Abstract
After administration of nanoparticle (NP) into biological fluids, an NP–protein complex is formed, which represents the “true identity” of NP in our body. Hence, protein–NP interaction should be carefully investigated to predict and control the fate of NPs or drug-loaded NPs, including systemic circulation, biodistribution, and bioavailability. In this review, we mainly focus on the formation of protein corona and its potential applications in pharmaceutical sciences such as prediction modeling based on NP-adsorbed proteins, usage of active proteins for modifying NP to achieve toxicity reduction, circulation time enhancement, and targeting effect. Validated correlative models for NP biological responses mainly based on protein corona fingerprints of NPs are more highly accurate than the models solely set up from NP properties. Based on these models, effectiveness as well as the toxicity of NPs can be predicted without in vivo tests, while novel cell receptors could be identified from prominent proteins which play important key roles in the models. The ungoverned protein adsorption onto NPs may have generally negative effects such as rapid clearance from the bloodstream, hindrance of targeting capacity, and induction of toxicity. In contrast, controlling protein adsorption by modifying NPs with diverse functional proteins or tailoring appropriate NPs which favor selective endogenous peptides and proteins will bring promising therapeutic benefits in drug delivery and targeted cancer treatment.
Introduction
After systemic administration, nanomaterials are exposed to various physiological fluids, mostly blood. There are several thousand proteins in blood at up to a billion time differences in relative concentrations of protein corona.Citation1 The adsorption of proteins on nanpoparticles (NPs) can modify the diverse physicochemical properties of NPs such as size, surface charge, surface composition, and functionality, hence giving NPs a new biological identity. This NP–protein complex, not bare NP, determines various biological responses such as fibrillation, cellular uptake, circulation time, bioavailability, and even toxicity. The layers consisting of bound or adsorbed proteins around NPs are called as protein corona. High surface energy may enhance the binding of protein on to NP surface.
shows the formation of protein corona and exchange of adsorbed proteins over time in biological conditions. Protein corona patterns mainly depend on the physicochemical properties of NPs (nanomaterial, size, charge, surface functional groups, shape) and exposing environments including immersed media components, temperature, pH, dynamic shear stress, and interaction (or exposing) time. Proteins with large quantities are first bound to NP surface, and then gradually replaced by higher affinity proteins (Vroman effect).Citation2 The detailed information of protein corona is described in detail in the following section.
Figure 1 The formation of protein corona and exchange of adsorbed proteins over time in biological conditions.
Notes: (A) Immediately upon exposure. (B) After a longer exposure time, with displacement of proteins among the hard corona, soft corona, and cellular environment. (C) Major factors affecting protein corona pattern divided into two categorized properties: nanoparticle and environment.
Abbreviation: NP, nanoparticle.
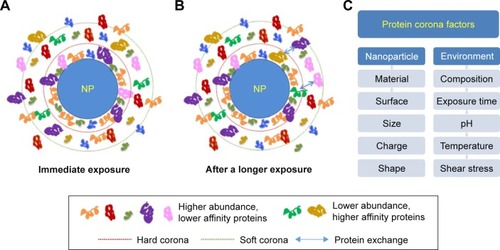
Protein corona pattern
The differences in NP properties and the environments influence protein corona by interacting with diverse protein compositions via adsorption.Citation3 Additionally, protein corona is complex and unique to each nanomaterial and NP. Furthermore, the composition and relative quantity of adsorbed proteins may also not correspond with protein components and relative concentrations in exposure media. In other words, the adsorption of proteins onto NPs is controlled by the protein–NP interaction and protein–protein interaction. shows the diversity of the top ten proteins adsorbed to silica NPs and the most abundant proteins in human plasma. Albumin is the most abundant protein in blood and is often found in protein corona fingerprints of various NPs. In addition, other common proteins to form adsorbed protein patterns include complement proteins, fibrinogen, and immunoglobulins, which significantly alter NP opsonization, allocation, and targeting efficiency.Citation4,Citation5 Protein pattern refers to some important and recognizable sequence features such as binding sites or the active sites of protein and enzymes because they have only a few amino acids that are essential for protein function.
Table 1 Diversity of top ten proteins adsorbed to silica NPs and the most abundant proteins in human plasma
Protein corona is divided into “hard corona” and “soft corona”. Hard corona contains higher affinity proteins on the NP surface that may irreversibly bind to NPs. In contrast, soft corona formed by lower affinity proteins is reversibly bound to NPs, which can be further exchangeable over time or easily detached by washing steps during experiments. Protein corona is a dynamic layer in which the arrangement and amount of protein are changeable according to the conditions of physicochemical and biological interaction.
Protein conformation
In general, protein conformation refers to the characteristic dimensional shapes or structures of a reversible protein formed by arrangement of amino acid molecules, including the secondary, super-secondary (motifs), tertiary (domains), and quaternary structure of the peptide chain. Reversible structural changes or alternative structures of the same protein are referred to as conformational isomers, or simply, conformations or conformational changes. Thus, protein functions depend on their secondary and tertiary structures. Hence, the change in protein structure can affect their pharmacological functions and activities due to the dynamic changes of exposing conditions. When the protein is bound to NPs to form protein corona, proteins may rearrange their structures to adapt to NPs surface and surrounding environments. This event is known as “conformational changes”, in which the secondary and/or tertiary protein structure is modified. Proteins are required to interact with other biomolecules and substances to activate or express their biological functions. Therefore, a small modification in protein structure caused by mutual interaction with NPs may induce an enormous impact on their pharmacological activities, association with other proteins, and also other biological responses.Citation6
The correlation between NP size and protein size is an important factor for determining protein structures. In case NPs are bigger than proteins, proteins may stretch to adapt to the NP surface. Smaller NPs decrease the interaction with proteins, causing less structural changes.Citation7
The secondary structure of proteins is strongly affected by the surface charge of NPs. For example, gold NPs with identical properties, but different surface charges, showed similar adsorbed bovine serum albumin (BSA) amounts. However, the NPs having positive charges expressed much higher cell association and a faster cellular uptake rate than the negatively charged counterparts. This result suggested the internal structure modification of BSA might be caused by the NP charge.Citation8 Indeed, adsorbed BSA onto negatively charged polystyrene NPs retains it native structure compared with the conformational change of BSA with positively charged NPs, which leads to the redirection of the protein–NP complex to scavenger receptors. The adsorbed BSA on anionic polystyrene NPs could inhibit cellular binding, but its counterpart enhanced the cellular binding of NPs and, unexpectedly, also toxicity.Citation9 Consistently, other NPs carrying a negative charge, such as quantum dots decorated with carboxylate groups or gold NPs stabilized by citrate, inhibited cellular binding after incubation with serum proteins or BSA alone although the size, material, and surface modifications of NPs were diverse.Citation10
Instrumental analysis for evaluating protein corona
The protein–NP interaction is studied by incubating NPs in protein solutions for a period of time, either a single protein solution such as BSA, fibronectin and IgG,Citation11–Citation14 or biological fluid containing multiple proteins such as serum,Citation15,Citation16 plasma, cytosol,Citation17 fetal bovine serum (FBS),Citation18 fetal calf serum, synovial fluid, and cerebrospinal fluid.Citation19 Protein–NP complexes are then separated from the protein solution via instrumental analysis. The protein corona is widely characterized by total quantity, density, thickness, composition, relative abundance of each protein, protein binding affinity, and protein conformation.
The interaction between protein and NPs can be simply confirmed by the physical changes of NPs in sizeCitation18 and zeta potential.Citation20 Common techniques to evaluate NP size after protein adsorption include dynamic light scattering (DLS), transmission electron microscopy (TEM), and sometimes, atomic force microscopy (AFM). DLS is conventional and fast, but may be influenced by NP agglomeration, so that in several cases data collected by DLS and TEM are different.Citation21 More advantageously, TEM and AFM give more clear information on single NP size, shape, and the protein corona covering the NP surface. The protein corona can be further characterized by UV-Vis absorption spectra. For example, Au NPs incubated with Dulbecco’s Modified Eagle’s Medium (DMEM) exhibited a slight red shift and higher absorption intensity over time in UV-Vis spectra than those that interacted with Roswell Park Memorial Institute (RPMI) medium. This suggests that a larger quantity of proteins were adsorbed on Au NPs when incubated with DMEM than RPMI.Citation18
Centrifugation is the typical method to collect NP–protein complex from the interaction media and loosely adsorbed proteins, and sucrose cushion centrifugation allows the separation of complex in a short time (30 sec).Citation11,Citation12,Citation22,Citation23 The secondary structure of proteins can be evaluated by circular dichroism. However, this method cannot be used for a mixture of proteins due to its spectral complexity. Isothermal titration calorimetry (ITC) is another common method used to evaluate the molecular interaction between proteins and NPs.Citation9,Citation21,Citation24–Citation26 The positive points of the ITC method are that it directly characterizes and measures nanoparticle–protein interactions, such as total binding proteins and protein binding affinity, without using separation and isolation processes.Citation26
After separation of protein–NP complexes from incubation media or, isolation of protein from NP with further steps, electrophoresis was often applied for identification and qualitative determination of protein corona composition.Citation19,Citation24,Citation25,Citation27 In addition, gel filtration can be used to not only separate protein–NP complexes from incubation media,Citation28,Citation29 but also to isolate protein from NP surfaces as well as provide information of kinetic exchange rate for adsorbed proteins.Citation30 A positive point about gel filtration is that it can both separate and isolate adsorbed proteins from easily deformed and low-density NPs such as liposomes and exosomes. Protein identification and quantity determination can be characterized by mass spectrometry, in which protein samples have been digested into small peptides and simply injected into analysis instrument.Citation23,Citation31 The protein corona results will be determined by comparison with standard protein data.
Protein corona evaluation may be obtained differently, depending on the types of applied analytical characterization. For example, human serum albumin (HSA) adsorbed on hydroxyethyl starch nanocapsules could be detected by ITC, while there was no trace of HSA when evaluated by SDS-PAGE.Citation32 Hence, it is pivotal to choose suitable analytical techniques to accurately characterize protein corona properties since protein corona is related with physicochemical properties and biological identity of NPs.
Effect of exposure conditions on protein corona
Media composition
Media with different protein components strongly affect protein corona properties. Interestingly, after only 1 h incubated in serum-free medium, the pristine NPs get coated by proteins located in either cell membrane or cytosol. Serum proteins adsorbed to silica NP surface showed reduced interaction with cells, resulting in lower cellular uptake of silica NPs in the media supplemented with serum compared with media with no serum addition.Citation33 It was known that protein corona reduced the NP cell adhesion and cellular uptake.Citation34 In another study, the influence of biological fluid in cellular uptake pathway of NPs on the evolution of the protein corona was examined after incubating NPs with plasma followed by cytosolic fluid. The protein corona of NPs after exposure to the cytosolic fluid was significantly different, but protein pattern in hard corona was retained after plasma incubation,Citation17 which was in agreement with a previous study.Citation35 DMEM encouraged higher total protein quantity adsorbed onto gold NPs than RPMI after 48 h incubation, but similar amounts were observed at the 1-h incubation.Citation18
Protein concentrations
The protein corona motif is significantly affected by the protein concentration.Citation34 Silica NPs expressed different protein patterns when incubated with 3%, 20%, and 80% plasma, explaining the replacement of proteins present in small quantities but with a higher binding affinity with proteins with lower binding affinity and higher concentrations in human plasma.Citation27 Moreover, the higher human serum concentration in ranges of 0.5–50 wt/v%, gradually increase the relative abundance of Apo E compared to serum albumin and transferrin. It could be considered as the major proteins on the magnetic NP at 50% serum concentration.Citation36 In contrast, phagocytosis of NPs by dendritic cells was identical in various concentrations of protein mixtures. This result could be explained by particle uptake by phagocytes, possibly not dependent on the total binding protein quantity but the abundance of recognizable proteins on the particle surface.Citation37
Exposure time
Protein adsorption onto NPs is also controlled by the exposure time of NPs to the fluidic media. Protein corona is immediately formed when NPs come into contact with biological fluids. After only 30 sec interaction with human plasma, nearly 300 proteins binding to NPs surface are detected.Citation23 Longer duration of exposure time enhances the total protein quantity adsorbed.Citation18 Additionally, the exchange of adsorbed proteins over time significantly modifies the abundance of each binding protein, hence resulting in different protein corona patterns. This can be explained by the replacement of higher affinity proteins to lower counterparts or favorable adsorption of lower molecular weight protein compared to higher ones. However, the composition of protein corona remains the same.Citation18
Static and fluidic conditions
The difference in protein corona composition and quantity under static and flow conditions must be taken into account. Various previous studies on protein–NP interactions were often performed under static conditions,Citation38 while NPs are often administrated through intravenous injection and immediately interact with blood protein under various shear stresses in the hydrodynamic body conditions. It is known that blood flow velocity ranges from 0.03 cm/sec in capillaries to 15 cm/sec in superior and inferior vena cava and 40 cm/sec in the aorta.Citation39,Citation40 The protein corona of NPs under dynamic fluidic conditions is obviously different from the one constructed in static conditions. At 40 cm/sec, equal to abdominal aortic speed, the PEG-coated liposomes under dynamic fluidic conditions carried more negative charge compared to their static counterparts but their sizes were similar, suggesting the difference of binding protein components and each protein quantity. Additionally, the protein diversity in the protein corona under fluidic flow was considerably broadened. Therefore, it is essential to evaluate protein–NP interactions in a biomimetic environment which mimics in vivo physiological conditions to more accurately predict the fate of NPs in vivo.Citation22
Temperature
Temperature also alters protein–NP interaction and was studied in several previous works.Citation11,Citation41,Citation42 It was known that temperature caused significant changes in protein corona pattern of magnetic NPs in the human body temperature, ranging between 37°C and 41°C during exposure to various concentrations of HSA as well as apo-transferrin (apo-Tf).Citation42 Protein quantity adsorbed on Cu NPs increased when incubation temperature increased from 15°C, 27°C, and 37°C to 42°C.Citation41 Therefore, the health condition of the patients also needs to be considered, as fever or some diseases can change the body temperature which facilitates the NP interaction with protein, leading to changes in biodistribution and bio-availability of NPs.
pH of biological media
pH condition can be also considered as an important factor in the evaluation of the interaction of NPs with proteins. The change of environmental pH may alter protein binding affinity,Citation43 leading to modification of adsorbed protein pattern. In the cellular uptake pathway, normally nanomaterials undergo several different pH of biological fluids such as blood (neutral pH), exposure media (pH 6.9–7.4), intracellular fluid (pH 6.8), and lysosome (pH 4.5–5). Additionally, upon administration, NPs enter the systemic blood circulation and are biodistributed to different organs. Cancer or tumor always produce an acid microenvironment and contain specific types of proteins which may particularly modify the protein corona around NPs, resulting in the changes of bioavailability and therapeutic response. Therefore, it is essential to focus on the effect of biological pH on protein–NP interactions for precise evaluation of NP fate inside our body.
Effect of nanomaterial properties on protein corona formation
It is well known that different characteristics of NPs produce different protein corona profiles.Citation44 Hence, physicochemical and biological properties of NPs through NP–protein complexes can control cellular behavior, bioavailability, and biological responses. shows the effect of various NP parameters on the changes of protein corona. Generally, hydrophobic nanomaterial mostly has a higher quantity of adsorbed proteins than the hydrophilic one. Therefore, protein corona around hydrophobic NPs may cause agglomeration and higher opsonization, leading to shorter systemic circulation time in blood than hydrophilic NPs.Citation45 Even with lower amount of binding protein, hydrophilic nanomaterials adsorb proteins when exposed to the media that have commonly found proteins such as albumin, fibrinogen, and IgG.Citation15 NPs with different functional groups on their surfaces can also carry individual protein “fingerprints”.Citation23 Protein fingerprint refers to an analytical technique or any of the several methods for identifying or differentiating proteins. Peptide fragments can form via chemical and enzymatic treatments, followed by separation and identification in a reproducible manner using protein mass spectrometry following SDS-PAGE, two-dimensional chromatography, and/or protein electrophoresis.
Table 2 Effect of NP parameters on the changes of protein corona
Notably, differences in mechanical properties of nanomaterials also ensure significant changes in protein adsorption and biological responses of NPs. Both emulsion droplets and solid polystyrene NPs at the same size were evaluated for IgG adsorption behavior and phagocytic efficiency. The IgG distribution homogeneously around the polystyrene beads was independent with IgG density due to the prevention of IgG lateral mobility caused by solid nanomaterial. Oppositely, IgGs were concentrated in the interface between the emulsion droplets and the cell in case of low IgG densities, while at high IgG densities, there was no appearance of IgG clusters. This can be explained that emulsions allow surface for adsorbed proteins to diffuse and relocate at the interface. Hence, the emulsion formulations can reduce the required protein quantity, or further surface active moieties compared with solid NPs, while achieving the same effect. This suggestion might be valuable in the NP design for diverse pharmaceutical applications.Citation46
Protein corona applications
Physiological prediction via modeling
The biological interactions of an NP depend on its physicochemical properties as discussed previously. Some studies have focused on establishing correlations between NP characteristics and biological outcomes for predicting the biological behavior of NPs without explicit experimentation.Citation47 Due to the infinite number of NP designs with nature diversity and the complexity of biological systems, it is challenging to set up relationships between NP characteristics and biological responses. A scheme based on correlations between NP physicochemical features and cellular responses, such as cell morphology modifications, cell proliferation, viability and functionality, and reactive oxygen species (ROS) induction,Citation48 was suggested for optimizing NP properties to reduce cellular toxicity.Citation49,Citation50 Quantitative structure–activity relationship (QSAR) modeling to establish a statistically significant connection between biological activities of NPs and their physicochemical properties was investigated in several studies.Citation51–Citation53 The model prediction power for cellular uptake, which was established from 109 manufactured NPs with similar cores decorated by various functional groups, was shown to have a coefficient of determination (R2) value as high as 0.72, which means the model was validated and can be used to predict the cellular responses of various nanomaterials as well as promote the tailoring and fabrication of nanomaterials.Citation54
The first predictive model based on the protein corona was developed using gold NPs with different sizes and surface alterations. The relative model was built based only on the serum protein corona fingerprint of 129 adsorbed proteins to predict the cell associations of NPs. Applying a bioinformatics-inspired approach, this model reached a high R2 value of 0.81, which is 50% more precise than previous models that only consider NP physicochemical assessments. Physicochemical property data such as size, zeta potential, and absorbance spectra could not provide sufficient information to establish an acceptably accurate model prediction. However, a model based on the protein corona fingerprint and physicochemical properties of NPs gave a more accurate prediction of Au NP cell associations, with R2=0.86. Hence, the protein corona reflects more NP biological details than its physicochemical features.Citation31
In the above study, the best prediction model was formed based on 52 independent factors, including various Au NP-adsorbed proteins and numerous physicochemical properties. The latter study suggests a more facile way to establish linear and nonlinear predictive models, based mainly on representative adsorbed proteins and zeta potential of Au NPs with different sizes and surface modifications. The model building factors can be divided into two categories: NP physicochemical properties as synthesized, and with serum (hydrodynamic diameter, total adsorbed protein, binding protein density and so on) and protein corona fingerprints (the type and abundance of adsorbed protein). The highest R2 value of the models which were mainly built from the NP physicochemical properties was 0.695. This result showed that the physicochemical properties of NPs have no relationship to cellular responses. For either linear or nonlinear models, the higher the number of influencing factors, the more accurate the models are established. For example, in cases of non-linear models based on protein corona fingerprints, R2 was around 0.62 based on 3 factors, while it was more improved to 0.85 based on 9 factors. Additionally, the prediction accuracy of the nonlinear models was much higher relative to linear models when the same number of factors was used. The most precise model had R2=0.850, based on 11 different adsorbed proteins. More advantageously, the best nonlinear model was established with only 7 factors, including 6 adsorbed proteins and one NP zeta potential, showing higher accuracy value at R2=0.895.Citation55
Another study was also investigated the relationship between cellular interactions with human prostate cancer cells and HeLa cells of diverse liposomes and their physicochemical properties as well as protein corona characteristics. The predictive models were well established, considering cellular viability and uptake in two different cell lines, with R2 in the range of 0.79–0.94. The best variables selected in all correlation models included both physicochemical properties of liposome and descriptors of protein corona fingerprint.Citation56 A predictive-validation model of cell association of lipid NPs based on the specifically adsorbed proteins was developed. Interestingly, a small part of the hard corona proteins, consisting of only 8 adsorbed proteins (vitronectin, Apo A1, A2, B, and C2, and so on), was recognized to include the most important promoters of NP adhesion with HeLa cells among a massive number of possible descriptors (N=436 proteins). Remarkably, the identification of those importantly adsorbed proteins was found with several high-expression receptors of Hela cell membrane.Citation57
shows a scheme for the establishment of predictive model. From the model, biological responses can be predicted without further experiments and highly expressed receptors can be identified from the most influencing proteins for NP design. The abovementioned predictive studies indicate the methodological advantage for the model establishment in which the most important factors are screened first, followed by trying the highly accurate models with small numbers of influencing factors. Reducing the numbers of independent factors simplifies predictive model development, and makes their applicability more practical and economical. Moreover, from the validated model established, the proteins that play important functions correlated with biological responses are identified. Based on these proteins, targeting receptors that are highly expressed on the desired cells can be labeled and applied in NP design. Furthermore, the correlative models between cell association of NPs and both protein corona as well as physicochemical properties of NPs can provide highly accurate information and data for the NP design and make NPs nontoxic.
Modification of NP properties for drug delivery
The advantages of nanomaterial as drug delivery system are due to the nano sizability that allows NPs to have high drug loading efficiency and to escape from biological barrier for facilitate absorption and biodistribution. The ability of NPs to escape from the reticuloendothelial system, to increase half-life, to improve distribution time and accumulation in targeting tissue, and control their toxicity are determining factors for the design and therapeutic efficiency of NPs. To enhance NPs bioavailability and therapeutic response, NP size, nanomaterial, and NP surface are required to be considered for the design and fabrication of NPs. Furthermore, NP surfaces can be modified with diverse functional groups, either inorganic or organic, including proteins with specific activities.
Systemic circulation time of NPs
The adsorption of protein onto NPs in contact with biological fluid can alter the interactive interface between the NPs and cell membranes, from macrophages and endothelial cells to targeted cells. The binding protein pattern strongly influences phagocytosis and transport of NPs, hence the retention of NPs. If complement factors, fibrinogen, or IgG, called opsonins, are relatively high in protein corona, adsorbed proteins will induce macrophage recognition and engulfment of NPs, leading to the fast removal of NPs out of the body. In contrast, the circulation time is extended in case the protein corona is enriched with albumin or apolipoproteins (Apos), named dysopsonins.Citation37,Citation58–Citation60 Therefore, the design of NPs should be aimed to reduce the total adsorption of protein, specifically opsonins, and assist the binding of dysopsonins to prolong NP circulation time in the body as the first key factor for better in vivo performances of NPs.
To enhance the NP bioavailability and therapeutic response, NPs should be initially protected from recruitment of blood proteins. Previous studies used various ligands to pursue NP “stealth effect”, for example, peptides designed from human CD47, which prohibits clearance by phagocytes.Citation61 Another example is hydrophobin, a fungi secretory substance, which was used to coat NPs in order to reduce protein adsorption.Citation62
Another method is furnishing different types of NPs with hydrophilic polymers and surfactants to improve their systemic circulation properties and decrease macrophage recognition.Citation63,Citation64 Several well-known hydrophilic polymers include polyethylene glycol (PEG),Citation65 poloxamer,Citation25 polyvinylpyrrolidone (PVP), and dextran. The hydrophilic properties, the length, and charge from these polymers prevent the interaction of NPs with other biomolecules, including opsonins.Citation66,Citation67 Besides, the degree of surface coverage is also an important consideration in design and preparation of NPs to prevent protein adsorption.Citation68,Citation69 Among all polymers, PEG is the most commonly used polymer for NP surface modification. NPs surrounded by PEG increased blood circulation time by several orders of magnitude and reduced liver accumulation, depending on the coated PEG molecular weight and surface density.Citation70 However, repeated administration of PEG-coated NPs may cause the accelerated blood clearance (ABC) phenomenon as antibodies against PEG increase.Citation71
A new finding on the “stealth effect” mechanism by hydrophilic polymers has been elucidated. Previously, this effect is assumed to come from the hydrophilic nature of these polymers, which lowers the quantity of adsorbed proteins. However, PEG-modified NPs showed high and nonspecific cellular uptake when incubated in the media without plasma proteins. This result indicated that the presence of the binding proteins onto hydrophilic polymer layer is essential to reduce NPs internalization into cells.Citation72 NPs coated by PVP and PEG increase the systemic circulation time of NPs, compared to the bare ones. Nevertheless, PEG-coated NPs had longer circulations time than PVP-coated NPs. While protein corona of PEG-coated NPs had higher Apo quantities, opsonizing proteins were the major proteins binding to PVP-coated NPs. Therefore, the presence and pattern of binding protein layers strongly affect the “stealth effect” of hydrophilic polymers coated on NPs.Citation14 Moreover, “stealth effect” of polymers depends on their individual structure, which is able to induce the selective suppression of the adsorption of opsonins and the favorable binding of “dysopsonin”.Citation69,Citation72
Another important concept to increase systemic circulation time of NPs in blood is to mask NPs with dysopsonin proteins. By simple incubation, albumin could form a pure protein corona around poly (3-hydroxybutyrate-co-3-hy-droxyhexanoate) (PHBHHx) NPs. The albumin corona inhibited plasma protein adsorption and decreased complement activation, resulting in prolonged blood circulation time and reduced cytotoxicity of the biopolymeric PHBHHx NPs.Citation59 It was also known that the inclusion of albumin domain into recombinant adenovirus envelope repelled the macrophage recognition and lengthened their retention in the body.Citation73
Several apolipoproteins such as ApoA4 and ApoC3 significantly reduced cellular uptake when used for NP precoating.Citation15 Apo J, also known as clusterin, has been described to act as a dysopsonin.Citation15,Citation72 Apo J, was identified as a major component in the protein corona of both PEG-and poly(phosphoester) (PPE)-coated NPs, and induced secondary “stealth effects” in addition to the hydrophilic and neutrally charged properties of the polymers themselves.Citation72 Therefore, Apo J might be used as a dysopsonin for decorating NP surface in order to reduce macrophage recognition and lengthen NP circulation time in blood.
One special case is the well-known opsonin, fibrinogen, which may be used to inhibit cell adhesion at suitable concentrations. In particular, at low-density, fibrinogen forms a monolayer that directly attaches to the NP surface,Citation74 leading to high adhesion with platelets and leukocytes. While at high density, the fibrinogen molecules form an extensible multilayer matrix by self-assembly through the interaction between their αC domains,Citation75,Citation76 this dramatically decrease cellular association under both static and flow conditions.Citation74,Citation77 These results can suggest a new approach for synthesis and development of biomaterials, specifically in implantation in order to decrease initial thrombogenesis. Thus, using fibrinogen as a surface precoating material in reasonable quantities provides opportunities to protect NPs from endocytosis and prolong circulation time.
Targeting purpose
NPs with long blood circulation time are a prerequisite for targeted drug delivery, but the accumulation of drug-loaded NPs at the target site is more important than their longer duration of circulation time in the body. Therefore, the surface of conventional NPs has been modified to enhance the stability and lengthen the circulation time of NPs,Citation78 as well as to control biodistribution. Utilizing proteins adsorbed on NP surfaces alters NP distribution in the body. With protein masks, NP size, shape, and surface properties of NPs are readily modified.
Proteins adsorbed onto NP surfaces increase the NP size.Citation20 Specifically, the size of DC-Chol-DOPE/DNA lipoplexes increased from 244±4 nm to 741±1 nm after incubation with FBS. Hence, the entry pathway of the NPs into cells was switched from clathrin-dependent to caveolae-mediated.Citation79 This phenomenon leads to changes in NP cellular internalization, depending on NP size. Moreover, the size of NPs dictates their distribution in the body, as NPs with different size will be accumulated in various organs.Citation80 Hence, besides surface modification, size should be controlled to achieve distribution and accumulation at target sites. Protein coronas may induce NP aggregation due to the construction of protein bridges.Citation81–Citation83 Conversely, in several studies, the adsorption of proteins onto NPs also improves NP stability and prevents formation of NP aggregates.Citation84,Citation85 Therefore, the protein corona can adjust the average size of NPs and may change the cellular uptake pathway and biodistribution.
NPs are decorated with various active moieties and functional groups to selectively deliver drugs to targeted sites in the body. Several targeting functional biomolecules include transferrin, insulin, folic acid, apo A-1, and apo E, which are commonly used in designing cancer-targeting drug delivery systems. Other small molecules such as anhydride, amine, hydroxyl, carboxyl, thiol, and epoxy could be used to alter NP delivery, as they directed NPs to high cellular association with endothelial cells, pancreatic cancer cells, or activated human macrophages.Citation86 Some apolipoproteins, Apo E, ApoA1, and ApoB-100, could functionalize NPs to target nervous system.Citation87,Citation88 Covalently binding Apo E NPs are clearly taken up by the brain endothelial cells while PEGylated NPs did not appear in brain tissue 30 min postadministration.Citation87
Surface modification of NPs by various functional proteins before an administration can be done simply by incubationCitation59,Citation89 or by covalent conjugation.Citation37 A well-known example of this method is nanoparticle albumin-bound (nab) technology, in which albumin is attached to the NP surface to successfully target tumors and reduce toxicity. Abraxane, a prominently marketed anticancer product containing paclitaxel, was fabricated using the nab technique. BSA and human IgG as functional groups were conjugated to polystyrene NP surfaces.Citation37 Artificially decorating NPs with Apo H increased their cellular uptake in HeLa human cancer cells.Citation15 Albumin and Apo E coating of gold NPs not only increases blood circulation time compared to citrate-stabilized gold NPs, but also affects NP biodistribution, shifting NP accumulation from the liver to enhanced amounts in the spleen. Importantly, albumin coating of NP surfaces significantly increased NP delivery into the nervous system and lung. These results could be applied to tailor nanomaterials that target the brain and the lung.Citation90
Though in some reported cases, the targeting properties of NPs may be hindered by protein adsorption, preventing the interaction of functional ligands with corresponding cell receptors.Citation91 For example, transferrin-modified NPs lose their targeting effect due to surface adsorption of biomolecules.Citation92 Additionally, the masking efficiency of proteins onto NPs can be relatively reduced by the presence of competitive proteins that have the same receptors. The cellular uptake of BSA preadsorbed polystyrene NPs was reduced by nearly 70% in MEM supplemented with 10% BSA compared to MEM without BSA addition. Hence, in order to reach to the targeted cell receptors, the active groups, specifically biomolecules which bind to NP surface, must have higher affinity with receptor binding region than nonspecific proteins.Citation9
A more advantageous method for targeting purpose is tailoring the NP properties that are able to selectively adsorb endogenous proteins, which leads the NP to desired cells.Citation93 shows a new targeting strategy for NP design to cells using different surface-modified ligands. Adsorbed proteins may hinder targeting ligands conjugated on the NP surface, but some surface-modified NPs favoring functional proteins can direct NPs to the desired target cells. Modification of NP characteristics, especially their surfaces, will turn the protein corona of NPs by altering interaction between NP and cell membrane receptors, hence governing cellular uptake and responses of NPs.
Figure 3 New targeting strategy for NP design to cells using different surface-modified ligands.
Notes: Left: Adsorbed proteins hinder targeting ligands conjugated on the NP surface. Right: Surface-modified NPs favor functional proteins, which direct NPs to the desired cell.
Abbreviation: NP, nanoparticle.
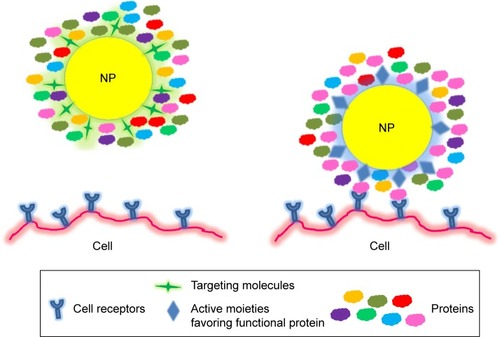
The main strategy in novel NP targeting design includes three steps. First, finding particular proteins that can bind to NPs may be further enhanced with cumulative activity proteins to bring NPs to desired locations. Second, identifying NP-specific characteristics that strengthen the recruitment of the desired proteins and accomplish the formation of a suitable protein corona with or without modifying the natural protein structure so as to pursue the intended targeting responses. Third, considering the influence of protein exchange over time on biological and therapeutic outcomes.Citation94
For example, when incubating with 50% plasma, liposomes prepared from 1,2-dioleoyl-3-trimethylammonium propane were enriched with vitronectin, which is a major ligand for the vitronectin receptor in several tumor cell lines. Protein–NP complex showed higher cellular uptake in metastatic MDA-MB-435 cells than bare NPs.Citation95 Retinol binding protein 4 (RBP) was favorably adsorbed to retinol-conjugated polyetherimide NPs. RBP was found to bind retinol and direct the retinol-conjugated polyetherimine (RcP) NPs to hepatic stellate cells.Citation96
After exposing to plasma, polysorbate-coated NPs were enriched with Apo E or Apo AI, and highly distributed in brain endothelium, which was also observed in the case of covalent Apo E binding NPs.Citation88,Citation97 Polybutyl cyanoacrylate (PBC) NPs and polymethyl methacrylate (PMMA) NPs covered by polysorbates could also selectively recruit apolipoproteins binding to their surfaces for brain-targeting applications.Citation98–Citation100
Coating NPs with particular proteins can help determine the adsorbed protein patterns onto NPs. Preformed NPs with HSA decrease the recruitment of coagulation factors, especially fibrinogen, while γ globulin layer around NPs enhanced the relative quantities of complement factors and immunoglobulins. However, the adsorbed proteins recruited from 55% plasma screened the effect of γ globulin layer, which was indicated by lower cellular uptake on RAW 264.7 cells than the ones that interacted with 10% FBS-containing media. This can be explained by the difference of protein quantity between 55% plasma solution and 10% FBS. Therefore, protein-functionalized NPs should be exposed to media for a shorter and more reasonable duration in which the efficiency of NP ligands still retain and express their effect before being affected by the adsorption of other media proteins.Citation101 When NPs come into contact with biological fluid, the evolution of proteins is essential to be considered as the difference in the adsorbed proteins’ compositions, their relative abundances, and total binding proteins strongly affect cellular uptake and therapeutic responses.Citation101
Small libraries of synthetic polymeric NPs mainly fabricated with poly(N-isopropylacrylamide) (NIPAm) and other functional monomers at different ratios could be screened for control of protein adsorption. NP compositions were successfully optimized for binding affinity and selectivity toward desired proteins in complicated biological environments. Hence, these NIPAm NPs can be applied in protein purification, sequestration of lipid-bound toxins, and targeted drug delivery systems by turning the protein corona through NP tailoring.Citation43
Toxicity reduction
The interaction with NP has a strong impact on cellular behaviors and may cause cell death through a disruption of the integrity of plasma membrane, mitochondrial impairment, and nucleus damage. NPs could provoke inflammatory and oxidative stress, which finally results in cell death. The formation of protein corona alters the immune response of NPs.Citation102 The protective effect of adsorbed protein against cell damage induced by the bare NPs was investigated in numerous studies,Citation35,Citation103,Citation104 possibly because protein covered the NP surface and made NPs more biocompatible to the human body.
A BSA adsorbed layer reduced the cell cytotoxicity of naked PHBHHx NPs, obviously with high NP treatment of three different cell lines: A549, HepG2, and L929 cells.Citation59 The masking effects of several types of human blood proteins, specifically, bovine fibrinogen (BFG), BSA, transferrin (Tf), and γ globulin (Ig), on single wall carbon nanotubes (SWCNTs) decreased toxicity compared to bare SWCNTs in human acute monocytic leukemia cells (THP-1) and human umbilical vein endothelial cells (HUVECs). The impacts of these SWCNT–protein complexes had a similar decreasing order to their protein affinities: BFG > Ig > Tf > BSA for THP-1 cells.Citation105 Using BSA for coating of ZnO NPs, prepared at 4°C, reduced the toxicity of uncoated ZnO NPs on CHO cells and inhibited ZnO NPs from inducing ROS generation. However, the change of BSA structure when BSA coating happened at an elevated 20°C lessened the toxicity reduction effect of BSA for ZnO NPs.Citation106 Hence, surface modification procedure by adsorbing proteins needs to be carefully considered and optimized to obtain the best therapeutic effects. Adsorbed proteins covering positively charged polystyrene compensated the NP surface charges and were retained when NPs made contact with cell (lung epithelial A549 cells and human brain astrocytoma 1321N1 cells) membranes, and only degraded when trafficked to lysosomes. In this case, the protein corona could mask the polystyrene NPs by preventing direct contact between bare NPs and cell membranes, thereby inhibiting cell death caused by positively charged NPs.Citation35
Future perspectives
It is not bare NPs, but protein-adsorbed NPs that represent the true identity and therapeutic responses of NPs when they enter into the body. Therefore, a thorough knowledge and understanding of protein–NP complex and its interactions is important to predict and govern the in vivo fate of NPs after administration, including biodistribution, bioavailability responses, and toxicity. The correlation models for biological and therapeutic outcomes based on protein corona patterns of NPs are validated with considerably higher accuracy than previous models whose input factors include mainly NP physicochemical properties. Hence, bioavailability of an NP product would be able to be precisely measured without in vivo tests on humans. From these correlative models, nanomaterials can be synthesized for determination of the required binding protein motifs for novel drug delivery carriers or diagnostic devices.Citation107 Protein corona patterns, especially proteins which have powerful impacts on cellular uptake and biological responses, can be used to identify the overexpressed receptors on targeting cells as novel pharmacological approaches are discovered.Citation108 Moreover, the presence of different types of adsorbed proteins on NPs provides details about the biological fluids and blood components which NPs go through in the body. Thus, these information can be used for examining and diagnosing the physiological changes in our body related to protein modulation and structural deformation, such as in tumorigenesis and disease development.Citation93
To enhance the precise prediction of in vivo biological outcomes and practical applications of protein corona in pharmaceutical sciences, initially a full assessment of NP–protein interaction in stimulated biomimetic administration conditions must be done.Citation109 Specifically, media exposure factors including proteins, fluidic shear stress, and also degradable enzymes should be carefully set up. Besides, the invention of new pharmaceutical technologies such as tumor-on-a-chip models and biomimetic microfluidic systems allow for more deeper evaluation of NP behaviors.Citation110
Notably, different types of diseases can cause decoration of the NP protein.Citation111 The changes in plasma protein concentrations, protein structures, and body temperature mediated by different disease states and medical conditions, extensively including breast cancer, diabetes, hypercholesterolemia, rheumatism, fauvism, smoking, hemodialysis, thalassemia, hemophilia A and B, pregnancy, common cold, and hypofibrinogenemia, alter NP-adsorbed protein pattern This new finding also suggests new avenues for creating and designing safe and highly efficient NPs for personalized drug delivery system.Citation112
NP–protein interaction offers potential way to design nanoparticulate drug delivery systems in which controlling protein and protein corona can mask NP surface and perform desirable biological functions.Citation113 The “dysopsonins” such as BSA and Apo J offer a simple way to create and enrich the “stealth effect” for NPs, in which NPs are just mixed and controlled with human plasma or a specific protein component before administration,Citation69,Citation72 instead of undergoing complicated preparation procedures of NPs. Protein structural modification and approachability of adsorbed proteins should be carefully considered in the tailoring of preformed protein NPs for effective therapeutic activities mediated through binding with relevant cell receptors.Citation114 Protein directionality decides the flexibility and availability of optimal binding sites and protein function. Therefore, the directionality of the protein as well as the degree of conjugation/adsorption is essential for NP construction and design in drug delivery. The orientation of the protein component and the level of affected protein structures/dysfunction depend on the interactive region of proteins. Cautious consideration about surface topography and chemistry as well as detailed structural protein information will lead to greater managing of the orientation and properties of decorated proteins on NP surface.Citation115 Materials or nanomaterials which poorly adsorb protein will lead to the positive effect of prolonged circulation time but also the negative effect of low cellular uptake, in the case of hydrophilic polymers and vice versa.Citation24,Citation72 Hence, the balance and harmonization between the complicated effect of diverse binding proteins on biological effectiveness and escape of phagocytosis is a challenging task in tailoring and designing NPs.
In summary, the in-depth understanding and knowledge of protein corona opens up a new method for NP design in which the creative production of novel nanomaterials would strategically begin with the identification of essential fingerprint pattern and conformation of protein corona to achieve the desired biological and therapeutic outomes.Citation116
Acknowledgments
This research was supported by a grant (16173MFDS542) from Ministry of Food and Drug Safety in 2016, Republic of Korea.
Disclosure
The authors report no conflicts of interest in this work.
References
- CorboCMolinaroRParodiAToledano FurmanNESalvatoreFTasciottiEThe impact of nanoparticle protein corona on cytotoxicity, immunotoxicity and target drug deliveryNanomedicine20161118110026653875
- VromanLAdamsAFischerGCMunozPCInteraction of high molecular weight kininogen, factor XII, and fibrinogen in plasma at interfacesBlood19805511561597350935
- MahmoudiMSheibaniSMilaniASCrucial role of the protein corona for the specific targeting of nanoparticlesNanomedicine201510221522625600967
- LundqvistMStiglerJEliaGLynchICedervallTDawsonKANanoparticle size and surface properties determine the protein corona with possible implications for biological impactsProc Natl Acad Sci U S A200810538142651427018809927
- SacchettiCMotamedchabokiKMagriniASurface polyethylene glycol conformation influences the protein corona of polyethylene glycol-modified single-walled carbon nanotubes: potential implications on biological performanceACS Nano2013731974198923413928
- LynchIDawsonKAProtein-nanoparticle interactionsNano Today2008314047
- KoeglerPClaytonAThissenHSantosGNKingshottPThe influence of nanostructured materials on biointerfacial interactionsAdv Drug Deliv Rev201264151820183922705547
- HühnDKantnerKGeidelCPolymer-coated nanoparticles interacting with proteins and cells: focusing on the sign of the net chargeACS Nano2013743253326323566380
- FleischerCCPayneCKNanoparticle–cell interactions: molecular structure of the protein corona and cellular outcomesAcc Chem Res20144782651265925014679
- FleischerCCKumarUPayneCKCellular binding of anionic nanoparticles is inhibited by serum proteins independent of nanoparticle compositionBiomater Sci20131997598223956836
- Goy-LópezSJuárezJAlatorre-MedaMPhysicochemical characteristics of protein–NP bioconjugates: the role of particle curvature and solution conditions on human serum albumin conformation and fibrillogenesis inhibitionLangmuir201228249113912622439664
- Orts-GilGNatteKThiermannROn the role of surface composition and curvature on biointerface formation and colloidal stability of nanoparticles in a protein-rich model systemColloids Surf B Biointerfaces201310811011923528607
- StaufenbielSMerinoMLiWSurface characterization and protein interaction of a series of model poly[acrylonitrile-co-(N-vinyl pyrrolidone)] nanocarriers for drug targetingInt J Pharm20154851–2879625746947
- GaucherGAsahinaKWangJLerouxJCEffect of poly (N-vinyl-pyrrolidone)-block-poly (D, L-lactide) as coating agent on the opsonization, phagocytosis, and pharmacokinetics of biodegradable nanoparticlesBiomacromolecules200910240841619133718
- RitzSSchöttlerSKotmanNProtein corona of nanoparticles: distinct proteins regulate the cellular uptakeBiomacromolecules20151641311132125794196
- KangBOkwiekaPSchöttlerSTailoring the stealth properties of biocompatible polysaccharide nanocontainersBiomaterials20154912513425725561
- LundqvistMStiglerJCedervallTThe evolution of the protein corona around nanoparticles: a test studyACS Nano2011597503750921861491
- MaioranoGSabellaSSorceBEffects of cell culture media on the dynamic formation of protein–nanoparticle complexes and influence on the cellular responseACS Nano20104127481749121082814
- MartelJYoungDYoungAComprehensive proteomic analysis of mineral nanoparticles derived from human body fluids and analyzed by liquid chromatography–tandem mass spectrometryAnal Biochem2011418111112521741946
- DobrovolskaiaMAPatriAKZhengJInteraction of colloidal gold nanoparticles with human blood: effects on particle size and analysis of plasma protein binding profilesNanomedicine20095210611719071065
- JosephDSacharSKishoreNChandraSMechanistic insights into the interactions of magnetic nanoparticles with bovine serum albumin in presence of surfactantsColloids Surf B Biointerfaces201513559660326322473
- PalchettiSColapicchioniVDigiacomoLThe protein corona of circulating PEGylated liposomesBiochim Biophys Acta201618582189196
- TenzerSDocterDKuharevJRapid formation of plasma protein corona critically affects nanoparticle pathophysiologyNat Nanotechnol201381077278124056901
- Sanchez-MorenoPBuzonPBoulaizHBalancing the effect of corona on therapeutic efficacy and macrophage uptake of lipid nanocapsulesBiomaterials20156126627826005765
- ShubhraQTTothJGyenisJFeczkoTSurface modification of HSA containing magnetic PLGA nanoparticles by poloxamer to decrease plasma protein adsorptionColloids Surf B Biointerfaces201412252953625092588
- LindmanSLynchIThulinENilssonHDawsonKALinseSSystematic investigation of the thermodynamics of HSA adsorption to N-iso-propylacrylamide/N-tert-butylacrylamide copolymer nanoparticles. Effects of particle size and hydrophobicityNano Lett20077491492017335269
- MonopoliMPWalczykDCampbellAPhysical − chemical aspects of protein corona: relevance to in vitro and in vivo biological impacts of nanoparticlesJ Am Chem Soc201113382525253421288025
- DiederichsJEPlasma protein adsorption patterns on liposomes: establishment of analytical procedureElectrophoresis19961736076118740186
- ThodeKLückMSemmlerWMüllerRHKresseMDetermination of plasma protein adsorption on magnetic iron oxides: sample preparationPharm Res19971479059109244148
- CedervallTLynchILindmanSUnderstanding the nanoparticle–protein corona using methods to quantify exchange rates and affinities of proteins for nanoparticlesProc Natl Acad Sci U S A200710472050205517267609
- WalkeyCDOlsenJBSongFProtein corona fingerprinting predicts the cellular interaction of gold and silver nanoparticlesACS Nano2014832439245524517450
- WinzenSSchoettlerSBaierGComplementary analysis of the hard and soft protein corona: sample preparation critically effects corona compositionNanoscale2015772992300125599336
- LesniakAFenaroliFMonopoliMPÅbergCDawsonKASalvatiAEffects of the presence or absence of a protein corona on silica nanoparticle uptake and impact on cellsACS Nano2012675845585722721453
- GrafeCWeidnerALuheMVIntentional formation of a protein corona on nanoparticles: serum concentration affects protein corona mass, surface charge, and nanoparticle-cell interactionInt J Biochem Cell Biol20167519620226556312
- WangFYuLMonopoliMPThe biomolecular corona is retained during nanoparticle uptake and protects the cells from the damage induced by cationic nanoparticles until degraded in the lysosomesNanomedicine2013981159116823660460
- YallapuMMChauhanNOthmanSFImplications of protein corona on physico-chemical and biological properties of magnetic nanoparticlesBiomaterials20154611225678111
- ThieleLDiederichsJEReszkaRMerkleHPWalterECompetitive adsorption of serum proteins at microparticles affects phagocytosis by dendritic cellsBiomaterials20032481409141812527282
- BehzadiSSerpooshanVSakhtianchiRProtein corona change the drug release profile of nanocarriers: the “overlooked” factor at the nanobio interfaceColloids Surf B Biointerfaces201412314314925262409
- KangTParkCChoiJSCuiJHLeeBJEffects of shear stress on the cellular distribution of polystyrene nanoparticles in a biomimetic microfluidic systemJ Drug Deliv Sci Technol201631130136
- MariebENHoehnKThe Cardiovascular System: Blood Vessels9th edUpper Saddle River, NJPearson Education, Inc2013
- BhogaleAPatelNMariamJDongrePMiotelloAKothariDComprehensive studies on the interaction of copper nanoparticles with bovine serum albumin using various spectroscopiesColloids Surf B Biointerfaces201411327628424121071
- MahmoudiMAbdelmonemAMBehzadiSTemperature: the “ignored” factor at the NanoBio interfaceACS Nano2013786555656223808533
- O’BrienJSheaKJTuning the protein corona of hydrogel nanoparticles: the synthesis of abiotic protein and peptide affinity reagentsAcc Chem Res20164961200121027254382
- CapriottiALCaraccioloGCarusoGDifferential analysis of “protein corona” profile adsorbed onto different nonviral gene delivery systemsAnal Biochem2011419218018921867671
- MahmoudiMLynchIEjtehadiMRMonopoliMPBombelliFBLaurentSProtein-nanoparticle interactions: opportunities and challengesChem Rev201111195610563721688848
- M’BarekKBMolinoDQuignardSPhagocytosis of immunoglobulin-coated emulsion dropletsBiomaterials20155127027725771017
- ShawSYWestlyECPittetMJSubramanianASchreiberSLWeisslederRPerturbational profiling of nanomaterial biologic activityProc Natl Acad Sci U S A2008105217387739218492802
- SoenenSJHimmelreichUNuyttenNDe CuyperMCytotoxic effects of iron oxide nanoparticles and implications for safety in cell labellingBiomaterials201132119520520863560
- ZhangHJiZXiaTUse of metal oxide nanoparticle band gap to develop a predictive paradigm for oxidative stress and acute pulmonary inflammationACS Nano2012654349436822502734
- SoenenSJRivera-GilPMontenegroJMParakWJDe SmedtSCBraeckmansKCellular toxicity of inorganic nanoparticles: common aspects and guidelines for improved nanotoxicity evaluationNano Today201165446465
- LiuRRalloRGeorgeSClassification NanoSAR development for cytotoxicity of metal oxide nanoparticlesSmall2011781118112621456088
- PuzynTLeszczynskaDLeszczynskiJToward the development of “nano-QSARs”: Advances and challengesSmall20095222494250919787675
- PuzynTRasulevBGajewiczAUsing nano-QSAR to predict the cytotoxicity of metal oxide nanoparticlesNature Nanotechnol20116317517821317892
- FourchesDPuDTassaCQuantitative nanostructure − activity relationship modelingACS Nano20104105703571220857979
- LiuRJiangWWalkeyCDChanWCCohenYPrediction of nanoparticles-cell association based on corona proteins and physicochemical propertiesNanoscale20157219664967525959034
- BigdeliAPalchettiSPozziDExploring cellular interactions of liposomes using protein corona fingerprints and physicochemical propertiesACS Nano20161033723373726882007
- PalchettiSDigiacomoLPozziDNanoparticles-cell association predicted by protein corona fingerprintsNanoscale2016825127551276327279572
- OgawaraKFurumotoKNagayamaSPre-coating with serum albumin reduces receptor-mediated hepatic disposition of polystyrene nanosphere: implications for rational design of nanoparticlesJ Control Release2004100345145515567509
- PengQZhangSYangQPreformed albumin corona, a protective coating for nanoparticles based drug delivery systemBiomaterials201334338521853023932500
- GöppertTMüllerRAdsorption kinetics of plasma proteins on solid lipid nanoparticles for drug targetingInt J Pharm2005302117218616098695
- RodriguezPLHaradaTChristianDAPantanoDATsaiRKDischerDEMinimal “Self” peptides that inhibit phagocytic clearance and enhance delivery of nanoparticlesScience2013339612297197523430657
- GrunérMKauscherULinderMMonopoliMAn environmental route of exposure affects the formation of nanoparticle coronas in blood plasmaJ Proteomics2016137525826546559
- MüllerJBauerKNProzellerDCoating nanoparticles with tunable surfactants facilitates control over the protein coronaBiomaterials20171151827871002
- AggarwalPHallJBMcLelandCBDobrovolskaiaMAMcNeilSENanoparticle interaction with plasma proteins as it relates to particle biodistribution, biocompatibility and therapeutic efficacyAdv Drug Deliv Rev200961642843719376175
- WangQSunYZhangZDuanYTargeted polymeric therapeutic nanoparticles: Design and interactions with hepatocellular carcinomaBiomaterials20155622924025934295
- OwensDEPeppasNAOpsonization, biodistribution, and pharmacokinetics of polymeric nanoparticlesInt J Pharm200630719310216303268
- LemarchandCGrefRLesieurSPhysico-chemical characterization of polysaccharide-coated nanoparticlesJ Control Release200510819711116169626
- SzleiferICarignanoMTethered polymer layers: phase transitions and reduction of protein adsorptionMacromol Rapid Commun2000218423448
- NatteKFriedrichJFWohlrabSImpact of polymer shell on the formation and time evolution of nanoparticle – protein coronaColloids Surf B Biointerfaces201310421322023318220
- GrefRDombAQuellecPThe controlled intravenous delivery of drugs using PEG-coated sterically stabilized nanospheresAdv Drug Deliv Rev201264316326
- KnopKHoogenboomRFischerDSchubertUSPoly (ethylene glycol) in drug delivery: pros and cons as well as potential alternativesAngew Chem Int Ed Engl201049366288630820648499
- SchöttlerSBeckerGWinzenSProtein adsorption is required for stealth effect of poly (ethylene glycol)-and poly (phosphoester)-coated nanocarriersNat Nanotechnol201611437237726878141
- RojasLACondezoGNMorenoRAlbumin-binding adenoviruses circumvent pre-existing neutralizing antibodies upon systemic deliveryJ Control Release2016237788827388756
- YermolenkoISFuhrmannAMagonovSNOrigin of the nonadhesive properties of fibrinogen matrices probed by force spectroscopyLangmuir20102622172691727720883009
- YermolenkoISGorkunOVFuhrmannAThe assembly of nonadhesive fibrinogen matrices depends on the αC regions of the fibrinogen moleculeJ Biol Chem201228750419794199023086938
- SafiullinRChristensonWOwaynatHFibrinogen matrix deposited on the surface of biomaterials acts as a natural anti-adhesive coatingBiomaterials20156715115926210181
- LishkoVKBurkeTUgarovaTAntiadhesive effect of fibrinogen: a safeguard for thrombus stabilityBlood200710941541154916849640
- StormGBelliotSODaemenTLasicDDSurface modification of nanoparticles to oppose uptake by the mononuclear phagocyte systemAdv Drug Deliv Rev19951713148
- CaraccioloGCallipoLDe SanctisSCCavaliereCPozziDLaganàASurface adsorption of protein corona controls the cell internalization mechanism of DC-Chol–DOPE/DNA lipoplexes in serumBiochim Biophys Acta20101798353654319917267
- GaumetMVargasAGurnyRDelieFNanoparticles for drug delivery: the need for precision in reporting particle size parametersEur J Pharm Biopharm20086911917826969
- DengZJLiangMTothIMonteiroMJMinchinRFMolecular interaction of poly (acrylic acid) gold nanoparticles with human fibrinogenACS Nano20126108962896922998416
- ZhuJSunZLiJJZhaoJWBovine serum albumins (BSA) induced aggregation and separation of gold colloid nanoparticlesJ Nanosci Nanotechnol20121232206221122755039
- KendallMDingPKendallKParticle and nanoparticle interactions with fibrinogen: the importance of aggregation in nanotoxicologyNanotoxicology201151556521417688
- Dominguez-MedinaSBlankenburgJOlsonJLandesCFLinkSAdsorption of a protein monolayer via hydrophobic interactions prevents nanoparticle aggregation under harsh environmental conditionsACS Sustain Chem Eng20131783384223914342
- WellsMAAbidAKennedyIMBarakatAISerum proteins prevent aggregation of Fe2O3 and ZnO nanoparticlesNanotoxicology20126883784622149273
- WeisslederRKellyKSunEYShtatlandTJosephsonLCell-specific targeting of nanoparticles by multivalent attachment of small moleculesNat Biotechnol200523111418142316244656
- ZensiABegleyDPontikisCAlbumin nanoparticles targeted with Apo E enter the CNS by transcytosis and are delivered to neuronesJ Control Release20091371788619285109
- KreuterJHekmataraTDreisSVogelTGelperinaSLangerKCovalent attachment of apolipoprotein AI and apolipoprotein B-100 to albumin nanoparticles enables drug transport into the brainJ Control Release20071181545817250920
- VenerandoRMiottoGMagroMMagnetic nanoparticles with covalently bound self-assembled protein corona for advanced biomedical applicationsJ Phys Chem C2013117392032020331
- SchäfflerMSousaFWenkABlood protein coating of gold nanoparticles as potential tool for organ targetingBiomaterials201435103455346624461938
- MirshafieeVMahmoudiMLouKChengJKraftMLProtein corona significantly reduces active targeting yieldChem Commun2013492525572559
- SalvatiAPitekASMonopoliMPTransferrin-functionalized nanoparticles lose their targeting capabilities when a biomolecule corona adsorbs on the surfaceNat Nanotechnol20138213714323334168
- CaraccioloGLiposome–protein corona in a physiological environment: challenges and opportunities for targeted delivery of nanomedicinesNanomedicine201511354355725555353
- RahmanMLaurentSTawilNYahiaLMahmoudiMProtein-Nanoparticle Interactions151st edBerlin, GermanySpringer-Verlag2013
- CaraccioloGPozziDCapriottiACancer cell targeting of lipid gene vectors by protein coronaNanotechnology20123354357
- ZhangZWangCZhaYCorona-directed nucleic acid delivery into hepatic stellate cells for liver fibrosis therapyACS Nano2015932405241925587629
- PetriBBootzAKhalanskyAChemotherapy of brain tumour using doxorubicin bound to surfactant-coated poly (butyl cyanoacrylate) nanoparticles: revisiting the role of surfactantsJ Control Release20071171515817150277
- BorchardGAudusKLShiFKreuterJUptake of surfactant-coated poly (methyl methacrylate)-nanoparticles by bovine brain microvessel endothelial cell monolayersInt J Pharm199411012935
- GulyaevAEGelperinaSESkidanINAntropovASKivmanGYKreuterJSignificant transport of doxorubicin into the brain with polysorbate 80-coated nanoparticlesPharm Res199916101564156910554098
- KreuterJPetrovVKharkevichDAlyautdinRInfluence of the type of surfactant on the analgesic effects induced by the peptide dalargin after its delivery across the blood–brain barrier using surfactant-coated nanoparticlesJ Control Release19974918187
- MirshafieeVKimRParkSMahmoudiMKraftMLImpact of protein pre-coating on the protein corona composition and nanoparticle cellular uptakeBiomaterials20167529530426513421
- LeeYKChoiEJWebsterTJKimSHKhangDEffect of the protein corona on nanoparticles for modulating cytotoxicity and immunotoxicityInt J Nanomedicine2015109725565807
- CliftMJBhattacharjeeSBrownDMStoneVThe effects of serum on the toxicity of manufactured nanoparticlesToxicol Lett2010198335836520705123
- TedjaRLimMAmalRMarquisCEffects of serum adsorption on cellular uptake profile and consequent impact of titanium dioxide nanoparticles on human lung cell linesACS Nano2012654083409322515565
- GeCDuJZhaoLBinding of blood proteins to carbon nanotubes reduces cytotoxicityProc Nat Acad Sci201110841169681697321969544
- ŽūkienėRSnitkaVZinc oxide nanoparticle and bovine serum albumin interaction and nanoparticles influence on cytotoxicity in vitroColloids Surf B Biointerfaces201513531632326275837
- MiottoGMagroMTerzoMProtein corona as a proteome fingerprint: the example of hidden biomarkers for cow mastitisColloids Surf B Biointerfaces2016140404926735893
- ArvizoRRGiriKMoyanoDIdentifying new therapeutic targets via modulation of protein corona formation by engineered nanoparticlesPloS One201273e3365022442705
- MirshafieeVKimRMahmoudiMKraftMLThe importance of selecting a proper biological milieu for protein corona analysis in vitro: human plasma versus human serumInt J Biochem Cell Biol20167518819526643610
- MaioloDDel PinoPMetrangoloPParakWJBaldelli BombelliFNanomedicine delivery: does protein corona route to the target or off road?Nanomedicine201510213231324726470748
- CaraccioloGCaputoDPozziDColapicchioniVCoppolaRSize and charge of nanoparticles following incubation with human plasma of healthy and pancreatic cancer patientsColloids Surf B Biointerfaces201412367367825456990
- HajipourMJLaurentSAghaieARezaeeFMahmoudiMPersonalized protein coronas: a “key” factor at the nanobiointerfaceBiomat Sci20142912101221
- PengQMuHThe potential of protein–nanomaterial interaction for advanced drug deliveryJ Control Release201622512113226812004
- ZanganehSSpitlerRErfanzadehMAlkilanyAMMahmoudiMProtein corona: opportunities and challengesInt J Biochemist Cell Biol201675143147
- MahonESalvatiABombelliFBLynchIDawsonKADesigning the nanoparticle–biomolecule interface for “targeting and therapeutic delivery”J Control Release2012161216417422516097
- Hamad-SchifferliKExploiting the novel properties of protein coronas: emerging applications in nanomedicineNanomedicine (Lond)201510101663167426008198
- SchuchardMMelmCCrawfordHSpecific depletion of twenty high abundance proteins from human plasmaPaper presented at: NCI Proteomic Technologies Reagents Resource WorkshopDecember 12–13; 2005Chicago, IL
- MuQLiuWXingYProtein binding by functionalized multiwalled carbon nanotubes is governed by the surface chemistry of both parties and the nanotube diameterJ Phys Chem C2008112933003307
- LundqvistMSethsonIJonssonBHProtein adsorption onto silica nanoparticles: conformational changes depend on the particles’ curvature and the protein stabilityLangmuir20042024106391064715544396
- XuFReiserMYuXGummuluruSWetzlerLReinhardBMLipid-mediated targeting with membrane-wrapped nanoparticles in the presence of corona formationACS Nano20161011189120026720275
- WalkeyCDOlsenJBGuoHEmiliAChanWCNanoparticle size and surface chemistry determine serum protein adsorption and macrophage uptakeJ Am Chem Soc201213442139214722191645
- TenzerSDocterDRosfaSNanoparticle size is a critical physicochemical determinant of the human blood plasma corona: a comprehensive quantitative proteomic analysisACS Nano2011597155716721866933
- GagnerJELopezMDDordickJSSiegelRWEffect of gold nanoparticle morphology on adsorbed protein structure and functionBiomaterials201132297241725221705074
- ChellatFMerhiYMoreauAYahiaLTherapeutic potential of nanoparticulate systems for macrophage targetingBiomaterials200526357260727516023200
- GessnerAWaiczRLieskeAPaulkeBRMäderKMüllerRNanoparticles with decreasing surface hydrophobicities: influence on plasma protein adsorptionInt J Pharm2000196224524910699728