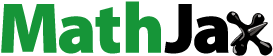
Abstract
Bloodstream infection, especially with implants involved, is an often life-threatening condition with high mortality rates, imposing a heavy burden on patients and medical systems. Herein, we firstly deposited homogeneous vanadium metal, V2O3, VO2, and V2O5 nanofilms on quartz glass by magnetron sputtering. Using these platforms, we further investigated the potential antimicrobial efficiency of these nano-VOx films and the interactions of human erythrocytes and bacteria (methicillin-resistant Staphylococcus aureus and Pseudomonas aeruginosa) with our samples in a novel cell–bacteria coculture model. It was demonstrated that these nano-VOx precipitated favorable antibacterial activity on both bacteria, especially on S. aureus, and this effect increased with higher vanadium valence. A possible mechanism accountable for these results might be elevated levels of vanadium-induced intracellular reactive oxygen species. More importantly, based on hemolysis assays, our nano-VOx films were found to be able to kill prokaryotic cells but were not toxic to mammalian cells, holding the potential for the prevention of implant-related hematogenous infections. As far as we know, this is the first report wherein such nano-VOx films have assisted human erythrocytes to combat bacteria in a valence-dependent manner. Additionally, vanadium ions were released from these nano-VOx films in a sustained manner, and low-valence films possessed better biocompatibility with human fibroblasts. This work may provide new insights for biomedical applications of inorganic vanadium compounds and attract growing attention in this field. From the perspective of surface modification and functionalization, this study holds promise to avail the prophylaxis of bloodstream infections involving implantable biomedical devices.
Introduction
Nowadays, medical implants have become critical devices in modern health care, though they cause major risks of serious bloodstream infections (BSIs), since these inserts act as a portal for the entry of pathogens into the host bloodstream.Citation1–Citation3 Currently, an increasing number of implant-related BSIs not only present high mortality and disability rates but also bring heavy financial burden to families and societies, despite the rapid development of antimicrobial agents, adjunctive therapies, and life-support facilities.Citation4,Citation5 Gram-positive Staphylococcus aureus is one of the most common pathogens causing BSIs,Citation3,Citation6 and methicillin-resistant S. aureus (MRSA) is of great danger especially, since it can invalidate the therapeutic use of most β-lactam antibiotics of first choice against staphylococci.Citation7 Also, in many hospitals Pseudomonas aeruginosa has become an epidemic BSI-associated Gram-negative bacteria, leading to significant patient mortality and health care costs.Citation8 Moreover, early bacterial colonization on the surface of implants and following biofilm formation contribute to the extreme difficulties in curing such BSIs.Citation9,Citation10 Inappropriate antibiotic treatment may exacerbate BSI-associated mortality.Citation11 Therefore, compared with the arduous treatment for these refractory BSIs, preventive strategies seem more effective to combat them. In this context, there is a need to develop new antimicrobial agents and strategies to prevent implant BSIs, especially those applicable in the blood microenvironment. As a promising alternative to the current antibiotic-based method, nanomaterial-based antimicrobials provide a new paradigm for conquering antibiotic-resistant pathogens.Citation12–Citation14
Among subcellular organelles, the intracellular mitochondrion has been found to be the main target for the accumulation and cytotoxicity of vanadium species in biological systems.Citation15–Citation17 On the basis of endosymbiotic theory, a bacterium is the analogue of the mitochondrion of a mammalian cell.Citation18 Therefore, vanadium species have the potential to combat bacteria. In fact, previous works together with our recent study have observed the antibacterial activity of vanadium-coordination organic compounds and inorganic vanadium dioxide (VO2).Citation19–Citation21 The vanadium element has six natural oxidation states. Trivalence (+3), tetravalence (+4), and pentavalence (+5) are the most common forms. For inorganic vanadium oxides, the corresponding oxides are V2O3, VO2 and V2O5. The cytotoxicity of vanadium shows dose dependence.Citation22,Citation23 Actually, just as Paracelsus stated, “Alle Dinge sind Gift und nichts ist ohne Gift; allein die Dosis macht daß ein Ding kein Gift ist” (“all substances are poisonous; it is only the dose that differentiates between a poison and a remedy”).Citation24 No solid basis exists for categorizing vanadium compounds as harmful toxicants when administered at sensible dosage.Citation22 Furthermore, vanadium is presumably one of the essential trace elements in the human body, though this has not yet been definitely proven,Citation22 and appropriate dosage of vanadium can regulate phosphate metabolism.Citation25 Only high doses of vanadium may cause toxic effects on normal cells.Citation26,Citation27
Considering the facts that human red blood cells (erythrocytes) have no mitochondria, bacteria resemble mitochondria, and S. aureus causes lysis of host cells by secreting α-hemolysin monomers and cytolytic peptides,Citation28,Citation29 we were inspired to coculture erythrocytes and bacteria (MRSA and P. aeruginosa) on vanadium-containing films and investigate the biological response of erythrocytes and bacteria in this novel coculturing system. Our present work aims to explore whether vanadium-containing surface can show erythrocyte-assisting but bacteria-killing functions. For this purpose, we firstly designed V metal, V2O3, VO2, and V2O5 nanoparticle films on quartz glass substrates by magnetron sputtering, and further explored the antimicrobial activity of these nano-VOx films and interactions of human erythrocytes and bacteria with these samples. We hope this work can provide new insight for potential applications of inorganic vanadium-based nanomaterials as surface functional layers in BSI prophylaxis.
Materials and methods
Sample preparation and characterization
Homogeneous V metal, V2O3, and VO2 films of high quality were prepared by a magnetron-sputtering apparatus (ACS-4000-C4; Ulvac Technologies, Methuen, MA, USA) using corresponding vanadium metal and oxide targets. To obtain V2O5 film, deposited VO2 film underwent subsequent annealing at 450°C in O2 atmosphere. The thicknesses of these films were detected by an F20 thin-film analyzer (Filmetrics, San Diego, CA, USA). The samples used all had the same thickness of 50 nm. Fused quartz (SiO2) acted as the deposition substrate for films. The whole fabrication process is illustrated in . The samples were denoted as glass (quartz), V0 (homogeneous vanadium metal nanoparticles deposited on quartz glass), V3 (homogeneous V2O3 nanoparticles deposited on quartz glass), V4 (homogeneous VO2 nanoparticles deposited on quartz glass), and V5 (homogeneous V2O5 nanoparticles deposited on quartz glass).
Field-emission scanning electron microscopy (SEM; S-4800; Hitachi, Tokyo, Japan) was used to observe the surface morphology of the samples. X-ray diffraction (XRD; Ultima IV; Rigaku, Tokyo, Japan) was utilized to study the crystalline structure of films, fitted with CuKα radiation (λ=1.541 Å) at voltage of 40 kV and current of 40 mA. The XRD patterns were recorded in the range of 15°–80° (2θ) with step size of 0.02° and scanning rate of 2°/min. For the XRD test, the glancing-incidence angle was fixed at 1°. Phase identification was performed with the help of the standard Joint Committee on Powder Diffraction Standards database. The chemical composition, chemical state, and elemental depth profile were studied by X-ray photoelectron spectroscopy (XPS; Escalab 250Xi; Thermo Fisher Scientific, Waltham, MA, USA) with AlKα (1,486.6 eV) illumination.
Vanadium-ion release
The release profiles of vanadium ions from the V0, V3, V4 and V5 samples immersed in Dulbecco’s Modified Eagle’s Medium (DMEM), trypticase soy broth (TSB), and phosphate-buffered saline (PBS) were detected by inductively coupled plasma optical emission spectrometry (Liberty 150; Varian Medical Systems, Palo Alto, CA, USA). The testing samples were positioned in sterile micro-centrifuge tubes (15 mL) filled with 10 mL fresh medium, followed by 1, 4, 7 and 14 days of sequential incubation at 37°C in static conditions. At the end of each incubation period, all the leachates were collected for detection and the microcentrifuge tubes supplemented with fresh medium for subsequent incubation.
In vitro cytotoxicity evaluation
The human HFF1 cell line was utilized to assess the cytotoxicity of our specimens, and cell viability was determined with the CCK-8 assay (Beyotime, Haimen, China). The glass, V0, V3, V4, and V5 samples were sterilized in ethanol solution (75 v/v%) and dried on a clean bench. HFF1 cells were kindly provided by the Stem Cell Bank, Chinese Academy of Sciences (Beijing, China). The cell-growth medium consisted of DMEM and 10% fetal bovine serum (both Thermo Fisher Scientific). The five samples were first placed on a 24-well plate, and then 1 mL cell suspensions with a density of 5×104/mL were added. They were statically cultured for 1 day at 37°C in a humidified atmosphere filled with 5% CO2. The next day, we removed the stale culture medium and added 1 mL fresh medium, including 10% CCK-8, into each well for incubation of 2 hours. Lastly, we transferred 100 μL of the medium from the 24-well plate to a new 96-well plate, and absorbance was detected by a microplate reader at 450 nm wavelength.
Qualitative assessment of adherent HFF1 cells by fluorescence microscopy
To evaluate cell morphology and adhesion and spreading behaviors, the HFF1 cells were seeded on various samples at a density of 5×104 cells/mL, incubated for 24 hours at 37°C, and then gently washed twice with fresh PBS, fixed with 4% formaldehyde for 30 minutes, permeabilized with PBS containing 0.1% Triton X-100, and finally stained with tetraethyl rhodamine isothiocyanate–phalloidin and 4′,6-diamidino-2-phenylindole. Cell nuclei and cytoskeletal actin were observed by fluorescence microscopy (Leica Microsystems, Wetzlar, Germany).
In vitro antibacterial assay
Gram-positive MRSA (biofilm-positive; American Type Culture Collection 43300) and Gram-negative P. aeruginosa (biofilm-positive; American Type Culture Collection 27853) were used to determine the antibacterial property of the glass, V0, V3, V4, and V5 samples. The two representative strains were first cultured in fresh TSB at 37°C overnight, and then (based on McFarland standards) their inoculation concentrations were both adjusted to 106 CFU/mL in TSB. Each specimen was placed into a sterile 24-well plate, and 500 μL of prepared bacterial suspension was added to the wells. The 24-well plate was then kept in an incubator statically at 37°C for 18 hours. At the end, we dealt with the original medium with planktonic bacteria through serial dilution, and viable swimming bacteria were counted by the spread-plate method (SPM). In addition, we used sterile tweezers to take out the samples from the suspension and gently dislodged the nonadherent bacteria with fresh PBS twice. Then, each sample was put in a sterile test tube that included 1 mL fresh PBS and oscillated by ultrasonic vibration for 15 minutes (150 W; B3500S-MT; Emerson, St Louis, MO, USA) and vortex mixing (Vortex Genie 2; Scientific Industries, Bohemia, NY, USA) for 1 minute. A bacterial suspension with adhered bacteria was acquired and viable bacteria enumerated by the SPM. In line with the National Standard of China GB/T 4789.2 protocol, CFU amounts on sheep-blood agar (SBA) were enumerated.
Antimicrobial rates for planktonic and sessile bacteria were computed on the basis of the following formulae:
where b is the average number of living bacteria in TSB medium with glass, V0, V3, V4, or V5, a mean viable cells in TSB inoculated with glass, d average number of living bacteria on the surface of glass, V0, V3, V4, or V5, and c mean viable cells on the surface of glass.
Qualitative evaluation of planktonic bacteria by improved SPM
The antibacterial efficiency of our samples against plank-tonic bacteria was also qualitatively assessed by improved SPM, ie, 100 μL of diluent bacterial suspension was evenly spread on the fresh SBA and cultivated overnight at 37°C. Representative photos were obtained.
Qualitative assessment of adherent bacteria by SEM
Incubated specimens were gently washed with fresh PBS three times to remove nonadherent bacteria, fixed with 2.5% glutaraldehyde overnight at 4°C in a freezer, and subsequently dehydrated in the ethanol series 30, 50, 70, 80, 90, and 95 v/v% for 10 minutes and in absolute ethanol solution (twice for 10 minutes). Then, our samples were treated with desiccation and sprayed gold, and finally examined by SEM (JSM-6310LV; JEOL, Tokyo, Japan).
Qualitative assessment of adherent bacteria by CLSM
Surfaces of incubated specimens were gently washed with sterile PBS three times to dislodge nonadherent bacteria. Then, these samples were placed into a new 24-well plate, stained with 500 μL of mixed dye (BacLight live/dead bacteria-viability kit; Thermo Fisher Scientific) in the dark for 20 minutes. Lastly, the samples were lightly rinsed with fresh PBS and subsequently observed by confocal laser-scanning microscopy (CLSM; 510 Meta; Carl Zeiss Meditec AG, Jena, Germany). Cells stained with green fluorescent dye represented viable bacteria, and those with red fluorescent dye were dead bacteria.
Intracellular reactive oxygen species assay
Oxidative stress levels in bacteria upon exposure to nano-particle films were estimated by an intracellular reactive oxygen species (ROS) assay kit (Beyotime) using 2′,7′-dichlorodihydrofluorescein diacetate (DCFH-DA) as a fluorescent probe.Citation30 All procedures followed the manufacturer’s instructions strictly. In brief, after overnight culture, the bacteria were gently rinsed with PBS twice. Then, the DCFH-DA was diluted into a final concentration of 10 μM staining solution using PBS. Subsequently, the DCFH-DA staining solution was added to each well sample, followed by 20 minutes of incubation at 37°C, and then observed immediately on CLSM at excitation/emission wavelengths of 488/535 nm. Laser-beam intensity and photodetector sensitivity were kept constant to compare the relative fluorescence intensities among various groups.
Antioxidant-incubation study
We intended to investigate whether oxidative stress plays a role in bacterial response to the present nanoparticle films. Therefore, according to a previous strategy,Citation20 we coincubated S. aureus and P. aeruginosa in TSB with 10 mM glutathione (GSH; reduced form), the primary endogenous antioxidant, to neutralize the function of oxidative stress when bacteria were exposed to our nanosamples. The bacterial viability of swimming and adhered cells was quantitatively assessed through using the aforementioned methods. Moreover, SEM and CLSM were also used to observe the morphology and viability of adherent bacteria in TSB and GSH media.
Hemolysis assays
Hemolysis assays were completed based on previous literature.Citation20 Our experiments were approved by the ethics review committee of Shanghai Jiao Tong University Sixth People’s Hospital. Volunteers whose blood was used provided informed consent to donate the blood. Red blood cells (RBCs) were isolated from the venous blood of healthy young volunteers, washed with sterile PBS three times, and finally resuspended in PBS at 2% hematocrit. In order to examine the effect of VOx nanoparticle films on erythrocyte lysis, RBCs were incubated with or without bacteria (S. aureus and P. aeruginosa), followed by treatment with various samples for 24 hours at 37°C. The final concentration of bacterial suspension in the bacteria–biomaterial coculture system was 106 CFU/mL. Aseptic distilled water was used as a positive control. At the end of incubation, one aliquot of reaction mixture was withdrawn and centrifuged at 1,200 g for 5 minutes. Supernatants were acquired and hemolysis determined by measuring their absorbance at 540 nm. The percentage of hemolysis was calculated thus:
where A was the absorbance of our samples and B the absorbance of the positive control.
Statistical analysis
Statistically significant differences between various groups were measured using one-way analysis of variance and Tukey’s multiple-comparison tests on GraphPad Prism 5 statistical software. All data are expressed as means ± standard deviation. Values of P<0.05 were considered statistically significant.
Results and discussion
Sample characterization
shows the surface morphologies of various samples. At room-temperature sputtering using the V-metal target, homogeneous nanoparticle film appeared on the substrate surface (). Similarly, using the V2O3 target, a uniform film of nanoparticle topography emerged on the surface after magnetron sputtering (). This was also the same for VO2-target sputtering, as seen in . For V2O5 preparation, predeposited VO2 film underwent subsequent annealing and produced the observed surface-nanoparticle topography in , indicating the development of nanoparticles due to phase evolution.
Figure 1 SEM morphology of various surfaces of samples V0 (A), V3 (B), V4 (C), and V5 (D).
Abbreviations: SEM, scanning electron microscopy; V0, homogeneous vanadium metal nanoparticles deposited on quartz glass; V3, homogeneous V2O3 nanoparticles deposited on quartz glass; V4, homogeneous VO2 nanoparticles deposited on quartz glass; V5, homogeneous V2O5 nanoparticles deposited on quartz glass.
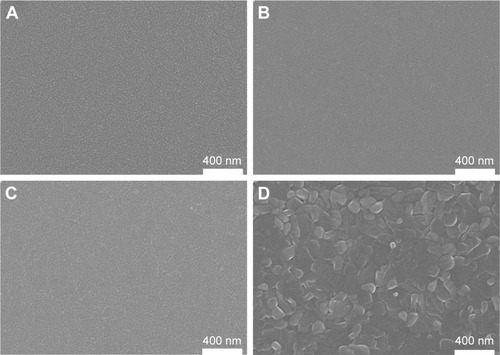
gives the XRD patterns of various samples. For quartz glass (substrate), only an amorphous peak was detected. For sample V0, the diffraction peak of the (111) plane was detected for the V-metal phase. For sample V3, the diffraction peaks of the (110), (113), and (116) planes were determined for the V2O3 phase. For sample V4, the peaks of the (011) and (220) planes were observed for the VO2 phase. As for sample V5, the characteristic peaks of the (010), (110), (120), and (320) planes were detected for the V2O5 phase. shows the V2p–O1s XPS spectra acquired from various surfaces. A binding energy of around 530.4 eV was found for the O1s peak in vanadium oxides. For sample V0, as seen in , two doublet peaks appeared for V2p. Doublet peaks at 512.9 eV and 520.7 eV corresponded to V2p3/2 and V2p1/2 in V metal.Citation31 Other doublet peaks at 515.7 eV and 523.4 eV belonged to V2p3/2 and V2p1/2 in V2O3,Citation32 indicating the partial natural oxidation of the V-metal nanoparticle surface (4V +3O2 → 2V2O3). As for sample V3 (), doublet peaks at 515.6 eV and 523.1 eV belonged to V2p3/2 and V2p1/2 in V2O3. With regard to sample V4 (), doublet peaks at 516.3 eV and 523.5 eV were assigned to V2p3/2 and V2p1/2 in VO2.Citation33 With regard to sample V5, as shown in , doublet peaks at 517.6 eV and 524.8 eV belonged to V2p3/2 and V2p1/2 in V2O5.Citation34 Overall, the XPS and XRD data were quite consistent.
Figure 2 XRD patterns (A) of samples V0, V3, V4, and V5, accompanied by the V2p XPS spectra of samples V0 (B), V3 (C), V4 (D), and V5 (E).
Abbreviations: XRD, X-ray diffraction; XPS, X-ray photoelectron spectroscopy; V0, homogeneous vanadium metal nanoparticles deposited on quartz glass; V3, homogeneous V2O3 nanoparticles deposited on quartz glass; V4, homogeneous VO2 nanoparticles deposited on quartz glass; V5, homogeneous V2O5 nanoparticles deposited on quartz glass.
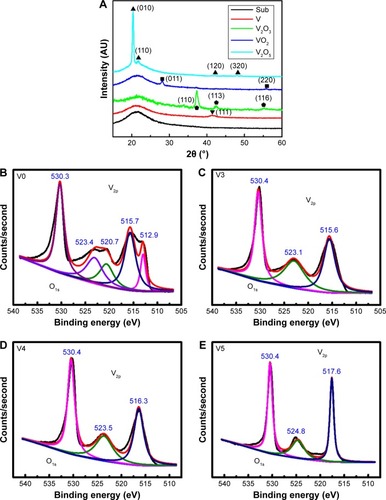
Vanadium-ion release
shows the release profiles of vanadium ions in DMEM, TSB, and PBS media after immersion for 1, 4, 7, and 14 days. With DMEM (), vanadium ions were continuously released from various samples, especially for sample V0. This was also true for vanadium release from samples V3, V4, and V5 in TSB () and PBS ().
Figure 3 Release profiles of vanadium ions from various samples after soaking in DMEM (A), TSB (B), and PBS (C).
Abbreviations: DMEM, Dulbecco’s Modified Eagle’s Medium; TSB, trypticase soy broth; PBS, phosphate-buffered saline; V0, homogeneous vanadium metal nanoparticles deposited on quartz glass; V3, homogeneous V2O3 nanoparticles deposited on quartz glass; V4, homogeneous VO2 nanoparticles deposited on quartz glass; V5, homogeneous V2O5 nanoparticles deposited on quartz glass.
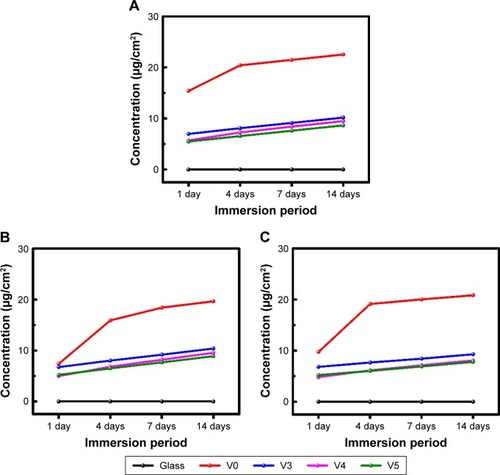
Biocompatibility
Normally, indwelling medical devices holding promise for future clinical transformation should possess good biocompatibility. At first, we used human HFF1 fibroblasts to evaluate the biocompatibility of glass, V0, V3, V4, and V5 samples. As seen in , after 1 day of culture on various samples, cell viability in V0/V3/V4 and glass showed no significant difference, while V5 produced an obvious difference compared with glass. V3/V4/V5 also showed significant differences in comparison to V0. Vanadium has six chemical valence states, and vanadium cytotoxicity ascends with valence states.Citation23 This may account for V3/V4/V5 with relatively low vanadium-release amounts producing more toxic effects on HFF1 compared with V0 with relatively high vanadium-release amounts. Furthermore, as seen in , cell adhesion and the cytoskeleton were also fluorescently stained to visualize the HFF1 state. In detail, the HFF1 cells adhered and spread well on glass and V0 surfaces. This was also similar for samples V3 and V4. By contrast, the adhesion and spreading of HFF1 cells on V5 were obviously inhibited. Overall, this revealed a valence-state-dependent trend.
Figure 4 Proliferation viability of HFF1 cells after 24-hour culture on the glass, V0, V3, V4, and V5 samples.
Notes: ***P<0.001 vs glass; #P<0.05; ###P<0.001 vs V0; $$$P<0.001 vs V3; &&&P<0.001 vs V4. Data expressed as means ± standard deviation (n=3). DAPI (blue)- and TRITC-phalloidin (red)-stained fluorescent images of HFF1 cells on the five specimens after 1-day culture.
Abbreviations: V0, homogeneous vanadium metal nanoparticles deposited on quartz glass; V3, homogeneous V2O3 nanoparticles deposited on quartz glass; V4, homogeneous VO2 nanoparticles deposited on quartz glass; V5, homogeneous V2O5 nanoparticles deposited on quartz glass; DAPI, 4′,6-diamidino-2-phenylindole; TRITC, tetraethyl rhodamine isothiocyanate.
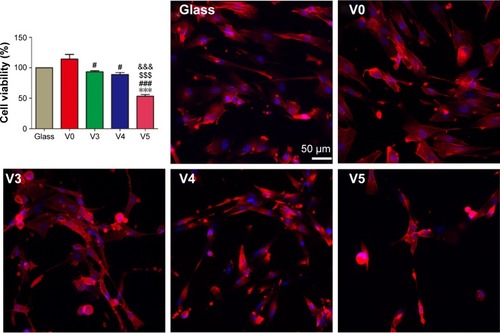
Antibacterial activity
To investigate the antimicrobial behavior and mechanism of the vanadium-nanoparticle films, we adopted a comparative study method of using GSH antioxidant to evaluate antibacterial ability, with reference to in vitro antibacterial tests in previous studies,Citation20,Citation35 which usually focused on exploring the effects that the materials exerted on planktonic and sessile bacteria. After 24 hours of culture on glass, V0, V3, V4, and V5 with/without GSH coincubation, the planktonic bacteria (MRSA and P. aeruginosa) were firstly collected and recultivated on SBA overnight. Representative images are given in . Combined with the corresponding counting results in , it can be seen that for Gram-positive MRSA, the V, V2O3, VO2, and V2O5 films exhibited significant antibacterial activity toward MRSA in the order of V0, V3, V4, and V5, with obvious differences among groups, showing the close correlation with the valence states of vanadium (). Interestingly, for Gram-negative P. aeruginosa, the trend was not completely the same. As seen in , V0 showed good antibacterial ability against P. aeruginosa, and this may have resulted from the natural oxidation of V metal upon air exposure to form the V2O3 phase on the outermost surface. Sample V3 presented stronger antibacterial activity than V0. However, sample V4 did not exhibit antibacterial properties, which was consistent with our recent work.Citation20 Compared with V0 and V3, sample V5 revealed more effective antibacterial capacity on P. aeruginosa. As a result, the antiplanktonic bacterial ability of the various samples had a relationship with the vanadium-oxidation states, and this is further discussed in the next section.
Figure 5 Qualitative assessment of antiseptic capacity against two types of planktonic bacteria.
Notes: (A) For Staphylococcus aureus, representative pictures of recultivated bacteria colonies on SBA for five samples in the TSB or TSB + GSH culture medium; (B) for Pseudomonas aeruginosa, representative pictures of recultivated bacteria colonies on SBA for five samples in the TSB or TSB + GSH culture medium.
Abbreviations: SBA, sheep-blood agar; TSB, trypticase soy broth; V0, homogeneous vanadium metal nanoparticles deposited on quartz glass; V3, homogeneous V2O3 nanoparticles deposited on quartz glass; V4, homogeneous VO2 nanoparticles deposited on quartz glass; V5, homogeneous V2O5 nanoparticles deposited on quartz glass.
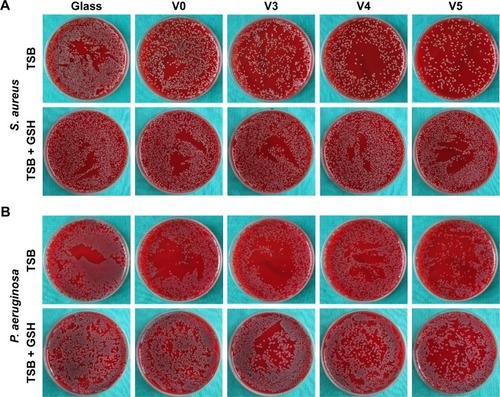
Figure 6 Quantitative evaluation for antiseptic property against planktonic bacteria.
Notes: (A) Antibacterial rates of planktonic Gram-positive Staphylococcus aureus for the five specimens in TSB medium; (B) antibacterial rates of planktonic Gram-negative Pseudomonas aeruginosa for the five specimens in TSB medium; (C) antibacterial rates of planktonic Gram-positive S. aureus for the five specimens in TSB + GSH medium; (D) antibacterial rates of planktonic Gram-negative P. aeruginosa for the five specimens in TSB + GSH medium. **P<0.01, ***P<0.001 vs glass; #P<0.05, ###P<0.001 vs V0; $P<0.05, $$P<0.01, $$$P<0.001 vs V3; &&P<0.01, &&&P<0.001 vs V4. Data expressed as means ± standard deviation (n=3).
Abbreviations: V0, homogeneous vanadium metal nanoparticles deposited on quartz glass; V3, homogeneous V2O3 nanoparticles deposited on quartz glass; V4, homogeneous VO2 nanoparticles deposited on quartz glass; V5, homogeneous V2O5 nanoparticles deposited on quartz glass; Rap, antibacterial rates of planktonic bacteria; TSB, trypticase soy broth; GSH, glutathione.
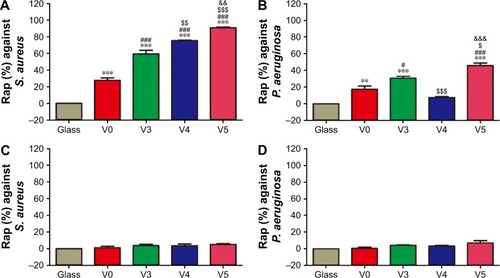
Furthermore, from oxidation and reduction chemistry, we tried to explore the role of vanadium-valence states in oxidative stress induction using GSH coincubation. As seen in , after coincubation with GSH antioxidant, no visible difference in planktonic bacteria-colony formation was observed among groups. Combined with the corresponding quantitative data in , there were no significant differences among the bacteria-counting results of various groups, indicating that GSH eliminated the antibacterial capability of V0, V3, V4 and V5 against planktonic MRSA or P. aeruginosa. This sharp contrast further implied that these samples should exert antibacterial activity by inducing oxidative stress and oxidative damage. In addition, overall, the V0, V3, and V5 samples possessed relatively stronger antibacterial activity on MRSA than on P. aeruginosa. This can be attributed to the higher tolerance of P. aeruginosa toward oxidative stress.Citation20 It should also be noted that antibacterial efficiency of around 20% is actually low for combating bacteria.
Likewise, we detached the adherent bacteria (MRSA and P. aeruginosa) from sample surfaces and then recultivated them on the SBA overnight. The counting results of bacteria colonies are given in . As seen in , the antiadherent bacterial activity of various samples showed a similar trend to the antiplanktonic bacterial ability seen in . Similarly, coincubation with GSH antioxidant also removed the antiadherent bacterial ability of the various samples, as evidenced in . This further confirmed that these samples also imposed antiadherent bacterial ability by triggering oxidative stress and oxidative damage.
Figure 7 Quantitative evaluation for antiseptic property against sessile bacteria.
Notes: (A) Antimicrobial rates of adherent Gram-positive Staphylococcus aureus for the five samples in TSB medium; (B) antimicrobial rates of adherent Gram-negative Pseudomonas aeruginosa for the five samples in TSB medium; (C) antimicrobial rates of adherent Gram-positive S. aureus for the five samples in TSB + GSH medium; (D) antimicrobial rates of adherent Gram-negative P. aeruginosa for the five samples in TSB + GSH medium. **P<0.01, ***P<0.001 vs glass; #P<0.05, ###P<0.001 vs V0; $$P<0.01, $$$P<0.001 vs V3; &P<0.05, &&&P<0.001 vs V4. Data expressed as means ± standard deviation (n=3).
Abbreviations: V0, homogeneous vanadium metal nanoparticles deposited on quartz glass; V3, homogeneous V2O3 nanoparticles deposited on quartz glass; V4, homogeneous VO2 nanoparticles deposited on quartz glass; V5, homogeneous V2O5 nanoparticles deposited on quartz glass; Raa, antibacterial rates of adhered bacteria; TSB, trypticase soy broth; GSH, glutathione.
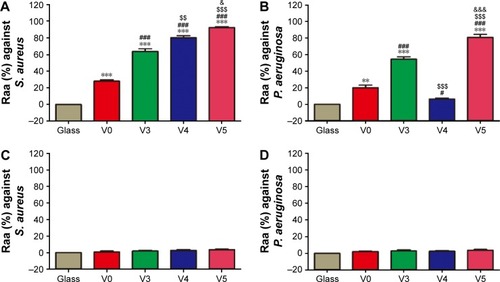
As a kind of visual qualitative evaluation method, SEM was also performed to investigate the adherent bacteria state and morphology. The results are shown in . As seen in , counts of adherent MRSA were regularly reduced on various samples in the order of V0, V3, V4, and V5 compared with glass, especially for samples V3, V4, and V5, whereas after GSH coincubation, all samples promoted bacterial adhesion and proliferation on the surface, showing no obvious differences among groups. This was accordant with the aforementioned quantitative results (). Likewise, for adherent P. aeruginosa, the SEM results in also agreed well with bacteria-counting results (), especially for V3 and V5. To visualize the bacterial viability and membrane integrity further, live/dead fluorescence staining was conducted on both adherent bacteria, as shown in . For both MRSA () and P. aeruginosa (), fluorescence results were quite consistent with the aforementioned qualitative and quantitative results. Overall, V3, V4, and V5 possessed good antibacterial activity on Gram-positive MRSA, and V3 and V5 had favorable antibacterial ability on Gram-negative P. aeruginosa.
Figure 8 Qualitative assessment of antimicrobial capacity against adherent bacteria by SEM.
Notes: (A) For Gram-positive Staphylococcus aureus, bacterial morphology and biofilm formation on the five samples in TSB (upper) or TSB + GSH (lower) medium; (B) for Gram-negative Pseudomonas aeruginosa, bacterial morphology and biofilm formation on the five samples in TSB (upper) or TSB + GSH (lower) medium. Magnification ×2,000.
Abbreviations: SEM, scanning electron microscopy; V0, homogeneous vanadium metal nanoparticles deposited on quartz glass; V3, homogeneous V2O3 nanoparticles deposited on quartz glass; V4, homogeneous VO2 nanoparticles deposited on quartz glass; V5, homogeneous V2O5 nanoparticles deposited on quartz glass; TSB, trypticase soy broth; GSH, glutathione.
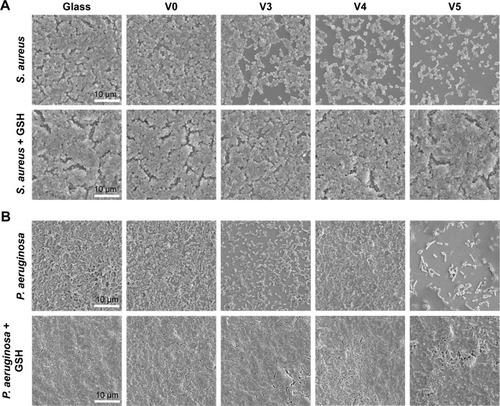
Figure 9 Qualitative assessment of antiseptic property against adherent bacteria by CLSM fluorescence.
Notes: (A) For Gram-positive Staphylococcus aureus, biofilm formation illustrated by live/dead staining results on the five samples in TSB medium with or without GSH antioxidant; (B) for Gram-negative Pseudomonas aeruginosa, biofilm formation illustrated by live/dead staining results on the five samples in TSB medium with or without GSH antioxidant. Magnification ×200.
Abbreviations: CLSM, confocal laser-scanning microscopy; V0, homogeneous vanadium metal nanoparticles deposited on quartz glass; V3, homogeneous V2O3 nanoparticles deposited on quartz glass; V4, homogeneous VO2 nanoparticles deposited on quartz glass; V5, homogeneous V2O5 nanoparticles deposited on quartz glass; TSB, trypticase soy broth; GSH, glutathione.
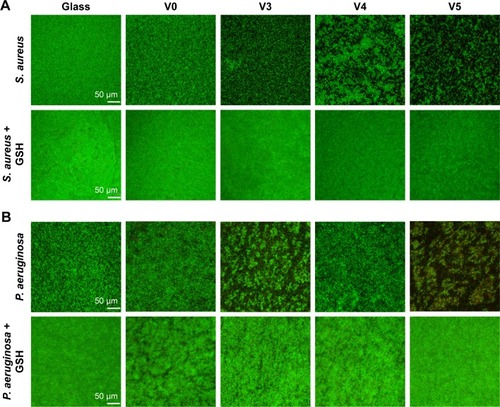
Plausible antimicrobial mechanism
Herein, we tried to understand the possible antibacterial mechanism of the various samples: V0, V3, V4 and V5. For sample, V0 (V-metal nanoparticle film), as evidenced by XPS analysis, natural oxidation occurred on the outermost surface of V-metal nanoparticles (4V +3O2 → 2V2O3). The observed antibacterial ability with relatively low efficiency can be attributed to the generation of the V2O3 phase on the surface. With regard to sample V3 (V2O3 nanoparticle film), since V2O3 is a strong reductant, it may allow electronic charge flowing between V2O3 nanoparticles and adherent bacteria,Citation36,Citation37 which thus disrupts the membrane electron transfer and causes oxidative stress and damage to adherent bacteria. For planktonic bacteria, V2O3 holds the potential to react gradually with dissolved oxygen in aqueous solution to form VO2 (2V2O3 + O2 → 4VO2). The intermediate state of VO2 can gradually oxidize to V2O5 (4VO2 + O2 → 2V2O5), followed by formation of a hydrated surface (V2O5 +3H2O → 2H3VO4).Citation38 In a slightly alkaline medium (blood), the following reaction occurs: H3VO4 + OH− → H2VO4− + H2O.Citation23,Citation39,Citation40 The H2VO4− anions will gradually enter the aqueous solution and account for the observed antiplanktonic bacteria activity. As for sample V4 (VO2 nanoparticle film), on one hand, for adherent bacteria, intermediate VO2 exhibited good antibacterial ability on MRSA, while it did not exert this antibacterial effect on P. aeruginosa, since it has high tolerance for oxidative stress or ROS level.Citation20 On the other hand, for planktonic bacteria, as analyzed herein, the intermediate state VO2 will gradually oxidize (4VO2 + O2 → 2V2O5), hydrate (V2O5 + 3H2O → 2H3VO4), and form vanadate anions (H3VO4 + OH− → H2VO4− + H2O) in slightly alkaline media. The formed vanadate ions can be released from the surface and lead to the antibacterial effect on planktonic MRSA, while similarly they had no obvious antibacterial activity on planktonic P. aeruginosa.
In regard to sample V5 (V2O5 nanoparticle film), V2O5 is a strong oxidant and may be able to extract electrons from the bacteria membrane and disturb the electron-transport chain, thus causing oxidative damage to adherent bacteria.Citation36,Citation37 It has been reported that the redox activity of V2O5 can be used as a biosensor to detect biological macromolecules in which V2O5 acts as the charge-exchanging catalyst to complete the oxidation–reduction cycle.Citation41 As for the planktonic bacteria, as discussed earlier, the +5-valence vanadate anions (H2VO4−) can speciate from the surface (V2O5 +3H2O → 2H3VO4; H3VO4 + OH− → H2VO4− + H2O) and then enter aqueous solution, finally imposing an antibacterial effect on MRSA and even on P. aeruginosa. For the antibacterial difference in samples V5 and V4 against planktonic P. aeruginosa, this may have been due to the difference in the availability of H2VO4− speciating from V2O5 and VO2 in the slightly alkaline medium. Nevertheless, obviously further work needs to be done to explore this.
Bacteria can carry out respiratory processes to produce power for various life movements.Citation42,Citation43 Electrons are provided by low-redox potential donors (eg, NADH, NADPH) and then transferred in the membrane electron-transport chain by a series of redox cofactors, finally to terminal electron acceptors, such as O2 molecules.Citation44,Citation45 The electron-transfer chain is the main site for intracellular ROS production.Citation46 In normal physiological conditions, bacteria can self-regulate to maintain intracellular redox hemostasis through an antioxidant defense system. However, upon exposure to extraneous toxicants, the intracellular redox homeostasis may be disrupted, due to the elevation of the intracellular ROS level caused by the toxicant-induced dysfunction of the membrane electron-transport chain. Here, vanadium exposure holds the high risk of inducing intracellular ROS production elevation in bacteria, because the high redox activity of vanadium species has the potential to interfere with the redox cofactors (eg, NADP+/NADPH, FAD2+/FADH2, FMN2+/FMNH2) in the bacterial electron-transfer chain.Citation17,Citation47 As reported, disruption to the membrane electron-transport chain will cause toxicity to bacteria.Citation18,Citation48 This is similar to the vanadium-induced toxicity on cellular mitochondria.Citation46,Citation49
As discussed earlier, the exposure of bacteria to V metal, V2O3, VO2, and V2O5 nanofilms may elevate intracellular ROS levels and thus cause oxidative stress and damage. To verify this, we further used DCFH-DA fluorescence dye to visualize intracellular ROS levels, which can be indicated by green fluorescence. As seen in , for Gram-positive MRSA, after vanadium exposure, compared with sample glass intracellular ROS levels were sharply elevated in the order of V0, V3, V4, and V5, especially for samples V4 and V5. By comparison, the Gram-negative P. aeruginosa were not as sensitive to vanadium exposure as Gram-positive MRSA. As a result, intracellular ROS levels showed no obvious increase on samples V0, V3, and V4 compared with glass, but sample V5 induced a relatively apparent elevation in intracellular ROS production in P. aeruginosa. Overall, the ROS staining results were quite consistent with these data and analyses. GSH is an important antioxidant for living cells,Citation50 and maintains intracellular redox homeostasis by scavenging intracellular ROS.Citation51,Citation52 As evidenced here, coincubation of bacteria with GSH antioxidant can apparently alleviate or even eliminate the antibacterial activity of various VOx nanoparticle films, further supporting their oxidative stress-mediated antibacterial effect.
Figure 10 Intracellular ROS levels of Staphylococcus aureus and Pseudomonas aeruginosa on the samples after culturing overnight.
Note: Magnification ×200.
Abbreviations: ROS, reactive oxygen species; V0, homogeneous vanadium metal nanoparticles deposited on quartz glass; V3, homogeneous V2O3 nanoparticles deposited on quartz glass; V4, homogeneous VO2 nanoparticles deposited on quartz glass; V5, homogeneous V2O5 nanoparticles deposited on quartz glass.
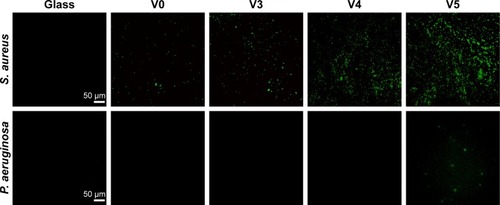
Erythrocyte-assisting property
Considering that we intended to use the vanadium-nanoparticle films to resist implant-associated BSI and human erythrocytes do not have mitochondria, we supposed that when coculturing erythrocytes and bacteria on VOx nanoparticle films, VOx might help erythrocytes fight against bacteria to reduce the risk of hemolysis caused by bacteria exposure. As seen in , without adding bacteria, various samples induced relatively low hemolysis rates (less than 2%) in a relatively safe range. By contrast, after Gram-positive MRSA exposure, the glass sample produced a relatively high hemolysis rate of over 60% (). By comparison, V0, V3, V4, and V5 produced apparently decreased hemolysis rates of around 20%, especially for sample V5. At the same time, the erythrocytes were also exposed to the Gram-negative P. aeruginosa. Corresponding hemolysis rates are given in . From this, it can be seen that only V5 had an obvious assistance effect on inhibiting bacteria-induced hemolysis. Basically, the obtained results were quite accordant with the antibacterial efficiency of the designed VOx nanoparticle films, together demonstrating the erythrocyte-assisting but bacteria-combating dual functions. The whole interaction process is illustrated in .
Figure 11 Hemolysis rate of red blood cells after 24 hours of incubation on the five specimens.
Notes: (A) Erythrocytes were incubated on the five specimens without bacteria; (B) erythrocytes were incubated on the five specimens with Gram-positive Staphylococcus aureus; (C) erythrocytes were incubated on the five specimens with Gram-negative Pseudomonas aeruginosa; (D) interactions of erythrocytes and bacteria upon coexistence in the presence/absence of VOx films. *P<0.05, ***P<0.001 vs Glass; #P<0.05 vs V0. Data expressed as means ± standard deviation (n=3).
Abbreviations: V0, homogeneous vanadium metal nanoparticles deposited on quartz glass; V3, homogeneous V2O3 nanoparticles deposited on quartz glass; V4, homogeneous VO2 nanoparticles deposited on quartz glass; V5, homogeneous V2O5 nanoparticles deposited on quartz glass; VOx, vanadium of various valences.
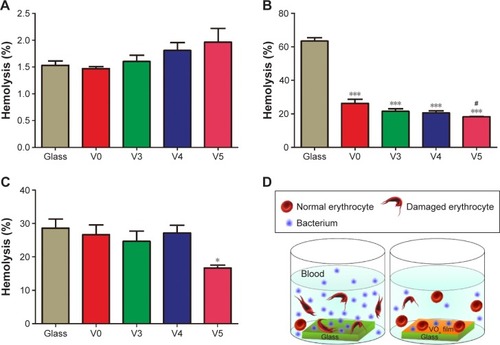
Scheme 1 Illustration of the fabrication process of samples V0, V3, V4, and V5 by magnetron sputtering.
Abbreviations: RT, room temperature; V0, homogeneous vanadium metal nanoparticles deposited on quartz glass; V3, homogeneous V2O3 nanoparticles deposited on quartz glass; V4, homogeneous VO2 nanoparticles deposited on quartz glass; V5, homogeneous V2O5 nanoparticles deposited on quartz glass.
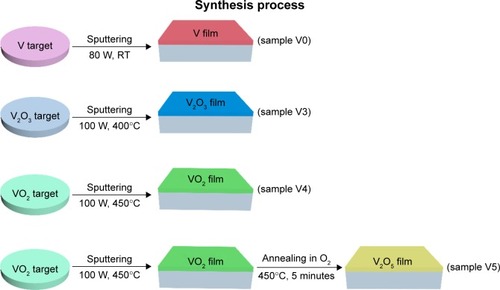
Vanadium compounds end up in the human bloodstream.Citation53 The major transporter for both vanadate anions (H2VO4−) and vanadyl cations (VO2+) is transferrin. In addition, H2VO4− is easily soluble and also exists as a free anion in blood (ie, unbound to transferrin).Citation54 From the bloodstream, vanadium will be distributed to body tissues and bones. Actually, vanadium is presumably one of the essential trace elements in the human body.Citation22 Bones can serve as a storage pool for vanadate. Vanadium holds the potential to promote soft tissue–implant integrationCitation55 and participate in the bone-mineralization process.Citation56 These can control the potential toxicity of vanadium on normal cells if delivered in an appropriate dosage for safety. In addition, bacteria in the human bloodstream require free iron to produce lipopolysaccharide endotoxins and cause inflammation.Citation57 The inhibition of hemolysis can prevent bacteria from acquiring iron from erythrocytes in bloodstream. Basically, the designed VOx nanoparticle films can exert favorable anti-infection function that assists erythrocytes to combat bacteria in the human bloodstream, which from the perspective of surface modification and functionalization may contribute to the BSI prophylaxis of biomedical devices, such as implants, stents, and catheters.
Conclusion
In the present study, we firstly deposited V metal, V2O3, VO2 and V2O5 nanofilms on quartz glass by magnetron sputtering. These nano-VOx films were homogeneous and released vanadium ions in a continuous fashion. It was found that low-valence VOx films had better cytocompatibility. Furthermore, these VOx nanofilms possessed excellent antibacterial ability against MRSA and P. aeruginosa, especially for MRSA, and this antibacterial effect increased with higher vanadium chemical valence. Moreover, the VOx films effectively inhibited the hemolysis of human erythrocytes caused by bacteria in a novel coculture model. As a result, they imposed favorable anti-infection effects that assisted erythrocytes to combat bacteria in the human bloodstream. We hope this work can provide new insights for biomedical applications of inorganic vanadium compounds and attract growing interest in this area. From the viewpoint of surface modification, this work may be conducive to the prophylaxis of BSI related to biomedical devices, such as catheters and stents.
Acknowledgments
This work was jointly supported by the National Natural Science Foundation of China (grants 81271962, 81472109, 81472108, and 81301571), the high-tech project of MOST (2014AA032802), and the Science and Technology Commission of Shanghai Municipality (STCSM; 14DZ2261203).
Disclosure
The authors report no conflicts of interest in this work.
References
- CaseyALMermelLANightingalePElliottTSAntimicrobial central venous catheters in adults: a systematic review and meta-analysisLancet Infect Dis200881276377619022192
- SendiPBanderetFGraberPZimmerliWPeriprosthetic joint infection following Staphylococcus aureus bacteremiaJ Infect2011631172221663971
- BoucherHWChallenges in anti-infective development in the era of bad bugs, no drugs: a regulatory perspective using the example of bloodstream infection as an indicationClin Infect Dis201050Suppl 1S4S920067391
- ViolaGMRosenblattJRaadIIDrug eluting antimicrobial vascular catheters: progress and promiseAdv Drug Deliv Rev Epub201682
- CassALKellyJWProbstJCAddyCLMcKeownREIdentification of device-associated infections utilizing administrative dataAm J Infect Control201341121195119923768437
- LauplandKBLyytikäinenOSøgaardMThe changing epidemiology of Staphylococcus aureus bloodstream infection: a multinational population-based surveillance studyClin Microbiol Infect201319546547122616816
- LimCJChengACKongDCPelegAYCommunity-onset bloodstream infection with multidrug-resistant organisms: a matched case-control studyBMC Infect Dis20141412624592979
- MicekSTLloydAERitchieDJReichleyRMFraserVJKollefMHPseudomonas aeruginosa bloodstream infection: importance of appropriate initial antimicrobial treatmentAntimicrob Agents Chemother20054941306131115793102
- DonlanRMBiofilm elimination on intravascular catheters: important considerations for the infectious disease practitionerClin Infect Dis20115281038104521460321
- WuKYangYZhangYDengJLinCAntimicrobial activity and cytocompatibility of silver nanoparticles coated catheters via a biomimetic surface functionalization strategyInt J Nanomedicine2015107241725226664115
- VallésJRelloJOchagavíaAGarnachoJAlcaláMACommunity-acquired bloodstream infection in critically ill adult patients: impact of shock and inappropriate antibiotic therapy on survivalChest200312351615162412740282
- ZazoHColinoCILanaoJMCurrent applications of nanoparticles in infectious diseasesJ Control Release20162248610226772877
- HuhAJKwonYJ“Nanoantibiotics”: a new paradigm for treating infectious diseases using nanomaterials in the antibiotics resistant eraJ Control Release2011156212814521763369
- TaylorEWebsterTJReducing infections through nanotechnology and nanoparticlesInt J Nanomedicine201161463147321796248
- SoaresSSMartinsHDuarteROVanadium distribution, lipid peroxidation and oxidative stress markers upon decavanadate in vivo administrationJ Inorg Biochem20071011808817030392
- SoaresSSGutieŕrez-MerinoCAurelianoMMitochondria as a target for decavanadate toxicity in Sparus aurata heartAquat Toxicol20078311917420061
- AurelianoMDecavanadate: a journey in a search of a roleDalton Trans2009429093910020449180
- LiJWangGZhuHAntibacterial activity of large-area monolayer graphene film manipulated by charge transferSci Rep20144435924619247
- MaiaPIPavanFRLeiteCQVanadium complexes with thiosemicarbazones: synthesis, characterization, crystal structures and anti-Mycobacterium tuberculosis activityPolyhedron2009282398406
- LiJZhouHWangJOxidative stress-mediated selective antimicrobial ability of nano-VO2 against Gram-positive bacteria for environmental and biomedical applicationsNanoscale2016823119071192327240639
- GajeraSBMehtaJVPatelMNMetal-based biologically active compounds: design, synthesis, medicinal, toxicity and DNA interaction assayMed Chem Res2016253526537
- MichibataHVanadium: Biochemical and Molecular Biological ApproachesHeidelbergSpringer2011
- CransDCSmeeJJGaidamauskasEYangLThe chemistry and biochemistry of vanadium and the biological activities exerted by vanadium compoundsChem Rev2004104284990214871144
- ChadwickWMaudsleySThe devil is in the dose: complexity of receptor systems and responsesMattsonMPCalabreseEJHormesis: A Revolution in Biology, Toxicology and MedicineHeidelbergSpringer201095108
- GresserMTraceyAVanadates as phosphate analogs in biochemistryChasteenNDVanadium in Biological Systems: Physiology and BiochemistryDordrecht, NetherlandsKluwer19906379
- ZhangYYangXDWangKCransDCThe permeability and cytotoxicity of insulin-mimetic vanadium (III, IV, V)-dipicolinate complexesJ Inorg Biochem20061001808716321441
- CapellaLSGeféMRSilvaEFMechanisms of vanadate-induced cellular toxicity: role of cellular glutathione and NADPHArch Biochem Biophys20024061657212234491
- WangRBraughtonKRKretschmerDIdentification of novel cytolytic peptides as key virulence determinants for community-associated MRSANat Med200713121510151417994102
- SoongGChunJParkerDPrinceAStaphylococcus aureus activation of caspase 1/calpain signaling mediates invasion through human keratinocytesJ Infect Dis2012205101571157922457275
- EstebanMAWangTQinBVitamin C enhances the generation of mouse and human induced pluripotent stem cellsCell Stem Cell201061717920036631
- KasperkiewiczJKovacichJALichtmanDXPS studies of vanadium and vanadium oxidesJ Electron Spectros Relat Phenomena1983322123132
- ColtonRJGuzmanAMRabalaisJWElectrochromism in some thin-film transition-metal oxides characterized by X-ray electron spectroscopyJ Appl Phys1978491409416
- ZhouHLiJXinYCaoXBaoSJinPElectron transfer induced thermochromism in a VO2-graphene-Ge heterostructureJ Mater Chem C Mater Opt Electron Devices201531950895097
- BerryFJBrettMEMarbrowRAPattersonWRNotes. An X-ray photoelectron spectroscopic study of the surface properties of vanadium antimonate and β-antimony tetroxideDalton Trans19845985987
- WangJLiJQianSAntibacterial surface design of titanium-based biomaterials for enhanced bacteria-killing and cell-assisting functions against periprosthetic joint infectionACS Appl Mater Interfaces2016817111621117827054673
- ZhangHJiZXiaTUse of metal oxide nanoparticle band gap to develop a predictive paradigm for oxidative stress and acute pulmonary inflammationACS Nano2012654349436822502734
- KaweeteerawatCIvaskALiuRToxicity of metal oxide nanoparticles in Escherichia coli correlates with conduction band and hydration energiesEnviron Sci Technol20154921105111225563693
- ParksGAThe isoelectric points of solid oxides, solid hydroxides, and aqueous hydroxo complex systemsChem Rev1965652177198
- ChasteenNDThe biochemistry of vanadiumCopper, Molybdenum, and Vanadium in Biological Systems53HeidelbergSpringer1983105138
- ZhouHLiJBaoSWangDLiuXJinPThe potential cytotoxicity and mechanism of VO2 thin films for intelligent thermochromic windowsRSC Adv20155129106315106324
- BezerraAGBarisonAOliveiraVSThe mechanism of cysteine detection in biological media by means of vanadium oxide nanoparticlesJ Nanopart Res20121491123
- ReeceSYNoceraDGProton-coupled electron transfer in biology: results from synergistic studies in natural and model systemsAnnu Rev Biochem20097867369919344235
- MyersCNealsonKHBacterial manganese reduction and growth with manganese oxide as the sole electron acceptorScience198824048571319132117815852
- HarrisHWEl-NaggarMYBretschgerOElectrokinesis is a microbial behavior that requires extracellular electron transportProc Natl Acad Sci U S A2010107132633120018675
- HartshorneRSReardonCLRossDCharacterization of an electron conduit between bacteria and the extracellular environmentProc Natl Acad Sci U S A200910652221692217420018742
- HosseiniMJShakiFGhazi-KhansariMPourahmadJToxicity of vanadium on isolated rat liver mitochondria: a new mechanistic approachMetallomics20135215216623306434
- GhoshSKSahaRSahaBToxicity of inorganic vanadium compoundsRes Chem Intermed201441748734897
- PangMHuJZengHCSynthesis, morphological control, and antibacterial properties of hollow/solid Ag2S/Ag heterodimersJ Am Chem Soc201013231107711078520681710
- ZhaoYYeLLiuHVanadium compounds induced mitochondria permeability transition pore (PTP) opening related to oxidative stressJ Inorg Biochem2010104437137820015552
- FaheyRCBrownWCAdamsWBWorshamMBOccurrence of glutathione in bacteriaJ Bacteriol1978133311261129417060
- WinterbournCCMetodiewaDReactivity of biologically important thiol compounds with superoxide and hydrogen peroxideFree Radic Biol Med1999273–432232810468205
- Carmel-HarelOStorzGRoles of the glutathione- and thioredoxin-dependent reduction systems in the Escherichia coli and Saccharomyces cerevisiae responses to oxidative stressAnnu Rev Microbiol20005443946111018134
- GorzsaśAAnderssonIPetterssonLOn the fate of vanadate in human bloodEur J Inorg Chem200620061835593565
- RehderDImplications of vanadium in technical applications and pharmaceutical issuesInorg Chim Acta20174552378389
- JarrellJDDollyBMorganJRControlled release of vanadium from titanium oxide coatings for improved integration of soft tissue implantsJ Biomed Mater Res A200990127228118496866
- BarrioDAEtcheverrySBPotential use of vanadium compounds in therapeuticsCurr Med Chem201017313632364220846114
- KellDBPretoriusEOn the translocation of bacteria and their lipopolysaccharides between blood and peripheral locations in chronic, inflammatory diseases: the central roles of LPS and LPS-induced cell deathIntegr Biol (Camb)20157111339137726345428