Abstract
The discovery of biogenic magnetic nanoparticles (BMNPs) in the human brain gives a strong impulse to study and understand their origin. Although knowledge of the subject is increasing continuously, much remains to be done for further development to help our society fight a number of pathologies related to BMNPs. This review provides an insight into the puzzle of the physiological origin of BMNPs in organisms of all three domains of life: prokaryotes, archaea, and eukaryotes, including humans. Predictions based on comparative genomic studies are presented along with experimental data obtained by physical methods. State-of-the-art understanding of the genetic control of biomineralization of BMNPs and their properties are discussed in detail. We present data on the differences in BMNP levels in health and disease (cancer, neurodegenerative disorders, and atherosclerosis), and discuss the existing hypotheses on the biological functions of BMNPs, with special attention paid to the role of the ferritin core and apoferritin.
Video abstract
Point your SmartPhone at the code above. If you have a QR code reader the video abstract will appear. Or use:
Introduction
Living organisms have a genetically programmed ability to synthesize a wide spectrum of minerals and other inorganic substances in a process known as biomineralization.Citation1–Citation3 Biosynthesis of so-called biogenic magnetic nanoparticles (BMNPs) from inorganic iron compounds is of particular interest, because of the magnetic properties of BMNPs.
BMNPs have been the object of intensive research since 1975, when they were first detected in magnetotactic bacteria (MTB). MTB exhibit magnetotaxis, or movement in response to a magnetic field, which makes them migrate along geomagnetic field lines.Citation4,Citation5 In later studies, BMNPs were found in a large number of organisms of all three domains, ie, prokaryotes, archaea, and eukaryotes ().
Table 1 Properties of BMNPs in different organisms
Formed in the process of biomineralization, crystalline BMNPs are nanocrystalline forms of antiferromagnets or ferrites, such as magnetite, maghemite, or greigite ().Citation6–Citation9 From the point of view of magnetic properties, there are two types of BMNPs, with and without remanent magnetization: those without remanent magnetization include antiferromagnetic BMNPs and ferrite BMNPs in the super-paramagnetic (SPM) phase; remanent magnetization is displayed by ferrite BMNPs in single-domain (SD) and multidomain (MD) states. As a rule, ferrite BMNPs, besides being very sensitive to applied magnetic fields, are permanent nanomagnets, showing remanent magnetization in a wide temperature range () and generating a stray magnetic field, which in their vicinity is approximately four orders of magnitude stronger than the magnetic field of the Earth. The puzzle of the role of BMNPs in living organisms has not been solved to date, and it remains to be established whether they are involved in any biological functions other than the navigation of MTB under a geomagnetic field. Geomagnetic navigation of some migratory birds and other animals can also be explained by an alternative cryptochrome model.Citation10
Table 2 Magnetic iron minerals found in BMNPs
The genetic control of BMNP biomineralization has only been studied in detail in MTB, with the corresponding biomineralization proteins identified.Citation11–Citation15 However, the physiological origin of BMNPs in other organisms, including humans, has attracted much attention for more than 30 years. The problem is very important, since elevated BMNP levels are associated with a number of human diseases, including neurodegenerative disorders and cancer.Citation16–Citation21 Until recently, there had been only one hypothesis: that ferrihydrite present in the ferritin core might be a precursor of biogenic magnetite. Indeed, ferrihydrite has been proved experimentally to be a transient mineral in the formation of magnetite in cells of MTBCitation22 and in chiton teeth.Citation9 Still, ferritin has not been found experimentally to participate in biomineralization of BMNPs. Another hypothesis on the physiological origin of BMNPs in prokaryotes, archaea, and eukaryotes, including humans, recently predicted by bioinformatic methods, postulates a common genetic mechanism of BMNP biomineralization based on homologues of biomineralization proteins of MTB.Citation23 In this review, we outline experimental and theoretical investigations of the role of biomineralization proteins, especially ferritin, in the physiological origin of BMNPs, and we point out the importance of environmental pollution only as another source of MNPs in the human body. Our intention is to aid in better understanding of the cellular production of BMNPs for efficient treatment of a number of diseases representing an urgent problem that our society is facing now and will face in the future. In the following sections, we discuss the physicochemical properties of BMNPs, the genetic control of BMNP biomineralization, the physicochemical characteristics of natural and synthetic ferritin, and the question of whether the ferritin core is a precursor of BMNPs.
Physicochemical properties of BMNPs
BMNPs in MTB magnetosomes
MTB synthesize NPs of magnetic crystals enclosed in a membrane, a structure known as a magnetosome vesicle. Single-crystal magnetite or greigite is incorporated in the magnetosome membrane, which besides phospholipids contains a number of magnetosome-associated proteins ().Citation12,Citation24,Citation25 Magnetosomes in MTB are arranged in chains,Citation4–Citation6 bearing dozens of separate magnetite NPs of size 10–135 nmCitation26,Citation27 (see ). These BMNP chains follow the long axis of the bacterium, and are attached to its membrane.Citation11 The NPs in a chain may have different crystal geometries, including octahedral, cubic, hexagonal prismatic, bullet, teardrop, and arrowhead morphologies (see examples in ).Citation28–Citation30 BMNPs are not the byproduct in MTB, but the process of biomineralization of BMNPs is energy-consuming.Citation31
Figure 1 (A) TEM of magnetotactic bacterium (Magnetospirillum strain AMB1); (B) BMNP chain in a magnetotactic bacterium; (C) zoomed BMNP chain, showing several hexagonal grains (TEM courtesy of B Leszczyński); (D) schematic representation of BMNPs in a magnetosome vesicle. BMNPs of typical shapes observed in magnetotactic bacteria, each surrounded by lipid bilayers.
Abbreviations: TEM, transmission electron microscopy; BMNP, biogenic magnetic nanoparticle.
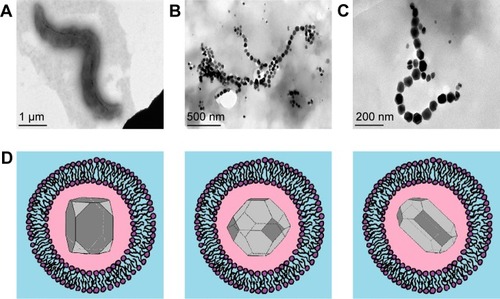
BMNPs in bacteria, archaea, and multicellular organisms
BMNPs have been observed in many prokaryotes, archaea, and eukaryotes (, ).Citation4–Citation7,Citation9,Citation32–Citation40 However, as already mentioned, for the first time BMNPs have been revealed in MTB that are characterized by the ability to “taxi” or navigate under a geomagnetic field. That is why historically the idea of magnetotaxis was transformed into an idea of magnetoreception as a basic function of BMNPs in other organisms. However, many bacteria with BMNPsCitation39 are immovable, and some multicellular organisms with BMNPs are not able to migrate long distances.Citation35 Consequently, the question about the physiological origin of BMNPs and properties of BMNPs in organisms should be considered from a broader aspect, independently of their ability to navigate Earth’s magnetic field.
Figure 2 Taxa in which BMNPs have been observed experimentally.
Notes: Red boxes, BMNPs found in representatives of the given taxa; black rectangle, reported presence of biogenic magnetite in an unclear form (either phytoferritin or botanic BMNPs).
Abbreviation: BMNPs, biogenic magnetic nanoparticles.
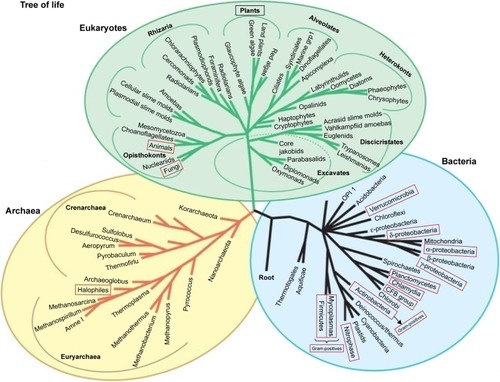
More specifically, BMNPs have been found experimentally in algae, protists,Citation41 worms,Citation35 chitons,Citation37 armored snails,Citation42 ants, butterflies,Citation43–Citation45 honeybees,Citation33,Citation44 termites,Citation34 lobster,Citation46 newts,Citation47 fish,Citation36,Citation48–Citation53 sea turtles,Citation54 birds,Citation38,Citation55–Citation58 bats,Citation59 dolphins, whales,Citation60 and humans.Citation8,Citation16,Citation17,Citation22,Citation61–Citation67 provides general information on BMNPs in different organisms.
The phenotypic manifestation of BMNPs in multicellular organisms, archaea, and non-MTB differs in many ways from that of BMNPs in MTB. As a rule, BMNPs in multicellular organisms vary in shape, size, and other properties, and are not enclosed in magnetosome vesicles.Citation39,Citation68 In archaea, in which the surroundings of BMNPs have been adequately investigated, magnet-sensitive inclusions have been found, which are surrounded by a homogeneous matrix with organic (mainly protein) components.Citation39 The absence of vesicles or a similar organic matrix consisting of protein filaments around magnetite BMNPsCitation68 has also been observed in human cells. In multicellular organisms, most BMNPs display remnant magnetization and are SD structures. However, MD and/or SPM phases have also been observed in some multicellular organisms ( and ).
In magnetite, transition from the SPM to SD phase typically occurs in the size range 25–30 nm,Citation77 and transition from SD to MD around 70 nm.Citation78 Magnetostatic interactions between magnetite NPs reduce the SPM-to-SD transition size in chains of SD grains; at the same time, the SD-to-MD transition size increases as a result of this interaction, which significantly extends the size range of the SD phase.Citation78 This is due to magnetostatic interaction fields wherein the largest magnetosome crystals observed in living bacteria (250 nm long with an aspect ratio of 0.84)Citation79 have an SD structure;Citation80 without magnetostatic interaction, they would be in an MD state.
BMNPs in normal human organs and tissues
BMNPs have been found in various human organs, including heart, liver, spleen,Citation64,Citation86,Citation87 adrenal glands,Citation65 ethmoid bone,Citation88 and brain.Citation8,Citation20,Citation61,Citation89,Citation90 In terms of phenotypic manifestation, human BMNPs differ in many ways from those observed in MTB. Separate single-crystal BMNPs and BMNP clumps of rather irregular shape have been observed in human tissues. Clumps are arranged in long chainsCitation61,Citation91 associated with the membrane. A chain is several micrometers long and comprises up to 80 NPs.Citation61 Magnetite clumps in tissues are aggregates of single-crystal particles that have remnant magnetization at 150 K. This excludes magnetic contribution from ferritin (which is superantiferromagnetic and behaves as a paramagnet at this temperature), diamagnetic bulk tissue, and paramagnetic (PM) ions.Citation19 Unlike MTB, human BMNPs have a wide size range and are observed in all phases of growth. Consequently, SPM and SD phases coexist,Citation8 and human BMNPs vary in shape, size, and other properties. Also, the number of BMNPs in normal human tissues varies widely. For example, the number of MNPs per gram of tissue in pia and dura ranges from 5 million to 100 million.Citation61
In summary, there are similarities between the production of BMNPs in humans and MTB production of magnetite NPs, organized in chains (see , , and ) of dozens of NPs, attached to the cell membrane. There are also different features of BMNPs in MTB and humans. BMNPs in humans are not characterized by control of morphology or size and are not surrounded by lipid magnetosome vesicles.
Figure 3 Ehrlich carcinoma cell.
Notes: AFM (left), MFM (right). Data from Chekchun et al.Citation21 MFM shows BMNPs in the form of dark spots arranged in chains (arrow).
Abbreviations: AFM, atomic force microscopy; MFM, magnetic force microscopy; BMNPs, biogenic magnetic nanoparticles.
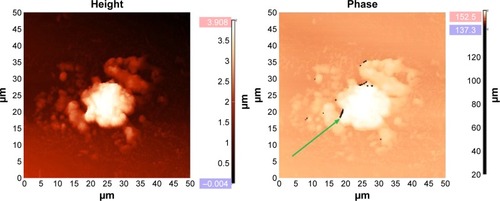
Figure 4 Fragment of an atherosclerotic plaque of mixed composition.
Notes: AFM (left), MFM (right). MFM shows BMNPs in the form of dark spots arranged in chains (arrow). There were no bacteria-like magnetosome BMNPs, but alignment of the BMNPs is visible along the plaque surface. Samples of atherosclerotic vessels were extracted from iliac artery during shunting surgery.
Abbreviations: AFM, atomic force microscopy; MFM, magnetic force microscopy; BMNPs, biogenic magnetic nanoparticles.
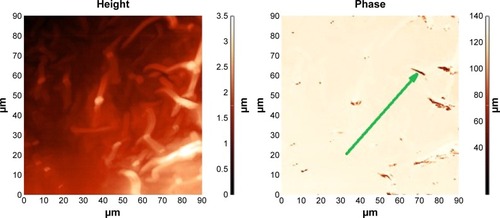
BMNPs in human disease
The problem of the physiological origin of BMNPs gains in importance in light of the discovery of BMNPs in a number of human organs and tissuesCitation61,Citation91 and their observed relation to neurodegenerative disorders,Citation16,Citation17,Citation19,Citation67 cancer (),Citation20,Citation21 and atherosclerosis ().Citation92 Concentrations of BMNPs in inflammation zones in neurodegenerative diseasesCitation93–Citation97 and cancerCitation8,Citation20 are higher than those observed in normal tissues.
Therefore, normal iron homeostasis is disrupted in the brain in many neurodegenerative diseases.Citation20 BMNPs (magnetite and maghemite) are detected in senile plaques and tau filaments in brain tissue affected by neurological and neurodegenerative diseases, such as epilepsy and Alzheimer’s disease (AD).Citation19,Citation66,Citation98 Concentrations of magnetite are significantly higher in samples of AD tissue than in healthy tissue.Citation19 The total concentration of biogenic magnetite is 15 times greater in the AD brain than controls in some cases.Citation16 Magnetite NPs are present in amyloid-β (Aβ) plaque cores, and are directly bound into fibrillar Aβ.Citation99 Magnetite NPs have been found to be directly associated with AD plaques and tangles.Citation66,Citation89,Citation99,Citation100 In vitro experimental data show that magnetite enhances the toxicity of Aβ.Citation101
BMNPs have been detected in various human tumor tissues, including melanoma, breast, ovary, testicle, sarcoma, meningioma, glioblastoma, astrocytoma, glioma, and metastasis.Citation20 An elevated quantity of BMNPs is observed in tumor samples in comparison with normal ones. For example, human meningioma brain-tumor tissues contain approximately ten times the concentration of BMNPs of nontumor hippocampus.Citation8
Magnetic force microscopy (MFM) reveals BMNPs arranged in chains of various lengths up to several microns long (see the MFM images in and ). Both biogenic and anthropogenic origins of MNPs are found in human tissues.Citation102,Citation103 The presence of BMNPs can lead to increased accumulation of MNPs, due to magnetic dipole–dipole interaction between biogenic and anthropogenic MNPs. This is important in connection with the influence of polluted urban environments on human health.Citation102
Genetic control of biomineralization of BMNPs
Detection of elevated levels of BMNPs in disease raises questions. Is elevated BMNP production the cause or consequence of disease? What genes are controlling this process in humans? In perspective, the answers to these questions would result in new methods of treatment and diagnostics of cancer, atherosclerosis, and neurodegenerative disorders, with application of advances in genetic therapy and more sensitive specialized analytical methods. Besides, revealing the genetic mechanisms of biomineralization of BMNPs in bacteria would allow the spread of applications of bacteria with natural ferrimagnetic properties as vectors for magnetic field-assisted drug delivery systems,Citation104 including non-MTB.Citation105 For example, recent achievements have demonstrated experimental transfection of human mesenchymal stem cells with the MMS6 gene of Magnetospirillum magneticum AMB1 and bioassimilated synthesis of intracytoplasmic MNPs by mammalian cells.Citation106
Biomineralization proteins of MTB
To date, genetic control of the synthesis of BMNPs has only been studied experimentally in MTB,Citation11–Citation15 where it appears to be strictly regulated by the properties and structural organization of the BMNPs.Citation11,Citation12 Most of the magnetosome-associated proteins are encoded in the magnetosome island, in MamGFDC, Mms, and MamAB operons.Citation107 Also, the shape and size of mature magnetite NPs (), precisely defined for each strain of MTB, are a manifestation of genes of the magnetosome island.Citation11,Citation29,Citation30,Citation108
There are two functional classes of proteins of the MTB magnetosome island (): proteins indispensable for the process of biomineralization of BMNPs, and other proteins, including regulatory proteins, which exercise strict genetic control of size distribution, species-specific morphologies, and localization of single-crystal magnetite NPs in the cell of a magnetotactic bacterium and proteins with unknown functions or of no effect on BMNP biomineralization.Citation1,Citation11,Citation12 The loss of the magnetosome island leads to a nonmagnetic phenotype of MTB demonstrating its key role in the biogenesis of BMNPs.Citation11,Citation97,Citation109–Citation113 shows the participation of proteins of a magnetosome island in the biomineralization of BMNPs in a magnetotactic bacterium. The magnetosome vesicle represents a unique pool providing favorable chemical surroundings for magnetite crystal growth. Black hexagonal symbols represent BMNP crystal growth inside magnetosome vesicles (). The magnetosome membrane contains the magnetosome-associated Mam and Mms proteins of the magnetosome island ().
Table 3 Functional classes of proteins encoded by genes in the magnetosome islandCitation26,Citation30,Citation107,Citation109–Citation113
Figure 5 Schematic representation of BMNP biomineralization in magnetotactic bacteria.
Note: Black hexagonal symbols represent magnetic crystal growth inside magnetosome vesicles.
Abbreviation: BMNP, biogenic magnetic nanoparticle.
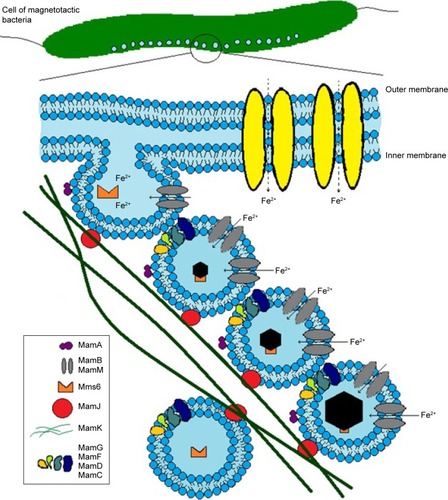
The protein MamA (which is known also as Mms24 or Mam22) takes part in the activation of BMNP biomineralization and formation of magnetosome vesicles.Citation26,Citation110 The proteins MamB and MamM are transporters of Fe2+ and other cations, such as Co2+, Zn2+, and Cd2+. The proteins MamB and MamM take part in the formation of BMNP crystals.Citation11,Citation107,Citation109–Citation113 It has been proved that mutant MTB lacking MamA, MamE, MamO, MamB, MamM, or MamN proteins can form only empty magnetosome vesicles without BMNPs.Citation11,Citation107,Citation109–Citation113 That is why these proteins are indispensable for the process of biomineralization of BMNPs in MTB. The proteins MamK and MamJ take part in the formation of linear chains of BMNPs.Citation11,Citation107,Citation109–Citation114 Proteins indispensable for the process of biomineralization of BMNPs have been studied,Citation26,Citation199,Citation110 and also regulatory proteins of the magnetosome island.Citation107,Citation109,Citation111,Citation112 depicts the biomineralization of BMNPs in MTB and specifies the localization of biomineralization proteins.
Human homologues of MTB proteins involved in BMNP biomineralization
Comparative genomic studies have been carried out in search of common ways of genetic regulation of BMNP biomineralization in both prokaryotes and eukaryotes, including humans.Citation23,Citation115 Sequence alignment of human proteins with the magnetosome-island proteins of bacteria M. gryphiswaldense have shown significant similarities with proteins indispensable for magnetite biomineralization.Citation23 Of the 17 regulatory proteins of the magnetosome island, only one, MamK, responsible for the formation of BMNP chains, has a human homologue.Citation23 This is consistent with the phenotypic manifestations of BMNPs in MTB and in humans. Chains of BMNPs have been observed experimentally in human tissues in MFM studies ( and ). The absence of human homologues of the other regulatory proteins of the magnetosome island in MTB is in agreement with experimental data,Citation20,Citation61 indicating the lack of control of the size, form, and quantity of BMNPs and the absence of magnetosome vesicles in human cells.
A common genetic mechanism of BMNP biomineralization, shared by organisms of all three domains of life and based on homologues of MTB proteins indispensable for BMNP biomineralization, has been predicted by comparative genomic methods ( and ).Citation23,Citation115 Human homologues of MTB proteins indispensable for biomineralization of BMNPs are specified in . provides a functional comparison between MTB proteins indispensable for BMNP biomineralization and their human homologues, based on National Center for Biotechnology Information (NCBI) functional annotation. The Mam proteins and their human homologues are found to have the same known functions and domains.
Table 4 Statistically significant alignment of MTB proteins with human proteins based on BLAST standard parametersCitation23
Table 5 Comparative table of functions of MTB proteins and their human homologuesCitation23,Citation116
Predicted common genetic mechanism of BMNP biomineralization in prokaryotes, archaea, and eukaryotes
Bioinformatic methods allow comparison of a query protein with a specific set of proteins from a database by sequence alignment of amino acid residues. Statistically significant alignments or matches among the sequences compared are used for finding homologues, ie, proteins descending from a common ancestor. Homologues of proteins necessary for BMNP biomineralization in MTB have been found in nonmagnetotactic organisms with intracellular BMNPs by sequence alignment of Mam proteins with proteins from the NCBI database.Citation116 This has provided the basis for a prediction of the biomineralization of intracellular BMNPs in eukaryotes, nonmagnetotactic prokaryotes, and archaea. depicts such a prediction with indicated homologues of MTB proteins indispensable for BMNP biomineralization.
Figure 6 Predicted biomineralization of intracellular BMNPs in eukaryotes, nonmagnetotactic prokaryotes, and archaea.
Note: Black shapes represent the magnetic crystals inside some organic material.
Abbreviation: BMNPs, biogenic magnetic nanoparticles.
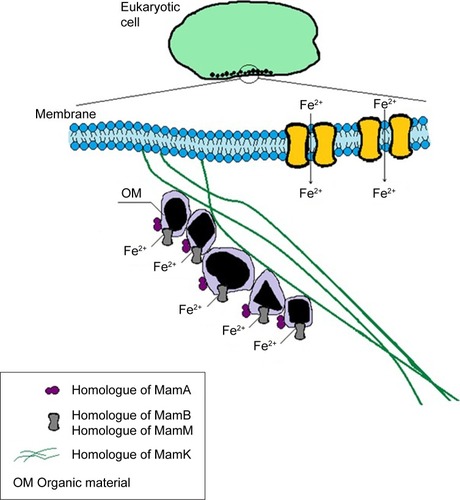
It is predictedCitation23,Citation115 that homologues of indispensable biomineralization proteins of magnetosome islands have analogous functions in control of biomineralization of BMNPs in nonmagnetotactic organisms, including humans (, and ). There is no lipid magnetosome vesicle surrounding BMNPsCitation68 or homologues of proteins responsible for vesicle formation in humans.Citation23,Citation115 That is why it is possible that the favorable chemical surroundings for BMNP growth are provided inside the organic matrix or another type of organic material (), as is observed in non-MTB with BMNPs.Citation39
Not all nonmagnetotactic organisms have a homologue of the MamK protein.Citation116 This is consistent with the experimental observation of BMNPs not arranged in chains.Citation39 However, BMNP chains can form even without MamK. Biogenic and artificial magnetite NPs can arrange in chains also as a result of magnetic dipole–dipole interaction ().Citation117,Citation118 Aggregation of BMNPs, based on magnetic forces alone, can allow the formation of less orderly aggregate assemblies from archaea to human cell systems.
Figure 7 Saccharomyces cerevisiae yeast cells with magnetic nanoparticles.
Note: AFM (left) and MFM image (right), showing chains (arrow) of artificial magnetic nanoparticles formed at the biomembranes of the cells, due to magnetic dipole–dipole interaction among the magnetic nanoparticles.
Abbreviations: AFM, atomic force microscopy; MFM, magnetic force microscopy.
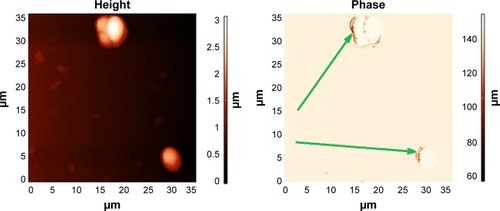
The mineral core of ferritin: a precursor of BMNPs?
A protein that supplies iron in cells,Citation119–Citation121 ferritin has been considered a possible precursor of BMNPs since 1996.Citation22,Citation122,Citation123 A hypothesis has been proposed that the ferrihydrite core of ferritin can be a precursor of magnetite, by analogy to what is observed in MTB, which in an early growth stage produce noncrystalline ferrihydrite, later converted to magnetite, within the magnetosome vesicle.Citation22 However, the relation between ferritin and BMNPs seems indirect, involving the protein shell rather than the mineral core of ferritin, as indicated by its role in neurodegeneration, associated with the presence of MNPs in the human brain.Citation122,Citation124 Though long considered,Citation22 whether the ferritin molecule is the precursor of BMNPs in human tissues has not been established to date. Also, the role of ferritin in the physiological origin of BMNPs from the point of view of the predicted common genetic mechanism of BMNP biomineralization,Citation23 as well as the interaction between ferritin and the basic set of proteins indispensable for BMNP biomineralization, remains an open question.
Basic physicochemical characterization of ferritin
Iron is an element of vital importance to nearly all living organisms. At the same time, it is highly toxic in excess. Free Fe2+ ions are known to produce highly reactive oxygen species that may cause cell damage. Organisms have developed a way of fast scavenging of excess iron ions by storing them in a safe mineral form inside ferritin molecules. Discovered by Laufberger in 1937,Citation125 ferritin has been found since in most living organisms, from microorganisms to plants, invertebrates, and vertebrates, particularly mammals. Covering a broad spectrum of its properties and applications, the literature on ferritin is voluminous. We refer the reader to classicalCitation126,Citation127 and recent review papersCitation128,Citation129 discussing the most important aspects of its physicochemical properties. Herein, we only outline these briefly, along with the methods used for studying the magnetic properties of ferritin.
Ferritins
Ferritin is a hollow spherical protein made up of 24 subunits; iron ions are sequestered in its cavity. The iron core of the protein is described as a mineral with a form similar to that of ferrihydrite, with a stoichiometry of [FeO(OH)]8 [FeO(H2PO4)] and different amounts of phosphate.Citation126,Citation128,Citation129 The empty ferritin shell is known as apoferritin. The protein with iron in the cavity is referred to as holoferritin, or very often simply ferritin.
For decades ferritins, have been considered synonymous with the function of iron storage. The ferritin family includes subfamilies of mammalian, plant, and bacterial ferritins, each composed of 24 subunits. However, in recent years it has become clear that there are also other proteins able to store iron in the form of a mineral core in their central cavity. These include Dps, a subfamily of proteins made up of 12 subunits, often referred to as miniferritins. Another subfamily of protein cages, frataxin is composed of up to 48 subunits. In , we provide the main information on proteins belonging to the ferritin family. It is worthy of note that the Fe:P ratio varies considerably in native ferritin cores. The Fe:P ratio has an influence on the core morphology; however, no general rules have been observed to date.
Table 6 Properties of proteins of the ferritin family
The ferritin nanocage has a 432-point symmetry with three fourfold, four threefold and six twofold axesCitation126,Citation129 (see ). Dps family members have only 32-point symmetry. The maximal number of iron ions that can be stored in the ferritin cavity is 4,500 (500 in Dps). The actual number of iron ions in natural ferritin depends on the organism and the organ in which it is stored. There are two types of monomers among the 24 subunits that constitute the ferritin molecule: the heavy (~21 kDa) subunit, or H-chain, comprising 178 amino acids, and the light (~19 kDa) subunit, or L-chain, of 171 amino acids.
Figure 8 Schematic representation of ferritin molecule with twofold-, threefold-, and fourfold-symmetry axes.
Notes: Dps, PDB – 4DYU (top left); structure of human ferritin L-chain, PDB – 2FG8 (top center); structure of human ferritin L-chain, PDB – 2FFX (top right) (NCBI). The protein-shell diameter is 12 nm, and the core diameter 7–8 nm (right).
Abbreviations: PDB, Protein Data Bank; NCBI, National Center for Biotechnology Information.
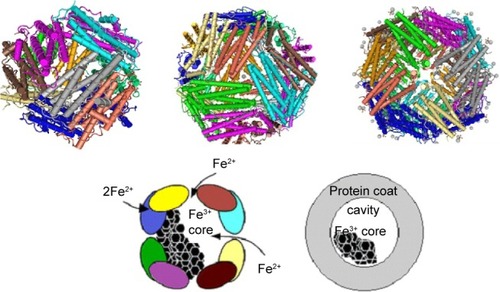
Only one type of subunit, with a mass of 26.5 kDa, has been identified in the plant ferritin phytoferritin.Citation131 However, some authors distinguish its three forms with different molecular weight.Citation131 Some bacteria have a heme group incorporated in their ferritin, which in that case is referred to as bacterioferritin.
The H-chain carries a ferroxidase center, which appears to be essential for iron incorporation, whereas the L-chain facilitates iron mineralization within the cavity. Plant and bacterial ferritins have only a single type of subunit, which probably fulfills both functions. Vertebrate ferritins are heteropolymers that assemble in specific H:L ratios and vary widely with organism and tissue.Citation126,Citation129 The most often studied – horse-spleen ferritin (HSF) – is composed of ~10% of H-chains and ~90% of L-chains. Ferritins rich in L-chains predominate in iron-storage organs, such as spleen and liver, whereas heart cells and a majority of brain cells tend to contain mostly H-chain-rich ferritins. Apparently, the H:L ratio plays an important role in controlling iron concentration in cells; this has not been fully elucidated to date, but may be related to several pathologies observed in humans.Citation124,Citation134
shows transmission electron microscopy (TEM) of well-defined NP crystallites, ie, the core of ferritin of size 3.5–8 nm within holoferritin. The particle-size distribution of ferritin, but also of any MNPs, is very often described by known distribution functions.Citation135 The log-normal distribution function is the most commonly used,Citation136 and allows the calculation of average diameter of the particle and standard deviation ().
Figure 9 (A) Ferritin TEM (HSF), the white stripes inside the black box indicate the scale bar 100 nm; (B) particle-size distribution based on TEM.
Abbreviations: TEM, transmission electron microscopy; HSF, horse-spleen ferritin; SD, standard deviation.
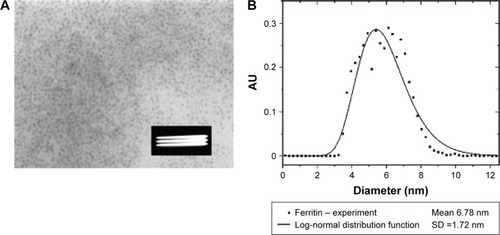
The ferritin core is antiferromagneticCitation137 with a Néel temperature of 240–460 K, but not uniquely determined.Citation138 The magnetization of the core is about two orders of magnitude lower than that of magnetiteCitation84 (see ). The magnetic moment of ferritin ranges from 100 μB to 300 μB, while the magnetic moment of magnetite of similar dimensions is of the order of 104 μB.Citation139 The superantiferromagnetism of ferritin NPs has been investigated recently by a combination of low-field susceptibility and magnetization measurements, and full-saturation magnetization has proved unattainable even in a magnetic field as high as 55 T.Citation140,Citation141
HSF tends to be regarded as a model system, and has been studied by several techniques, specified in . However, the physicochemical properties of human isoferritins have not been sufficiently studied to date, and require further investigations and correlation with human health data.
Table 7 Methods for study of magnetic properties of biogenic and synthetic ferritin and BMNPs
Today, ferritin is a powerful clinical tool,Citation121 but the magnetic structure of its core has not yet been explored by clinicians. It is noteworthy that a number of currently available techniques allow the study of the magnetic properties of the core of ferritin of any origin. These include X-ray absorption near-edge structure (XANES) spectroscopy, Mössbauer spectroscopy, MFM, and magneto-optical spectroscopy (see ).
Some of the methods specified in can have clinical analytical applications. Magneto-optical spectroscopy seems a very good candidate, as it is not too sophisticated, relatively inexpensive, and can be used for studying liquid and thin-tissue samples. It is important to note that recently a rapid detection method for malaria diagnosis based on a magneto-optical method (Cotton–Mouton effect) was presented and tested.Citation163,Citation164 Magneto-optical relaxation methods are successfully used in controlling the functionalization of magnetic particles through measurements of size of prepared molecules.Citation165
Elevated ferritin levels are associated with inflammatory conditions, including malignancy.Citation166 In plasma, which normally contains mostly L-chain-rich ferritin, increased concentrations of H-chain-rich ferritin are observed in some pathological conditions.Citation167 Also, pathological ferritin is observed in a number of human diseases.Citation133,Citation168,Citation169 The interest in the chemical composition of both physiological and pathological ferritin is stimulated also by the hypothesis that the magnetic core of ferritin and aggregates of pathological ferritin might be a precursor of BMNPs.Citation62 A large number of papers have been aimed at verification of the idea that biogenic magnetite in humans might originate from ferritin, and the determination of the role of ferritin and BMNPs in the pathogenesis of diseases.Citation100,Citation122,Citation124,Citation169,Citation170 We would like to point out the environmental pollution by radiofrequency electromagnetic fields used in mobile telephony throughout the world and the long-term exposure of those who use mobile phones. There are very few papers devoted to the possible biological effects of this radiofrequency field studied at the molecular level, though the problem has at least been discussed by Céspedes et al.Citation171,Citation172 They found that exposure of about 2 hours to a magnetic field of 30 μT at 1 MHz on ferritin have reduced their iron intake rate by about 20%.
Hemosiderin
The literature devoted to hemosiderin is strongly correlated with ferritin; however few studies on hemosiderin alone have been undertaken,Citation100,Citation173–Citation177 although it is thought that hemosiderin may play an important role directly or indirectly in iron cytotoxicity and thus deserves more intensive study. The hemosiderin aggregates found in diseases might play a similar role as ferritin doses as precursors of BMNPs; however, no direct evidence for this is known.
Water-insoluble tissue iron deposits are known as hemosiderin. Hemosiderin is thought to be a breakdown product of ferritin, and is much less soluble than ferritin. In several iron-overload diseases (eg, thalassemia or hemochromatosis) iron oxyhydroxide particles are mostly found in the form of insoluble aggregates associated with protein residues.Citation173 Hemosiderin mineral particles vary even more widely in structure than those stored in ferritin.Citation174 Allen et al identified three main particle structures in hemosiderin: a ferrihydrite-based structure, a highly defective structure based on goethite (α-FeOOH), and a noncrystalline Fe(III) structure.Citation174 The same authors found that hemosiderin particles generally have larger size distributions than particles in ferritins. As in the case of ferritins, the structural and compositional characteristics of hemosiderin vary with biological source and pathological condition.Citation174 Miayazaki et al established that denatured H-chain-rich ferritin is a major constituent of hemosiderin in the liver of patients with iron overload.Citation175
Mutant ferritins
Genetic engineering has been mostly used for producing ferritin from a specific chain, eg, the H-chainCitation178 or frog M ferritin.Citation179 Spectacular results obtained by replacing a particular amino acid in the H- or L-chain shed light on the entry of Fe(II) into the ferritin cavity, its pathway to the center, oxidation, and formation of Fe(III). Detailed information on this procedure can be found in a very recent review by Ebrahimi et al.Citation128 Some mutations of human ferritin in vivo may be the origin of severe malignancy; this problem is discussed further in a following section.
Synthetic ferritins
As demonstrated by Meldrum et al,Citation180 apoferritin can be used in vitro as a bioreactor for producing monodisperse metal or metal oxide NPs. In particular, magnetite and/or maghemite have been synthesized in vitro inside apoferritin to produce a novel material known as magnetoferritin. Further development of this biomimetic way of nanosynthesis within the ferritin cavityCitation181,Citation182 has allowed the production of ferritin with various cores, as illustrated in . In general, HSF is used as the starting material for preparation of apoferritin, which is used as a nanoreactor. In special cases, genetically modified apoferritin is also used. Homochains, ie, only L-chains or H-chains, were synthesized to follow iron entry and its mineralization process in ferritin.Citation128 H-chain magnetoferritin was used for enhanced magnetic resonance imaging (MRI).Citation178 Worthy of note are also other considered approaches based on the disassembly of the apoferritin shell and its reassembly in the presence of the species to be encapsulated.Citation183–Citation185 Besides the possible applications of synthetic ferritin in electronicsCitation186 and biomedicine,Citation119,Citation120 the interest in this unique material has been stimulated also by its role as the artificial modeling object for the development of methods for the determination and study of the physical and chemical properties of the hypothetical precursor of BMNPs.Citation139,Citation160
The magnetic core of mutant ferritin as a precursor of BMNPs
Pathological ferritin is observed in a number of diseases, including neuroferritinopathy (NF), a rare genetic disorder resulting directly from a known mutation in the gene encoding the ferritin light polypeptide (L-subunit).Citation134,Citation170 A slowly progressive adult-onset disease, NF is characterized by the aggregation of ferritin clusters in the brain and other organs.Citation187 It has been shown to provide direct evidence that changes in the ferritin structure resulting in inefficient iron storage can lead to neurodegeneration, with damage to neural cells probably caused by an excess of labile iron, rather than by iron or ferritin aggregates.Citation168 Oxidative stress injury may be caused by labile iron available for the Fenton reaction. This may be related to a decreased ability of ferritin to retain iron within its core.Citation124,Citation169 Iron leakage from ferritin is observed also in Parkinson’s disease. However, in this condition there is a pathological decrease in the concentration of ferritin L-subunits, while in NF a genetically induced mutation in the L-subunit of ferritin results in its loss of function.Citation124 Oxidative stress is considered one of the pathways leading to neuronal cell death in neurodegenerative diseases. A number of studies have aimed to assess the possible role of iron in this process, but no consensus has been reached. We refer the reader to recent papers of Collingwood et alCitation188,Citation189 for details.
As mentioned earlier, a number of authors have considered the biomineralization of the ferrihydrite core of ferritin a possible pathway for the formation of larger magnetite particles.Citation19,Citation62,Citation76,Citation148,Citation190–Citation192 The structure of nanocrystals in the cores of physiological horse spleen, human liver, and human brain ferritin and pathological human brain ferritin of patients with progressive supranuclear palsy and AD has been studied by electron nanodiffraction and high-resolution TEM.Citation62 A polyphase structure of the ferritin core has been postulated, but not unequivocally confirmed to date.Citation147,Citation193 There are significant differences in the mineral composition between physiological and pathological ferritins. Although both physiological and pathological ferritin cores have a poly-phase composition, hexagonal ferric iron oxide (ferrihydrite) predominates in physiological ferritin cores, while the major phases in AD brain ferritin cores are two cubic mixed ferric–ferrous iron oxides (magnetite and wüstite).Citation62,Citation100,Citation148
HSFs from which iron had been gradually removed, yielding samples containing 200, 500, 1,200, and 2,200 iron atoms, were studied by TEM, XANES spectroscopy, electron energy-loss spectroscopy, small-angle X-ray scattering, and superconducting quantum-interference device (SQUID) magnetometry.Citation154 The relative amount of magnetite in fer-ritin containing 200–2,200 iron atoms rose steadily from approximately 20% to approximately 70%, whereas that of ferrihydrite fell from approximately 60% to approximately 20%. These results indicate a ferrihydrite–magnetite core–shell structure.Citation154
However, these data may be questionable, as the mineral ferrihydrite is electron beam-sensitive and will undergo internal atomic rearrangements when exposed to the electron beam in TEM and scanning TEM studies, or studied by other techniques using electron beams.Citation147,Citation193 Results obtained by nuclear magnetic resonance (NMR) relaxometry indicate that the proportion of iron contained in brain ferritin in the form of well-crystallized magnetite, rather than ferrihydrite, must be <1%,Citation149 much less than the reported percentages in the dozens of particles of a “magnetite-like” phase found in TEM studies of similar samples.Citation62,Citation154 Consequently, the magnetization of this “magnetite-like” phase must be very low compared with that of magnetite.Citation149 Also, recent magneto- optical and NMR studies of reconstructed and reduced HSF in aqueous solution, presented at the Tenth International Conference on the Scientific and Clinical Applications of Magnetic Carriers, indicate that high-magnetization minerals, such as magnetite or maghemite, are not present in the core of such ferritins.Citation194
Search for magnetoferritin in normal organs containing BMNPs
The hypothesis of the presence of a magnetically strong phase in pathological ferritin has inspired a number of experimental studies in which magnetoferritin, synthetic ferritin with a magnetic core, has been produced and investigated. However, to our best knowledge, the presence of magnetoferritin in specific human organs, serum, or cerebrospinal fluid has not been reported to date.
Various MRI methods for the estimation of iron levels in the human brainCitation195–Citation200 are the most promising in vivo techniques. Also, SQUID susceptometry is being developed for the assessment of iron content in human tissues.Citation201 Of particular interest is the search for methods for the detection of magnetite in ferritin both in vitro and in vivo. Koralewski et al proposed a magneto-optical method based on the measurement of magnetic linear birefringence (MLB; Cotton–Mouton effect) followed by that of magnetic circular birefringence dispersion (Faraday rotation dispersion, or magnetic optical rotatory dispersion [MORD]) for the discrimination of the core mineral in magnetoferritin or any other MNP.Citation139,Citation157–Citation160 The method is depicted in . First, MLB is measured, which allows the classification of unknown samples to a magnetically strong material, eg, magnetite or maghemite, or a magnetically weak one, eg, ferrihydrite or akaganeite (see ). MLB dependence on magnetic field is very characteristic for such classes of materials, as is shown in . For magnetically strong materials, we observe saturation of MLB, but for magnetically weak materials, typical parabolic dependence on H is observed, ie, Δn~HCitation2. Next, MORD measurements should be performed to obtain spectra of the studied sample. Features of MORD spectra are quite different for Fe3+ or Fe2+ ions present in studied magnetic materials (see ), so information on the oxidation state of Fe will be acquired. Taking into account results from both methods, discrimination of minerals for particular MNPs may be obtained.
Figure 11 Schematic representation of discrimination.
Notes: Based on differences in magnetic linear birefringence and MORD spectra between ferrite (magnetically strong) materials, such as magnetite/maghemite, and antiferromagnetic (magnetically weak) materials, such as ferrihydrite or akaganeite. For details of magnetically strong MORD data, see and Koralewski et al.Citation159 Magnetically weak MORD data were obtained for akaganeite-type materials, the same as were studied in Koralewski et al.Citation160
Abbreviations: MORD, magnetic optical rotatory dispersion; CCM, Cotton-Mouton constant; V, Verdet constant; D, particle diameter.
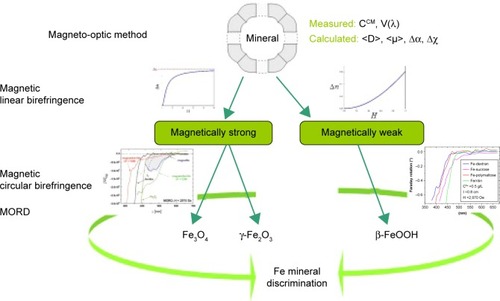
Koralewski et al studied the MLB of biogenic HSF and synthetic magnetoferritin.Citation139,Citation160 Direct observation in a relatively low magnetic field has shown a saturation effect in artificial magnetoferritin, but not in HSF.Citation139,Citation158,Citation160 As may be seen in , distinguishing between magnetically strong and weak materials is obvious; however, discrimination between magnetite or maghemite cores of magnetoferritin is not achieved with this single method.
Figure 12 Reduced linear magnetic birefringence.
Notes: HSF, synthetic magnetoferritin (loading factor 1,250), magnetite, and a mixture of HSF and magnetite (Fe weight proportion 23:1) versus the square of the applied magnetic field – HCitation2. Dashed line indicates the low-field region in which the Cotton–Mouton (CCM) constant was determined (ie, Δn=CCMλHCitation2, where λ is light wavelength and H magnetic field intensity); solid line represents the best fit for the Langevin function. Reprinted from J Magn Magn Mater. Vol 323. Koralewski M, Pochylski M, Mitroova Z, Timko M, Kopcansky P, Melnıkova L. Magnetic birefringence of natural and synthetic ferritin. Pages 2413–2417. Copyright 2011, with permission from Elsevier.Citation139
Abbreviation: HSF, horse-spleen ferritin.
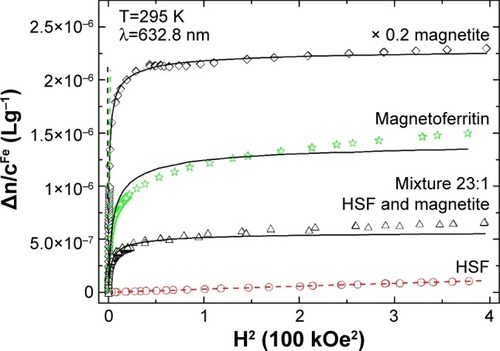
Further information on the magnetic core and oxidation state of Fe can be acquired from Faraday-effect dispersion studies.Citation159 Characteristic bands of 450–478 nm are associated with magnetite NPs and magnetoferritin with a magnetite core. Observed in the visible spectrum, this feature is directly related to Fe2+ ions, which indicate the presence of magnetite in the studied suspension. It should be noted that Fe2+ ion is by definition absent in maghemite. The lack of these bands indicates a mineral other than magnetite (see ).Citation159 The characteristic band intensity may be correlated with the amount of magnetite in the studied suspension. In , magnetoferritin with low iron content (loading factor 1,250; the same material presented in showing saturation of MLB) shows a very low intensity in the region of Fe2+ ion bands. This means that the core of this magnetoferritin consists mainly of maghemite, with a very small amount of magnetite, in contrast to highly loaded magnetoferritin (loading factor 3,250), in which magnetite is the major component of the core.
Figure 13 Wavelength dependence.
Notes: Faraday rotation (MORD) for HSF, synthetic magnetoferritin with different loading factors (loading factor 1,250 and 3,280), Fe2O3, and magnetite suspensions. Reprinted from Koralewski M, Kłos JW, Baranowski M, et al. The Faraday effect of natural and artificial ferritins. Nanotechnology. 2012;23:355704.Citation159 Copyright 2012. IOP Publishing Ltd.
Abbreviations: MORD, magnetic optical rotatory dispersion; HSF, horse-spleen ferritin; LF, loading factor.
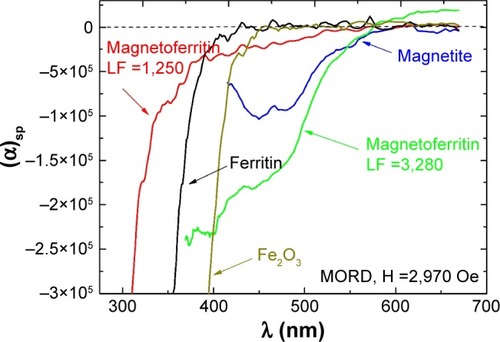
Among the methods indicated in , Raman spectroscopy is also a very promising tool for discrimination among different iron minerals.Citation202 Both solutions and tissue samples have been studied by this technique; we refer the reader to the latest review papersCitation203,Citation204 for details of these studies in biological materials. Also, the recently developed MFM may be very useful in clinical analysis of biomaterials (see and ).Citation21,Citation92,Citation150,Citation151
Correlation between BMNP and ferritin distribution in human tissues
Several studies have been carried out to investigate the possible correlation between the levels of ferritin and BMNPs for the purpose of elucidating the role of ferritin in the process of BMNP biomineralization. However, elevated BMNP levels, associated with various diseases, are not necessarily correlated with increased levels of iron-storage or -transport proteins (ferritin or transferrin, respectively).Citation20,Citation166 Magnetic materials in various human tumor tissues, including melanoma, breast, ovarian and testicle tumors, sarcoma, meningioma, glioblastoma, astrocytoma, glioma, and metastasis, were first detected by Kobayashi et al in a study using SQUID magnetometry.Citation20 However, it was impossible to demonstrate a covariance between ferritin and magnetite in that study. Investigations by magnetic methods reported by Brem et alCitation8 showed that the concentration of ferrimagnetic particles in human meningioma brain-tumor tissues was an order of magnitude higher than in nontumor hippocampus. In a comparative study of ferritin and magnetite distributions in the human brain, it has been established that magnetite is distributed homogeneously throughout the whole brain, except for the meninges, but ferritin is not.Citation90 These results suggest that magnetite in the human brain and tumor tissues does not originate from ferritin;Citation20 however, further experimental documentation is necessary to confirm this conclusion.
Comparison between size distributions of clumps of biogenic magnetite and ferritin aggregates
Measurements of the size distribution of BMNPs and aggregates of pathological ferritin do not confirm the hypothesis that ferritin might be a precursor of BMNPs either.Citation170,Citation205 Biogenic magnetite observed in brain tissuesCitation97,Citation192,Citation206,Citation207 has a wide particle-size range, including both SPM and larger magnetite particles (clump magnetite) with a size of 10–200 nm. Larger particles were observed in tissue extracts; optical microscopy studies revealed 5–10 μm clusters of finer opaque particles in tissue slices.Citation205 The magnetite-clump level measured in NF tissue was 57 and 128 ng/g wet tissue, higher than that normally measured in age/sex-matched freeze-dried control tissues from the same region of the brain (12–27 ng/g wet tissue).Citation170 The observed nontrivial magnetization corresponds to clumps of magnetite with a coercivity larger than 200 Oe, which is consistent with the results of isothermal remnant-magnetization measurements, indicative of magnetite clumps.Citation170 Also coercivity data reported by Hautot et alCitation170 and TEM imaging of magnetic extracts from hippocampal tissueCitation205 indicate the presence of larger clumps of magnetite particles. Moreover, the ferrihydrite core of ferritin is superantiferromagnetic,Citation137 and the saturation magnetization of ferritin proves unattainable, even in a field as strong as 550 kOe.Citation140,Citation141 Therefore, direct formation of BMNPs from the ferrihydrite core of ferritin is impossible. Also the origin of large clumps of magnetite crystals (BMNPs) remains unclear, according to Hautot et al,Citation170 because of the inconsistency in size distribution and magnetic properties between clumps of biogenic magnetite and ferritin aggregates.
Biological formation of BMNPs in vitro
There are a number of papers on the influence of MTB proteins involved in BMNP biomineralization on the formation of magnetite nanocrystals in vitro.Citation208,Citation209 For example, the magnetosome-membrane protein Mms6 has allowed the artificial control of the morphology, crystallinity, and magnetism of nanocrystals in a manner observed in MTB.Citation209–Citation211 To investigate the conditions of biological formation of magnetite nanocrystals, Prozorov et al precipitated iron oxide in an aqueous viscous Pluronic solution of Fe(II) and Fe(III) by increasing the pH in the presence of various proteins.Citation208 A protein isolated from magnetosome membranes mediated the formation of 30 nm-large magnetite crystals with good crystallinity; NPs formed within ferritin under the same conditions were much smaller and largely amorphousCitation208 (NP growth within the ferritin core is limited by the protein shell; see ). Nanocrystals formed in the presence of ferritin do not have uniform sizes and shapes, in contrast to those produced in the presence of the biomineralization protein Mms6.Citation208 A protein assisting magnetite production, MamP has been established recentlyCitation212 as an iron oxidase contributing to the formation of Fe(III) ferrihydrite. As such, this protein will be the next candidate for in vitro testing.
Genetic analysis of role of ferritin in BMNP biomineralization
The elucidation of the physiological origin of BMNPs in eukaryotes, including humans, is still more complex because of the difficulties in the detection of BMNPs in human tissues. Finding magnetite in tissue is a nontrivial problem, due to its low concentrations, of the order of dozens of ng/g tissue. A powerful tool in this field is provided by bioinformatic methods based on comparative genomics. These bioinformatic methods are complementary to physical methods. The sophisticated physical methods for BMNP detection () do not always allow the distinction of BMNPs from artificial MNPs in human tissues in contact with the environment. Also, by using only physical methods, it is often difficult to distinguish extracellular biomineralization of MNPs by microorganisms from their biosorption from the environment.Citation116
As we have mentioned, the genetic mechanism of BMNP biomineralization is predicted to be based on homologues of MTB magnetosome-island proteins indispensable for the biomineralization of BMNPs in MTB.Citation23,Citation213,Citation214 However, the question remains open as to whether ferritin also, together with homologues of MTB magnetosome-island proteins, is involved in the metabolic pathway of BMNP biomineralization. An answer has been proposedCitation214 on the basis of comparative genomic studies. To this end, ferritin-encoding genes were searched for full genomes of MTB.Citation108,Citation113,Citation215–Citation218 Gorobets et alCitation214 demonstrated that the magnetotactic bacterium Magnetococcus marinus MC1 had no genes encoding ferritin or ferritin-like proteins in their full genomes.
ResearchCitation214 has led to the conclusion that the presence of ferritin in cells of MTB is not necessary for the biomineralization of BMNPs, that ferrihydrite in ferritin is not a precursor of biogenic magnetite in MTB, and that prokaryotes and eukaryotes have a common genetic mechanism of biomineralization of intracellular BMNPs.Citation23,Citation213,Citation214 In general, ferrihydrite in the ferritin core is not a precursor of BMNPs in humans or other multicellular organisms. As mentioned, this has been confirmed by an experimental study of conditions of magnetite biomineralization in vitro.Citation208
These findings of bioinformatic studies are in accordance with the aforementioned experimental data on the lack of correlation between BMNP and ferritin distributions in human tissues, and the inconsistency in size distribution between clumps of biogenic magnetite and ferritin aggregates. In this context, the bioinformatic analysis in the paperCitation214 proves that the biomineralization of BMNPs is in general a process independent of iron storage in the core of ferritin and ferritin-like proteins. However, in our opinion, an excess of labile iron related to the decreased ability of ferritin to retain iron within its core in pathological conditionsCitation123,Citation169 may represent an additional iron supply for BMNP biomineralization. Besides, the biomineralization of BMNPs can protect tissues against oxidative stress, since iron in magnetite is bound irreversibly and cannot be released for the Fenton reaction.Citation219 In this regard, iron storage in organisms can be classified as either reversible (in iron-storage proteins) or irreversible (in BMNPs).
Metals accumulated in AD and other neurodegenerative disorders are known to include (besides iron) such elements as copper or zinc.Citation220,Citation221 The accumulation of copper and zinc can be explained by a common genetic mechanism of BMNP biomineralization, since the proteins indispensable for BMNP biomineralization include divalent cation (Zn+2, Cu+2, Cd+2, Ni+2, and Fe+2) transporters. Therefore, Co, Ni, Zn, Cu, Mn, and Cd can be incorporated in the magnetite structureCitation211,Citation222 and bound irreversibly in BMNP biomineralization. On the other hand, purified ferritin cages in vitro can incorporate a variety of metal ions (Fe, Au, Pd, Rh, Pt, Ni, Cr, Cd, Ti, Tb, Co, Cu, and Zn), most likely through a hydrophilic threefold ion channel.Citation183,Citation223,Citation224 Therefore, both ferritin and BMNPs might contribute to the excess of zinc and copper in neurodegenerative disorders.Citation225
Conclusion and prospects
In this review, we have outlined the research efforts toward understanding the biomineralization of BMNPs and especially their role in human health and disease. In general, three basic steps can be distinguished in BMNP biomineralization in organisms of all three domains (prokaryotes, archaea, and eukaryotes, including humans): 1) formation of a specific organic material, organic matrix, or vesicle to prepare a chemical medium favorable for BMNP biomineralization; 2) formation of a transient iron mineral; and 3) conversion of the transient iron-mineral precursor into the target BMNP mineral.
Each of these steps requires the participation of a set of specific proteins. The formation of an organic matrix or other organic surroundings of BMNPs involves proteins that are not homologues of those controlling the formation of vesicles in MTB.Citation23,Citation115 Steps 2 and 3 are predicted to be controlled by homologues of MTB biomineralization proteins.Citation23,Citation115
Ferrihydrite is the most probable transient mineral and precursor of biogenic magnetite.Citation9,Citation214 However, the accumulation of iron in BMNP biomineralization and the accumulation of iron in the ferrihydrite core of ferritin are independent processes in general.Citation9,Citation214 The role of ferritin in BMNP biomineralization remains an open question. In diseases and mutations, an increase in labile iron due to the reduced ability of ferritin to retain iron within its core can influence the process of biomineralization of BMNPs. There are two modes of iron storage in living organisms: reversible storage in iron-storage proteins, and irreversible storage in BMNPs, in which iron is bound in magnetite or other chemically resistant iron oxides and cannot be released. Since ferrites represent the most magnetically sensitive compounds of biogenic origin and elevated BMNP levels are related to a number of human diseases, such as cancer, neurodegenerative disorders, or atherosclerosis, the study of the influence of magnetic fields on the expression of genes encoding biomineralization proteinsCitation226,Citation227 is of key importance in the investigation of the influence of magnetic fields on living organisms.
The discovery of BMNPs in organisms of all three domains – prokaryotes, archaea, and eukaryotes – and the observed peculiarities of their formation in health and disease have prompted the investigation of their metabolic functions. Controlled application of weak static magnetic fields may cause rotation of SD magnetite, which may result in the opening of ionic membrane channels, modulation of the transmembrane potential, and/or generation of action potentials in neurons.Citation48,Citation228 Simulations performed by Jandacka et alCitation229 show that SPM ferritin molecules cannot be deformed or rotated in weak geomagnetic fields, and thus cannot be involved in magnetoreception by deformation. Furthermore, there is an alternative hypothesis that ferritin molecules in the avian ear may function as intracellular electromagnetic oscillators, generating additional electrical fields with an amplitude of ~0.1 pV in receptor cells and consequently auditory neurons.Citation229 However, we think that a voltage of 0.1 pV is too low to produce any significant biological effect, since the working membrane voltages are of the order of dozens of millivolts. There is also a hypothesis that BMNP chains represent nanoscale high-gradient magnetic separators of cluster components (eg, vesicles, granules, cells) in organisms.Citation229–Citation231
We hope that further development of physical methods for the discrimination of the core minerals in ferritin in physiological fluids and/or tissue will provide powerful tools to fight neurodegenerative disorders. In this regard, we think that the magnetism of the ferritin core may be a useful prognostic indicator, especially in some neurodegenerative diseases. Better understanding of core mineral content and its composition and also tissue–organ distribution of ferritin will certainly help in clarifying the etiology of several diseases. This calls for an intensification of research into the magnetic properties of ferritin from different organs (see ) from healthy and pathological human subjects. The mapping of ferritin and BMNP in different organs and especially the brain would be very important, and not only from the clinical analytical point of view. Such studies may shed new light on long-term memory in which it is believed magnetite can be involved.Citation232,Citation233 Recent studies by the Kirschvink groupCitation234 on possible human magnetic sensing await further proof, and many questions remain unanswered.
Acknowledgments
We appreciate the reviewer’s comments, which improved our manuscript. This project received funding from the European Union’s Horizon 2020 Research and Innovation program under a Marie Skłodowska-Curie grant (agreement 644348 [MagIC]). We would like to thank M Molcan (SAS Košice) for growing MTB and B Leszczyński (Adam Mickiewicz University, Poznań) for providing TEM of MTB.
Disclosure
The authors report no conflicts of interest in this work.
References
- MeldrumFCCölfenHControlling mineral morphologies and structures in biological and synthetic systemsChem Rev20081084332443219006397
- EherlichHBiomineralization special issueActa Biomater2014103813381424932770
- MannSBiomineralization: Principles and Concepts in Bioorganic Materials ChemistryNew YorkOxford University Press2001
- BlakemoreRMagnetotactic bacteriaScience1975190377379170679
- FrankelRBBlakemoreRPWolfeRSMagnetite in freshwater magnetotactic bacteriaScience19792031355135617780480
- SakaguchiTBurgessJGMatsunagaTMagnetite formation by a sulphate-reducing bacteriumNature19933654749
- MannSSparksNHFrankelRBBazylinskiDAJannaschHWBiomineralization of ferrimagnetic greigite (Fe3S4) and iron pyrite (FeS2) in a magnetotactic bacteriumNature1990343258261
- BremFHirtAMWinklhoferMMagnetic iron compounds in the human brain: a comparison of tumor and hippocampal tissueJ R Soc Interface2006383384117015303
- FaivreDGodecTUFrom bacteria to mollusks: the principles underlying the biomineralization of iron oxide materialsAngew Chem Int Ed Engl2015544728474725851816
- QinSYYinHYangCLA magnetic protein biocompassNat Mater20161521722626569474
- RichterMKubeMBazylinskiDAComparative genome analysis of four magnetotactic bacteria reveals a complex set of group-specific genes implicated in magnetosome biomineralization and functionJ Bacteriol20071894899491017449609
- AbreuFCantãoMENicolásMFCommon ancestry of iron oxide- and iron-sulfide-based biomineralization in magnetotactic bacteriaISME J201151634164021509043
- KomeiliALiZNewmanDKJensenGJMagnetosomes are cell membrane invaginations organized by the action-like protein MamKScience200631124224516373532
- KomeiliAMolecular mechanisms of magnetosome formationAnnu Rev Biochem20077635136617371202
- KomeiliAMolecular mechanisms of compartmentalization and biomineralization in magnetotactic bacteriaFEMS Microbiol Rev20123623225522092030
- PankhurstQHautotDKhanNDobsonJIncreased levels of magnetic iron compounds in Alzheimer’s diseaseJ Alzheimers Dis200813495218334756
- BremFHirtAMSimonCWieserHGDobsonJCharacterization of iron compounds in tumour tissue from temporal lobe epilepsy patients using low temperature magnetic methodsBiometals20051819119715954745
- BartzokisGTishlerTALuPHBrain ferritin iron may influence age- and gender-related risk of neurodegenerationNeurobiol Aging20072841442316563566
- HautotDPankhurstQAKhanNDobsonJPreliminary evaluation of nanoscale biogenic magnetite and Alzheimer’s diseaseProc Biol Sci2003270S62S6412952638
- KobayashiAYamamotoNKirschvinkJStudies of inorganic crystals in biological tissue: magnetite in human tumorJ Jpn Soc Powder Powder Metall199744294300
- ChekchunVFGorobetsSGorobetsODemianenkoIVMagnet-sensitive nanostructures of endogenous origin in Ehrlich carcinoma cellsNanostruct Mater20112102109
- KobayashiAYamamotoNKirschvinkJStudy of inorganic crystalline solids in biosystems: magnetite in the human bodyJ Soc Powder and Powder Metall19964313541360
- GorobetsOGorobetsSGorobetsYBiogenic magnetic nanoparticles: biomineralization in prokaryotes and eukaryotesLyshevskiSEDekker Encyclopedia of Nanoscience and Nanotechnology3rd edNew YorkCRC Press2014300308
- GrünbergKMüllerEOttoABiochemical and proteomic analysis of the magnetosome membrane in Magnetospirillum gryphiswaldenseAppl Environ Microbiol2004701040105014766587
- ArakakiANakazawaHNemotoMMoriTMatsunagaTFormation of magnetite by bacteria and its applicationJ R Soc Interface2008597799918559314
- KomeiliAValiHBeveridgeTJMagnetosome vesicles are present before magnetite formation, and MamA is required for their activationProc Natl Acad Sci U S A20041013839384415004275
- FukumoriYOyanagiHYoshimatsuKEnzymatic iron oxidation and reduction in magnetite synthesizing Magnetospirillum magnetotacticumJ Phys IV France19977659662
- ProzorovTMagnetic microbes: bacterial magnetite biomineralizationSemin Cell Dev Biol201546364326382301
- DevouardBPósfaiMHuaXBazylinskiDAFrankelRBBuseckPRMagnetite from magnetotactic bacteria: size distributions and twiningAm Mineralogist19988313871398
- TanakaMMazugamaEAvahakiAMatsunagaTMMS6 protein regulates crystal morphology during nano-sized magnetite biomineralization in vivoJ Biol Chem20112866386639221169637
- NareshMHasijaVSharmaMMittalASynthesis of cellular organelles containing nano-magnets stunts growth of magnetotactic bacteriaJ Nanosci Nanotechnol2010104135414421128392
- Thomas-KeprtaKLBazylinskiDAKirschvinkJLElongated prismatic magnetite crystals in ALH84001 carbonate globules: potential Martian magnetofossilsGeochim Cosmochim Acta2000644049408111543573
- HsuCYKoFYLiCWFannKLueJTMagnetoreception system in honeybees (Apis mellifera)PLoS One20074e395
- MaherBAMagnetite biomineralization in termitesProc Biol Sci1998265733737
- CranfieldCGDaweAKarloukovskiVDunin-BorkowskiREde PomeraiDDobsonJBiogenic magnetite in the nematode Caenorhabditis elegansProc Biol Sci2004271436439
- MannSSparksNHWalkerMMKirschvinkJLUltrastructure, morphology and organization of biogenic magnetite from sockeye salmon, Oncorhynchus nerka: implications for magnetoreceptionJ Exp Biol198814035493204335
- WiltschkoRWiltschkoWMagnetic Orientation in AnimalsBerlinSpringer1995
- WalcottCGouldJLKirschvinkJLPigeons have magnetsScience1979184180182
- VainshteinMSuzinaNKudryashovaEAriskinaENew magnet-sensitive structures in bacterial and archaeal cellsBiol Cell200294293512000144
- Gajdardziska-JosifovskaMMcCleanRGSchofieldMASommerCVKeanWFDiscovery of nanocrystalline botanical magnetiteEur J Mineral200113863870
- de BarrosHGEsquivelDMDanonJde OliveiraLPMagnetotactic algaeAn Acad Bras Cienc198254257258
- SuzukiaYKoppREKogureTSclerite formation in the hydrothermal-vent “scaly-foot” gastropod: possible control of iron sulfide biomineralization by the animalEarth Planet Sci Lett20062423950
- de OliveiraJFWajnbergEEsquivelDMWeinkaufSWinklhoferMHanzlikMAnt antennae: are they sites for magnetoreception?J R Soc Interface2010714315219474081
- GouldJLKirschvinkJLDeffeyesKSBees have magnetic remanenceScience197820210261028
- Acosta-AvalosDWajnbergEOliveiraPSLealIFarinaMEsquivelDMIsolation of magnetic nanoparticles from Pachycondyla marginata antsJ Exp Biol19992022687269210482727
- LohmannKJMagnetic remanence in the western Atlantic spiny lobster, Panulirus argusJ Exp Biol19841132941
- BrassartJKirschvinkJLPhillipsJBBorlandSCFerromagnetic material in the eastern red-spotted newt Notophthalmus viridescensJ Exp Biol19992023155316010539964
- KirschvinkJLMagnetite biomineralization and geomagnetic sensitivity in higher animals: an update and recommendations for future studyBioelectromagnetics1989102392592665750
- DiebelCEProkschRGreenkCRWalkerPNWalkerMMMagnetite defines a vertebrate magnetoreceptorNature200040629930210917530
- EderSHCadiouHMuhamadAMcNaughtonPAKirschvinkJLWinklhoferMMagnetic characterization of isolated candidate vertebrate magnetoreceptor cellProc Natl Acad Sci U S A2012109120221202722778440
- MooreAFreakeSMThomasIMMagnetic particles in the lateral line of the Atlantic salmon (Salmo salar L.)Philos Trans R Soc Lond B Biol Sci19903291115
- MooreARileyWDMagnetic particles associated with the lateral line of the European eel Anguilla anguillaJ Fish Biol2009741629163420735659
- OguramMKatomMArainNSasadatTSakakyiYMagnetic particles in chum salmon (Oncorhynchus keta): extraction and transmission electron microscopyCan J Zool199270874877
- IrwinWPLohmannKJDisruption of magnetic orientation in hatchling loggerhead sea turtles by pulsed magnetic fieldsJ Comp Physiol A Neuroethol Sens Neural Behav Physiol200519147548015765235
- FalkenbergGFleissnerGSchuchardtKAvian magnetoreception: elaborate iron mineral containing dendrites in the upper beak seem to be a common feature of birdsPLoS One20105e923120169083
- FleissnerGFleissnerGStahlBFalkenbergGIron-mineral-based magnetoreception in birds: the stimulus conducting systemJ Orintholog2003148S643S648
- EdwardsHHSchnellGDDuBoisRLHutchisonVHNatural and induced remanent magnetism in birdsAuk19921094356
- EdelmanNBFritzTNimpSNo evidence for intracellular magnetite in putative vertebrate magnetoreceptors identified by magnetic screeningProc Natl Acad Sci U S A201511226226725535350
- HollandRAKirschvinkJLDoakTGWikelskiMBats use magnetite to detect the earth’s magnetic fieldPLoS One20083e167618301753
- ZoegerJDunnJRFullerMMagnetic material in the head of the common Pacific dolphinScience19812138928947256282
- KirschvinkJLKobayashi-KirschvinkAWoodfordBJMagnetite biomineralization in the human brainProc Natl Acad Sci U S A199289768376871502184
- QuintanaCCowleyJMMarhicCElectron nanodiffraction and high-resolution electron microscopy studies of the structure and composition of physiological and pathological ferritinJ Struct Biol200414716617815193645
- CollingwoodJChongRKKasamaTThree-dimensional tomographic imaging and characterization of iron compounds within Alzheimer’s plaque core materialJ Alzheimers Dis20081423524518560134
- Grassi-SchultheissPPHellerFDobsonJAnalysis of magnetic material in the human heart, spleen and liverBiometals1997103513559353885
- KirschvinkJLFerromagnetic crystals (magnetite?) in human tissueJ Exp Biol1981923333357264554
- Schultheiss-GrassiPPDobsonJMagnetic analysis of human brain tissueBiometals199912677210420576
- PósfaiMDunin-BorkowskiREMagnetic nanocrystals in organismsElements20095235240
- KirschvinkJLKobayashi-KirschvinkADiaz-RicciJCKirschvinkSJMagnetite in human tissues: a mechanism for the biological effects of weak ELF magnetic fieldsBioelectromagnetics19921Suppl1011131285705
- BazylinskiDAFrankelRBMagnetosome ferritin in prokaryotesNat Rev Microbiol2004221723015083157
- FaivreDSchülerDMagnetotactic bacteria and magnetosomesChem Rev20081084875489818855486
- SchülerDMagnetoreception and Magnetosomes in BacteriaHeidelbergSpringer2007
- LowenstаmHAMagnetite in denticle capping in recent chitons (Polyplacophora)Geol Soc Am Bull196273435438
- WalkerMMKirschvinkJLChangSBDizonAEA candidate magnetic sense organ in the yellowfin tuna, Thunnus albacaresScience198422475175317780625
- HanzlikMHeunemannCHoltkamp-RötzlerEWinklhoferMPetersenNFleissnerGSuperparamagnetic magnetite in the upper beak tissue of homing pigeonsBiometals20001332533111247039
- CadiouHMcNaughtonPAAvian magnetite-based magnetoreception: a physiologist’s perspectiveJ R Soc Interface20107S193S20520106875
- CollingwoodJFMikhaylovaADavidsonMIn situ characterization and mapping of iron compounds in Alzheimer’s disease tissueJ Alzheimers Dis2005726727216131727
- DunlopDJÖzdemirÖRock Magnetism: Fundamentals and FrontiersCambridgeCambridge University Press1997
- MuxworthyARWilliamsWCritical superparamagnetic/single-domain grain sizes in interacting magnetite particles: implications for magnetosome crystalsJ R Soc Interface200961207201219091684
- LinsUMcCartneyMRFarinaMFrankelRBBuseckPRHabits of magnetosome crystals in coccoid magnetotactic bacteriaAppl Environ Microbiol2005714902490516085893
- MuxworthyARWilliamsWCritical single domain/multidomain grain-sizes in non-interacting and interacting elongated magnetite particles: implications for magnetosomesJ Geophys Res2006111B12S12
- GossCJSaturation magnetisation, coercivity and lattice parameter changes in the system Fe304-γFe2O3, and their relationship to structurePhys Chem Miner198816164171
- KletetschkaGWasilewskiPJTaylorPTHematite vs. magnetite as the signature for planetary magnetic anomalies?Phys Earth Planet Inter2000119259267
- PannalalSJCroweSACioppaMTSymonsDTSturmAFowleDARoom-temperature magnetic properties of ferrihydrite: a potential magnetic remanence carrier?Earth Planet Sci Lett2005236856870
- CornellMRSchwertmannUThe Iron Oxides: Structure, Properties, Reactions, Occurrences and UsesWeinheim, GermanyWiley2004
- RobertsAPChangLRowanCJHorngCSFlorindoFMagnetic properties of sedimentary greigite (Fe3S4): an updateRev Geophys201149RG1002
- FelnerIAlenkinaIVVinogradovAVOshtrakhMJPeculiar magnetic observations in pathological human liverJ Magn Magn Mater2016399118122
- DubielSMZabłotna-RypienBMackeyJBMagnetic properties of human liver and brain ferritinEur Biophys J19992826326710192938
- BakerRRMatherJGKennaughJHMagnetic bones in human sinusesNature19833037880
- CollingwoodJDobsonJMapping and characterization of iron compounds in Alzheimer’s tissueJ Alzheimers Dis20061021522217119289
- ChenJHardyPAClaubergMT2 values in the human brain: comparison with quantitative assays of iron and ferritinRadiology19891735215262798884
- Schultheiss-GrassiPPWessikenRDobsonJTEM observation of biogenic magnetite extracted from the human hippocampusBiochim Biophys Acta199914262122169878742
- AlekseevaTAGorobetsSGorobetsODemyanenkoIVLazarenkoOMMagnetic force microscopy of atherosclerotic plaqueMed Perspect20141410
- MoosTMorganEHThe metabolism of neuronal iron and its pathogenic role in neurological disease: reviewAnn N Y Acad Sci20041012142615105252
- BartzokisGTishlerTAMRI evaluation of basal ganglia ferritin iron and neurotoxicity in Alzheimer’s and Huntington’s diseaseCell Mol Biol (Noisy-le-grand)20004682183310875443
- LovellMARobertsonJDTeesdaleWJCampbellJLMarkesberyWRCopper, iron and zinc in Alzheimer’s disease senile plaquesJ Neurol Sci199815847529667777
- CollingwoodJFMikhaylovaADavidsonMRHigh-resolution X-ray absorption spectroscopy studies of metal compounds in neurogenerative brain tissueJ Phys Conf Ser2005175460
- DobsonJInvestigation of age-related variations in biogenic magnetite levels in the human hippocampusExp Brain Res200214412212611976766
- FullerMDobsonJWieserHGMoserSOn the sensitivity of the human brain to magnetic fields: evocation of epileptiform activityBrain Res Bull1995361551597895093
- Plascencia-VillaGPonceACollingwoodJFHigh-resolution analytical imaging and electron holography of magnetite particles in amyloid cores of Alzheimer’s diseaseSci Rep201662487327121137
- QuintanaCBellefqihSLavalJYStudy of the localization of iron, ferritin, and hemosiderin in Alzheimer’s disease hippocampus by analytical microscopy at the subcellular levelJ Struct Biol2006153425416364657
- TellerSTahirbegiIBMirMSamitierJSorianoJMagnetite-amyloid-β deteriorates activity and functional organization in an in vitro model for Alzheimer’s diseaseSci Rep201551726126608215
- GieréRMagnetite in the human body: biogenic vs. anthropogenicProc Natl Acad Sci U S A2016113119861198727729531
- MaherBAAhmedIAKarloukovskiVMagnetite pollution nanoparticles in the human brainProc Natl Acad Sci U S A2016113107971080127601646
- FelfoulOMohammadiMTaherkhaniSMagneto-aerotactic bacteria deliver drug-containing nanoliposomes to tumour hypoxic regionsNat Nanotechnol20161194194727525475
- GorobetsSGorobetsOButenkoKChizhYMBiomineralization of magnetic nanoparticles by bacterial symbionts of humanMed Perspect201419412
- ElfickARischitorGMourasRBiosynthesis of magnetic nanoparticles by human mesenchymal stem cells following transfection with the magnetotactic bacterial gene mms6Sci Rep201773975528051139
- UllrichSKubeMSchübbeSReinhardtRSchülerDA hypervariable 130-kilobase genomic region of Magnetospirillum gryphiswaldense comprises a magnetosome island which undergoes frequent rearrangements during stationary growthJ Bacteriol20051877176718416237001
- SchübbeSWilliamsTJXieGComplete genome sequence of the chemolithoautotrophic marine magnetotactic coccus strain MC-1Appl Environ Microbiol2009754835485219465526
- MuratDQuinlanAValiHKomeiliAComprehensive genetic dissection of the magnetosome gene island reveals the step-wise assembly of a prokaryotic organelleProc Natl Acad Sci U S A20101075593559820212111
- ZeytuniNOzyamakEBen-HarushKSelf-recognition mechanism of MamA, a magnetosome-associated TPR-containing protein, promotes complex assemblyProc Natl Acad Sci U S A2011108E480E48721784982
- ScheffelAGärdesAGrünbergKWannerGSchülerDThe major magnetosome proteins MamGFDC are not essential for magnetite biomineralization in Magnetospirillum gryphiswaldense but regulate the size of magnetosome crystalsJ Bacteriol200819037738617965152
- LoheAUllrichSKatzmannEFunctional analysis of the mag-netosome island in Magnetospirillum gryphiswaldense: the mamAB operon is sufficient for magnetite biomineralizationPLoS One20116e2556122043287
- GrünbergKWawerCTeboBMSchülerDA large gene cluster encoding several magnetosome proteins is conserved in different species of magnetotactic bacteriaAppl Envir Microbiol20016745734582
- WeddemannAEnnenIRegtmeierAReview and outlook: from single nanoparticles to self-assembled monolayers and granular GMR sensorsBeilstein J Nanotechnol20101759321977397
- GorobetsOGorobetsSGorobetsYBiomineralization of intracellular biogenic magnetic nanoparticles and their possible functionsRes Bull NTUU201332833
- GorobetsOGorobetsSSorokinaLBiomineralization and synthesis of biogenic magnetic nanoparticles and magnetosensitive inclusions in microorganisms and fungiFunct Mater201421427436
- WangHYuYFSunYBChenQWMagnetic nanochains: a reviewNano20116117
- GorobetsSGorobetsODemyanenkoIVSelf-organization of magnetite nanoparticles in providing Saccharamyces cerevisiaе yeasts with magnetic propertiesJ Magn Magn Mater20133375357
- JordanVCCaplanMBennettKMSimplified synthesis and relaxometry of magnetoferritin for magnetic resonance imagingMagn Res Med20106412601266
- FiguerolaADi CoratoRMannaLPellegrinoTFrom iron oxide nanoparticle towards advanced iron-based inorganic materials designed for biomedical applicationsPharmacol Res20106212614320044004
- KnovichMAStoreyJACoffmanLGTortiSVTortiFMFerritin for the clinicianBlood Rev2009239510418835072
- QuintanaCGutiérrezLCould dysfunction of ferritin be a determinant factor in the aetiology of some neurodegenerative diseases?Biochim Biophys Acta2010180077078220447447
- DoubleKLMaywaldMSchmittelMReidererPGerlachMIn vitro studies of ferritin iron release and neurotoxicityJ Neurochem199870249224999603214
- FriedmanAArosioPFinazziDKoziorowskiDGalazka-FriedmanJFerritin as an important player in neurodegenerationParkinsonism Relat Disord20111742343021550835
- LaufbergerVSur la crystallization de la ferritineBull Soc Chim Biol19371915751582
- HarrisonPMArosioPThe ferritins: molecular properties, iron storage function and cellular regulationBiochim Biophys Acta199612751612038695634
- ThielEFerritin: structure, gene regulation, and cellular function in animals, plants and microorganismsAnnu Rev Biochem1987562893153304136
- EbrahimiKHHagedoornPLHagenWRUnity in the biochemistry of the iron-storage proteins ferritin and bacterioferritinChem Rev201511529532625418839
- JutzGvan RijnPMirandaBSBökerAFerritin: a versatile building block for bionanotechnologyChem Rev20151151653170125683244
- FinazziDArosioPBiology of ferritin in mammals: an update on iron storage, oxidative damage and neurodegenerationArch Toxicol2014881787180225119494
- ZhaoGPhytoferritin and its implications for human health and nutritionBiochim Biophys Acta2010180081582320100546
- AlmirónMLinkAJFurlongDKolterRA novel DNA-binding protein with regulatory and protective roles in starved Escherichia coliGenes Dev19926264626541340475
- LewinAMooreGRLe BrunNEFormation of protein-coated iron mineralsDalton Trans20053597361016258608
- LeviSCozziAArosioPNeuroferritinopathy: a neurodegenerative disorder associated with L-ferritin mutationBest Pract Res Clin Haematol20051826527615737889
- HastingsNAPeacockJBStatistical DistributionsLondonButterworth1975
- PopplewellJSakhniniLThe dependence of the physical and magnetic properties of magnetic fluids on particle sizeJ Magn Magn Mater19951497278
- GillesGBonvillePRakotoHBrotoJMWongKKMannSMagnetic hysteresis and superantiferromagnetism in ferritin nanoparticlesJ Magn Magn Mater2002241430440
- MakhloufSAParkerFTBerkowitzAEMagnetic hysteresis anomalies in ferritinPhys Rev B Condens Matter Mater Phys199755R14717R14720
- KoralewskiMPochylskiMMitroovaZTimkoMKopcanskyPMelnıkovaLMagnetic birefringence of natural and synthetic ferritinJ Magn Magn Mater201132324132417
- GuertinRPHarrisonNZhouZXMcCallSDrymiotisFVery high field magnetization and AC susceptibility of native horse spleen ferritinJ Magn Magn Mater200730897100
- SilvaNJMillanAPalacioFTemperature dependence of anti-ferromagnetic susceptibility of ferritinsPhys Rev B Condens Matter200979104405
- ChuanlinLYunanHChenghuaGChengshengLXiguangCProperties of biogenic magnetite nanoparticles in the radula of chiton Acanthochiton rubrolineatus LischkeJ Wuhan Univ Technol Mater Sci201126478482
- PapaefthymiouGCThe Mössbauer and magnetic properties of ferritin coresBiochim Biophys Acta2010180088689720363296
- OshtrakhMIAlenkinaIVKuzmannEKlencsarZSiemionkinVAAnomalous Mössbauer line broadening for nanosized hydrous ferric oxide cores in ferritin and its pharmaceutical analogue Ferrum Lek in the temperature range 295-90 KJ Nanopart Res2014162363
- JoosARümenappCWagnerFEGleichBCharacterisation of iron oxide nanoparticles by Mössbauer spectroscopy at ambient temperatureJ Magn Magn Mater2016399123129
- JinWYXuGZSclabassiJZhuJGBagicASunMGDetection of magnetic nanoparticles with magnetoencephalographyJ Magn Magn Mater200832014721478
- PanYHSaderKPowellJJ3D morphology of the human hepatic ferritin mineral core: new evidence for a submit structure revealed by single particle analysis of HAADF-STEM imagesJ Struct Biol2009166223119116170
- QuintanaCLancinMMarhicCPérezMAvilaJCarrascosaJLPreliminary high resolution TEM and electron energy loss spectroscopy studies of ferritin cores extracted from brain in patients with neurodegenerative PSP and Alzheimer diseasesCell Mol Biol20004680782010875442
- GossuinYHautotDMullerRNLooking for biogenic magnetite in brain ferritin using NMR relaxometryNMR Biomed20051846947216177954
- PasseriDDongCReggenteMMagnetic force microscopy: quantitative issues in biomaterialsBiomatter20144e2950725050758
- DanielsSLNgunjiriJNGarnoJCInvestigation of the magnetic properties of ferritin by AFM imaging with magnetic sample modulationAnal Bioanal Chem200939421522319205674
- MikhaylovaADavidsonMToastmannHDetection, identification and mapping of iron anomalies in brain tissue using X-ray absorption spectroscopyJ R Soc Interface20052333716849161
- EspinosaASerranoALlavonaAOn the discrimination between magnetite and maghemite by XANES measurements in fluorescence modeMeas Sci Technol201223015602
- GálvezNFernándezBSánchezPComparative structural and chemical studies of ferritin cores with gradual removal of their iron contentsJ Am Chem Soc20081308062806818507465
- MelníkováLPetrenkoVIAvdeevMVEffect of iron oxide loading on magnetoferritin structure in solution as revealed by SAXS and SANSColloids Surf B Biointerfaces2014123828825249246
- PershantPSMagneto-optical effectsJ Appl Phys19673814821490
- KoralewskiMPochylskiMGierszewskiJMagnetic birefringence of iron oxyhydroxide nanoparticles stabilised by sucroseJ Magn Magn Mater201132311401144
- KoralewskiMPochylskiMMitróováZMagnetic birefringence study of the magnetic core structure of ferritinActa Phys Pol A201212112371239
- KoralewskiMKłosJWBaranowskiMThe Faraday effect of natural and artificial ferritinsNanotechnology20122335570422894853
- KoralewskiMPochylskiMGierszewskiJMagnetic properties of ferritin and akaganeite nanoparticles in aqueous suspensionJ Nanopart Res201315190224532980
- DoboszBKrzyminewskiRKoralewskiMHałupka-BrylMComputer enhancement of ESR spectra of magnetite nanoparticlesJ Magn Magn Mater2016407114121
- WajnbergEEl-JaickLJLinharesMPEsquivelDMFerromagnetic resonance of horse spleen ferritin: core blocking and surface ordering temperaturesJ Magn Reson2001153697411700082
- NewmanDMHeptinstallJMatelonRJA magneto-optic route toward the in vivo diagnosis of malaria: preliminary results and preclinical trial dataBiophys J200895994100018390603
- MensPFMatelonRJNourBYNewmanDMSchalligHDLaboratory evaluation on the sensitivity and specificity of a novel and rapid detection method for malaria diagnosis based on magneto-optical technology (MOT)Malaria J20109207
- KuBYChanMLMaZHorsleyDAFrequency-domain birefringence measurement of biological binding to magnetic nanoparticlesJ Magn Magn Mater20083202279228320463913
- WangWKnovichMACoffmanLGTortiFMTortiSVSerum ferritin: past, present and futureBioch Biophys Acta20101800760769
- WorwoodMFerritinBlood Rev199042592692076473
- CozziARovelliEFrizzaleGOxidative stress and cell death in cells expressing L-ferritin variants causing neuroferritinopathyNeurobiol Dis200937778519781644
- Galazka-FriedmanJBaumingerERSzlachtaKFriedmanAThe role of iron in neurodegeneration: Mössbauer spectroscopy, electron microscopy, enzyme-linked immunosorbent assay and neuroimaging studiesJ Phys Condens Matter20122424410622595616
- HautotDPankhurstQAMorrisCMCurtisABurnJDobsonJPreliminary observation of elevated levels of nanocrystalline iron oxides in the basal ganglia of neuroferritinopathy patientsBiochim Biophys Acta20071772212517097860
- CéspedesOUenoSEffect of radio frequency magnetic fields on iron release from cage proteinsBioelectromagnetics20093033634219274682
- CéspedesOInamotoOKaiSNibuYRadio frequency magnetic fields effects on molecular dynamics and iron uptake in cage proteinsBioelectromagnetics20103131131720082334
- WebbJMaceyDJChua-AnsuornWIron biominerals in medicine and the environmentCoord Chem Rev1999190–19211991215
- AllenPDSt PierreTGChua-AnsuornWStrömVRaoKVLow-frequency low-field magnetic susceptibility of ferritin and hemosiderinBiochim Biophys Acta2000150018619610657588
- MiayazakiEKatoJKobuneMDenaturated H-ferritin is a major constituent of hemosiderin in the liver of patient with iron overloadGut20025041341911839724
- WilliamsJMAndrewsSCTreffryAHarrisonPMThe relationship between ferritin and haemosiderinHyperfine Interact19862914471450
- WebbJSt PierreTGTranKCChua-AnusomWMaceyDJPootrakulPBiologically significant iron(III) oxyhydroxy polymers: Mössbauer spectroscopic study of ferritin and hemosiderin in pancreas tissue of β-thalassemia/hemoglobin E diseaseInorg Chim Acta1996243121125
- CaiYCaoChHeXEnhanced magnetic resonance imaging and staining of cancer cells using ferromagnetic H-ferritin nano-particles with increasing core sizeInt J Nanomedicine2015102619263425878496
- SchwartzJLiuXSTosaTThielECSolomonEISpectroscopic definition of the ferroxidase site in M ferritin: comparison of binu-clear substrate vs cofactor active sitesJ Am Chem Soc20081309441945018576633
- MeldrumFCHeywoodBRMannSMagnetoferrin: in vitro synthesis of a novel magnetic proteinScience19922575225231636086
- KlemMTYoungMDouglasTBiomimetic magnetic nanoparticlesMater Today200582837
- UchidaMKangSReichhardtCHarlenKDouglasTThe ferritin superfamily: supramolecular templates for materials synthesisBiochim Biophys Acta2010180083484520026386
- ValeroETambaloSMarzolaPMagnetic nanoparticles-templated assembly of protein subunits: new platforms for carbohydrate-based MRI nanoprobesJ Am Chem Soc20111334889489521384882
- JutzGBoekerABionanoparticles as functional macromolecular building blocks: a new class of nanomaterialPolymer201152211232
- JääskeläinenASoukkaTLamminmäkiUKorpimäkiTVirtaMDevelopment of a denaturation/renaturation-based production process for ferritin nanoparticlesBiotechnol Bioeng20091021012102418958859
- YamashitaIIwahoriKKumagaSFerritin in the field on nanodevicesBiochim Biophys Acta2010180084685720227466
- CromptonDEChinneryPFBatesDSpectrum of movement disorders in neuroferritinopathyMov Disord200520959915390132
- CollingwoodJFDavidsonMRThe role of iron in neurodegenerative disorders: insights and opportunities with synchrotron lightFront Pharmacol2014519125191270
- EverettJCéspedesEShelfordLRFerrous iron formation following the co-aggregation of ferric iron and the Alzheimer’s disease peptide β-amyloid (1–42)J R Soc Interface2014112014016524671940
- HautotDPankhurstQADobsonJSuperconducting quantum interference device measurements of dilute magnetic materials in biological samplesRev Sci Instrum200576045101
- DobsonJNanoscale biogenic iron oxides and neurodegenerative diseaseFEBS Lett20014961511343696
- DobsonJMagnetic iron compounds in neurological disordersAnn N Y Acad Sci2004101218319215105266
- PannYBrownYABrydsonRWarleyALiAPowellJElectron beam damage studies of synthetic 6-line ferrihydrite and ferritin molecule cores within a human liver biopsyMicron20063740341116466926
- KoralewskiMMelníkováLMitróováZPochylskiMBaranowskiMKopČanskýPMagnetooptical investigation of ferritin iron uptake and releasePoster presented at: 10th International Conference on the Scientific and Clinical Applications of Magnetic CarriersJune 10–14, 2014Dresden, Germany
- BilgicBPfefferbaumARohlfingTSullivanEVAdalsteinssonEMRI estimates of brain iron concentration in normal aging using quantitative susceptibility mappingNeuroimage2012592625263521925274
- HaackeEMChengNYHouseMJImaging iron stores in the brain using magnetic imagingMagn Reson Imaging20052312515733784
- LangkammerCSchweserFKrebsNQuantitative susceptibility mapping (QSM) as a means to measure brain iron? A post mortem validation studyNeuroimage2012621593159922634862
- LangkammerCRopeleSPirpamerLFazekasFSchmidtRMRI for iron mapping in Alzheimer’s diseaseNeurodegener Dis20141318919123942230
- RamosPSantosAPintoNRMendesRMagalhãesTAlmeidaAIron levels in the human brain: a post-mortem study of anatomical region differences age-related changesJ Trace Elem Med Biol201428131724075790
- SchweserFDeistungALehrBWReichenbachJRQuantitative imaging of intrinsic magnetic tissue properties using MRI signal phase: an approach to in vivo brain iron metabolism?Neuroimage2011542789280721040794
- MigllieriniMBocaRKopániMLancokAMössbauer and SQUID characterization of iron in human tissue: case of globus pallidusActa Phys Pol A2014126240241
- SzybowiczMKoralewskiMKarońJMelnikowaLMicro-Raman spectroscopy of natural and synthetic ferritins and their mimeticsActa Phys Pol A2015127534536
- StöckelSKirchhoffJNeugebauerURöschPPoppJThe application of Raman spectroscopy for the detection and identification of microorganismsJ Raman Spectrosc20164789109
- ButlerHJAshtonLBirdBUsing Raman spectroscopy to characterized biological materialsNat Protoc20161166468726963630
- DunnJRFullerMZoegerJMagnetic material in the human hippocampusBrain Res Bull1995361491537895092
- CollingwoodJFAdamsFChemical imaging analysis of the brain with X-ray methodsSpectrochim Acta Part B2017130101118
- DobsonJGrassiPMagnetic properties of human hippocampal tissue: evaluation of artefact and contamination sourcesBrain Res Bull1996392552598963692
- ProzorovTMallapragadaSKNarasimhanBProtein-mediated synthesis of uniform superparamagnetic magnetite nanocrystalsAdv Funct Mater200717951957
- AmemiyaYArakakiAStanilandSSTanakaTMatsunagaTControlled formation of magnetite crystal by partial oxidation of ferrous hydroxide in the presence of recombinant magnetotacticBiomaterials2007285381538917720242
- ArakakiAMasudaFAmemiyaYTanakaTMatsunagaTControl of the morphology and size of magnetite particles with peptides mimicking the Mms6 protein from magnetotactic bacteriaJ Colloid Interface Sci2010343657020006848
- GallowayJMArakakiAMasudaFTanakaTMatsunagaTStanilandSSMagnetic bacterial protein Mms6 controls morphology, crystallinity and magnetism of cobalt-doped magnetite nanoparticles in vitroJ Mater Chem2011211524415254
- SiponenMILegrandBWiddratMStructural insight into magnetochrome-mediated magnetite biomineralizationNature201350268168424097349
- GorobetsSGorobetsOFunctions of biogenic magnetic nanoparticles in organismsFunct Mater2012191826
- GorobetsSGorobetsODemyanenkoIFerritin and biomineralization of magnetic nanoparticles in microorganismsRes Bull NTUU201333441
- MatsunagaTOkamuraYFukudaYWahyudiATMuraseYTakeyamaHComplete genome sequence of the facultative anaerobic magnetotactic bacterium Magnetospirillum sp. strain AMB-1DNA Res20051215716616303747
- NakazawaHArakakiANarita-YamadaWhole genome sequence of Desulfovibrio magneticus strain RS-1 revealed common gene clusters in magnetotactic bacteriaGenome Res2009191801180819675025
- MetheBANelsonKEEisenJAGenome of Geobacter sulfurreducens: metal reduction in subsurface environmentsScience20033021967196914671304
- AklujkarMKrushkaJDiBartoloGLapidusALandMLLovleyDRThe genome sequence of Geobacter metallireducens: features of metabolism, physiology and regulation common and dissimilar to Geobacter sulfurreducenesBMC Microbiol2009910912219473543
- GuoFFYangWJiangWGengSPengTLiJLMagnetosomes eliminate intracellular reactive oxygen species in Magnetospirillum gryphiswaldense MSR-1Environ Microbiol2012141722172922360568
- BushACopper, zinc, and the metallobiology of Alzheimer diseaseAlzheimer Dis Assoc Disord20031714715014512827
- HouseEEsiriMForsterGIncePGExleyCAluminium, iron and copper in human brain tissues donated to the Medical Research Council’s Cognitive Function and Ageing studyMetallomics20124566522045115
- SidhuPSGilkesRJPosnerAMThe synthesis and some properties of Co, Ni, Zn, Cu, Mn and Cd substituted magnetitesJ Inorg Nucl Chem197840429435
- ToshaTNgHLBhattasaliOAlberTTheilECMoving metal ions through ferritin-protein nanocages from three-fold pores to catalytic sitesJ Am Chem Soc2010132145621456920866049
- IwahoriKYamashitaISize-controlled one-pot synthesis of fluorescent cadmium sulfide semiconductor nanoparticle in an apoferritin cavityNanotechnology20081949560121730676
- KozlowskiHLuczkowskiMRemelliMValensinDCopper, zinc and iron in neurodegenerative diseases (Alzheimer’s, Parkinson’s and prion diseases)Coord Chem Rev201225621292141
- WangXLiangLEffects of static magnetic field on magnetosome formation and expression of mamA, mms13, mms6 and magA in Magnetospirillum magneticum AMB-1Bioelectromagnetics20094313321
- WangXLiangLSongTWuLMagnetosome formation and expression of mamA, mms13, mms6 and magA in Magnetospirillum magneticum AMB-1 exposed to pulsed magnetic fieldCurr Microbiol20095922122619459005
- SonnierHMarinoAASensory transduction as a proposed model for biological detection of electromagnetic fieldsElectro Magnetobiol200120153175
- JandackaPBurdaHPistoraJMagnetically induced behaviour of ferritin corpuscles in avian ears: can cuticulosomes function as magnetosomes?J R Soc Interface2015122014108725551148
- GorobetsYGorobetsSStationary flows of electrolytes in the vicinity of ferromagnetic particles in a constant magnetic fieldBull Kherson State Tech Univ20003276281
- GorobetsOBiomagnetism and biogenic magnetic nanoparticlesHer Natl Acad Sci Ukr201575364
- BanaclochaMABókkonIBanaclochaHMLong-term memory in brain magnetiteMed Hypotheses20107425425719815351
- BókkonISalariVInformation storing by biomagnetitesJ Biol Phys20103610912019728122
- HandEJoe Kirschvink thinks he has found a magnetic sixth sense in humansScience201635215091513