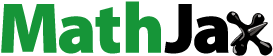
Abstract
Natural polyelectrolyte multilayers of chitosan (CHI) and alginate (ALG) were alternately deposited on doxorubicin (DOX)-loaded poly (lactic-co-glycolic acid) (PLGA) nanoparticles (NPs) with layer by layer self-assembly to control drug release for antitumor activity. Numerous factors which influenced the multilayer growth on nano-colloidal particles were studied: polyelectrolyte concentration, NaCl concentration and temperature. Then the growth regime of the CHI/ALG multilayers was elucidated. The coated NPs were characterized by transmission electron microscopy, atomic force microscopy, X-ray diffraction and a zeta potential analyzer. In vitro studies demonstrated an undesirable initial burst release of DOX-loaded PLGA NPs (DOX-PLGA NPs), which was relieved from 55.12% to 5.78% through the use of the layer by layer technique. The release of DOX increased more than 40% as the pH of media decreased from 7.4 to 5.0. More importantly, DOX-PLGA (CHI/ALG)3 NPs had superior in vivo tumor inhibition rates at 83.17% and decreased toxicity, compared with DOX-PLGA NPs and DOX in solution. Thus, the presently formulated PLGA-polyelectrolyte NPs have strong potential applications for numerous controlled anticancer drug release applications.
Introduction
Cancer remains a major cause of death in the world, killing approximately 570,000 people in the USA in 2011 alone.Citation1 Over the past several decades, significant advancements have been made to understand cancer biology. However, most current anticancer agents lead to significant systemic toxicity and side effects (such as bone marrow suppression, cardiomyopathy, nephrotoxicity, neurotoxicity and multidrug resistance), which limits their clinical use.Citation2
Recently, nanoscale drug delivery systems have been gaining in popularity. Entrapping drugs in nanocarriers can bypass the above impediments by modifying drug biodistribution and controlling drug release.Citation3–Citation5 Santhi et al demonstrated that methotrexate-loaded bovine serum albumin nanoparticles (NPs) caused a significant increase in drug distribution to the spleen, liver and lungs, in comparison to the drug power.Citation6 Feng et al developed paclitaxel-loaded poly (lactic-co-glycolic acid) (PLGA) nanospheres to achieve prolonged drug release profiles and better therapeutic effects.Citation7 Moreover, NPs not only effectively protect drugs from ionic or enzymatic degradation, but also increase their efficacy and stability and decrease their toxicity.Citation4,Citation8
PLGA copolymers are degraded in vivo by hydrolysis of the ester linkage to glycolic acid and lactic acid, which are then easily metabolized by the Krebs cycle and excreted as water and carbon dioxide.Citation9,Citation10 Owing to good biocompatibility properties, biodegradability, nontoxicity, nonimmunogenicity and sustained release properties, PLGA NPs have been widely used as drug delivery carriers for anticancer drugs.Citation11–Citation15 PLGA is approved by the Food and Drug Administration and the European Medicines Agency for various drug delivery systems for human applications.Citation11 Several drugs (including dexamethasone, 5-fluorouracil and paclitaxel) have been successfully incorporated into PLGA.Citation16 In addition, PLGA-based nanomedicine products have been investigated in clinical trials.Citation17,Citation18 However, the obvious initial burst release phenomenon of PLGA NPs is unfavorable for anticancer drugs with significant systemic toxicity, thus indicating that drug release needs to be better controlled.Citation19,Citation20
Recently, some strategies to control initial drug burst release have received increasing attention. Adibkia et al demonstrated that naproxen–eudragit® RS100 NPs showed a slow drug release rate with reduced burst release compared to the free drug.Citation21 Ud Din et al developed hydrogel-loaded solid lipid NPs for rectal administration of flurbiprofen to reduce the initial burst effect.Citation22 Although these NPs could decrease the initial burst release to some degree, there were still some disadvantages, including the poor biodegradability of these polymers, the use of surfactants and so on. In addition, the classical methods for controlling the release of drugs from PLGA particles are inconvenient, such as using various additivesCitation23,Citation24 and preparing particles mixed with other polymers,Citation25 limiting their wide application. Therefore, a simple and effective method is required for achieving lower initial drug burst release and well-controlled drug release from PLGA NPs.
Layer by layer (LbL) assembled NPs are promising strategies for incorporating polyelectrolytes onto the surfaces of nanocarriers to provide enhanced stability, cellular uptake, regulation of drug release and targeting capabilities,Citation26–Citation29 and have recently shown great value in gene and drug delivery.Citation28,Citation30–Citation32 With multilayer alternative deposition of oppositely charged polyelectrolytes, drug-loaded carriers and drug microcrystals could decrease initial drug burst release and sustain drug release.Citation33–Citation35 The release of several drugs, including dexamethasone, artemesinin and ampicillin, has been prolonged by the use of LbL NPs.Citation36–Citation38 Although LbL self-assembly has been widely used recently to control initial burst release, research on the use of LbL for controlling drug release over a long period of time from NPs is limited. The elucidation of factors that influence multilayer growth on nanoscale particles is also largely absent, which plays an important role in multilayer growth.
In this study, multilayers of the bio-polyelectrolytes chitosan (CHI) and alginate (ALG) were successfully deposited by LbL on the surface of doxorubicin-loaded PLGA NPs (DOX-PLGA NPs; ). Factors influencing the CHI/ALG coating were investigated by monitoring the coating weight of the LbL multilayers, and the growth regime of the CHI/ALG multilayers on nano-colloidal particles was demonstrated as well. A systematic characterization of coated and bare NPs, including transmission electron microscopy (TEM), atomic force microscopy, zeta potential and X-ray diffraction (XRD), was performed here, and the effects of the different layers and medium pH on the controlled release of doxorubicin (DOX) were also studied. Finally, the in vivo antitumor efficiency and toxicity of DOX-loaded NPs were evaluated.
Materials and methods
Materials
DOX (99.41% purity) was purchased from HuaFeng United Technology Co. Ltd (Beijing, People’s Republic of China). PLGA 50:50 (molecular weight 15–20 kDa) was obtained from the Institute of Medical Instruments (Shandong, People’s Republic of China). ALG (200 MPa⋅s), CHI (molecular weight 49 kDa) and fluorescein isothiocyanate (FITC) were purchased from Sigma Aldrich. FITC-labeled ALG (FITC-ALG) was synthesized as reported in the literature.Citation39 All other materials were of analytical grade.
Preparation of DOX-PLGA NPs
DOX-PLGA NPs were prepared by the double water- in-oil-in-water (W1/O/W2) emulsion-solvent evaporation technique.Citation40 Briefly, 0.25 mL of a 2 mg/mL DOX solution was emulsified in a mixture of dichloromethane (1 mL) and acetone (1 mL) containing 50 mg of PLGA by sonication for 15 min (100 W) in an ice bath. The resulting primary emulsion was added to 8 mL of 2% (w/v) Poloxamer188 solution and was sonicated at 300 W for 10 min in an ice bath to form the double emulsion. The resulting double emulsion was diluted with a 0.3% Poloxamer188 aqueous solution and was stirred at 150 rpm for 4 h at room temperature to evaporate the organic solvents. The NPs were collected by ultracentrifugation at 12,000 rpm for 20 min, and washed with distilled water repeatedly to remove the residual Poloxamer188 and excess DOX. The PLGA NPs were resuspended in solution with a DOX concentration of 0.183 mg/mL for further study.
LbL self-assembly on DOX-PLGA NPs
Polyelectrolyte multilayers of CHI and ALG were deposited on the DOX-PLGA NPs through LbL self-assembly in water. All factors influencing the LbL process were investigated and optimized as summarized in (when the main factor was studied in the experiment and other factors were selected at level 3).
Table 1 Factors influencing the multilayer growth
The first layer was deposited by adding 4 mL of a CHI solution (pH 3.5, 2 mg/mL) to 2 mL of DOX-PLGA NPs. The mixture was incubated under gentle shaking to allow for the adsorption of CHI onto the negatively charged NPs. Excess CHI was removed by three cycles of centrifugation (12,000 rpm, 10 min), washing and redispersing with water. The following FITC-ALG layer was deposited with an FITC-ALG solution (pH 6–8) and the processes of centrifugation/washing were performed as noted above. A bilayer coating was then finished. The two polyelectrolytes were deposited alternatively until the desired number of LbL coating layers was reached. Finally, the PLGA-polyelectrolyte NPs were redispersed in water.
To determine the adsorption time and the amount of adsorbed FITC-ALG on the PLGA NPs, the fluorescence intensity of the supernatant after adsorption was measured with an RF-5301 PC spectrofluorophotometer (Shimadzu Co. Ltd., Tokyo, Japan) at each end of the CHI/FITC-ALG adsorption cycle. The excitation wavelength was 495 nm and the emission wavelength was 515 nm.
Characterization
Particle size and ζ-potential
Particle sizes and ζ-potential of DOX-PLGA NPs and CHI/ALG-coated DOX-PLGA NPs after every layer of deposition were measured by laser light scattering (ZetaPlus; Brookhaven Instrument Corp., Holtsville, NY, USA) at a scattering angle of 90°C at 25°C. Each sample was measured in triplicate.
Transmission electron microscopy
TEM measurements for the coated and uncoated NPs were performed with a JEM-1010 electron microscope operating at an accelerating voltage of 80 kV. Samples were prepared by depositing the NP suspension on a copper grid. The suspension was then air-dried before all measurements.
Powder XRD measurement
Powder X-ray diffractograms of DOX, blank PLGA NPs, DOX-PLGA NPs with the physical mixture of DOX and blank PLGA NPs were obtained with an X-ray diffractometer (D8 Advance, Bruker AXS). All samples were used with lyophilized powder. Typically, the diffractogram was recorded in the 2θ range of 3–40.
In vitro drug release
Triplicate samples of DOX-PLGA, DOX-PLGA (CHI/ALG)1, DOX-PLGA (CHI/ALG)2 and DOX-PLGA (CHI/ALG)3 NPs were respectively suspended in 1 mL of isotonic phosphate-buffered saline (PBS; 0.05 M, pH 7.4) in a microcentrifuge tube. The samples were then incubated on a shaker at a fixed speed of about 100 rpm (SHY-2A, Jin Tan, People’s Republic of China) at 37°C. At defined time intervals, the NPs were centrifuged, the supernatant was removed, and fresh phosphate buffer was replaced. The concentration of each supernatant sample was measured by an ultraviolet spectrophotometer (Agilent 8453; Agilent Technologies, Santa Clara, CA, USA) at 480 nm. The cumulative percentage of the drug released in the first hour was expressed as the initial burst release. The time at which half of the final theoretically encapsulated drug released was expressed as the half release time (t1/2).
Triplicate samples of DOX-PLGA (CHI/ALG)3 NPs were suspended in 1 mL PBS at different pH values (pH 7.4, pH 6.8 and pH 5.0) in a microcentrifuge tube, and other measurements were performed as described above.
In vivo study
Animals
Male mice (4–6 weeks old, 20–25 g) bearing mouse sarcoma 180 (S180) tumors were supplied by Origin Biosciences Inc. (Nanjing, People’s Republic of China). All studies were conducted in accordance with the principles of Laboratory Animal Care and were approved by the Department of Laboratory Animal Research at China Pharmaceutical University. The usage of animals was agreed upon by the China Pharmaceutical University Animal Management and Ethics Committee.
Antitumor efficacy of DOX-PLGA (CHI/ALG)3 NPs
The effect of the developed formulations on tumor growth was assessed by daily measurements of tumor size with a digital caliper. The mice were randomly divided into five groups with six males in each group and intravenously administered one of the following treatments through the tail vein: 1) saline (the control group), 2) blank PLGA NPs, 3) DOX solution, 4) DOX-PLGA NPs and 5) DOX-PLGA (CHI/ALG)3 NPs with a DOX dose of 5 mg/kg on days 0, 2, 4 and 6.
After administration, the animals were monitored carefully, and the body weight and tumor size were measured at given time intervals. Tumor volumes were calculated as follows:
where a represents the length of the tumor and b represents the width.Citation41
After 8 days of observation, the mice were sacrificed by cervical dislocation, and tumor inhibition rate was calculated by the equation:
where Wtreated and Wcontrol are the average tumor weight of the treated group and the normal saline group, respectively, and TIR is the tumor inhibition rate.
Statistical analysis
All the results were presented as mean ± standard deviation (SD) and were analyzed by one-way analysis of variance. Statistical significance was set at *P<0.05 and **P<0.01 throughout the study.
Results and discussion
Preparation of DOX-PLGA NPs
PLGA NPs with a DOX concentration of 0.183 mg/mL were successfully prepared. As shown in , the resulting colloidal solution was a translucent pale blue liquid with ζ-potential −30.5 mV. The particle size and the polydispersity index of DOX-PLGA NPs were 172.3±3.9 nm and 0.156±0.002, respectively.
Table 2 Physicochemical properties of DOX-PLGA NPs and chitosan/alginate-coated DOX-PLGA NPs (n=3; mean ± standard deviation)
The XRD patterns are shown in . DOX showed one intense peak at 2θ of 4.5° and numerous small peaks between 16.75° and 27° (). The XRD thermogram of DOX-PLGA NPs () was similar to that of the blank PLGA NPs (), while the characteristic crystalline peaks of DOX disappeared in the NPs compared with the physical mixture of DOX and blank PLGA NPs (), indicating that DOX was encapsulated in DOX-PLGA NPs successfully.
Factors affecting the growth of the CHI/ALG multilayer
Saturation adsorption time
The same amounts of DOX-PLGA NPs and polyelectrolyte were added to each tube, and then the FITC-ALG of the supernatant was determined after 5, 10, 15, 20 and 30 min. The results are shown in . Initially, the concentration of FITC-ALG decreased remarkably from 1.31 mg/mL at 0 min to 0.47 mg/mL at 10 min, and then achieved a balance at 20 min after centrifugation. It was found that the adsorption of polyelectrolyte on the colloidal particles reached a maximum after 20 min of incubation. Therefore, the following experiments were performed at this level (20 min).
Effect of polyelectrolyte concentration
Three concentrations of FITC-ALG were used for (CHI/ALG)3 deposition. Growth of the polyelectrolyte multilayer was monitored by determining the fluorescence intensity of the supernatant after each adsorption cycle of FITC-ALG. The results () showed that the coating weight of the multilayer increased with increased concentration of the polyelectrolyte. This could be explained by a change in polymer conformation. At low polyelectrolyte concentrations, the conformation of the absorbed chain was rather flat without irregular structure because each polyelectrolyte chain could adsorb on many binding sites.Citation42 In contrast, there were not enough binding sites for polyelectrolyte chains and each could adsorb on just a few binding sites at high polyelectrolyte concentrations, which resulted in more polymer chains absorbed and an increase of the coating weight of the multilayer.Citation42,Citation43
Figure 4 Relationship between the bilayer number and coating weight of the self-assembly film in (A) different polyelectrolyte concentrations, (B) different NaCl concentrations and (C) different temperatures (n=3; mean ± standard deviation).
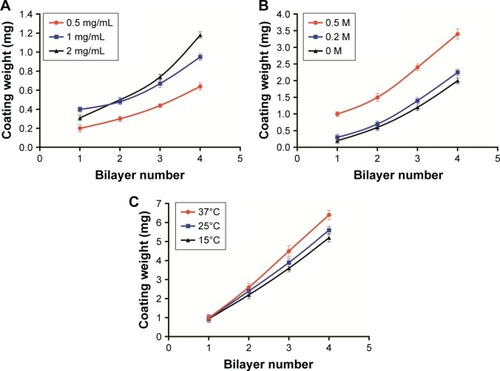
Moreover, the intersection of the curves with 1 and 2 mg/mL was probably due to the viscosity and film-forming properties of the polyelectrolyte.Citation44,Citation45 Initially, the growth rate of the multilayer with 2 mg/mL was very slow, which was limited by the high viscosity of the polyelectrolyte solution. After several adsorption cycles, the growth of the multilayer was more stable and regular with little effect of viscosity on polyelectrolyte adsorption. So, the growth of the multilayer increased with polyelectrolyte concentration, which was also verified by Voigt et al.Citation46
During the coating process, irreversible particle aggregation occurred due to poor dispersion of the polyelectrolyte solution at concentrations beyond 2 mg/mL. Tan et al found that thicker multilayers could significantly reduce the burst release of the drug from a nanogel.Citation47 In order to obtain a satisfactory release profile, 2 mg/mL of the polyelectrolyte was selected as the optimal concentration for subsequent experiments.
Effect of NaCl concentration
During (CHI/ALG)3 deposition, growth of the polyelectrolyte multilayer was determined as described above. The results observed in revealed that with increasing NaCl concentration, the coating weight of the multilayer film increased, and a similar trend was also seen in the modifications of (CHI/ALG)1, (CHI/ALG)2 and (CHI/ALG)4 for the PLGA NPs. This could be explained by the fact that ions in the solution can shield the charge from the long polymer chain and reduce repulsion between charged groups in polymer chains which became curled to form a thicker multilayer.Citation48 Ye et al reported that the average thickness of the CHI/ALG multilayer was maintained when the added NaCl concentration exceeded 0.5 mg/mL.Citation49 In our experiment, it was also found that the coating weight profiles of the multilayer film became similar when the NaCl concentration was 0.5 mg/mL. Therefore, 0.5 mg/mL NaCl was chosen as the optimal condition in our study.
Effect of temperature
Three temperatures were investigated when the CHI/ALG was deposited, as the growth of the polyelectrolyte multilayer was monitored. The findings () suggested that the increase in temperature caused a gradual increase in the multilayer coating weight at two or more bilayers of PLGA NP, and that temperature had no significant influence on the multilayer coating weight of one bilayer of NPs. High temperature was supposed to increase the thermal motion of the polymer long chain, which improved the fluidity of the multilayer.Citation47 Therefore, more polyelectrolytes can infiltrate into the layer, which increases the thickness of the film. The glass transition temperature of PLGA used in our study was about 45°C;Citation50 therefore, the temperature was fixed at 37°C to avoid PLGA phase changes.
Growth regime of CHI/ALG multilayer on three-dimensional PLGA NPs
As shown in , the fitting equations for growth curves at different polyelectrolyte concentrations, NaCl concentrations (>0) and adsorption temperatures were exponential, power and power, respectively, showing that the multilayer growth was asymmetrical. The asymmetrical-like growth behavior could be attributed to the lower charge density of bio-polyelectrolyte chains, resulting in weakened electrostatic repulsion between charged segments and highly coiled conformations of polymer chains.Citation46 The adsorption of highly coiled polymer chains led to a thicker multilayer with a rough surface. This result indicated that bio-polyelectrolyte multilayers on three-dimensional colloidal particles grew asymmetrically, which was similar to the results on two-dimensional silicon substrates.Citation51,Citation52 Compared with a bi-dimensional environment, three-dimensional colloidal particles could be used to investigate the coating process quantitatively by monitoring the coating weight of the LbL multilayers, which could reflect the film-forming process more accurately.
Table 3 The regression equations of the layer number and the coating weight (n=3; mean ± standard deviation)
With these factors influencing multilayer growth and the asymmetrical-like growth of the CHI/ALG multilayers, the CHI/ALG coating could be adjusted to reduce a burst release and achieve a satisfied and sustained release of anticancer drugs.
Characterization
Particle size and ζ-potential characterization
The particle sizes, polydispersity indexes and zeta potentials of DOX-PLGA NPs and CHI/ALG-coated DOX-PLGA NPs are presented in . The size and the polydispersity index of DOX-PLGA (CHI/ALG)3 NPs prepared in optimal conditions were 196.3±6.7 nm and 0.179±0.007, respectively. With the coating of a polyelectrolyte, the sizes of the PLGA-polyelectrolyte NPs gradually increased. shows the ζ-potential as a function of the layer number of polyelectrolytes for the DOX-PLGA NPs coated with CHI/ALG layers. The value of ζ-potential for the first polyelectrolyte layer (36.4 mV) corresponds to the deposition of CHI, the second (−42.2 mV) corresponds to the deposition of ALG, the third (36.7 mV) corresponds to another layer of CHI and the fourth (−48.0 mV) corresponds to a new layer of ALG NPs. The obvious switching of ζ-potential indicated a successful self-assembly on the DOX-PLGA NPs, and there was no significant difference between the zeta potentials of the different bilayers of the PLGA NPs. Moreover, the negative surface charge also reflected the physical stability of the DOX-PLGA (CHI/ALG)3 NPs.
Morphology characterization
TEM was used to examine the morphology of the NPs. As seen in , the DOX-PLGA NPs were found to be spherical and uniform with sizes of <150 nm (). With LbL self-assembly of CHI and ALG, a core–shell structure was observed, and the DOX-PLGA (CHI/ALG)3 NP periphery showed nonuniform polyelectrolyte anisomerous textures (). This phenomenon provided further proof of formation of polyelectrolyte multilayers and was a reflection of the nonuniform asymmetrical-like growth of the multilayer. Meanwhile, the average sizes of the DOX-PLGA NPs and DOX-PLGA (CHI/ALG)3 NPs, as preliminarily determined by dynamic light scattering (172.3±3.9 and 196.3±6.7 nm, respectively), were larger than those determined from TEM images (118.7±7.3 and 179.4±4.2 nm, respectively). This could be attributed to the fact that the intensity of the scattered light is proportional to the sixth power of the droplet size, and dynamic light scattering overdepends on larger size fractions.Citation53
In vitro drug release
Effect of different layer numbers on the drug release
The in vitro release behavior of DOX from the NPs with different bilayers was investigated. As shown in and , the initial burst release was obviously reduced, compared with the bare DOX-PLGA NPs. The release rate and release percentage of DOX decreased with an increase of CHI/ALG layer numbers on the surface of the NPs. With CHI/ALG coating, the initial burst release from the DOX-PLGA (CHI/ALG)3 NPs was reduced from 55.12% to 5.78%, while the half release time (t1/2) was prolonged from 0.78 to 61.58 h, which could be attributed to the increased layer thickness that made DOX hard to permeate. Zhou et al developed poly (acrylic acid) and polyethyleneimine-coated PLGA NPs, and it was also found that the more layers, the slower the drug release rate.Citation54 Based on the above results, it could be concluded that polyelectrolyte multilayers coated on NP surfaces can not only prolong drug release time, but also relieve initial burst release, which may decrease systemic adverse reactions toward drug resistance, diminish their toxicity and improve their therapeutic efficacy. In comparison to common PLGA NPs, based on these results, DOX-PLGA (CHI/ALG)3 NPs may present higher safety profiles and better antitumor efficacy.
Table 4 Effect of bilayer number on DOX release (n=3; mean ± standard deviation)
Effect of medium pH on the release of DOX-PLGA (CHI/ALG)3 NPs
and show the release behavior of DOX-PLGA (CHI/ALG)3 NPs for different values of isotonic PBS (pH 5.0, pH 6.8 and pH 7.4). A significant decrease in half release time (t1/2) and an increase in the release rate of DOX were observed at a decreased medium pH, from a t1/2 of 61.58 h at pH 7.4 to 20.5 h at pH 5.0, similar to earlier reports.Citation55,Citation56 This could be attributed to the fact that DOX, a basic drug, had a strongly pH-dependent solubility with decreased solubility at basic pH values, and the medium with low pH could facilitate drug release.Citation57 In addition, acidic conditions are conducive to enhancing the hydrolysis of the ester bonds in the polymer chains, resulting in the degradation of the polymer and further acceleration of DOX release.Citation58 Typically, the environment of stromal cells in the tumor tissue is weakly acidic (pH <7), while the pH of endosomes and lysosomes in tumor cells is even lower (pH 4–6).Citation59–Citation65 These results indicated that there would be superiority of antitumor therapy to some degree and DOX-loaded PLGA-polyelectrolyte NPs would be appropriate for antitumor therapy in acidic tumor environments.
Table 5 Effect of medium pH on drug release from doxorubicin-poly (lactic-co-glycolic acid) (chitosan/alginate)3 nanoparticles (n=3; mean ± standard deviation)
In vivo antitumor efficacy
To confirm the antitumor potential of DOX-PLGA (CHI/ALG)3 NPs in vivo, the antitumor efficiency was evaluated in S180 tumor-bearing mice.Citation66 As shown in , all DOX formulations effectively inhibited tumor growth, compared with the saline group and blank PLGA NPs (P<0.01). Among all groups, DOX-PLGA (CHI/ALG)3 NPs exhibited the greatest tumor growth inhibition, followed by DOX-PLGA NPs and the DOX solution, and a significant difference was found between DOX-PLGA (CHI/ALG)3 NPs and DOX-PLGA NPs (P<0.05). Moreover, tumor inhibition rates for the different treatments based on tumor weights () were in agreement with the results of the tumor volume experiment. DOX-PLGA (CHI/ALG)3 NPs exhibited the best antitumor efficiency (83.17%), followed by DOX-PLGA NPs (61.35%) and DOX solution (23.77%). CHI and ALG could increase the hydrophilicity of the PLGA NPs, which shields the NPs from clearance by the mononuclear phagocytic system/reticuloendothelial system. In addition, the enhanced permeability and retention affect has the ability to passively target NPs to the tumor sites.Citation67,Citation68 NPs with particle sizes of 20–200 nm tend to concentrate in tumors by the enhanced permeability and retention phenomenon.Citation69,Citation70
Table 6 Tumor weight and tumor inhibition rate of normal saline and different DOX formulations on S180 tumor-bearing mice (n=6; mean ± standard deviation)
Figure 9 (A) In vivo antitumor efficacy of DOX solution, DOX-PLGA NPs, DOX-PLGA (CHI/ALG)3 NPs, blank PLGA NPs and saline on S180 tumor-mice following i.v. administration (dose =5 mg/kg; n=6; mean ± SD). (B) Changes in body weight of tumor-bearing mice with time after i.v. administration (dose =5 mg/kg; n=6; mean ± SD).
Notes: *P<0.05; **P<0.01.
Abbreviations: ALG, alginate; CHI, chitosan; DOX, doxorubicin; i.v., intravenous; NPs, nanoparticles; PLGA, poly (lactic-co-glycolic acid); SD, standard deviation.
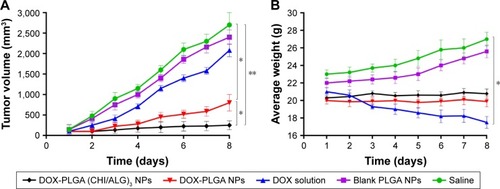
In order to estimate the in vivo toxicity of the DOX formulations, the body weight of the mice was measured. Obvious body weight loss after drug administration is usually associated with drug toxicity. As presented in , there was a significant decrease in body weight for the DOX solution treated group (16.67%). However, this phenomenon was not found after administration of the DOX-PLGA (CHI/ALG)3 NPs, indicating that DOX-PLGA (CHI/ALG)3 NPs could significantly decrease the toxicity of the free DOX and could be well tolerated at the tested dose level (5 mg DOX/kg). It could be easily envisioned that the lower drug burst release decreased toxicity. The integrated results verified that the DOX-PLGA (CHI/ALG)3 NPs were much safer and could effectively improve antitumor efficacy, compared with the DOX solution and the DOX-PLGA NPs.
Conclusion
DOX-PLGA NPs coated with CHI and ALG were successfully prepared by the LbL self-assembly process, and factors influencing the multilayer growth on the PLGA NPs were investigated. DOX-PLGA NPs sized below 200 nm were formed. The undesired initial burst release of DOX was significantly reduced from 55.12% to 5.78% through the LbL approach, and the half release time (t1/2) increased from 0.78 to 61.58 h with increasing layer number. Meanwhile, the NPs coated with CHI and ALG showed pH-dependent characteristics and the release of DOX increased with a decrease in medium pH. Compared with the DOX solution and DOX-PLGA NPs, DOX-PLGA (CHI/ALG)3 NPs exhibited a superior in vivo tumor inhibition rate of 83.17% and decreased toxicity.
To conclude, PLGA NPs coated with CHI/ALG by LbL is a novel and effective approach to minimize initial burst release, sustain release of DOX and decrease toxicity. These findings indicate that PLGA-polyelectrolyte NPs have potential numerous applications for controlled anticancer drug release.
Acknowledgments
This work was financially supported by the Natural Science Foundation of China (no 81402878, 81572978 and 81473160) and the Scientific Foundation of Jiangsu Provincial Commission of Health (no Z201508). The abstract of this paper was presented at the American Institute of Chemical Engineers Conference on Nov 16, 2016 as a poster presentation with preliminary findings. The poster’s abstract was published in “Poster Abstracts” on the website http://www3.aiche.org/proceedings/Abstract.aspx?PaperID=471267.
Disclosure
The authors report no conflicts of interest in this work.
References
- SiegelRWardEBrawleyOJemalAThe impact of eliminating socioeconomic and racial disparities on premature cancer deathsCA Cancer J Clin201161421223621685461
- TacarOSriamornsakPDassCRDoxorubicin: an update on anticancer molecular action, toxicity and novel drug delivery systemsJ Pharm Pharmacol201365215717023278683
- KamalyNYameenBWuJFarokhzadOCDegradable controlled-release polymers and polymeric nanoparticles: mechanisms of controlling drug releaseChem Rev201611642602266326854975
- ElzoghbyAOSamyWMElgindyNAAlbumin-based nanoparticles as potential controlled release drug delivery systemsJ Control Release2012157216818221839127
- ArvizoRRMirandaORMoyanoDFModulating pharmacokinetics, tumor uptake and biodistribution by engineered nanoparticlesPloS One201169e2437421931696
- SanthiKDhanarajSAKoshyMPonnusankarSSureshBStudy of biodistribution of methotrexate-loaded bovine serum albumin nanospheres in miceDrug Dev Ind Pharm2000261293129611147130
- FengSSMuLChenBHPackDPolymeric nanospheres fabricated with natural emulsifiers for clinical administration of an anticancer drug paclitaxel (Taxol®)Mater Sci Eng C20022018592
- Merisko-LiversidgeEMLiversidgeGGDrug nanoparticles: formulating poorly water-soluble compoundsToxicol Pathol2008361434818337220
- JainRAThe manufacturing techniques of various drug loaded biodegradable poly (lactide-co-glycolide) (PLGA) devicesBiomaterials200021232475249011055295
- PanyamJZhouWZPrabhaSSahooSKLabhasetwarVRapid endo-lysosomal escape of poly (DL-lactide-co-glycolide) nanoparticles: implications for drug and gene deliveryFASEB J200216101217122612153989
- ThamakeSIRautSLGryczynskiZRanjanAPVishwanathaJKAlendronate coated poly-lactic-co-glycolic acid (PLGA) nanoparticles for active targeting of metastatic breast cancerBiomaterials201233297164717322795543
- HrkachJVon HoffDAliMMPreclinical development and clinical translation of a PSMA-targeted docetaxel nanoparticle with a differentiated pharmacological profileSci Transl Med20124128128139
- DanhierFAnsorenaESilvaJMCocoRLe BretonAPréatVPLGA-based nanoparticles: an overview of biomedical applicationsJ Control Release2012161250552222353619
- DawidczykCMRussellLMSearsonPCNanomedicines for cancer therapy: state-of-the-art and limitations to pre-clinical studies that hinder future developmentsFrontiers Chem2014269
- TosiGBortotBRuoziBPotential use of polymeric nanoparticles for drug delivery across the blood-brain barrierCurr Med Chem201320172212222523458620
- AcharyaSSahooSKPLGA nanoparticles containing various anticancer agents and tumour delivery by EPR effectAdv Drug Delivery Rev2011633170183
- GanjuAYallapuMMKhanSBehrmanSWChauhanSCJaggiMNanoways to overcome docetaxel resistance in prostate cancerDrug Resist Updat2014171132324853766
- YallapuMMGuptaBKJaggiMChauhanSCFabrication of curcumin encapsulated PLGA nanoparticles for improved therapeutic effects in metastatic cancer cellsJ Colloid Interface Sci20103511192920627257
- GomesCMoreiraRGCastell-PerezEPoly (DL-lactide-co-glycolide) (PLGA) nanoparticles with entrapped trans-cinnamaldehyde and eugenol for antimicrobial delivery applicationsJ Food Sci2011762N16N2421535781
- SejuUKumarASawantKKDevelopment and evaluation of olanzapine-loaded PLGA nanoparticles for nose-to-brain delivery: in vitro and in vivo studiesActa Biomater20117124169417621839863
- AdibkiaKJavadzadehYDastmalchiSMohammadiGNiriFKAlaei-BeiramiMNaproxen–Eudragit® RS100 nanoparticles: preparation and physicochemical characterizationColloid Surf B2011831155159
- Ud DinFMustaphaOKimDWNovel dual-reverse thermosensitive solid lipid nanoparticle-loaded hydrogel for rectal administration of flurbiprofen with improved bioavailability and reduced initial burst effectEur J Pharm Biopharm201594647225979136
- RojasJPinto-AlphandaryHLeoEPecquetSCouvreurPFattalEOptimization of the encapsulation and release of β-lactoglobulin entrapped poly (DL-lactide-co-glycolide) microspheresInt J Pharm19991831677110361157
- Sheikh HasanASochaMLamprechtAEffect of the micro-encapsulation of nanoparticles on the reduction of burst releaseInt J Pharm20073441536117643878
- MatsumotoAMatsukawaYSuzukiTYoshinoHKobayashiMThe polymer-alloys method as a new preparation method of biodegradable microspheres: principle and application to cisplatin-loaded micro-spheresJ Control Release19974811927
- PoonZLeeJBMortonSWHammondPTControlling in vivo stability and biodistribution in electrostatically assembled nanoparticles for systemic deliveryNano Lett20111152096210321524115
- PoonZChangDZhaoXHammondPTLayer-by-layer nanoparticles with a pH-sheddable layer for in vivo targeting of tumor hypoxiaACS Nano2011564284429221513353
- HammondPTPolyelectrolyte multilayered nanoparticles: using nanolayers for controlled and targeted systemic releaseNanomedicine20127561962222630144
- MortonSWPoonZHammondPTThe architecture and biological performance of drug-loaded LbL nanoparticlesBiomaterials201334215328533523618629
- PanyamJLabhasetwarVBiodegradable nanoparticles for drug and gene delivery to cells and tissueAdv Drug Deliv Rev2012646171
- LavanDALynnDMLangerRMoving smaller in drug discovery and deliveryNat Rev Drug Discov200211778412119612
- HammondPTBuilding biomedical materials layer-by-layerMater Today2012155196206
- LiHZhuXXuJPengWZhongSWangYThe combination of adsorption by functionalized halloysite nanotubes and encapsulation by polyelectrolyte coatings for sustained drug deliveryRSC Adv20166595446354470
- AmanchaKPBalkundiSLvovYHussainAPulmonary sustained release of insulin from microparticles composed of polyelectrolyte layer-by-layer assemblyInt J Pharm201446619610824566038
- ParkMRSeoBBSongSCDual ionic interaction system based on polyelectrolyte complex and ionic, injectable, and thermosensitive hydrogel for sustained release of human growth hormoneBiomaterials20133441327133623149013
- PargaonkarNLvovYMLiNSteenekampJHde VilliersMMControlled release of dexamethasone from microcapsules produced by polyelectrolyte layer-by-layer nanoassemblyPharm Res200522582683515906179
- AnalAKStevensWFChitosan–alginate multilayer beads for controlled release of ampicillinInt J Pharm20052901455415664129
- ChenYLinXParkHGreeverRStudy of artemisinin nanocapsules as anticancer drug delivery systemsNanomed Nanotechnol Biol Med200953316322
- LamprechtASchäferUFLehrCCharacterization of microcapsules by confocal laser scanning microscopy: structure, capsule wall composition and encapsulation rateEur J Pharm Biopharm20004911910613921
- DördelmannGKozlovaDKarczewskiSLizioRKnauerSEppleMCalcium phosphate increases the encapsulation efficiency of hydrophilic drugs (proteins, nucleic acids) into poly (d, l-lactide-co-glycolide acid) nanoparticles for intracellular deliveryJ Mater Chem B201424172507259
- ZhenXWangXXieCWuWJiangXCellular uptake, antitumor response and tumor penetration of cisplatin-loaded milk protein nanoparticlesBiomaterials20133441372138223158934
- FleerGStuartMCScheutjensJMHMCosgroveTVincentBPolymers at InterfacesCambridge, UKChapman & Hall1993
- KlitzingRVKolarićBJaegerWBrandtAStructuring of poly (DADMAC) chains in aqueous media: a comparison between bulk and free-standing film measurementsPhys Chem Chem Phys200241019071914
- PicartCLavallePHubertPBuildup mechanism for poly (L-lysine)/hyaluronic acid films onto a solid surfaceLangmuir2001172374147424
- BoulmedaisFBallVSchwintePFrischBSchaafPVoegelJCBuildup of exponentially growing multilayer polypeptide films with internal secondary structureLangmuir2003192440445
- VoigtUJaegerWFindeneggGHKlitzingRVCharge effects on the formation of multilayers containing strong polyelectrolytesJ Phys Chem B20031072252735280
- TanHLMcMurdoMJPanGVan PattenPGTemperature dependence of polyelectrolyte multilayer assemblyLangmuir2003192293119314
- LöscheMSchmittJDecherGBouwmanWGKjaerKDetailed structure of molecularly thin polyelectrolyte multilayer films on solid substrates as revealed by neutron reflectometryMacromolecules1998312588938906
- YeSWangCLiuXTongZMultilayer nanocapsules of poly-saccharide chitosan and alginate through layer-by-layer assembly directly on PS nanoparticles for releaseJ Biomater Sci Polym Ed200516790992316128296
- MuthuMNanoparticles based on PLGA and its co-polymer: an overviewAsian J Pharm200934266273
- NazaranPBosioVJaegerWAnghelDFKlitzingRVLateral mobility of polyelectrolyte chains in multilayersJ Phys Chem B2007111298572858117461569
- PoptoshevESchoelerBCarusoFInfluence of solvent quality on the growth of polyelectrolyte multilayersLangmuir200420382983415773111
- CalzolaiLGillilandDGarcìaCPRossiFSeparation and characterization of gold nanoparticle mixtures by flow-field-flow fractionationJ Chromatogr A20111218274234423921288528
- ZhouJMoyaSMaLGaoCShenJPolyelectrolyte coated PLGA nanoparticles: templation and release BbehaviorMacromol Biosci20099432633519089871
- FengWZhouXHeCPolyelectrolyte multilayer functionalized mesoporous silica nanoparticles for pH-responsive drug delivery: layer thickness-dependent release profiles and biocompatibilityJ Mater Chem B201314358865898
- ZhengJTianXSunYLuDYangWpH-sensitive poly (glutamic acid) grafted mesoporous silica nanoparticles for drug deliveryInt J Pharm2013450129630323598077
- GautierJMunnierESoucéMChourpaIEyrollesLDAnalysis of doxorubicin distribution in MCF-7 cells treated with drug-loaded nanoparticles by combination of two fluorescence-based techniques, confocal spectral imaging and capillary electrophoresisAnal Bioanal Chem2015407123425343525749791
- NakayamaMAkimotoJOkanoTPolymeric micelles with stimuli-triggering systems for advanced cancer drug targetingJ Drug Target201422758459925012066
- TrédanOGalmariniCMPatelKTannockIFDrug resistance and the solid tumor microenvironmentJ Natl Cancer Inst200799191441145417895480
- YuanFDellianMFukumuraDVascular permeability in a human tumor xenograft: molecular size dependence and cutoff sizeCancer Res19955517375237567641188
- MatsumuraYMaedaHA new concept for macromolecular therapeutics in cancer chemotherapy: mechanism of tumoritropic accumulation of proteins and the antitumor agent smancsCancer Res19864612 Part 1638763922946403
- PopovićZLiuWChauhanVPA nanoparticle size series for in vivo fluorescence imagingAngew Chem20101224688318834
- GallagherFAKettunenMIDaySEMagnetic resonance imaging of pH in vivo using hyperpolarized 13C−labelled bicarbonateNature2008453719794094318509335
- UranoYAsanumaDHamaYSelective molecular imaging of viable cancer cells with pH-activatable fluorescence probesNat Med200815110410919029979
- LeeESKimDYounYSOhKTBaeYHA Virus-mimetic nanogel vehicleAngew Chem Int Ed2008471324182421
- HuMShenYZhangLQiuLPolymersomes via self-assembly of amphiphilic β-cyclodextrin-centered triarm star polymers for enhanced oral bioavailability of water-soluble chemotherapeuticsBiomacromolecules20161731026103926840277
- StormGBelliotSODaemenTLasicDDSurface modification of nanoparticles to oppose uptake by the mononuclear phagocyte systemAdv Drug Deliv Rev19951713148
- SteichenSDCaldorera-MooreMPeppasNAA review of current nanoparticle and targeting moieties for the delivery of cancer therapeuticsEur J Pharm Sci201348341642723262059
- LaneDDesigner combination therapy for cancerNat Biotechnol200624216316416465160
- ShiSShiKTanLWThe use of cationic MPEG-PCL-g-PEI micelles for co-delivery of Msurvivin T34A gene and doxorubicinBiomaterials201435154536454724582554