Abstract
Tumors are one of the most serious human diseases and cause numerous global deaths per year. In spite of many strategies applied in tumor therapy, such as radiation therapy, chemotherapy, surgery, and a combination of these treatments, tumors are still the foremost killer worldwide among human diseases, due to their specific limitations, such as multidrug resistance and side effects. Therefore, it is urgent and necessary to develop new strategies for tumor therapy. Recently, the fast development of nanoscience has paved the way for designing new strategies to treat tumors. Nanomaterials have shown great potential in tumor therapy, due to their unique properties, including passive targeting, hyperthermia effects, and tumor-specific inhibition. This review summarizes the recent progress using the innate antitumor properties of metallic and nonmetallic nanomaterials to treat tumors, and related challenges and prospects are discussed.
Introduction
The development of tumors occurs at the molecular levelCitation1 when multiple subgroups of genes undergo genetic alterations. Either tumor-suppressor genes are inactivated or oncogenes are activated, which leads to malignant proliferation of tumor cells, tissue infiltration, and organ dysfunction.Citation2 Many methods have been developed for tumor therapy, such as immunotherapy,Citation3,Citation4 gene therapy,Citation5,Citation6 and radiofrequency ablation (RFA).Citation7–Citation9 Nevertheless, traditional radiotherapy,Citation10,Citation11 surgery,Citation12–Citation14 and especially chemotherapyCitation15,Citation16 are still the major strategies utilized in clinical tumor treatments. However, chemotherapy has a number of problems, including nonspecific distribution of the drug and the lack of tumor-specific treatments.Citation17 In the continuous fight against tumors, patients have to be administered drugs that increase in dose over time, which is associated with considerable adverse effects, such as multidrug resistance and accumulative systemic toxicity. Chemotherapy also causes side effects in healthy tissues, which have negative impacts on the quality of life of the patients.Citation18,Citation19 In addition, chemotherapy may concomitantly damage adjacent organs or tissues, and these adverse effects are difficult to avoid.Citation20,Citation21
In recent decades, the development of nanotechnology has provided an opportunity to overcome the aforementioned side effects. The integration of various nanomaterials (NMs) with spectroscopic, biochemical, and optical methods has allowed the development of advanced methods for tumor therapy, which may revolutionize the treatment of tumors. An emerging approach is to utilize nanotechnology and NMs to minimize toxicity to healthy tissues. Various NMs have performed attractively in various aspects of antitumor treatment and garnered intense research interest, and they have presented great potential for antitumor treatment by enhancing the effectiveness of treatments and reducing systemic side effects.
The term “nano-” is derived from the Greek noun nanos,Citation22 and a nanometer (nm) is equivalent to 10−9 m.Citation23 The specific physical and/or chemical properties of NMs make them very attractive in biomedical applications.Citation24,Citation25 Over the past few decades, the explosion of studies involving NMs can be ascribed to their special optical, thermodynamic, magnetic, and mechanical properties.Citation26–Citation28 Potential medical applications of NMs have been recognized to have good biocompatibility.Citation29 Nanoparticles (NPs) with suitable diameter range have been reported to be able to enter blood circulation and undergo endocytosis into cells.Citation30 Furthermore, scientific research shows that NM-based therapeutics have great potential in the treatment of tumors, diabetes, infection, neurodegenerative disease, and inflammation.Citation31–Citation33 The integration of tumor therapy with NMs is expected to bring about new breakthroughs for nanotechnology in the field of medicine.
The physical and chemical properties of NMs impact on biodistribution, cellular processes, and cytotoxic effects.Citation27,Citation34,Citation35 For example, the size of the NM affects cellular tropism by facilitating or inhibiting actin polymerization.Citation36 In addition to size, the shape of NMs influences organ and cellular tropism.Citation37 Spherical NMs promote phagocytosis of macrophages, but disk-shaped structures do not. By tailoring these aspects, specific properties of NMs can be used to design new biomedical applications, which include various clinical and research activities and modulation of processes in target cells.Citation38 With the development of nanotechnology, the integration of NMs into tumor therapeutics is one of the rapidly advancing fields, and more new NMs will be increasingly used in the field of tumor treatment.
Based on nanotechnology, various NMs have been developed, such as iron oxide NPs, gold NPs (AuNPs), cerium oxide NPs, carbon-based materials, polymeric NPs, and quantum dots (QDs).Citation39–Citation44 NMs have shown great potential in tumor prevention, detection, diagnosis, imaging, and treatment. Due to their specific physical, chemical, and biological properties and unique structure, NMs are able to work at the molecular level. Therefore, NMs themselves are antigenic or medicinally active, and can stimulate a strong immune response in the body.Citation45 Some NMs can kill or inhibit pathogenic cell types by exploiting their autoimmunity or cellular toxicity (selective block by toxicity).Citation46–Citation48 Various NMs themselves are used as potential antitumor drugs, and are being developed as photothermal therapy (PTT) agents.Citation49,Citation50 Therefore, this review discusses some specific metallic NMs (MNMs) and nonmetallic NMs that act as a medicine or drug additive in tumor therapy ().
Metallic NMs
MNMs are metal or metal oxide nanocrystals. MNMs are readily prepared, have surfaces relatively easy to modify, and in general have low toxicity.Citation51,Citation52 Because of their unique properties, the advantage of using MNMs lies primarily in their ability to interact directly with cell membranes and intracellular structures.Citation41,Citation42 As discussed earlier, these special properties make MNMs useful for numerous diagnostic and therapeutic applications.Citation53 Most MNMs for tumor therapy consist of iron oxide, gold NPs, and cerium oxide NPs ().Citation54–Citation57
Figure 2 Transmission electron microscope images of (A) iron oxide nanoparticles; (B) gold nanoparticles; (C) gold nanocages; (D) cerium oxide nanoparticles.
Notes: Figure A adapted with permission from Bronstein LM, Huang XL, Retrum J, et al. Influence of iron oleate complex structure on iron oxide nanoparticle formation. Chem Mater. 2007;19(15):3624–3632. Copyright © 2007, American Chemical Society.Citation54 Figure B adapted with permission from Schulz-Dobrick M, Sarathy KV, Jansen M. Surfactant-free synthesis and functionalization of gold nanoparticles. J Am Chem Soc. 2005;127(37):12816–12817. Copyright © 2007, American Chemical Society.Citation55 Figure C adapted with permission from Chen JY, Wang DL, Xi JF, et al. Immuno gold nanocages with tailored optical properties for targeted photothermal destruction of cancer cells. Nano Lett. 2007;7(5):1318–1322. Copyright © 2007, American Chemical Society.Citation56 The method reported in Lee et alCitation57 was used when creating the nanoparticles shown in Figure D.
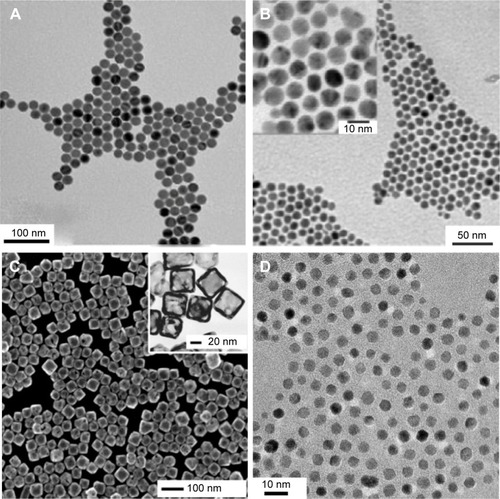
Iron oxide
Iron oxide is a type of magnetic material that is used in tumor PTT and targeted therapy. PTT is based on using optical heating to facilitate direct and specific destruction of tumor cells.Citation58 PTT is a highly promising approach to tumor therapy. Phototherapy employs near-infrared light (NIR)-absorbing agents with high absorption in the NIR region, which then release vibrational energy (heat) to kill the targeted tumor cells.
Fe3O4-based magnetic NPs are recognized as promising hyperthermia-specific agents. To treat metastatic bone tumors, Matsumine et alCitation59 used novel Fe3O4 powder for hyperthermia-induced apoptosis in several patients. One week later, after incorporating Fe3O4 in the adhesive that repaired the bone, patients were subjected to a localized electromagnetic field at a temperature estimated to be 40°C–45°C, in order to improve the lesion. This treatment stopped further metastatic progression, and demonstrated the benefit of using iron oxide in bone-tumor treatments.
In addition to PTT, potential medical applications of magnetic NPs have also been approved, due to their good biocompatibility.Citation26 According to a recent report, magnetic NPs with diameters ranging between several (~9 nm) and hundreds (~200 nm) of nanometers can circulate in blood vessels and undergo endocytosis into cells.Citation60 The authors also found that dimercaptosuccinic acid-coated iron oxide NPs have excellent potential for applications that target breast-tumor cells.
Furthermore, Fe3O4 NPs can be functionalized using antibodies and achieve active targeted tumor therapy. There is a report of using anti-HER2-conjugated polypyrrole-Fe3O4 NPs to target and damage SK-BR-3 breast tumor cells in vitro.Citation61 In fact, superparamagnetic iron oxide NPs (SPIONs; Fe3O4 or Fe2O3)Citation62 have been recognized for clinical use in the form of ferucarbotran (carboxydextran SPIONs), ferumoxides (dextran SPIONs), and ferumoxtran 10 (dextran SPIONs).Citation63 These SPIONs are highly biodegradable and biocompatible; the iron core is recycled into soluble ferritin iron or hemosiderin.Citation64 Iron oxide NPs are also intensively used in imaging system as contrast agents in pathophysiological studies.Citation65
Gold NMs
In recent years, AuNMs have shown important potential in various biomedical and research applications, such as catalysis, bioanalysis, and imaging.Citation66,Citation67 The preparation technology is simple, and it is easy to control the size and shape of AuNMs.Citation68,Citation69 It has been reported that AuNMs can selectively and specifically recognize tumor cells.Citation70,Citation71 Studies reported in the literature have shown that AuNMs are easily functionalized, increase the time of circulation, and are stable in systemic circulation. In addition, AuNMs have low toxicity and protect drugs from potential transformation in tissues and organs. Perhaps the most important property of AuNMs is their ability to destroy tumor cells.Citation72 Thus, such AuNMs as AuNPsCitation73,Citation74 and gold nanocagesCitation56 are a promising tool for selectively killing tumor cells through photothermal (PT) damage.
AuNPs can enhance the apoptotic effect of tumors,Citation7 which makes it possible to decrease radiation doses, thereby reducing side effects on surrounding normal tissues. AuNPs can also enhance the effect of radiotherapy, which has been verified in vivo and in vitro in animal models of tumors.Citation72 AuNPs are commonly used as nanobioconjugates.Citation69,Citation70,Citation73 The functionalization of NPs (eg, with polyethylene glycol [PEG] or antibodies) allows the nanobioconjugates to remain in the bloodstream and preferentially accumulate in tumor tissue. These nanobioconjugates have the ability to penetrate the cell nucleus, increasing the likelihood that the active cytostatic substance acts directly on DNA.Citation74 Studies reported in the literature have shown that multifunctional AuNPs can be used both to image and treat tumors.Citation70
RFA is a novel therapeutic method for the treatment of tumors. This method uses imaging guidance to place a needle electrode either through the skin or directly (during surgery) into the pathological tissue. High-frequency electrical currents pass through the electrode and create heat that destroys tumor cells. AuNPs have been used in this field, making it possible to destroy tumor cells noninvasively by RFA.Citation12–Citation14 The optical properties of nanoshells can be utilized in tumor-ablation techniques with NIR light or RFA. Laroui et al developed nanoshells coated with gold, absorbing specific wavelengths of light.Citation75 Fortina et al synthesized nanoshells linked to a ligand.Citation76 Citrate-coated gold has been shown to have antitumor properties.Citation77,Citation78
Xia et alCitation56 synthesized novel gold nanocages ~45 nm in edge length. These gold nanocages have shown potential for targeted PT inhibition of tumor cells. The nanocages were designed to convert 810 nm NIR light to heat via a large absorptive cross section of 3.48×10−14 cm2, and strongly absorbed light in the NIR region. PT studies of the nanocages showed that the PT cell-damage power-density threshold was 1.5 W/cm2, which is lower than that of gold nanoshells (35 W/cm2) and gold nanorods (10 W/cm2). By conjugating the nanocages with monoclonal antibodies (anti-HER2), the nanocages can target epidermal growth factor receptors (EGFRs) that are overexpressed on the surface of breast-tumor cells. These results indicated that shape- and size-modulated AuNMs could be potential tumor PTT reagents.
Cerium oxide nanoparticles
Cerium oxide NPs include a cerium core surrounded by an oxygen lattice.Citation79 In normal cells, these NPs have an antioxidant and cytoprotective role under neutral pH. In an acid medium, which is one of the characteristics of tumor cells, these NPs show prooxidant and cytotoxic effects. Pei et al studied the antitumor effects of cerium oxide NPs in in vitro models, and the results showed that cerium oxide NPs inhibited the growth of tumor cells.Citation80 There has been another report indicating that a high concentration (10 μg/mL) of cerium oxide NPs can suppress tumor-cell migration and inhibit tumor-cell proliferation.Citation81
Quantum dots
Another popular type of NM are QDs.Citation40 QDs are very small nanocrystals that emit fluorescence when stimulated by a specific wavelength of light, and their diameter is approximately 2–10 nm.Citation44,Citation82,Citation83 Their structure consists of an inorganic core and shell, which can be modified with biological molecules, such as PEG.Citation84 Their emission and absorption properties can be controlled precisely by modulating their size and shape.Citation85 The benefit of QDs for tumor therapy is attributed to their mechanisms of photosensitization and radiosensitization. With electronic energy levels in the 1–5 keV range, QDs can be used as photosensitizers for photodynamic treatments, which have become an approved therapy modality for some types of tumors. Because of their high electron and atom density, QDs act as radiosensitizers that absorb high-energy photons and cause localized or targeted damage to tumor cells.Citation86
Nonmetallic NMs
There are several different types of nonmetal NMs (), and carbon compounds are some of the most extensively studied. Several different “pure” carbon-based constructs have been studied, such as fullerenes and their derivatives, which are composed of carbon atoms arranged in a spherical shape called a truncated icosahedron, and carbon nanotubes (CNTs), which are most frequently studied in biological systems.Citation3,Citation87 Fullerenes and their derivatives are readily accessible to cells, and are conjugated with various therapeutic molecules because they have a unique nanostructure. In addition, the pure carbon composition of fullerenes gives them good biocompatibility. Moreover, carbon NMs have been linked with biological molecules and polymers, and so can be effectively used for antitumor applications in vivo.Citation40 Therefore, functional fullerenes and their derivatives can be linked to a wide variety of active molecules to target tumor cells.Citation87,Citation88 Based on these experiments, fullerenes and their derivatives have become a new class of promising candidates for tumor treatments. This portion of the review attempts to provide insight into the progress of the use of fullerenes and their derivatives as tumor-therapy drugs.
Figure 3 Structures of nonmetallic nanomaterials for tumor therapy.
Notes: (A) C60; (B) carbon nanotubes; (C) graphene; (D) chitosan-structure units β-(1,4)-2-amino-d-glucose and β-(1,4)-2-acetamido-d-glucose.
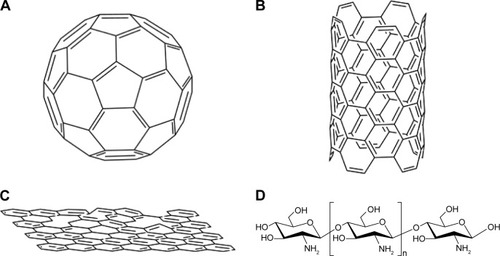
C60
C60 (or “bucky-ball” C60) is a hollow sphere with a diameter of 1 nm, and is made up of 60 carbon atoms. C60 is effective in terms of interactions with the immune system, and has dramatic potential as an antitumor drug. Compared with conventional antitumor chemicals, fullerenes and their derivatives are more efficient at inhibiting the growth of tumors. The result is not due to toxic effects on tumor cells, but the fullerenes, which have almost no toxicity in vivo or in vitro, have a strong ability to enhance immunity and protect normal tissues from tumor invasion.Citation89,Citation90
Endohedral metallofullerenes (fullerenes encapsulating a metal atom) have shown dramatic potential for biomedical applications. For example, Hirahara et alCitation91 synthesized a multihydroxylated fullerene – Gd@C82(OH)22. Compared with some other clinical antitumor drugs, C60(OH)20 and Gd@C82(OH)22 NPs are highly effective at suppressing tumor growth in mice with negligible side effects. The high antitumor activity may be because the water-soluble NMs can effectively trigger the host immune system to eliminate tumor cells and regulate the angiogenesis and oxidative defense system in vivo.Citation92
Carbon nanotubes
CNTs are coaxial graphite layers with cylinders.Citation93 CNTs are formed by polymerization of single carbon atoms under specific conditions.Citation94 According to their structure, CNTs can be divided into two categories: single-walled CNTs (SWCNTs) and multiwalled CNTs (MWCNTs). The former is a single cylindrical carbon wall, and the latter is an MW cylinder nested in other cylinders.Citation95 With the development of nanotechnology, integration of CNTs into tumor therapeutics is a rapidly advancing field.Citation96,Citation97 There are reports that CNTs promote the phagocytosis of dendritic cells at tumor sites, which produce more antigens to dendritic cells, significantly increasing the immunogenicity of proteins in the tumor.Citation98 Similarly, inhaled CNTs can inhibit lung-resident dendritic cells and promote lung immune suppression.Citation47 Regarding the direct therapeutic effect, CNTs can induce tumor-tissue destruction by PTT, based on strong optical absorbance under NIR-light excitation.Citation99,Citation100 Therefore, CNTs have attracted interest as efficient PTT agents for tumor treatments.Citation101–Citation103 Hyperthermia therapy is another type of optional solution for tumor treatment, especially for some solid malignant tumors, such as breast and liver tumors. CNTs are also used as PT tumor-ablation mediators.Citation100 Due to the thermal conductivity and optical properties of CNTs, they have become mediators to optically stimulate NTs that are placed inside living cells and that kill tumor cells via local hyperthermia.
There has been much research to show the feasibility of CNTs in the treatment of malignant tumors. SWCNTs are promising candidates as a PTT agent, because they also have high absorption in the NIR spectrum, which is ideal for inducing PT damage to tumor cells or tissues. Continuous NIR radiation can lead to cell death, because of excessive local heating of SWCNTs. Kam et alCitation104 showed that this approach is effective. In a similar work,Citation105 the authors showed that HER2-IGFR-SWCNTs were able to destroy all targeted tumor cells. MWCNTs also efficiently absorb NIR light and efficiently convert the absorbed energy into thermal energy. MWCNTs can be stimulated with NIR irradiation to damage cervical tumor HeLa cellsCitation106 and EAC cells.Citation107 CNTs make it possible to damage tumor cells noninvasively, and the potential applications for CNTs in tumor therapy have attracted much clinical interest. Therefore, CNTs can be considered another class of tumor PTT system, and are highly promising for clinical trials.
Graphene
Graphene is an emerging NM with single-layered carbon atoms in a two-dimensional honeycomb structure. Graphene has attracted great interest since its tremendous groundbreaking discovery in 2004.Citation108–Citation110 Graphene and its different subtypes, such as graphene oxide (GO) and reduced graphene oxide (RGO), have been extensively studied in the realm of nanomedicine, due to their exceptional physical, chemical, and mechanical properties.Citation111 Graphene-based NMs have a well-described role in tumor management.Citation112 Graphene-based NMs are used mainly for PTT.
Yang et alCitation113 reported that PEGylated GO (GO-PEG) showed highly efficient tumor ablation under NIR-light irradiation, and GO-PEG was used for in vivo PTT. These authors noticed that GO-PEG had no obvious side effects, and was safe during the treatment. In a follow-up study, Yang et alCitation114 verified that PEGylated nano-RGO (nRGO-PEG) with an ultrasmall average diameter of ~27 nm was a better NM than GO for PTT with low-power laser irradiation. The in vivo PTT study demonstrated that nRGO-PEG was more efficient in tumor ablation and that NIR laser-power density was greatly reduced. These results indicated that nRGO-PEG strongly absorbs light in the NIR region (808 nm), with an intensity threshold of 0.15 W/cm2, to induce thermal destruction of tumor cells. There have been some studies that reported that nanoparticles such as AuNPs,Citation115,Citation116 AgNPs,Citation117 copper monosulfide NPs,Citation118 and copper(I) oxide NPsCitation119 attached to the surface of GO synergistically enhanced PTT. These results highlight that both the size and surface chemistry of GO are critical to the effectiveness of in vivo PTT, which indicates that optimized nanographene could be a PTT reagent for treating tumors.
Chitosan nanoparticles
Chitosan is a type of cationic oligosaccharide that is the major component of the exoskeleton of crustaceans and insects.Citation120 Chitosan is a biopolymer containing β-(1,4)-2-amino-d-glucose and β-(1,4)-2-acetamido-d-glucose units.Citation73 Chitosan NPs (ChNPs) are obtained by deacetylation, which removes an acetate moiety from chitin. ChNPs are primarily degraded in the colon by the action of lysozyme and bacterial enzymes, and are finally eliminated in the feces. Chitosan is a natural polysaccharide that has excellent biocompatibility, biosecurity, biodegradability, and nontoxic characteristics.Citation121,Citation122 ChNPs are commonly modified with epichlorohydrin and PEG dicarboxylic acid.Citation123,Citation124 ChNPs also have dramatic potential for tumor therapy.
ChNPs can directly affect the metabolism of tumor cells by inducing apoptosis and inhibiting cellular growth. Likewise, ChNPs play a role in increasing the immune function of the organism by facilitating the contact between the drug and the tumor-cell membrane.Citation125,Citation126 Reports show that ChNPs have high stimulatory activity on B and T lymphocytes and the humoral immune system, which can be activated at the same time.Citation127 ChNPs also have a strong immunostimulating effect by increasing the activation and accumulation of polymorphonuclear and macrophage cells, and they induce cytokine production after intravenous injection.Citation128,Citation129 There is another example that indicates that ChNPs have their own antitumor effects. ChNPs themselves have a positive charge; therefore, they can neutralize the negative charges on the surface of tumor cells, which has a tendency to contribute to their selective uptake. In this way, ChNPs can effectively inhibit proliferation of tumor cells by increasing the concentration of cytostatic agents at the tumor sites.Citation130 All these attributes and examples show that ChNPs have great potential in tumor therapy.
Challenges and future perspectives
Over the last 20 years, NMs have come to play a significant role in commercial development. Indeed, we might expect to produce many breakthroughs and new prospects for the world economy from advances in nanotechnology. With the potentially wide application of NMs in the future, NMs may be extensively used in various fields, especially tumor therapy. NMs can be used for clinical diagnosis and tumor therapy, based on their size, biocompatibility, surface chemistry, relatively good stability, and adjustable toxicity in biological systems. It is highly expected that the application of NMs in tumor therapy will greatly improve current methods of tumor-cell detection, tumor imaging, and tumor therapy, while reducing toxicity compared to traditional tumor treatments.
However, there are several challenges in the field, and there are still controversies about the potential risk of anti-tumor therapies. The most pressing problems are potential chronic and acute toxic effects; the potential toxicity of NMs cannot be ignored in antitumor therapy.Citation131 There have been reports on the toxicity of NMs. NMs may be attached to the surface of biological membranes by adsorption or electrostatic interactions, and they can cause damage to cells by producing reactive oxygen species, leading to protein denaturation, lipid peroxidation, DNA damage, and ultimately cell death.Citation132–Citation134 For instance, NPs and CNTs can damage the respiratory and cardiovascular systems,Citation135,Citation136 and they can enter the centralnervous system through the blood–brain barrier, resulting in a variety of nervous system diseases.Citation137–Citation139 In vitro studies have shown that CNTs can induce cell apoptosis, decrease cell viability, and disrupt the cell cycle and inflammatory responses.Citation140–Citation142 It has been confirmed that CNTs can damage lung tissueCitation143 and that they are toxic to the immune system in mice.Citation46 Besides, the blood incompatibility of CNTs also limits their use in the clinic. Although many studies have shown that the functionalization of CNTs can improve their water-solubility, proof of their biocompatibility and safety is currently insufficient. For example, Li et alCitation144 reported that sugar-functionalized SWCNTs formed stable homogeneous aqueous solutions, Barzegar et alCitation145 reported that noncovalent functionalized SWCNTs could also improve the water solubility of SWCNTs, and Ali et alCitation146 functionalized SWCNTs with the TGAD enzyme to render SWCNTs soluble in a plethora of solvents. However, the biocompatibility of functionalized SWCNTs was not evaluated in these studies. When QDs are applied in the body, their toxicity cannot be ignored, because they may contain heavy metals. It is necessary to carry out a detailed toxicity study to ensure safety prior to further applications in humans. Further research will not be conducive to the clinical application of NMs and industrial production unless the toxicity problem is resolved. In addition, only a few types of materials have been approved by the US Food and Drug Administration, and very few NMs have been approved as antitumor agents to enter Phase III clinical trials or enter the market, which indicates that NMs need to be understood more deeply before their potential application in tumor therapy.
Therefore, the long-term toxicity of NMs to living systems needs to be intensively studied. There is an article that analyzes the possible toxicological implications of NMs in nanomedicine. The authors believed that it is necessary to research the mechanisms of injury in cells due to NMs extensively before NMs can be used in tumor treatments.Citation147 Development of tumor therapies is a multidisciplinary field, and with more in-depth research on tumor biology, tumor immunology, molecular biology, and NMs, an ideal therapy or NM will eventually be produced for the treatment of tumors. The conversion of nanotechnology to routine clinical practice will require a multidisciplinary approach guided by clinical, ethical, and social perceptions. In view of the significant research results being dedicated to the field, it may be expected that humans will greatly benefit from nanotechnology and NMs in the very near future, especially in tumor therapy.
Acknowledgments
This study was supported by the Chongqing Science & Technology Commission of China (cstc2016shmszx0915).
Disclosure
The authors report no conflicts of interest in this work.
References
- StevenASeligerBControl of CREB expression in tumors: from molecular mechanisms and signal transduction pathways to therapeutic targetOncotarget2016723354543546526934558
- SarkarFHBanerjeeSLiYWPancreatic cancer: pathogenesis, prevention and treatmentToxicol Appl Pharm20072243326336
- TangHDQiaoJFuYXImmunotherapy and tumor microenvironmentCancer Lett20163701859026477683
- LiBLiTWPignonJCLandscape of tumor-infiltrating T cell repertoire of human cancersNat Genet201648772573227240091
- LuoJFLuoYXSunJHZhouYRZhangYJYangXAdeno-associated virus-mediated cancer gene therapy: current statusCancer Lett2015356234735625444906
- KimSUNeural stem cell-based gene therapy for brain tumorsStem Cell Rev Rep201171130140
- ChenLMSunJHYangXMRadiofrequency ablation-combined multimodel therapies for hepatocellular carcinoma: current statusCancer Lett20163701788426472630
- IwahashiSShimadaMUtsunomiyaTEpithelial-mesenchymal transition-related genes are linked to aggressive local recurrence of hepatocellular carcinoma after radiofrequency ablationCancer Lett20163751475026940140
- GannonCJPatraCRBhattacharyaRMukherjeePCurleySAIntracellular gold nanoparticles enhance non-invasive radiofrequency thermal destruction of human gastrointestinal cancer cellsJ Nanobiotechnology20086218234109
- LauberKBrixNErnstATargeting the heat shock response in combination with radiotherapy: sensitizing cancer cells to irradiation-induced cell death and heating up their immunogenicityCancer Lett2015368220922925754814
- FavarettAPaselloGLoreggianLPreoperative concomitant chemo-radiotherapy in superior sulcus tumour: a mono-institutional experienceLung Cancer201068222823319632000
- JhaveriNAgasseFArmstrongDA novel drug conjugate, NEO212, targeting proneural and mesenchymal subtypes of patient-derived glioma cancer stem cellsCancer Lett2016371224025026683773
- BernardesSSde Souza-NetoFPRamalhoLNSystemic oxidative profile after tumor removal and the tumor microenvironment in melanoma patientsCancer Lett2015361122623225772650
- WenkCHPonceFGuillermetSNear-infrared optical guided surgery of highly infiltrative fibrosarcomas in cats using an anti-αvβ3 integrin molecular probeCancer Lett2013334218819523200675
- KarevaIWaxmanDJKlementGLMetronomic chemotherapy: an attractive alternative to maximum tolerated dose therapy that can activate anti-tumor immunity and minimize therapeutic resistanceCancer Lett2015358210010625541061
- XinYHuangQTangJQNanoscale drug delivery for targeted chemotherapyCancer Lett20163791243127235607
- BlancoEHsiaoAMannAPLandryMGMeric-BernstamFFerrariMNanomedicine in cancer therapy: innovative trends and prospectsCancer Sci201110271247125221447010
- D’AntonioCMilanoARighiniRPharmacogenomics in lung cancer chemotherapy: a review of what the oncologist should knowAnticancer Res201434105241525025275016
- ShabaruddinFHChenLCElliottRAPayneKA systematic review of utility values for chemotherapy-related adverse eventsPharmacoeconomics201331427728823529208
- BruheimKGurenMGSkovlundELate side effects and quality of life after radiotherapy for rectal cancerInt J Radiat Oncol201076410051011
- SalmenkyläSKouriMOsterlundPDoes preoperative radiotherapy with postoperative chemotherapy increase acute side-effects and postoperative complications of total mesorectal excision? Report of the randomized Finnish Rectal Cancer trialScand J Surg2012101427528223238504
- WalkerBJrMoutonCPNanotechnology and nanomedicine: a primerJ Natl Med Assoc200698121985198817225846
- GrudzienPLoSAlbainKSInhibition of notch signaling reduces the stem-like population of breast cancer cells and prevents mammosphere formationAnticancer Res201030103853386721036696
- MedinaCSantos-MartinezMJRadomskiACorriganOIRadomskiMWNanoparticles: pharmacological and toxicological significanceBr J Pharmacol2007150555255817245366
- MoghimiSMHunterACMurrayJCNanomedicine: current status and future prospectsFASEB J200519331133015746175
- YuXHRongJZhanZLLiuZLiuJXEffects of grain size and thermodynamic energy on the lattice parameters of metallic nanomaterialsMater Des201583159163
- KobayashiHOgawaMKosakaNChoykePLUranoYMulticolor imaging of lymphatic function with two nanomaterials: quantum dot-labeled cancer cells and dendrimer-based optical agentsNanomedicine (Lond)20094441141919505244
- YoonTJKimJSKimBGYuKNChoMHLeeJKMultifunctional nanoparticles possessing a “magnetic motor effect” for drug or gene deliveryAngew Chem Int Ed Engl20054471068107115635729
- TangFLiLChenDMesoporous silica nanoparticles: synthesis, biocompatibility and drug deliveryAdv Mater201224121504153422378538
- ManolovaVFlaceABauerMSchwarzKSaudanPBachmannMFNanoparticles target distinct dendritic cell populations according to their sizeEur J Immunol20083851404141318389478
- IbanezILNotcovichCCatalanoPNBellinoMGDuranHThe redox-active nanomaterial toolbox for cancer therapyCancer Lett2015359191925597786
- LiuLLvHYTengZYWangCYWangGXGlucose sensors based on core-shell magnetic nanomaterials and their application in diabetes management: a reviewCurr Pharm Des201521375359536826377660
- LvXNWangPBaiRInhibitory effect of silver nanomaterials on transmissible virus-induced host cell infectionsBiomaterials201435134195420324524838
- FischerHCChanWCNanotoxicity: the growing need for in vivo studyCurr Opin Biotech200718656557118160274
- AhsanFLRivasIPKhanMASuarezAITargeting to macrophages: role of physicochemical properties of particulate carriers-liposomes and microspheres-on the phagocytosis by macrophagesJ Control Release2002791–3294011853916
- ChithraniBDGhazaniAAChanWCDetermining the size and shape dependence of gold nanoparticle uptake into mammalian cellsNano Lett20066466266816608261
- ChampionJAKatareYKMitragotriSParticle shape: a new design parameter for micro- and nanoscale drug delivery carriersJ Control Release20071211–23917544538
- KaewsanehaCTangboriboonratPPolpanichDEissaMElaissariAJanus colloidal particles: preparation, properties, and biomedical applicationsACS Appl Mater Interfaces2013561857186923394306
- HuangYQiAHanBHExtraction of rutin and rhoifolin by inorganic borate functionalized magnetic particlesChin J Chem2016348823829
- ChoKWangXNieSChenZShinDMTherapeutic nanoparticles for drug delivery in cancerClin Cancer Res20081451310131618316549
- De JongWHBormPJDrug delivery and nanoparticles: applications and hazardsInt J Nanomedicine20083213314918686775
- HartmanKBWilsonLJRosenblumMGDetecting and treating cancer with nanotechnologyMol Diagn Ther200812111418288878
- CaiWHsuARLiZBChenXAre quantum dots ready for in vivo imaging in human subjects?Nanoscale Res Lett20072626528121394238
- CaiWChenXNanoplatforms for targeted molecular imaging in living subjectsSmall20073111840185417943716
- MalmoJSandvigAVårumKMStrandSPNanoparticle mediated P-glycoprotein silencing for improved drug delivery across the blood-brain barrier: a siRNA-chitosan approachPLoS One201381e5418223372682
- MitchellLALauerFTBurchielSWMcDonaldJDMechanisms for how inhaled multiwalled carbon nanotubes suppress systemic immune function in miceNat Nanotechnol20094745145619581899
- TkachAVShurinGVShurinMRDirect effects of carbon nanotubes on dendritic cells induce immune suppression upon pulmonary exposureACS Nano2011575755576221657201
- MitchellLAGaoJWalRVGigliottiABurchielSWMcDonaldJDPulmonary and systemic immune response to inhaled multiwalled carbon nanotubesToxicol Sci2007100120321417660506
- LiangHFChenCTChenSCPaclitaxel-loaded poly(γ-glutamic acid)-poly(lactide) nanoparticles as a targeted drug delivery system for the treatment of liver cancerBiomaterials20062792051205916307794
- ZhuLHuoZWangLTongXXiaoYNiKTargeted delivery of methotrexate to skeletal muscular tissue by thermosensitive magnetoliposomesInt J Pharm20093701–213614319114095
- NiidomeTYamagataMOkamotoYPEG-modified gold nanorods with a stealth character for in vivo applicationsJ Control Release2006114334334716876898
- ShenSKongFFGuoXMCMCTS stabilized Fe3O4 particles with extremely low toxicity as highly efficient near-infrared photothermal agents for in vivo tumor ablationNanoscale20135178056806623873020
- SubbiahRVeerapandianMYunKSNanoparticles: functionalization and multifunctional applications in biomedical sciencesCurr Med Chem201017364559457721062250
- BronsteinLMHuangXLRetrumJInfluence of iron oleate complex structure on iron oxide nanoparticle formationChem Mater2007191536243632
- Schulz-DobrickMSarathyKVJansenMSurfactant-free synthesis and functionalization of gold nanoparticlesJ Am Chem Soc200512737128161281716159272
- ChenJYWangDLXiJFImmuno gold nanocages with tailored optical properties for targeted photothermal destruction of cancer cellsNano Lett2007751318132217430005
- LeeSSZhuHContrerasEQPrakashAPuppalaHLColvinVLHigh temperature decomposition of cerium precursors to form ceria nanocrystal libraries for biological applicationsChem Mater2012243424432
- HirschLRStaffordRJBanksonJANanoshell-mediated near-infrared thermal therapy of tumors under magnetic resonance guidanceProc Natl Acad Sci U S A200310023135491355414597719
- MatsumineAKusuzakiKMatsubaraTNovel hyperthermia for metastatic bone tumors with magnetic materials by generating an alternating electromagnetic fieldClin Exp Metastasis200724319120017487560
- CaleroMChiappiMLazaro-CarrilloACharacterization of interaction of magnetic nanoparticles with breast cancer cellsJ Nanobiotechnology2015131525890025
- WuangSCNeohKGKangETPackDWLeckbandDEHER-2-mediated endocytosis of magnetic nanospheres and the implications in cell targeting and particle magnetizationBiomaterials200829142270227918289668
- WahajuddinAroraSSuperparamagnetic iron oxide nanoparticles: magnetic nanoplatforms as drug carriersInt J Nanomedicine201273445347122848170
- YangCYTaiMFLinCPMechanism of cellular uptake and impact of ferucarbotran on macrophage physiologyPLoS One201169e2552421991395
- LiuGGaoJAiHChenXApplications and potential toxicity of magnetic iron oxide nanoparticlesSmall201399–101533154523019129
- TangTYMullerKHGravesMJIron oxide particles for atheroma imagingArterioscler Throm Vasc Biol200929710011008
- EckWCraigGSigdelAPEGylated gold nanoparticles conjugated to monoclonal F19 antibodies as targeted labeling agents for human pancreatic carcinoma tissueACS Nano20082112263227219206392
- Wilton-ElyJDThe surface functionalisation of gold nanoparticles with metal complexesDalton Trans20081252918399221
- ZhangBChenJDZhuHFacile and green fabrication of size-controlled AuNPs/CNFs hybrids for the highly sensitive simultaneous detection of heavy metal ionsElectrochim Acta2016196422430
- BrownSDNativoPSmithJAGold nanoparticles for the improved anticancer drug delivery of the active component of oxaliplatinJ Am Chem Soc2010132134678468420225865
- LlevotAAstrucDApplications of vectorized gold nanoparticles to the diagnosis and therapy of cancerChem Soc Rev201241124225721785769
- WangJJZengZWXiaoRZRecent advances of chitosan nanoparticles as drug carriersInt J Nanomedicine2011676577421589644
- JokerstJVColeAJVan de SompelDGambhirSSGold nanorods for ovarian cancer detection with photoacoustic imaging and resection guidance via Raman imaging in living miceACS Nano2012611103661037723101432
- WangJJZengZWXiaoRZRecent advances of chitosan nanoparticles as drug carriersInt J Nanomedicine2011676577421589644
- VermaAUzunOHuYSurface-structure-regulated cell-membrane penetration by monolayer-protected nanoparticlesNat Mater20087758859518500347
- LarouiHRakhyaPXiaoBViennoisEMerlinDNanotechnology in diagnostics and therapeutics for gastrointestinal disordersDig Liver Dis20134512995100223660079
- FortinaPKrickaLJGravesDJApplications of nanoparticles to diagnostics and therapeutics in colorectal cancerTrends Biotechnol200725414515217316852
- SumbayevVVYasinskaIMGarciaCPGold nanoparticles downregulate interleukin-1-induced pro-inflammatory responsesSmall20139347247723112137
- ChenHDorriganASaadSHareDJCortieMBValenzuelaSMIn vivo study of spherical gold nanoparticles: inflammatory effects and distribution in micePLoS One201382e5820823469154
- WalkeyCDasSSealSCatalytic properties and biomedical applications of cerium oxide nanoparticlesEnviron Sci Nano201521335326207185
- PešićMPodolski-RenićAStojkovićSAnti-cancer effects of cerium oxide nanoparticles and its intracellular redox activityChem Biol Interact2015232859325813935
- XiaoYFLiJMWangSMCerium oxide nanoparticles inhibit the migration and proliferation of gastric cancer by increasing DHX15 expressionInt J Nanomedicine2016113023303427486320
- AkermanMEChanWCLaakkonenPBhatiaSNRuoslahtiENanocrystal targeting in vivoProc Natl Acad Sci U S A20029920126171262112235356
- KimKYNanotechnology platforms and physiological challenges for cancer therapeuticsNanomedicine20073210311017442621
- BallouBLagerholmBCErnstLABruchezMPWaggonerASNoninvasive imaging of quantum dots in miceBioconjug Chem2004151798614733586
- MorrowKJJrBawaRWeiCRecent advances in basic and clinical nanomedicineMed Clin North Am200791580584317826104
- JuzenasPChenWSunYPQuantum dots and nanoparticles for photodynamic and radiation therapies of cancerAdv Drug Deliv Rev200860151600161418840487
- AshcroftJMTsyboulskiDAHartmanKBFullerene (C60) immunoconjugates: interaction of water-soluble C60 derivatives with the murine anti-gp240 melanoma antibodyChem Commun20062830043006
- PratoMKostarelosKBiancoAFunctionalized carbon nanotubes in drug design and discoveryAcc Chem Res2008411606817867649
- QiaoRRobertsAPMountASKlaineSJKePCTranslocation of C60 and its derivatives across a lipid bilayerNano Lett20077361461917316055
- SatohMTakayanagiIPharmacological studies on fullerene (C60), a novel carbon allotrope, and its derivativesJ Pharmacol Sci2006100551351816682790
- HiraharaKSuenagaKBandowSOne-dimensional metallofullerene crystal generated inside single-walled carbon nanotubesPhys Rev Lett200085255384538711136002
- LiangXJMengHWangYMetallofullerene nanoparticles circumvent tumor resistance to cisplatin by reactivating endocytosisProc Natl Acad Sci U S A2010107167449745420368438
- ShvedovaAAKisinERPorterDMechanisms of pulmonary toxicity and medical applications of carbon nanotubes: two faces of Janus?Pharmacol Ther2009121219220419103221
- TerronesMTerronesHThe carbon nanocosmos: novel materials for the twenty-first centuryPhilos Trans A Math Phys Eng Sci200336118132789280614667298
- LacerdaLBiancoAPratoMKostarelosKCarbon nanotubes as nanomedicines: from toxicology to pharmacologyAdv Drug Deliv Rev200658141460147017113677
- KarmakarAIancuCBartosDMRaman spectroscopy as a detection and analysis tool for in vitro specific targeting of pancreatic cancer cells by EGF-conjugated, single-walled carbon nanotubesJ Appl Toxicol201232536537522147491
- MahmoodMKarmakarAFejlehASynergistic enhancement of cancer therapy using a combination of carbon nanotubes and anti-tumor drugNanomedicine (Lond)20094888389319958225
- ShenHAckermanALCodyVEnhanced and prolonged cross-presentation following endosomal escape of exogenous antigens encapsulated in biodegradable nanoparticlesImmunology20061171788816423043
- HeathJRDavisMENanotechnology and cancerAnnu Rev Med20085925126517937588
- O’NealDPHirschLRHalasNJPayneJDWestJLPhoto-thermal tumor ablation in mice using near infrared-absorbing nanoparticlesCancer Lett2004209217117615159019
- MocanLIlieITabaranFASurface plasmon resonance-induced photoactivation of gold nanoparticles as mitochondria-targeted therapeutic agents for pancreatic cancerExpert Opin Ther Targets201317121383139324188208
- MocanLTabaranFAMocanTSelective ex-vivo photothermal ablation of human pancreatic cancer with albumin functionalized multiwalled carbon nanotubesInt J Nanomedicine2011691592821720504
- MocanTMateaCTCojocaruIPhotothermal treatment of human pancreatic cancer using PEGylated multi-walled carbon nanotubes induces apoptosis by triggering mitochondrial membrane depolarization mechanismJ Cancer20145867968825258649
- KamNWO’ConnellMWisdomJADaiHJCarbon nanotubes as multifunctional biological transporters and near-infrared agents for selective cancer cell destructionProc Natl Acad Sci U S A200510233116001160516087878
- ShaoNLuSWickstromEPanchapakesanBIntegrated molecular targeting of IGF1R and HER2 surface receptors and destruction of breast cancer cells using single wall carbon nanotubesNanotechnology20071831315101
- BirisASBoldorDPalmerJNanophotothermolysis of multiple scattered cancer cells with carbon nanotubes guided by time-resolved infrared thermal imagingJ Biomed Opt200914202100719405720
- BurlakaALukinSPrylutskaSHyperthermic effect of multi-walled carbon nanotubes stimulated with near infrared irradiation for anticancer therapy: in vitro studiesExp Oncol2010321485020332757
- NovoselovKSGeimAKMorozovSVElectric field effect in atomically thin carbon filmsScience2004306569666666915499015
- RaoCNSoodAKSubrahmanyamKSGovindarajAGraphene: the new two-dimensional nanomaterialAngew Chem Int Ed Engl200948427752777719784976
- AllenMJTungVCKanerRBHoneycomb carbon: a review of grapheneChem Rev2010110113214519610631
- YangKFengLZShiXZLiuZNano-graphene in biomedicine: theranostic applicationsChem Soc Rev201342253054723059655
- YangKHuLLMaXXMultimodal imaging guided photothermal therapy using functionalized graphene nanosheets anchored with magnetic nanoparticlesAdv Mater201224141868187222378564
- YangKZhangSAZhangGXSunXMLeeSTLiuZAGraphene in mice: ultrahigh in vivo tumor uptake and efficient photothermal therapyNano Lett20101093318332320684528
- YangKWanJMZhangSTianBZhangYJLiuZThe influence of surface chemistry and size of nanoscale graphene oxide on photothermal therapy of cancer using ultra-low laser powerBiomaterials20123372206221422169821
- ZedanAFMoussaSTernerJAtkinsonGEl-ShallMSUltrasmall gold nanoparticles anchored to graphene and enhanced photothermal effects by laser irradiation of gold nanostructures in graphene oxide solutionsACS Nano20137162763623194145
- DembereldorjUChoiSYGanboldEOGold nanorod-assembled PEGylated graphene-oxide nanocomposites for photothermal cancer therapyPhotochem Photobiol201490365966624303894
- ShiJJWangLZhangJA tumor-targeting near-infrared laser-triggered drug delivery system based on GO@Ag nanoparticles for chemo-photothermal therapy and X-ray imagingBiomaterials201435225847586124746963
- BaiJLiuYWJiangXEMultifunctional PEG-GO/CuS nanocomposites for near-infrared chemo-photothermal therapyBiomaterials201435225805581324767788
- HouCYQuanHCDuanYRZhangQHWangHZLiYGFacile synthesis of water-dispersible Cu2O nanocrystal-reduced graphene oxide hybrid as a promising cancer therapeutic agentNanoscale2013531227123223302950
- FriedhuberAMChandoluVManchunSDonkorOSriamornsakPDassCRNucleotropic doxorubicin nanoparticles decrease cancer cell viability, destroy mitochondria, induce autophagy and enhance tumour necrosisJ Pharm Pharmacol2015671687725208603
- ShiSFJiaJFGuoXKBiocompatibility of chitosan-coated iron oxide nanoparticles with osteoblast cellsInt J Nanomedicine201275593560223118539
- UpadhyayaLSinghJAgarwalVTewariRPBiomedical applications of carboxymethyl chitosansCarbohydr Polym201391145246623044156
- BodnarMHartmannJFBorbelyJPreparation and characterization of chitosan-based nanoparticlesBiomacromolecules2005652521252716153088
- GoldbergMLangerRJiaXNanostructured materials for applications in drug delivery and tissue engineeringJ Biomater Sci Polym Ed200718324126817471764
- AmidiMMastrobattistaEJiskootWHenninkWEChitosan-based delivery systems for protein therapeutics and antigensAdv Drug Deliv Rev2010621598219925837
- BawarskiWEChidlowskyEBharaliDJMousaSAEmerging nanopharmaceuticalsNanomedicine20084427328218640076
- SeferianPGMartinezMLImmune stimulating activity of two new chitosan containing adjuvant formulationsVaccine200019666166811090719
- ShibataYFosterLAMetzgerWJMyrvikQNAlveolar macrophage priming by intravenous administration of chitin particles, polymers of n-acetyl-d-glucosamine, in miceInfect Immun1997655173417419125555
- CalvoPRemuñan-LópezCVilaJatoJLAlonsoMJChitosan and chitosan ethylene oxide propylene oxide block copolymer nanoparticles as novel carriers for proteins and vaccinesPharm Res19971410143114369358557
- JeongYIJinSGKimIYDoxorubicin-incorporated nanoparticles composed of poly(ethylene glycol)-grafted carboxymethyl chitosan and antitumor activity against glioma cells in vitroColloids Surf B Biointerfaces201079114915520427160
- KostarelosKBiancoAPratoMPromises, facts and challenges for carbon nanotubes in imaging and therapeuticsNat Nanotechnol200941062763319809452
- ZhangWXKarnBNanoscale environmental science and technology: challenges and opportunitiesEnviron Sci Technol200539594A95A
- LanoneSBoczkowskiJBiomedical applications and potential health risks of nanomaterials: molecular mechanismsCurr Mol Med20066665166317022735
- HoshinoAHanadaSYamamotoKToxicity of nanocrystal quantum dots: the relevance of surface modificationsArch Toxicol201185770772021445587
- ProffittFYellow light for nanotechScience20043055685762765
- LamCWJamesJTMcCluskeyRHunterRLPulmonary toxicity of single-wall carbon nanotubes in mice 7 and 90 days after intratracheal instillationToxicol Sci200477112613414514958
- ServiceRFNanotechnology grows upScience200430456781732173415205504
- LamCWJamesJTMcCluskeyRArepalliSHunterRLA review of carbon nanotube toxicity and assessment of potential occupational and environmental health risksCrit Rev Toxicol200636318921716686422
- NelAXiaTMadlerLLiNToxic potential of materials at the nanolevelScience2006311576162262716456071
- MannaSKSarkarSBarrJSingle-walled carbon nanotube induces oxidative stress and activates nuclear transcription factor-κB in human keratinocytesNano Lett2005591676168416159204
- RavichandranPBaluchamySSadanandanBMultiwalled carbon nanotubes activate NF-κB and AP-1 signaling pathways to induce apoptosis in rat lung epithelial cellsApoptosis201015121507151620694747
- CuiDXTianFROzkanCSWangMGaoHJEffect of single wall carbon nanotubes on human HEK293 cellsToxicol Lett20051551738515585362
- RenwickLCBrownDClouterADonaldsonKIncreased inflammation and altered macrophage chemotactic responses caused by two ultrafine particle typesOccup Environ Med200461544244715090666
- LiHChenQHanBHSugar-functionalized triptycenes used for dispersion of single-walled carbon nanotubes in aqueous solution by supramolecular interactionNew J Chem201640433003307
- BarzegarAMansouriAAzamatJMolecular dynamics simulation of non-covalent single-walled carbon nanotube functionalization with surfactant peptidesJ Mol Graph Model201664758426811869
- AliKMahmoudWEDevelopment of functionalized single walled carbon nanotubes based triacylglycerol acylhydrolasesSynth Met20131755255
- FirmeCP3rdBandaruPRToxicity issues in the application of carbon nanotubes to biological systemsNanomedicine20106224525619699321