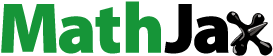
Abstract
Inhibition of tumor growth induced by treatment with direct electric current (DC) has been reported in several models. One of the mechanisms responsible for the antitumoral activity of DC is the generation of oxidative species, known as chloramines. With the aim of increasing chloramine production in the electrolytic medium and optimizing the antitumoral effects of DC, poly(ɛ-caprolactone) (PCL) nanoparticles (NPs) loaded with the amino acid tyrosine were obtained. The physical–chemical characterization showed that the NPs presented size in nanometric range and monomodal distribution. A slightly negative electrokinetic potential was also found in both blank NPs and l-tyrosine-loaded PCL NPs. The yield of the loading process was approximately 50%. Within 3 h of dissolution assay, a burst release of about 80% l-tyrosine was obtained. The in vitro cytotoxicity of DC was significantly increased when associated with l-tyrosine-loaded NPs, using a murine multidrug-resistant melanoma cell line model. This study showed that the use of the combination of nanotechnology and DC has a promising antineoplastic potential and opens a new perspective in cancer therapy.
Introduction
Nanoparticulate systems show promise as active vectors because of their capacity to release substances.Citation1 The advantages of using this approach for drug delivery result from some interesting properties of these systems. Because of their small size, they can travel through the endothelium of inflammatory sites, epithelium (eg, intestinal tract and liver), and tumors or penetrate microcapillaries.Citation2 In addition to their biodegradability and biocompatibility, the use of these delivery systems result in increased bioavailability of transported drugs with minimal toxicity.Citation3,Citation4 Nanoparticle (NP) systems have recently been incorporated within cancer therapies, because of their ability to concentrate drugs at the tumor site, which enhances their cytotoxic effects on the cancer cells and therefore reduces systemic side effects.Citation5–Citation7
Poly (ɛ-caprolactone) (PCL) is an aliphatic polyester that is used to develop inject-able sustained delivery systems, such as polymeric NPs.Citation8 PCL NPs have been used as drug-carrier systems for amphotericin B,Citation9,Citation10 indomethacin,Citation11 griseofulvin,Citation12 tamoxifen,Citation13 vaccines,Citation14 magnetic composites,Citation15 and cyclosporine A.Citation16 PCL NP production by the double emulsion solvent evaporation method (DEEM) has been shown to exhibit satisfactory encapsulation efficiency for hydrophilic drugs.Citation17–Citation19
Scientific investigations have demonstrated that electrotherapy (EChT) presents an effective approach for treating several types of cancer.Citation20–Citation22 EChT uses a low direct electric current (DC) to treat tumor tissue through two or more electrodes placed within the tumoral zone or within its surrounding area. This treatment is noted for its significant effectiveness, minimal invasiveness, localized action, and low cost.Citation23–Citation25 Nordenström discussed the mechanisms associated with this therapy, such as pH alterations, electroosmotic transport of water, and the effects on ionic transmembrane flow, as partially responsible for the efficacy of EChT.Citation26,Citation27
An important milestone for the study of EChT was the development of an in vitro experimental model for investigation of DC effects on culture cell lines.Citation28–Citation30 Using this model, Veiga et al showed that DC can destroy tumor cells via two distinct mechanisms: apoptosis and necrosis.Citation30 Both aforementioned mechanisms appear to be associated with the DC polarity: cells exposed to anodic flow (AF) undergo mostly apoptosis, whereas those exposed to cathodic flow (CF) die almost entirely as a result of necrosis.Citation30 These authors attributed the apoptotic cell death to chlorinated species (Cl−, Cl2, HOCl) generated by AF, which react with amino acids to produce oxidizing and apoptosis-inducing molecules called chloramines. As a matter of fact, other studies developed using different reactive oxygen species have proven that oxidants, hypochlorous acid and chloramines, induced apoptotic cell death from the reaction of chlorinated species with free amino acids present in the cell suspension.Citation31,Citation32 Englert and ShacterCitation32 compared the influence of different amino acids (taurine, arginine, lysine, glutamic acid, glutamine, and isoleucine) on the viability of lymphoma cells in the presence of oxidant compounds. These authors showed that depending on the kind of amino acids, cell death by necrosis or apoptosis was detected in the cellular medium. In their experimental model, the formation of chloramines generated by the reaction of H2O2 with glutamine and arginine induced exclusively apoptosis and necrosis, respectively.Citation32 Indeed, Veiga et al confirmed this hypothesis by adding L-glutamine to the electrolytic medium during exposure of human leukemic cells to AF generated by DC.Citation30 Besides, L-tyrosine, L-glutamine, and L-tryptophan were also tested in B16F10 murine melanoma cells, and the highest cytotoxic effects induced by chloramines generated by DC stimulation were obtained in the presence of L-tyrosine (unpublished data). Considering this preliminary screening, L-tyrosine was the amino acid chosen for the present study.
Given the results of these previous works, it is likely that amino acid encapsulation within NPs may modulate chloramine production within the AF and, most importantly, increase its delivery to target cells. Targeted drug delivery would enhance the damage induced by these molecules and thus improves DC antitumoral activity. To verify this hypothesis, the present work describes the use of nanotechnology in association with DC for the first time. l-Tyrosine was loaded onto PCL polymer, and the physical and chemical parameters of this loading process were determined. Additionally, the cytotoxicity of this association was evaluated in murine multidrug-resistant melanoma cell line B16F10. Our results show that the use of nanotechnology along with DC has a promising antineoplastic potential.
Material and methods
Chemicals
The following chemicals were purchased from VETEC Química Fina (Rio de Janeiro, Brazil): polyvinyl alcohol (PVA), trypan blue powder, dichloromethane (DCM), L-tyrosine, monobasic sodium phosphate, dibasic potassium phosphate, and sodium chloride. The following cell culture items were purchased from Gibco Invitrogen Corporation (New York, NY): Dulbecco’s modified Eagle’s medium (DMEM), glutamine, fetal bovine serum (FBS), sodium bicarbonate, sodium hydroxide, Pen Strep (penicillin 10,000 units/ml + streptomycin 10,000 μg/mL aqueous solution), and trypsin 1:250 powder. Finally, these items were purchased from Sigma-Aldrich (St Louis, MO): dimethyl sulfoxide, ethylenediaminetetraacetic acid, N-2-hydroxyethylpiperazine-N′-2-ethanesulfonic acid, [3-(4,5-dimethylthiazol-2-yl)-2,5-diphenyltetrazolium bromide] (MTT), and PCL.
Nanoparticle preparation
PCL NPs containing L-tyrosine were prepared by the double emulsification evaporation method. One hundred milligrams of PCL was briefly dissolved in 5 mL of DCM. This organic solution was emulsified in 1 mL of 1% PVA with 0.4 mg/mL of l-tyrosine aqueous internal phase for 1 min using an ultrasonicator (Ultrasonic Processor – UP 100 H, 60 W, 30 kHz; Hielscher, Germany) in an ice bath. This primary emulsion was poured into 20 mL of a 0.5% PVA aqueous solution and sonicated again with the same ultrasonic probe for 2 min under the same conditions to create the water for oil-in-water emulsion. The solvent (DCM) was evaporated with stirring at room temperature (28°C). NPs suspensions were purified twice by a 30-min centrifugation at 10,000 × g (Avanti J-2 cooled ultracentrifuge; Beckman Coulter, San Francisco, CA) for 40 min and followed by resuspension in 4 mL of distilled water.Citation33–Citation35
The suspension was transferred into a glass vial and stored at −20°C. Freeze-drying was carried out in a lyophilizer (freeze dry system; Labconco, Brazil) and yielded powdered NPs. The samples were stored at room temperature (28°C) before analysis. The lyophilized samples were resuspended in water and observed by optical microscopy to detect the presence of aggregates.Citation36
The process yield was calculated using EquationEq. 1(1) :
where Y (%) is the process yield, MNP is the mass of NPs recovered after freeze-drying, and MT is the mass of polymer plus the mass of L-tyrosine in formulation before the process. The encapsulation method was performed in triplicate (n = 3).
The NPs encapsulation efficiency was determined by a spectrophotometric method. The drug was dissolved in phosphate-buffered saline (PBS, g/L: 0.26 KH2PO4, 2.17 Na2HPO4·7H2O, 8.71 NaCl), and the absorbance was measured at 275 nm (spectrophotometer; UV 2401 PC; Shimadzu Kyoto, Japan). The calibration curve was linear (r = 0.999) for L-tyrosine in a range of 20–200 μg/mL. Drug concentrations were calculated based on the standard curve equation. Ten milligrams of freeze-dried NPs was dissolved in 5 mL of DCM to dissolve the polymer, and 5 mL of PBS was added to preferentially precipitate the polymer. The suspension was filtered through a membrane filter (0.22 μm; Millex, The Netherlands) to remove the insoluble polymer and rediluted with PBS for analysis via the spectrophotometric method as described above. Encapsulation efficiency was calculated from EquationEq. 2(2) :
where EE is the encapsulation efficiency, M1 is the mass of L-tyrosine (μg) on the NPs, and M2 is the mass of L-PCL (mg). The experiments were performed in triplicate (n = 3).
NPs were characterized by size, polydispersity index (PI), and electric potential (zeta potential). The size and PI of the particles were determined by photon correlation spectroscopy using a Zetasizer® 3000 (Malvern Instruments, Worcestershire, UK) with a laser reader of 633 nm, operated at an angle of 173°, and a temperature of 25°C. The samples of L-tyrosine-loaded and unloaded PCL NPs were diluted: 1:400 (p/v) in distilled water, and 1 mL of this solution was added to a plastic cuvette. The zeta potential was measured in a 10−3 M NaCl aqueous solution using the electrophoretic mode of the device.Citation35 Each sample was measured in triplicate for each parameter.
NPs morphology was studied by transmission electron microscopy (Morgagni 268; FEI Company, Eindhoven, The Netherlands). The sample preparation was performed from an aqueous suspension containing 50 mg of NPs in 20 mL of distilled water, and 10 μL of this suspension was placed on a carbon-coated electron microscopy grid following fixation with 10 μL of uranyl acetate 3% (w/v) for 1 min. The grid was properly dried and then examined using transmission electron microscope.Citation36
The release profile of L-tyrosine-loaded PCL NPs was assessed by a dissolution study with spectrophotometric analysis.Citation34 A 50-mg sample of NPs loaded with approximately 1.46 mg of L-tyrosine was resuspended in 10 mL of PBS, and this suspension was stirred at 200 rpm and 37°C. At time points of 1, 2, 3, 24, 48, 72, and 96 h, the entire content was withdrawn and centrifuged at 10,000 rpm for 30 min. The supernatant was collected and filtered with a 0.22-μm cellulose nitrate membrane; the L-tyrosine concentration was determined by spectrophotometry at a wavelength of 275 nm (spectrophotometer, UV 2401 PC; Shimadzu). The remaining NPs precipitate was resuspended in 10 mL of PBS and returned to stirring at 37°C until the next sampling time.
Cell culture
B16F10 cells were obtained from Rio de Janeiro’s Cell Bank (UFRJ, Brazil). They were cultured at 37°C in 25-cm2 culture flasks containing DMEM medium supplemented with 10% (v/v) FBS. pH was controlled by the addition of 3 g/L N-2-hydroxyethylpiperazine-N′-2-ethanesulfonic acid, 0.2 g/L NaHCO3, streptomycin (100 μg/mL), and penicillin (100 UI/mL) as previously described.Citation28,Citation37 The initial inoculum was 5 × 104 cells/mL, which was subcultured every 2 days as described elsewhereCitation28,Citation29 and maintained in a log-phase growth. Cell detachment was performed with 2 mL of trypsin 0.05% (w/v) for 2 min.
DC stimulation
After the detachment, B16F10 cells were collected by centrifugation, washed, and suspended (5.0 × 105 cells/mL) in PBS (pH 7.4) at 308 mOsm. The cellular suspensions were then distributed into a 24-well culture plate (2 mL of cell suspension/well). These wells were linked in series by filter paper bridges and fitted with platinum electrodes at their extremities, which were connected to a DC source (FA-3050 model; Instrutherm, Brazil). Control cells were presented with the same experimental conditions, except for the contact with the DC source (). Following this approach, cell suspensions can be exposed directly to CF, AF, and DC without contact with the electrodes, namely electroionic flow (EIF) at 25°C. The distance between the electrodes was 8 cm, and an electric field of approximately 2.5 V/cm was generated as a result. Cell suspensions were treated at room temperature with DC of 2 mA, for 0 (control), 2, 4, 6, 8, and 10 min, similar to the conditions previously usedCitation28–Citation30,Citation38 to assess cell viability. In all subsequent experiments, cells were treated for 6 min, an intermediate period that was representative of the alterations in morphology and viability. The B16F10 cells were preincubated for 30 min with different electrolytic suspensions, such as PBS, PBS + 181.19 mg of free L-tyrosine, PBS + 0.5 mg/mL of unloaded NPs, and PBS + 0.5 mg/L of L-tyrosine-loaded PCL NPs, which correspond to approximately 30 μg of L-tyrosine. After incubation, DC treatment was performed for 6 min, the electrolytic media was immediately removed, and all of the cells were washed twice with PBS. The cytotoxicity of the DC treatment was assessed by the MTT method after 24 h of incubation with DMEM supplemented with 10% FBS as described below.
Figure 1 Schematic representation of the in vitro system used for DC treatment of B16F10 cells. The cell suspensions are distributed over each individual well and connected to a positive pole (AF) and negative pole (CF). An intermediary well, namely electroionic flow (FEI), is connected to the other wells by filter paper bridges moistened with phosphate saline buffer. Platinum electrodes are inserted into the AF and CF poles, allowing the system to be connected to a DC source and an amperimeter to monitor DC intensity. This distribution permits the separate exposure of cells to cathodic and anodic reactions, and to the DC (FEI). Control cells are exposed to the same conditions, except for the use of DC. Internal volume of each well is 0.84 cm3. The distance between the electrodes was 8 cm, and an electric field of approximately 2.5 V/cm was generated.
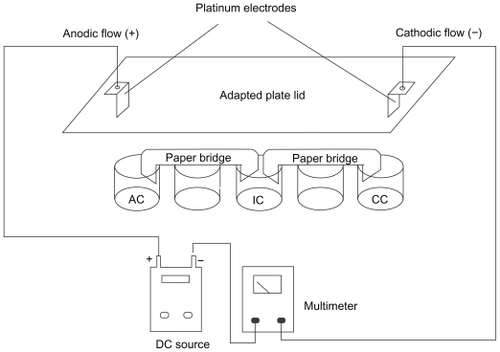
Cell viability
Cell viability was assessed by measuring the cellular mitochondrial activity by the MTT method.Citation39,Citation40 After DC treatment, cells were removed from the plate with trypsin (as described above) and resuspended in 2 mL of DMEM supplemented with FBS 10%; 180 μL of this cellular suspension was transferred to a 96-well microplate and incubated with 20 μL of a 5-mg/L solution of tetrazolium reagent (MTT; Sigma-Aldrich) for 3 h at 37°C. The supernatant was removed by centrifugation at 1100 rpm for 7 min using the CT-6000R Cientec centrifuge (Piracicaba, Brazil). The formazan crystals were diluted in 200 μL of pure dimethyl sulfoxide (Sigma-Aldrich), and the absorbance was read at 490 nm on a plate-reading spectrophotometer (thermoplate). Viability was calculated as the proportion of absorbance of each sample compared to that of the controls (Abssample/Abscontrol). This experiment was performed in quintuplicate.
Cell growth
To evaluate the influence of DC treatment on B16F10 growth, the cells were suspended in 2 mL of PBS (106 cells/mL) and treated for 6 min. After DC treatment, the cells were washed in PBS, and the suspensions containing similar cell numbers were cultivated in a 24-well plate in DMEM medium supplemented with FBS 10%. Aliquots of 25 μL of the cell suspensions were taken at 0, 24, and 48 h, and the number of trypan blue-stained and -unstained cells was determined in a Neubauer chamber. For the quantitative assessment of growth inhibition, trypan blue-stained cells were not considered.Citation28,Citation29
Results
shows the quantitative analysis of the encapsulation process and the characterization parameters of the NPs. The double emulsion technique yielded NPs with high homogeneity, as detected by the low PIs. PCL-loaded and -unloaded NPs showed the same morphological characteristics, as could be observed by transmission electron microscope, such as an elliptic form and regular borders, with sizes in the nanometer range (). Indeed, the mean NPs diameters measured at the Zetasizer ranged from 244.90 to 269.00 nm (). The unloaded NPs showed significantly lower mean diameters when compared to those of the L-tyrosine-loaded PCL NPs (P < 0.001). However, the size increase caused by amino acid insertion did not exceed 10% when compared to blank NPs.
Figure 2 Morphological features and mean diameter of L-tyrosine-loaded PCL NPs. A) Transmission electron micrograph of PCL NPs loaded with L-tyrosine. Scale bar: 100 nm. B) Diameter distribution of PCL NPs loaded with L-tyrosine determined by photon correlation spectroscopy, showing that most NPs presented diameter in the nanometer scale. The experiments were done three times independently (n = 3).
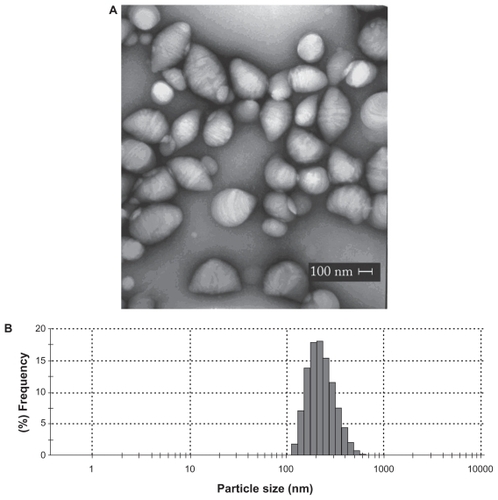
Table 1 Process yield, drug encapsulation efficiency, mean diameter, and PI of blank and l-tyrosine-loaded PCL NPs (n = 3 ± SD)
The NP surface potential did not show significant alterations after L-tyrosine encapsulation or after AF exposure (P > 0.05) (). The overall process yield was approximately 50% for both preparations, and the encapsulation efficiency reached approximately 30%.
Table 2 Zeta potential analyses of blank and l-tyrosine-loaded PCL NPs
The release kinetics of L-tyrosine from loaded PCL NPs is shown in . A burst release of approximately 80% of the total L-tyrosine was observed within the first 3 h of dissolution and subsequently followed by a drastic drop in the release rate. After 96 h, the NP content was virtually entirely released (data not shown).
Figure 3 Release kinetics of l-tyrosine from PCL NPs in a period of 24 h. The experiments were done three times independently (n = 3 ± SD).

Effects of various DC exposure times on B16F10 melanoma cell viability are shown in (immediately after exposure) and (24 h later). The AF and CF induced similar and statistically significant profiles of an immediate reduction in cell viability after four or more minutes of exposure, which was directly proportional to the stimulus time (P < 0.01). Twenty-four hours after DC exposure, the same viability profile was observed for the AF and CF in comparison to untreated control cells. The electroionic flow did not induce statistically significant changes in cell viability either immediately or 24 h after DC exposure (P > 0.05).
Figure 4 Kinetics of trypan blue incorporation by both treated and untreated B16F10 cells. A) B16F10 cells were treated with 2 mA of DC for periods varying from 0 to 10 min and their viability assayed immediately by the trypan blue method. Black squares represent the viability of control cells (■), and the open symbols represent the cells treated by the CF (⋄), AF (▴), and electroionic flow (□). Anodic and cathodic stimuli induced significant differences in the B16F10 viability when compared to untreated cells (P < 0.01), except for the AF stimulus of 2 min. Besides, the electroionic flow stimuli did not induce any changes in cell viability (P > 0.05). B) B16F10 cells were treated for periods varying from 0 to 10 min, and their viability was assayed by the trypan blue method 24 h after the treatment. Black squares represent the viability of control cells (■), and the open symbols represent the cells treated by the CF (⋄), AF (▴), and electroionic flow (□). This graph shows that the cell viability was not recovered, except for the cells treated for 4 min with the AF stimulus. As detected immediately after treatment, the electroionic flow stimuli did not induce any changes in cell viability (P > 0.05).
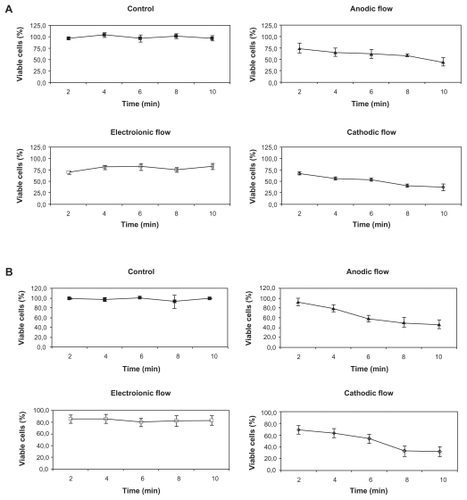
Cell proliferation was assessed 24 and 48 h after a 6-min anodic stimulation (). The time required to reduce the B16F10 cell viability to approximately 60% was 6 min. For the cell proliferation experiment, the initial inoculum of control and treated cells was 1.33 × 105 and 1.08 × 105 cells, respectively. After 48 h, the number of control cells increased from 1.33 × 105 to 2.55 × 105. However, after 24 h of treatment, using AF induced a significantly impaired growth of B16F10 cells; the number of cells dropped from 1.08 × 105 to 0.68 × 105, indicating a 54.7% reduction in cell number. This inhibition remained in this magnitude for 48 h of post-treatment, indicating that B16F10 cells were incapable of recovering their proliferation rates.
Figure 5 Treatment of B16F10 cells with DC inhibits cell proliferation. Cells were treated with 2 mA of AF for 6 min (▴) and inoculated into fresh media. The number of cells treated and untreated (■) was quantified by trypan blue dye after 24 h and 48 h, considering only the cells that were not permeable to the dye (viable cells). The proliferation rate of treated cells was 54.7% lower than the untreated cells. Results of five independent experiments expressed as mean ± SD are shown.

Effects of AF stimulation in the presence of free and encapsulated tyrosine on B16F10 viability are shown in . This experiment showed that loaded- and unloaded-PCL NPs did not exhibit any cytotoxic properties. However, using AF stimulation in association with tyrosine-loaded NPs induced a 40% decrease in cell viability immediately (data not shown) and 24 h after treatment, indicating that this combination has a promising antineoplastic potential. Additionally, the loading process enabled the use of a significantly lower amount of tyrosine in its encapsulated form when compared to its free form. In fact, the amount of tyrosine in the nanosystem was 6000-fold smaller and yet induced higher mortality rates (data not shown).
Figure 6 Decrease in the B16F10 viability induced by different experimental conditions measured by MTT assay. Cells were treated with A) L-tyrosine-loaded PCL NPs, B) unloaded NPs, C) 6 min of AF, D) unloaded NPs plus AF, and E) L-tyrosine-loaded PCL NPs plus AF. The percentage of viability decrease was determined immediately (data not shown) and 24 h after treatment. The major cell viability decrease (around 60%) was obtained when the L-tyrosine-loaded PCL NPs were associated with AF. Results of three independent experiments expressed as mean ± SD are shown.
Notes: *Significantly different from control with P < 0.05.
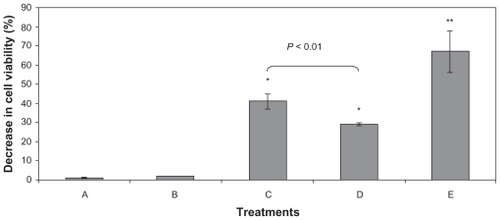
Discussion
Among the morphofunctional features of solid tumors are the abnormal function of the lymphatic vasculature, which causes fluid retention, and an increased permeability of blood vessel walls. Both of these characteristics lead to a higher retention of NPs in the tumor area when compared to that of normal tissues, resulting in a passive selectivity of the system.Citation7 Thus, a small particle size, which is directly related to the technique of NPs obtention, is crucial for the desired tissue penetration.Citation41
The size of DEEM-yielded NPs depends on many factors, such as stirring speed, solution viscosity, organic/aqueous phase ratio, and PVA concentration in external aqueous phase.Citation42 In the present work, the use of sonication in conjunction with DEEM produced fine, nanometric-scaled, homogeneous pools of loaded- or unloaded-PCL NPs. The polydispersity values obtained were approximately 0.1, indicating a monomodal distribution of particle size and a very high quality of preparation.Citation43 Furthermore, the loading yield is comparable to other findings in the literatureCitation4,Citation17 and is probably due to the low solubility of L-tyrosine in PBS aqueous phase. The surface charge of NPs can be characterized by the zeta potential,Citation44 which reflects the electric potential of particles and is influenced by their chemical composition as well as the medium in which they are dispersed.Citation2 To assess the influence of AF stimulation on the surface charge of the NPs, zeta potentials were also measured before and after a 6-min AF exposure for both preparations (loaded- and unloaded- NPs). Indeed, the surface potentials became slightly more electronegative after AF, although this increase did not prove to be statistically significant in our analysis (P > 0.05).
The majority of the amino acid content was released from loaded NPs within the first 3 h at a rate of 80%. The release speed depends on the nature of both polymer and encapsulated molecule.Citation3 The burst release effect observed in our system can be explained by molecules associated pre-dominantly with the surface and the outer layers of the NP.Citation34 The sustained release is due to the semicrystalline structure of PCL NPs and the pores formed in its polymeric matrix during the encapsulation process.Citation18 Once in an aqueous environment, the PCL NP matrix slowly undergoes erosion, allowing penetration of water into its structure and the outward diffusion of L-tyrosine.
This in vitro release study determined that a preincubation time of 30 min was adequate to ensure the delivery of a significant amount of L-tyrosine. In addition, exposure of B16F10 cells to a 6-min AF treatment in PBS resulted in an immediate cell death of approximately 50% and significant impairment of growth recovery after 24 h. These conditions have been defined as the most suitable parameters for comparing the different experimental groups.
DC is a physical agent capable of destroying different kinds of tumor cells, characterizing a tumor therapy (EChT). This treatment is noted for its great effectiveness, minimal invasiveness, local effect, and low cost.Citation24–Citation26 The principal tumor damage induced by DC has been attributed to the formation of electrolysis products, which were capable of killing the tumor cells.Citation21 Our research group has detected the effects of pH acidification and alkalinization induced by AF and CF on different tumor cell lines.Citation28–Citation30,Citation38 However, in these studies, tumor death was not only attributed exclusively to the electrolysis damage, but also to the oxidant species generated by the AF.Citation29,Citation30 In fact, the acidification of the AF-treated medium could not be held responsible for the cell killing, as the pH variation induced by anodic reactions is not significant (6.5 after 10 min of stimulation).Citation30 Besides, when the pH was artificially mimetized by the addition of HCl drops, cell viability was not diminished at equivalent rates as detected by AF generated by DC source, suggesting the role of other electrolysis products, such as reactive chlorine and oxygen species, in tumor killing.Citation30
It is common knowledge that DC can both change surface cell receptors and also modify cell membrane permeability.Citation28,Citation29,Citation45 In fact, this latter property is used therapeutically to ease drug penetration into tumor cells, a combined technique known as electrochemotherapy.Citation46–Citation48 In the present study, NPs were produced with PCL; this polymer has a negative electrokinetic potential, probably due to the presence of some residual functional groups with negative charges on the monomer employed in PCL polymerization.Citation44 After the loading process and the anodic stimulation, we detected a modification of the zeta potential, but the NPs remained negatively charged. As shown in , these values varied between −1.38 and −2.10 mV. Thus, the increase in the cytotoxic effect detected from using tyrosine-loaded NPs in conjunction with AF is probably not due to changes in electrostatic interactions. The use of NPs containing amino acids guaranteed a constant production and/or delivery of chloramines to tumoral cells, which is reflected in increased cellular mortality. In fact, using DC in conjunction with L-tyrosine-loaded PCL NPs reduced B16F10 viability to approximately 50%; this reduction in viability probably resulted from chloramines generated within the electrolytic medium, which led to cell destruction by apoptosis.Citation31,Citation49,Citation50 Interestingly, the total amount of encapsulated amino acid tested was 6000-fold lower than that used in the free amino acid experiment (data not shown), which justified the use of NPs systems to improve the cytotoxicity of DC.
Additionally, necrotic cell death induced by DC is an undesirable aspect of this therapy and seems to be directly associated to DC intensity, polarity, and time of exposure.Citation30 This is an important aspect that cannot be neglected in EChT, due to the triggering of inflammatory response as well as the disarray of tumor structure, which may lead to a systemic spread of tumor cells. The constant release of chloramines obtained from the interaction of amino groups present in the tyrosine and the chlorine species generated by DC is a possible strategy to promote cell death by apoptosis rather than necrosis, using milder DC intensity/time of exposure regimens. Besides, EChT application is most useful in the treatment of solid tumorsCitation51 in which different electrode configurations and DC intensities are applied. This procedure is not standardized in the literature, yet it has presented good results in making inoperable tumors into operable onesCitation52,Citation53 and in destroying tumor mass by necrosis.Citation54
The use of nanotechnology and EChT was first applied in C56BL/6Y black mice previously inoculated with B16F10 cells with the aim to verify the possibility of translating the in vitro model to treatment of in situ melanoma. In this model, the L-tyrosine-loaded PCL NPs must be injected into the solid tumor to avoid NP leakage to normal cells, protecting these cells from DC damage. The preliminary results obtained showed that the association of L-tyrosine-loaded PCL NPs with DC induced an almost total destruction of these cancer cells after 48 h of treatment, as detected by optical and electronic microscopy analyses, with no side effects in important organs, such as liver and breast. The safety of this treatment for normal cells is probably attributed to the use of a biodegradable polymer (PCL) as loader of tyrosine, which is a natural amino acid present in living animals, therefore, avoiding adverse effects common in traditional anticancer treatment. These results are still awaiting confirmation, and there are many challenges that need to be faced to permit the translatability of in vitro results to in vivo models. Before we can consider the use of L-tyrosine-loaded PCL NPs associated with DC for in situ cancer treatment, we must face challenges, such as the need for a higher number of animals to be studied, inoculation of different kinds of tumors, and different electrode configurations and DC intensities among others.
Conclusion
Nanotechnology is generating new perspectives in a number of scientific fields. Its applications in cancer medicine are currently being investigated with very promising results in an in vitro model. The never-before-described use of the combination of this technology with EChT aims to make DC tumor treatment even more efficient and opens the door for a new cancer therapeutic approach. One of the problems associated with EChT is the occurrence of necrosis, which seems to be directly proportional to the DC intensity applied. The use of nanotechnology in conjunction with DC may promote apoptotic cell death over necrotic damage, especially when the AF is applied in the presence of amino acid-loaded NPs. In fact, this hypothesis is being tested in C56BL/6Y black mice previously inoculated with B16F10 cells, and the results obtained so far, however preliminary, are quite successful, without any side effects commonly found in traditional anticancer treatment, probably due to the use of a biodegradable polymer loaded with a natural amino acid.
Acknowledgments
We thank Dr Marcio Lourenço Rodrigues for critical reading of the manuscript. This work was supported by Conselho Nacional de Desenvolvimento Científico e Tecnológico (CNPq), Fundação de Amparo à Pesquisa no Estado do Rio de Janeiro (FAPERJ), and Fundação José Bonifácio (FUJB).
Disclosure
The authors report no conflicts of interest in this work.
References
- Mora-HuertasCEFessiHElaissariAPolymer-based nanocapsules for drug deliveryInt J Pharm20103851211314219819319
- SinghRLillardJWJrNanoparticle-based targeted drug deliveryExp Mol Pathol200986321522319186176
- SoppimathKSAminabhaviTMKulkarniARRudzinskiWEBiodegradable polymeric nanoparticles as drug delivery devicesJ Control Release2001701212011166403
- HansMLLowmanAMBiodegradable nanoparticles for drug delivery and targetingCurr Opin Solid State Mater Sci200264319327
- FengSHuangGEffects of emulsifiers on the controlled release of paclitaxel (Taxol) from nanospheres of biodegradable polymersJ Control Release2001711536911245908
- FarokhzadOCChengJTeplyBATargeted nanoparticle-aptamer bioconjugates for cancer chemotherapy in vivoProc Natl Acad Sci U S A2006103166315632016606824
- AlexisFRheeJWRichieJPRadovic-MorenoAFLangerRFarokhzadOCNew frontiers in nanotechnology for cancer treatmentUrol Oncol2008261748518190835
- ChawlaJSAmijiMMBiodegradable poly(epsilon-caprolactone) nanoparticles for tumor-targeted delivery of tamoxifenInt J Pharm20022491212713812433429
- EspuelasMLegrandPCampaneroMPolymeric carriers for amphotericin B: in vitro activity, toxicity and therapeutic efficacy against systemic candidiasis in neutropenic miceJ Antimicrob Chemother200352341942712888593
- SinhaVRBansalKKaushikRKumriaRTrehanAPoly-epsilon-caprolactone microspheres and nanospheres: an overviewInt J Pharm2004278112315158945
- ParkSJLeeYMHongSKRelease behaviors of porous poly(butylene succinate)/poly(epsilon-caprolactone) microcapsules containing indomethacinColloids Surf B Biointerfaces200647221121516413177
- ZiliZSfarSFessiHPreparation and characterization of poly-epsilon-caprolactone nanoparticles containing griseofulvinInt J Pharm20052941226126715814226
- ShenoyDBAmijiMMPoly(ethylene oxide)-modified poly(epsilon-caprolactone) nanoparticles for targeted delivery of tamoxifen in breast cancerInt J Pharm20052931226127015778039
- SinghJPanditSBramwellVWAlparHODiphtheria toxoid loaded poly-(epsilon-caprolactone) nanoparticles as mucosal vaccine delivery systemsMethods20063829610516442811
- YangJParkSBYoonHGHuhYMHaamSPreparation of poly epsilon-caprolactone nanoparticles containing magnetite for magnetic drug carrierInt J Pharm2006324218519016872766
- YeniceIMocanMPalaskaEHyaluronic acid coated poly-epsilon-caprolactone nanospheres deliver high concentrations of cyclosporine A into the corneaExp Eye Res200887316216718675411
- BenoitMABarasBGillardJPreparation and characterization of protein-loaded poly(epsilon-caprolactone) microparticles for oral vaccine deliveryInt J Pharm19991841738410425353
- BermanBPerezOZellDImmunological strategies to fight skin cancerSkin Therapy Lett20061151716820869
- TewesFMunnierEAntoonBComparative study of doxorubicin-loaded poly(lactide-co-glycolide) nanoparticles prepared by single and double emulsion methodsEur J Pharm Biopharm200766348849217433641
- MiklavcicDJarmTCemazarMTumor treatment by direct electric current. Tumor perfusion changesBioelectrochem Bioenerg199743253256
- NilssonEvon EulerHBerendsonJElectrochemical treatment of tumoursBioelectrochemistry200051111110790774
- CiriaHCQuevedoMSCabralesLBAntitumor effectiveness of different amounts of electrical charge in Ehrlich and fibrosarcoma Sa-37 tumorsBMC Cancer200448715566572
- NordenströmBEWBiologically closed electric circuits: activation of vascular interstitial closed electric circuits for treatment of inoperable cancersBioelectromagnetics19843137153
- NordenströmBEElectrochemical treatment of cancer. I: Variable response to anodic and cathodic fieldsAm J Clin Oncol19891265305362556014
- NordenströmBESurvey of mechanisms in electrochemical treatment (ECT) of cancerEur J Surg Suppl1994574931097531033
- NordenströmBEWPreliminary clinical trials of electrophoretic ionization in the treatment of malignant tumorsIRCS Med Sci Biomed Technol19786537540
- NordenströmBEEksborgSBevingHElectrochemical treatment of cancer. II: Effect of electrophoretic influence on adriamycinAm J Clin Oncol199013175882305721
- HolandinoCVeigaVFRodriguesMLMoralesMMCapellaMAAlvianoCSDirect current decreases cell viability but not P-glycoprotein expression and function in human multidrug resistant leukemic cellsBioelectromagnetics200122747047811568932
- VeigaVHolandinoCRodriguesMCapellaMMenezesSAlvianoCCellular damage and altered carbohydrate expression in P815 tumor cells induced by direct electric current: an in vitro analysisBioelectromagnetics200021859760711102950
- VeigaVFNimrichterLTeixeiraCAExposure of human leukemic cells to direct electric current: generation of toxic compounds inducing cell death by different mechanismsCell Biochem Biophys2005421617415673929
- WagnerBABritiganBEReszkaKJMcCormickMLBurnsCPHydrogen peroxide-induced apoptosis of HL-60 human leukemia cells is mediated by the oxidants hypochlorous acid and chloraminesArch Biochem Biophys2002401222323412054473
- EnglertRPShacterEDistinct modes of cell death induced by different reactive oxygen species: amino acyl chloramines mediate hypochlorous acid-induced apoptosisJ Biol Chem200227723205182052611925431
- SantoyoSGa de JalónEYgartuaPRenedoMBlanco-PríetoMOptimization of topical cidofovir penetration using microparticlesInt J Pharm200224212107113
- HasanASSochaMLamprechtAEffect of the microencapsulation of nanoparticles on the reduction of burst releaseInt J Pharm2007344125361
- Ricci-JúniorEMarchettiJMZinc(II) phthalocyanine loaded PLGA nanoparticles for photodynamic therapy useInt J Pharm20063101218719516427222
- FonsecaMEFrimerNMendonçaRECouceiroJNMachadoRDA combined staining technique developed for virus particle observation in the electron microscopeRev Bras Biol198444137406206525
- FreshneyNWGoonesekeraSDFeigLAActivation of the exchange factor Ras-GRF by calcium requires an intact Dbl homology domainFEBS Lett199740711111159141492
- HolandinoCVeigaVFCapellaMMMenezesSAlvianoCSDamage induction by direct electric current in tumoural target cellsIndian J Exp Biol200038655455811116525
- MosmannTRapid colorimetric assay for cellular growth and survival: application to proliferation and cytotoxicity assaysJ Immunol Methods1983651255636361138
- MeiraDDMarinho-CarvalhoMMTeixeiraCAClotrimazole decreases human breast cancer cells viability through alterations in cytoskeleton-associated glycolytic enzymesMol Genet Metab200584435436215781197
- KongGBraunRDDewhirstMWHyperthermia enables tumor-specific nanoparticle delivery: effect of particle sizeCancer Res200060164440444510969790
- YangYYChungTSNgNPMorphology, drug distribution, and in vitro release profiles of biodegradable polymeric microspheres containing protein fabricated by double-emulsion solvent extraction/evaporation methodBiomaterials200122323124111197498
- GrafAAblingerEPetersSZimmerAHookSRadesTMicroemulsions containing lecithin and sugar-based surfactants: nanoparticle templates for delivery of proteins and peptidesInt J Pharm20083501235136018191925
- LinceFMarchisioDLBarresiAAStrategies to control the particle size distribution of poly-epsilon-caprolactone nanoparticles for pharmaceutical applicationsJ Colloid Interface Sci2008322250551518402975
- HolandinoCCapellaMAAnglusterJSilva-FilhoFCMenezesSAlvianoCSCell surface alterations induced by methylene blue and direct electric current in Escherichia coliIndian J Biochem Biophys199835528429010410461
- JanigroDPerjuCFazioVAlternating current electrical stimulation enhanced chemotherapy: a novel strategy to bypass multidrug resistance in tumor cellsBMC Cancer200667216545134
- ColomboLGonzálezGMarshallGIon transport in tumors under electrochemical treatment: in vivo, in vitro and in silico modelingBioelectrochemistry200771222323217689151
- SersaGMiklavcicDCemazarMRudolfZPuciharGSnojMElectrochemotherapy in treatment of tumoursEur J Surg Oncol200834223224017614247
- PeskinAVWinterbournCCHistamine chloramine reactivity with thiol compounds, ascorbate, and methionine and with intracellular glutathioneFree Radic Biol Med200335101252126014607524
- KlamtFShacterETaurine chloramine, an oxidant derived from neutrophils, induces apoptosis in human B lymphoma cells through mitochondrial damageJ Biol Chem200528022213462135215799967
- SalzbergMKirsonEPaltiYRochlitzCA pilot study with very low-intensity, intermediate-frequency electric fields in patients with locally advanced and/or metastatic solid tumorsOnkologie20083136236518596382
- GriffinDTDoddNJMooreJVPullanBRTaylorTVThe effects of low level direct current therapy on a preclinical mammary carcinoma: tumor regression and systemic biochemical sequelaeBr J Cancer19946958758788180017
- FoshBGFinchJGLeaMUse of electrolysis as an adjunct to liver resectionBr J Surg2002898999100212153624
- PlesnicarASersaGVodovnikLJancarJZaletel-KrageljLPlesnicarSElectric treatment of human melanoma skin lesions with low level direct electric current: an assessment of clinical experience following a preliminary study in five patientsEur J Surg Suppl199457445497531020