or alterations in systemic function.Citation1,Citation28–Citation30 The first three routes of exposure occur through external NP sources, but there is emerging evidence which indicates that humans may also be exposed internally, when orthopedic or surgical implant wear NPs are released locally from the implant site.Citation23,Citation31,Citation32 Most commonly, humans are exposed to NPs in their environments through the pulmonary route, by inhaling airborne NPs during normal breathing.Citation27,Citation33
Despite their potential toxic properties, it is worth mentioning that certain NPs have distinct advantages that outweigh the risk of use and have greatly improved consumer products on the market today, such as sunscreens and cosmetics.Citation22 For example, titanium dioxide NPs present in sunscreens and cosmetic products are highly beneficial, offering excellent protection from sun exposure, thus protecting the skin against UV damage and preventing sunburn. NPs have been approved for use under these conditions; however, the long-term effects of titanium dioxide NPs on aquatic life in contaminated water sources (lakes, oceans, etc) remain an important concern for future research.
As a direct result of the increased use of NPs and likelihood of exposure, the study of the acute and chronic effects of NP exposure has recently emerged as the field of “nanotoxicology”.Citation34 Currently, there is no standard approach for NP toxicity testing, and a number of arguments support the implementation of a standard testing procedure, so that results and outcomes can be directly comparable among all NPs tested. However, this remains as a difficult task because the route and realistic amount of exposure (dose) vary greatly depending on the particular NP in question.Citation35 In order to define the toxic potential of NPs, several key factors must be addressed (summarized in ).Citation1,Citation29,Citation34 First, it is imperative to characterize NPs in terms of size, shape, and properties (ie, morphology, surface charge), so that the observed effects can be attributed to a particular property or characteristic. Next, an appropriate dosage metric must be selected for the NPs to be examined. In the literature, NP dosages have been reported as concentration per volume or mass (mg/mL or mg/kg), as reactive surface area (cm2) or as particle number in solution, calculated based on NP size.Citation35
Table 1 Important components of NP toxicity testing
In addition to particle characterization and selection of the dosing metric, an appropriate model must be selected for toxicity testing. Typically, in vitro cell-based models are the starting point due to lower cost and relative ease of execution compared to in vivo animal-based model systems.Citation36 In vitro systems require small amounts of NPs for testing and allow for dose–response testing and sample collection over time in various systems, such as cells or tissues.Citation36 Primary or commercial cells are commonly used for ENM toxicity testing and cell selection for a given assay is frequently based on the potential route of NP exposure; some examples of in vitro models are summarized in . Aside from the advantage of being “fast and cheap,” in vitro models can be used to estimate toxico-kinetic parameters, identify potential target organ toxicity, and help define appropriate NP dosages for in vivo studies, which help to reduce the number of experimental animals required for nanotoxicity research.
Table 2 Examples of in vitro models used in NP toxicity testing
Although in vitro models and toxicity assays are in general reliable and used frequently, several groups have reported NP interference with commonly used cell-based assays,Citation37,Citation38 bringing into question whether the results are accurate or whether they are simply artifacts generated because of NP interference with the assay mechanism.Citation38,Citation39 To address this issue, a number of control experiments are required to identify any potential NP assay interference, and these effects must be considered while interpreting the final results.
Although they offer a number of advantages, cell-based systems are limited and generally demonstrate the effects of NPs within a very specific and small compartment compared to whole-animal in vivo models.Citation40 Animal models may offer more realistic insights as to the effects of NP exposure in humans because the body systems can be utilized as a whole, with all of its cells, tissues, and organs interacting with the NPs as would be encountered in a realistic exposure scenario.Citation40
Since there are a number of NP exposure routes for humans, a number of in vivo exposure approaches must be used such that the effects of the NP on the appropriate tissue or organ system can be identified. Commonly used in vivo NP exposure routes are summarized , including NP inhalation, intra-tracheal instillation (IT), injection, and ingestion or gavage. As mentioned earlier, NP inhalation and pulmonary exposure are most common in humans and are therefore the most frequent routes by which experimental animals are exposed in nanotoxicity studies. However, not all NPs are suitable for aerosolization and for the purposes of an inhalation chamber, so alternatively, pulmonary NP exposure can be achieved via IT.Citation41 IT is a highly reproducible, direct method for depositing NP within the lungs, and NPs delivered in this manner have very similar lung distribution to NP inhalation;Citation41 therefore, IT is considered as an excellent approach for the pulmonary delivery of NP. In addition to pulmonary routes of exposure, animals may also be exposed to NP via injection for the purposes of systemic or local NP exposure. Intra-peritoneal (IP) or intravenous (IV) NP injections can be used to achieve systemic NP exposure, as the NPs circulate through the vasculature and may deposit in organs such as the liver, spleen, or kidneys. Injection may also be used to achieve localized NP exposure within articular locations, such as the knee or hip, or may be used to target subcutaneous tumors.
Table 3 Examples of in vivo exposure models used in NP toxicity testing
Industrial hard metal (WC-Co) applications and exposure
Among the plethora of NPs gaining industrial use and popularity is tungsten carbide cobalt (WC-Co), which is a hard composite metal known for its extreme hardness, stability, and sharpness.Citation42,Citation43 The use of WC-Co NPs as a spray coating on heavy machinery, drill bits, and saw blades substantially increases strength, durability, and resistance to wear, which drastically improves the operating lifetimes in industrial applications.Citation42,Citation43 In particular, WC-Co coatings are popular in mining and drilling industries, where maintenance of sharp saw blades and drills is of critical importance.
Despite the improved durability and strength of WC-Co NP coatings compared to other conventional materials, these coatings do not last long and eventually wear out with extended use and over time. As a result, airborne WC-Co dusts containing particles of varying size can be generated during use.Citation44 These airborne WC-Co “dusts” present an occupational exposure hazard not only in hard metal manufacturing facilities but also in mining and drilling industries where these WC-Co-coated implements are used extensively in enclosed environments. Typical WC-Co dusts encountered in industrial environments have a reported size range of several microns down to highly respirable particles in the nano-size range.Citation45
Although exposure to WC-Co containing dusts has been identified as an occupational workplace hazard by the National Institutes of Occupational Safety and Health,Citation46 exposure limits for WC-Co dusts and powders remain undefined. Daily occupational exposures likely vary, as it is extremely difficult to predict an accurate exposure given the variability in dust generation and ventilation among worksites. Therefore, it is difficult to estimate what a relevant WC-Co dosage or concentration should be for experimental models. The existing exposure limits for cobalt metal and ionic cobaltCitation47–Citation49 are difficult to translate to WC-Co exposure; ionic cobalt is soluble in physiological fluids and cell culture buffers, and exposure modality would experience different physiological effects than exposure to a solid (non-soluble) WC-Co composite NP.Citation47–Citation49 Additionally, cobalt content can vary, depending on the application, and the dusts generated may not have uniform composition between multiple industrial sites.Citation50 More importantly, the combination of WC-Co is more toxic than Co, W, or WC particles alone (see section entitled Defining hard metal (WC-Co) toxicity: in vitro and in vivo studies). Therefore, understanding the toxic effects of hard metal WC-Co particles is crucial in order to develop occupational exposure guidelines.
Risk of WC-Co exposure: hard metal lung disease (HMLD) prognosis and cancer risk
Exposure to “hard metal dust” containing WC-Co is well associated with the occurrence of occupational asthma and is a known risk factor for the development of HMLD.Citation44,Citation51–Citation59 Diagnosis of HMLD is a challenge, as symptoms are general and often mistaken for other respiratory ailments: patients often report difficulty breathing and present with reduced lung capacity, progressive lung inflammation, and eventual fibrosis.Citation57,Citation60,Citation61 HMLD has been reported in hard metal manufacturing and oil and mining/drilling industries, where workers were exposed to WC-Co dusts or fumes on a daily basis for a number of years prior to diagnosis.Citation44,Citation51,Citation62–Citation65 Currently, treatments are limited and no disease-specific therapy or diagnostic tool exists. There has been some success in treating HMLD with corticosteroids or immunosuppressive therapies,Citation66,Citation67 typically resulting in moderate attenuation of breathing challenges and delayed onset of further symptoms (ie, fibrosis and fluid in the lungs), but in most cases, avoidance of further hard metal exposure is the recommended course of action.
It has recently been established that the defining characteristic of HMLD is the presence of “bizarre, cannibalistic, multinucleated giant cells” in lung biopsy specimens of workers exposed to WC-Co.Citation57,Citation61,Citation63,Citation65,Citation68,Citation69 These giant cell complexes are thought to originate from macrophages which have engulfed WC-Co particles, which then stimulate inflammatory and fibrotic processes in the surrounding lung tissue.Citation57,Citation63,Citation69 In some cases, WC-Co particle “deposits” have been found in biopsy specimens, confirming causative exposure to WC-Co dust and HMLD diagnosis.Citation62,Citation63,Citation69,Citation70 It has been hypothesized that there may be an allergic reaction or a genetic predisposition which may contribute to the development of HMLD in certain individuals.Citation71
There is also accumulating evidence that patients with HMLD are at a twofold increased risk of developing lung cancer.Citation54,Citation56 It has been argued that the generation of reactive oxygen species by WC-Co particles, which may directly cause DNA damage, along with the ability of cobalt ions to inhibit DNA repair mechanisms, may play a synergistic role in the development of lung cancer in HMLD patients.Citation56 However, this hypothesis is yet to be verified in vivo and the relationship between the inflammatory disease state and development of lung cancer remains unclear.
Defining hard metal (WC-Co) toxicity: in vitro and in vivo studies
Early data concerning the effects of inhaled WC-Co dusts first emerged in the 1960s and continued through the 1980s, providing researchers the foundation to further explore the toxic effects of hard metal exposure using in vitroCitation71–Citation88 and in vivoCitation72,Citation89–Citation96 models. Although cobalt itself was originally considered the causative agent of HMLD, several studies demonstrated that this is not the case and the disease mainly develops due to the simultaneous presence of WC with Co.Citation71,Citation74,Citation76–Citation79,Citation90,Citation91 It is currently understood that the combina tion of WC-Co is more toxic than Co, W, or WC particles alone, both in vitro and in vivo,Citation71,Citation72,Citation74,Citation78,Citation79,Citation81,Citation85,Citation90,Citation91,Citation93,Citation94,Citation97–Citation99 but the reason for this enhanced toxicity is still not well defined.
On the in vitro side, the effects of direct WC-Co particle exposure were examined by a number of research groups in terms of cell viability, apoptogenic potential, genotoxicity, oxidative stress, and DNA damage in multiple cell lines of various origins. The major outcomes of these early in vitro studies are summarized in , where the cell type, particle size, and dosage parameter is noted for each study. In alveolar macrophages, 2 μm WC-Co particles caused significant toxicity after 24 h of exposure at concentrations ranging from 50 to 1,667 μg/mL, but were less toxic toward type II pneumocytes under these conditions,Citation72,Citation99 confirming pulmonary WC-Co toxicity in a relevant in vitro model. Toxicity has also been reported in mouse peritoneal macrophages, where WC-Co particles ranging from 2 to 4 μm caused toxicity within 6 h of exposure at concentrations ranging from 50 to 300 μg/mL.Citation79,Citation98,Citation100 In human peripheral blood mononuclear cells, exposure to micron-sized WC-Co particles caused apoptosis, DNA damage, genotoxicity, and alterations in gene expression after exposure times as short as 15 min and up to 6 h.Citation81,Citation82,Citation92,Citation101 Additionally, a number of these studies compared the toxicity of WC-Co, WC, or Co particles and determined that the interaction of WC with Co significantly enhances the toxicity of the composite compared to any single component.Citation71,Citation74,Citation79,Citation101
Table 4 Summary of in vitro WC-Co toxicity studies
There are fewer studies that have examined the effects of nano-WC-Co particles; however, nano-WC-Co toxicity has been reported in human keratinocytes, liver carcinoma cells, oligodendroglial precursor cells, and neurons, at concentrations ranging from 3 to 30 μg/mL and exposure times from 1 h up to 3 days.Citation74,Citation75,Citation78 Internalization of nano-WC-Co has been reported in the keratinocytes (epidermal cells) after 2 days of exposure, which suggested that nano-WC-Co could potentially be absorbed through the skin.Citation74,Citation75 Nano-WC-Co toxicity has also been reported in rainbow trout gill cells, murine epidermal cells, and fibroblasts at concentrations <100 μg/mL for 3 h and up to 3 days.Citation83,Citation84 Nano-WC-Co has been shown to exert genotoxic effects by affecting the expression of genes involved in cellular apoptosis and stress responses.Citation75 In addition, nano-WC-Co has been found to induce greater cellular toxicity and higher levels of oxidative stress than micro-WC-Co particles of the same composition under identical conditions ().Citation86 These studies are consistent with the other reports where the enhanced toxicity of nano-sized over micro-sized particles has been clearly established.Citation22,Citation26,Citation102,Citation103
Figure 2 Cell viability after (A) nano-WC-Co and (B) micro-WC-Co particle exposure and (C) oxidative stress indicated by DCF fluorescence after exposure to 1,000 μg/mL nano- and micro-WC-Co particles.Citation86 *P<0.05, #P<0.001 compared to control, ‡P<0.05 compared to micro-WC-Co.
Note: Reproduced from Armstead AL, Arena CB, Li B. Exploring the potential role of tungsten carbide cobalt (WC-Co) nanoparticle internalization in observed toxicity toward lung epithelial cells in vitro. Toxicol Appl Pharmacol. 2014;278(1):1–8.Citation86
Abbreviations: DCF, 2′,7′-dichlorofluorescein; WC-Co, tungsten carbide cobalt.
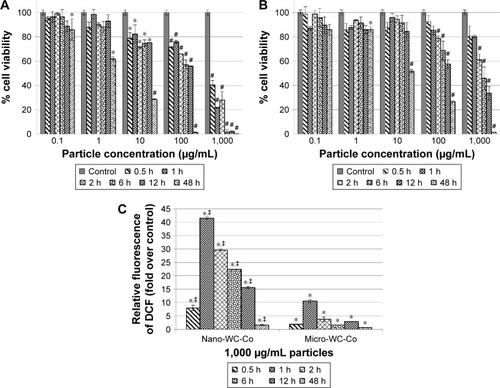
Although the effects of WC-Co particles have been established in multiple cell lines of varying origin, most of these studies used mono-culture systems in their examination of WC-Co particle toxicity, which may not provide an accurate assessment of what happens during/after WC-Co exposure since the local environment of the lung is highly dynamic and contains more than a single cell type. In a recent study,Citation87 the inflammatory response toward nano-WC-Co particles in macrophages and the toxicity of WC-Co have been examined using a co-culture model of lung epithelial cells and macrophages to more closely represent the dynamic tissue environment of the lung. Nano-WC-Co exposure has stimulated an inflammatory response in macrophages, marked by high levels of interleukin-12 (IL-12) and IL-1β secretion.Citation87 In HMLD, lung inflammation and fibrosis occur in a progressive fashion; hence, it has been speculated that the induction of a pro-inflammatory response in macrophages may be an important factor in HMLD. This idea is supported by another study which has indicated that IL-1 in particular may play a role in pulmonary fibrosis,Citation104 and it seems reasonable to suggest that WC-Co may induce a similar type of pulmonary inflammatory response which promotes lung fibrosis after inhalation.
The large majority of previous WC-Co animal studies summarized in employed the IT exposure model as the preferred means of delivery for hard metal particles and “dust” in vivo.Citation5,Citation72,Citation89–Citation92,Citation94 As noted in , the in vivo studies examined the effects of WC-Co particles in the micron-size range, using the mass-per-body weight dosing scheme (ie, mg/kg or mg/g), although some variation in particle size was noted in most cases (overall, WC-Co size ranging from 0.1 to 6 μm). Specifically, micro-sized WC-Co particle exposure caused significant pulmonary inflammation in rats, marked by increased bronchoalveolar lavage (BAL) fluid parameters such as lactate dehydrogenase (LDH) and albumin content, compared to control animals, in as little as 24 h after delivery the of WC-Co IT bolus.Citation72,Citation91,Citation92,Citation95 Significant pulmonary edema, interstitial lung fibrosis, alveolar congestion, and alveolitis were also reported in micro-sized WC-Co-exposed animals at 1 and 6 months following a single IT exposure.Citation89,Citation90,Citation95 Given this body of literature, the local effects of micro-sized WC-Co following pulmonary exposure have been characterized relatively well, and it is understood that micro-sized WC-Co exerts both acute and chronic effects in vivo.
Table 5 Summary of in vivo WC-Co toxicity studies
The local toxicity and potential systemic effects resulting from pulmonary nano-WC-Co exposure have recently been investigated in rats, and surprisingly, an overall lack of toxicity and pulmonary inflammation after 24 h exposure to a single dose of nano-WC-Co (50, 250, or 500 μg) has been observed, while exposure to CeO2 (400 μg) NPs has resulted in significant increases in the BAL fluid parameters examined (ie, LDH, albumin, and macrophage activation) ().Citation96 This outcome seems to be perplexing, as most evidence regarding the effects of WC-Co exposure thus far has suggested that nano-WC-Co should cause acute toxicity both in vivo and in vitro, and the doses selected were based on the known literature for other NP generating pulmonary toxicity. The inflammatory response might have been missed since only a single exposure time (24 h) has been studied, and it is possible that a single exposure at these concentrations in vivo did not generate enough pulmonary toxicity/inflammation to be effectively detected through the BAL technique;Citation96 toxicity and inflammation may have occurred but not at detectable levels after the 24 h exposure time. It is also possible that the effects of nano-WC-Co exposure may occur in a cumulative fashion (ie, toxicity is observed after repeated or chronic exposures) in vivo; hence, multiple/repeated exposure may be a prerequisite for WC-Co toxicity in whole animals.
Figure 3 Pulmonary inflammation parameters assessed in the BAL fluid following 24 h exposure to WC-Co and CeO2 NPs: (A) LDH activity, (B) albumin, and (C) AM chemiluminescence.Citation96 Values presented as mean ± SD. *P<0.05, ‡P<0.001 compared to the vehicle control, and #P<0.01 compared to WC-Co NP exposed groups.
Notes: Reproduced from Armstead AL, Minarchick VC, Porter DW, Nurkiewicz TR, Li B. Acute inflammatory responses of nanoparticles in an intra-tracheal instillation rat model. PLoS One. 2015;10(3):e0118778.Citation96
Abbreviations: BAL, bronchoalveolar lavage; LDH, lactate dehydrogenase; AM, alveolar macrophage; SD, standard deviation; NP, nanoparticle; WC-Co, tungsten carbide cobalt.
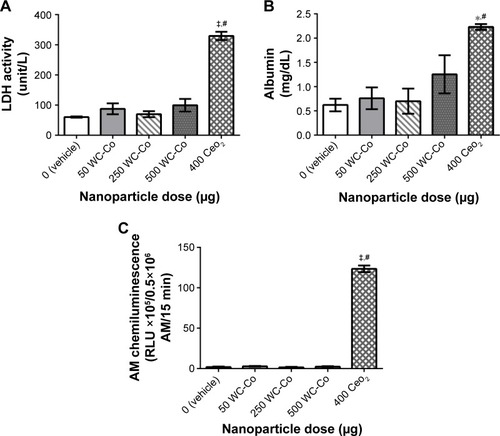
Summary
NP exposure can occur in various consumer and industrial settings through various means such as inhalation, ingestion, or dermal routes. NP exposure, either via internal or external routes, can produce detrimental effects and has been studied both in vitro and in vivo using cell or animal-based models. Occupational exposure to hard metals such as WC-Co is an important concern in the field of nanotoxicology, since inhalation of WC-Co dusts and particles is known to cause HMLD, marked by progressive lung inflammation, fibrosis, and an increased risk of lung cancer. The current body of research clearly demonstrates that WC-Co particles are highly toxic and are capable of inducing oxidative stress and genotoxicity following exposure, and highlights the detrimental effects of WC-Co NP exposure in occupational settings, including hard metal manufacturing facilities and mining and drilling industries. It is important to define occupational WC-Co exposure limits so that future generations of workers are protected from this harmful disease. However, the pathology of HMLD and presence of the “hallmark” multinucleated giant cells have yet to be successfully reproduced in any animal models. Therefore, future studies should further investigate the mechanism underlying acute and chronic WC-Co toxicity and progression to HMLD in exposed workers.
Acknowledgments
We acknowledge the financial support from WV NASA EPSCoR, AO Foundation (Project S-13-15L), Osteosynthesis and Trauma Care Foundation, and Orthopaedic Research and Education Foundation. Fellowship funding to A. Armstead was provided by the West Virginia University NANOSAFE graduate fellowship program 2010–12 (formerly WVNano; NSF Cooperative Agreement #1003907) and by the American Foundation for Pharmaceutical Education (Pre-Doctoral Fellowship in Pharmaceutical Science, 2012–14). The authors acknowledge the WVU Flow Cytometry Core facility, operated by Kathy Brundage, which is supported by the National Institutes of Health equipment grant number S10OD016165 and the Institutional Development Award (IDeA) from the National Institute of General Medical Sciences of the National Institutes of Health under grant numbers P30GM103488 (CoBRE) and P20GM103434 (INBRE).
Disclosure
The authors report no conflicts of interest in this work.
References
- SchrandAMDaiLSchlagerJJHussainSMToxicity testing of nanomaterialsAdv Exp Med Biol2012745587522437813
- HansenSFBaunAMichelsonESKamperABorlingPStuer-LauridsenFNanomaterials in consumer products: categorization and exposure assessmentNanomate rials: Risks and BenefitsLinkovISteevensJDordrecht, the NetherlandsSpringer2009359367
- StammHGibsonNAnklamEDetection of nanomaterials in food and consumer products: bridging the gap from legislation to enforcementFood Addit Contam Part A Chem Anal Control Expo Risk Assess20122981175118222725966
- de SilvaMNNanotechnology and nanomedicine: a new horizon for medical diagnostics and treatmentArch Soc Esp Oftalmol200782633133417573640
- WangQYanJYangJLiBNanomaterials promise better bone repairMater Today2016198451463
- WangXDingBLiBBiomimetic electrospun nanofibrous structures for tissue engineeringMater Today (Kidlington)201316622924125125992
- ZhaoJCastranovaVToxicology of nanomaterials used in nanomedicineJ Toxicol Environ Health B Crit Rev201114859363222008094
- CrommelinDJAParkKFlorenceAPharmaceutical nanotechnology: unmet needs in drug deliveryJ Control Release2010141326326419945491
- LuoHJiangBLiBLiZJiangBHChenYCKaempferol nanoparticles achieve strong and selective inhibition of ovarian cancer cell viabilityInt J Nanomedicine201273951395922866004
- ArmsteadALLiBYNanomedicine as an emerging approach against intracellular pathogensInt J Nanomedicine201163281329322228996
- CasseeFRvan BalenECSinghCExposure, health and ecological effects review of engineered nanoscale cerium and cerium oxide associated with its use as a fuel additiveCrit Rev Toxicol201141321322921244219
- LynamDRPfeiferGDFortBFGelbckeAAEnvironmental assessment of MMT fuel additiveSci Total Environ1990931071142113712
- MadlAKUniceKKreiderMKovochichMBebenekIGHealth risk ranking framework for the life cycle of nanomaterial-containing products: comparison of industrial versus consumer application settingsInt J Toxicol20133216569
- YanJHLiBYLiuXBNano-porous sulfur-polyaniline electrodes for lithium-sulfur batteriesNano Energy201518245252
- YanJHLiuXYaoMWangXWafleTKLiBLong-life, high-efficiency lithium-sulfur battery from a nanoassembled cathodeChem Mater2015271450805087
- YanJHLiuXWangXLiBLong-life, high-efficiency lithium/sulfur batteries from sulfurized carbon nanotube cathodesJ Mater Chem A20153181012710133
- YanJHLiuXBLiBYNano-assembled Na2FePO4F/carbon nanotube multi-layered cathodes for Na-ion batteriesElectrochem Commun2015564650
- Adlakha-HutcheonGKhaydarovRKorensteinRNanomaterials, nanotechnology: applications, consumer products, and benefitsLinkovISteevensJNanomaterials: Risks and BenefitsDordrecht, the NetherlandsSpringer2009195207
- Consumer Product Summary: Project on Emerging Nanotechnologies Available from: http://www.nanotechproject.org/cpi/about/analysis/Accessed September 14, 2014
- EckelmanMJMauterMSIsaacsJAElimelechMNew perspectives on nanomaterial aquatic ecotoxicity: production impacts exceed direct exposure impacts for carbon nanotoubesEnviron Sci Technol20124652902291022296240
- GaiserBKFernandesTJepsonMLeadJRTylerCRStoneVAssessing exposure, uptake and toxicity of silver and cerium dioxide nanoparticles from contaminated environmentsEnviron Health20098Suppl 1S220102587
- BuzeaCPachecoIIRobbieKNanomaterials and nanoparticles: sources and toxicityBiointerphases200724Mr17Mr7120419892
- KeeganGMLearmonthIDCaseCPA systematic comparison of the actual, potential, and theoretical health effects of cobalt and chromium exposures from industry and surgical implantsCrit Rev Toxicol200838864567418720105
- SansoneVPaganiDMelatoMThe effects on bone cells of metal ions released from orthopaedic implants. A reviewClin Cases Miner Bone Metab2013101344023858309
- SimeonovaPPErdelyAEngineered nanoparticle respiratory exposure and potential risks for cardiovascular toxicity: predictive tests and biomarkersInhal Toxicol200921Suppl 1687319558236
- CattaneoAGGornatiRSabbioniENanotechnology and human health: risks and benefitsJ Appl Toxicol201030873074421117037
- StapletonPAMinarchickVCMcCawleyMKnucklesTLNurkiewiczTRXenobiotic particle exposure and microvascular endpoints: a call to armsMicrocirculation201219212614221951337
- NelAEMädlerLVelegolDUnderstanding biophysicochemical interactions at the nano-bio interfaceNat Mater20098754355719525947
- SongYLiXWangLNanomaterials in humans: identification, characteristics, and potential damageToxicol Pathol201139584184921768271
- NurkiewiczTRPorterDWHubbsAFPulmonary particulate matter and systemic microvascular dysfunctionRes Rep Health Eff Inst2011164348
- GillHSGrammatopoulosGAdsheadSTsialogiannisETsiridisEMolecular and immune toxicity of CoCr nanoparticles in MoM hip arthroplastyTrends Mol Med201218314515522245020
- InghamEFisherJBiological reactions to wear debris in total joint replacementProc Inst Mech Eng H2000214H1213710718048
- DonaldsonKSchinwaldAMurphyFThe biologically effective dose in inhalation nanotoxicologyAcc Chem Res201346372373223003923
- HubbsAFMercerRRBenkovicSANanotoxicology – a pathologist’s perspectiveToxicol Pathol201139230132421422259
- LisonDViettiGvan den BruleSParacelsus in nanotoxicologyPart Fibre Toxicol20141113525138533
- TakharPMahantSIn vitro methods for nanotoxicity assessment: advantages and applicationsArch Appl Sci Res201132389403
- KrollAPillukatMHHahnDSchnekenburgerJInterference of engineered nanoparticles with in vitro toxicity assaysArch Toxicol20128671123113622407301
- LupuARPopescuTThe noncellular reduction of MTT tetrazolium salt by TiO(2) nanoparticles and its implications for cytotoxicity assaysToxicol In Vitro20132751445145023531555
- GuadagniniRHalamoda KenzaouiBWalkerLToxicity screenings of nanomaterials: challenges due to interference with assay processes and components of classic in vitro testsNanotoxicology20159Suppl 1132423889211
- FischerHCChanWCWNanotoxicity: the growing need for in vivo studyCurr Opin Biotechnol200718656557118160274
- HendersonRFDriscollKEHarkemaJRA comparison of the inflammatory response of the lung to inhaled versus instilled particles in F344 ratsFundam Appl Toxicol19952421831977737430
- YaoZStiglichJSudarshanTNanosized WC-Co holds promise for the futureMetal Powder Report19985332633
- YaoZStiglichJSudarshanTNano-grained tungsten carbide-cobalt (WC/Co)Materials Modification Inc.1995127 Available from: http://www.matmod.com/publications/armor_1.pdfAccessed October 21, 2014
- LinnainmaaMKangasJKalliokoskiPExposure to airborne metals in the manufacture and maintenance of hard metal and stellite bladesAm Ind Hyg Assoc J19965721962018615327
- StefaniakABVirjiMADayGACharacterization of exposures among cemented tungsten carbide workers. Part I: Size-fractionated exposures to airborne cobalt and tungsten particlesJ Expo Sci Environ Epidemiol200919547549118628793
- National Toxicology ProgramCobalt-tungsten carbide: powders and hard metalsRep Carcinog20111211512021822320
- DemedtsMCeuppensJLRespiratory diseases from hard metal or cobalt exposure. Solving the enigmaChest1989951232909336
- KumagaiSKusakaYGotoSCobalt exposure level and variability in the hard metal industry of JapanAm Ind Hyg Assoc J19965743653698901238
- KusakaYGotoSIs there an occupational exposure limit (TLV) present for cobalt? A lesson from hard metal disease in JapanAdv Prev Occup Respir Dis19981153388392
- KrausTSchramelPSchallerKHZöbeleinPWeberAAngererJExposure assessment in the hard metal manufacturing industry with special regard to tungsten and its compoundsOccup Environ Med2001581063163411555683
- AnttilaSSutinenSPaananenMHard metal lung disease: a clinical, histological, ultrastructural and X-ray microanalytical studyEur J Respir Dis198669283943758243
- GalyPBourretPTolotPPulmonary disorders in the hard metal industryRev Inst Hyg Mines (Hasselt)19742931301374478115
- HartungMEtiology, pathogenesis and clinical aspects of hard metal fibrosis of the lungPneumologie199044249542183208
- LasfarguesGWildPMoulinJJLung cancer mortality in a French cohort of hard-metal workersAm J Ind Med19942655855957832207
- Meyer-BischCPhamQTMurJMRespiratory hazards in hard metal workers: a cross sectional studyBr J Ind Med19894653023092787666
- MoulinJJWildPRomaziniSLung cancer risk in hard-metal workersAm J Epidemiol199814832412489690360
- NemeryBVerbekenEKDemedtsMGiant cell interstitial pneumonia (hard metal lung disease, cobalt lung)Semin Respir Crit Care Med200122443544816088691
- RivoltaGNocoliEFerrettiGTomasiniMHard metal lung disorders: analysis of a group of exposed workersSci Total Environ19941501–31611657939591
- RochatTKaelinRMBatawiAJunodAFRapidly progressive interstitial lung disease in a hard metal coating worker undergoing hemodialysisEur J Respir Dis198771146513653303
- ForniABronchoalveolar lavage in the diagnosis of hard metal diseaseSci Total Environ19941501–369767939611
- KinoshitaMSueyasuYWatanabeHGiant cell interstitial pneumonia in two hard metal workers: the role of bronchoalveolar lavage in diagnosisRespirology19994326326610489670
- DunlopPMüllerNLWilsonJFlintJChurgAHard metal lung disease: high resolution CT and histologic correlation of the initial findings and demonstration of interval improvementJ Thorac Imaging200520430130416282911
- MoriyamaHKobayashiMTakadaTTwo-dimensional analysis of elements and mononuclear cells in hard metal lung diseaseAm J Respir Crit Care Med20071761707717363774
- PotolicchioIMosconiGForniANemeryBSeghizziPSorrentinoRSusceptibility to hard metal lung disease is strongly associated with the presence of glutamate 69 in HLA-DP beta chainEur J Immunol19972710274127439368635
- TanakaJMoriyamaHTeradaMAn observational study of giant cell interstitial pneumonia and lung fibrosis in hard metal lung diseaseBMJ Open201443e004407
- NurekiSMiyazakiENishioSAndoMKumamotoTHard metal lung disease successfully treated with inhaled corticosteroidsIntern Med201352171957196123994991
- EnriquezLSMohammedTLJohnsonGLLeforMJBeasleyMBHard metal pneumoconiosis: a case of giant-cell interstitial pneumonitis in a machinistRespir Care200752219619917261209
- BlancPDIs giant cell interstitial pneumonitis synonymous with hard metal lung disease?Am J Respir Crit Care Med20071768834 author reply 834–83517914126
- NaqviAHHuntABurnettBRAbrahamJLPathologic spectrum and lung dust burden in giant cell interstitial pneumonia (hard metal disease/cobalt pneumonitis): review of 100 casesArch Environ Occup Health2008632517018628077
- TabatowskiKRoggliVLFulkersonWJLangleyRLBenningTJohnstonWWGiant cell interstitial pneumonia in a hard-metal worker. Cytologic, histologic and analytical electron microscopic investigationActa Cytol19883222402463348063
- LisonDLauwerysRDemedtsMNemeryBExperimental research into the pathogenesis of cobalt/hard metal lung diseaseEur Respir J199695102410288793467
- HuauxFLardotCArrasMLung toxicity of hard metal particles and production of interleukin-1, tumor necrosis factor-alpha, fibronectin, and cystatin-c by lung phagocytesToxicol Appl Pharmacol1995132153627747285
- AntoniniJMStarksKRobertsJRMillecchiaLYangHMRaoKMChanges in F-actin organization induced by hard metal particle exposure in rat pulmonary epithelial cells using laser scanning confocal microscopyIn Vitro Mol Toxicol2000131516
- BastianSBuschWKühnelDToxicity of tungsten carbide and cobalt-doped tungsten carbide nanoparticles in mammalian cells in vitroEnviron Health Perspect2009117453053619440490
- BuschWKühnelDSchirmerKScholzSTungsten carbide cobalt nanoparticles exert hypoxia-like effects on the gene expression level in human keratinocytesBMC Genomics2010116520105288
- EdelJSabbioniEPietraRTrace metal lung disease: in vitro interaction of hard metals with human lung and plasma componentsSci Total Environ1990951071172205918
- FenoglioICorazzariIFranciaCBodoardoSFubiniBThe oxidation of glutathione by cobalt/tungsten carbide contributes to hard metal-induced oxidative stressFree Radic Res200842843744518712631
- KuhnelDSchefflerKWellnerPComparative evaluation of particle properties, formation of reactive oxygen species and genotoxic potential of tungsten carbide based nanoparticles in vitroJ Hazard Mater2012227–228418426
- LisonDCarbonnellePMolloLLauwerysRFubiniBPhysicochemical mechanism of the interaction between cobalt metal and carbide particles to generate toxic activated oxygen speciesChem Res Toxicol1995846006067548741
- LisonDLauwerysRStudy of the mechanism responsible for the elective toxicity of tungsten carbide-cobalt powder toward macrophagesToxicol Lett19926022032101570634
- LombaertNCastrucciEDecordierIHard-metal (WC-Co) particles trigger a signaling cascade involving p38 MAPK, HIF-1alpha, HMOX1, and p53 activation in human PBMCArch Toxicol201287225926823052192
- LombaertNLisonDVan HummelenPKirsch-VoldersMIn vitro expression of hard metal dust (WC-Co) – responsive genes in human peripheral blood mononucleated cellsToxicol Appl Pharmacol2008227229931218078969
- AnardDKirsch-VoldersMElhajoujiABelpaemeKLisonDIn vitro genotoxic effects of hard metal particles assessed by alkaline single cell gel and elution assaysCarcinogenesis19971811771849054604
- DingMKisinERZhaoJSize-dependent effects of tungsten carbide-cobalt particles on oxygen radical production and activation of cell signaling pathways in murine epidermal cellsToxicol Appl Pharmacol2009241326026819747498
- ZhangXDZhaoJBowmanLShiXCastranovaVDingMTungsten carbide-cobalt particles activate Nrf2 and its downstream target genes in JB6 cells possibly by ROS generationJ Environ Pathol Toxicol Oncol2010291314020528745
- ArmsteadALArenaCBLiBExploring the potential role of tungsten carbide cobalt (WC-Co) nanoparticle internalization in observed toxicity toward lung epithelial cells in vitroToxicol Appl Pharmacol201427811824746988
- ArmsteadALLiBIn vitro inflammatory effects of hard metal (WC-Co) nanoparticle exposureInt J Nanomedicine20161161956206
- LiuLZDingMZhengJZTungsten carbide-cobalt nanoparticles induce reactive oxygen species, AKT, ERK, AP-1, NF-kappaB, VEGF, and angiogenesisBiol Trace Elem Res20151661576525893364
- KitamuraHYoshimuraYTozawaTKoshiKEffects of cemented tungsten carbide dust on rat lungs following intratracheal injection of saline suspensionActa Pathol Jpn19803022412537386201
- LasfarguesGLardotCDelosMLauwerysRLisonDThe delayed lung responses to single and repeated intratracheal administration of pure cobalt and hard metal powder in the ratEnviron Res19956921081218608770
- LasfarguesGLisonDMaldaguePLauwerysRComparative study of the acute lung toxicity of pure cobalt powder and cobalt-tungsten carbide mixture in ratToxicol Appl Pharmacol1992112141501733047
- De BoeckMHoetPLombaertNNemeryBKirsch-VoldersMLisonDIn vivo genotoxicity of hard metal dust: induction of micronuclei in rat type II epithelial lung cellsCarcinogenesis200324111793180012949052
- FedanJSCutlerDHard metal-induced disease: effects of metal cations in vitro on guinea pig isolated airwaysToxicol Appl Pharmacol2001174319920611485380
- RengasamyAKommineniCJonesJAFedanJSEffects of hard metal on nitric oxide pathways and airway reactivity to methacholine in rat lungsToxicol Appl Pharmacol1999157317819110373402
- AdamisZTátraiEHonmaKKárpátiJUngváryGA study on lung toxicity of respirable hard metal dusts in ratsAnn Occup Hyg19974155155269332157
- ArmsteadALMinarchickVCPorterDWNurkiewiczTRLiBAcute inflammatory responses of nanoparticles in an intra-tracheal instillation rat modelPLoS One2015103e011877825738830
- KerfootEJFredrickWGDomeierECobalt metal inhalation studies on miniature swineAm Ind Hyg Assoc J197536117251111264
- LisonDLauwerysREvaluation of the role of reactive oxygen species in the interactive toxicity of carbide-cobalt mixtures on macrophages in cultureArch Toxicol19936753473518396391
- RoesemsGHoetPHDinsdaleDDemedtsMNemeryBIn vitro cytotoxicity of various forms of cobalt for rat alveolar macrophages and type II pneumocytesToxicol Appl Pharmacol200016212910631122
- LisonDLauwerysRCobalt bioavailability from hard metal particles. Further evidence that cobalt alone is not responsible for the toxicity of hard metal particlesArch Toxicol19946885285317802596
- LombaertNDe BoeckMDecordierICundariELisonDKirsch-VoldersMEvaluation of the apoptogenic potential of hard metal dust (WC-Co), tungsten carbide and metallic cobaltToxicol Lett20041541–2233415475175
- AlbaneseATangPSChanWCThe effect of nanoparticle size, shape, and surface chemistry on biological systemsAnnu Rev Biomed Eng20121411622524388
- GeiserMMorphological aspects of particle uptake by lung phagocytesMicrosc Res Tech200257651252212112434
- GauldieJJordanaMCoxGCytokines and pulmonary fibrosisThorax19934899319358236078
- ParkBDonaldsonKDuffinRHazard and risk assessment of a nanoparticulate cerium oxide-based diesel fuel additive – a case studyInhal Toxicol200820654756618444008
- PapageorgiouIBrownCSchinsRThe effect of nano- and micron-sized particles of cobalt-chromium alloy on human fibroblasts in vitroBiomaterials200728192946295817379299
- PapageorgiouIShadrickVDavisSMacrophages detoxify the genotoxic and cytotoxic effects of surgical cobalt chrome alloy particles but not quartz particles on human cells in vitroMutat Res20086431–2111918614183
- PapageorgiouIYinZLadonDGenotoxic effects of particles of surgical cobalt chrome alloy on human cells of different age in vitroMutat Res20076191–2455817376492
- SunLLiYLiuXCytotoxicity and mitochondrial damage caused by silica nanoparticlesToxicol In Vitro20112581619162921723938
- ParkEJYiJChungKHRyuDYChoiJParkKOxidative stress and apoptosis induced by titanium dioxide nanoparticles in cultured BEAS-2B cellsToxicol Lett2008180322222918662754
- WilhelmiVFischerUvan BerloDSchulze-OsthoffKSchinsRPAlbrechtCEvaluation of apoptosis induced by nanoparticles and fine particles in RAW 264.7 macrophages: facts and artefactsToxicol In Vitro201226232333422198050
- BlumJLRosenblumLKGrunigGBeasleyMBXiongJQZelikoffJTShort-term inhalation of cadmium oxide nanoparticles alters pulmonary dynamics associated with lung injury, inflammation, and repair in a mouse modelInhal Toxicol2014261485824417406
- PauluhnJSubchronic inhalation toxicity of iron oxide (magnetite, Fe(3) O(4)) in rats: pulmonary toxicity is determined by the particle kinetics typical of poorly soluble particlesJ Appl Toxicol201232748850421456093
- MinarchickVCStapletonPAPorterDWPulmonary cerium dioxide nanoparticle exposure differentially impairs coronary and mesenteric arteriolar reactivityCardiovasc Toxicol201313432333723645470
- ChoiMChoMHanBSChitosan nanoparticles show rapid extrapulmonary tissue distribution and excretion with mild pulmonary inflammation to miceToxicol Lett2010199214415220816729
- BrownCLacharme-LoraLMukonoweshuroBConsequences of exposure to peri-articular injections of micro- and nano-particulate cobalt-chromium alloyBiomaterials201334348564858023932295
- WangZChenZZuoQReproductive toxicity in adult male rats following intra-articular injection of cobalt-chromium nanoparticlesJ Orthop Sci20131861020102624085379
- GaiserBKBiswasARosenkranzPEffects of silver and cerium dioxide micro- and nano-sized particles on Daphnia magnaJ Environ Monit20111351227123521499624
- De BoeckMLombaertNDe BackerSFinsyRLisonDKirsch-VoldersMIn vitro genotoxic effects of different combinations of cobalt and metallic carbide particlesMutagenesis200318217718612621074
- KuhnelDBuschaWMeißnerbTAgglomeration of tungsten carbide nanoparticles in exposure medium does not prevent uptake and toxicity toward a rainbow trout gill cell lineAquat Toxicol2009932–3919919439373
- LisonDLauwerysRIn vitro cytotoxic effects of cobalt-containing dusts on mouse peritoneal and rat alveolar macrophagesEnviron Res19905221871982168316
- LisonDLauwerysRBiological responses of isolated macrophages to cobalt metal and tungsten carbide-cobalt powdersPharmacol Toxicol19916942822851659697
- LisonDLauwerysRThe interaction of cobalt metal with different carbides and other mineral particles on mouse peritoneal macrophagesToxicol In Vitro19959334134720650096