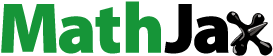
Abstract
Currently, therapy for thyroid cancer mainly involves surgery and radioiodine therapy. However, chemotherapy can be used in advanced and aggressive thyroid cancer that cannot be treated by other options. Nevertheless, a major obstacle to the successful treatment of thyroid cancer is the delivery of drugs to the thyroid gland. Here, we present an example of the construction of silicon dioxide nanoparticles with thyroid–stimulating-hormone receptor-targeting ligand that can specifically target the thyroid cancer. Doxorubicin nanoparticles can be triggered by acid to release the drug payload for cancer therapy. These nanoparticles shrink the tumor size in vivo with less toxic side effects. This research paves the way toward effective chemotherapy for thyroid cancer.
Introduction
Thyroid cancer is a rare disease where the tumor is located within the thyroid gland.Citation1–Citation3 Currently, thyroid cancer is mainly treated by surgery and radioiodine therapy (I-131 therapy) due to the excessive need for absorption and concentration of iodine in thyroid cells.Citation4–Citation6 Besides surgery and radioiodine therapy, chemotherapy – although uncommonly used for the treatment of thyroid cancer – could be used in combination with external beam radiation therapy for anaplastic thyroid cancer and other advanced cancers that are untreatable by other treatments.Citation7,Citation8 One major obstacle in thyroid cancer treatment is the specific delivery of the required drug to the thyroid gland, making drug delivery extremely essential and critical in this particular cancer.
It is well known that the thyroid–stimulating hormone receptor (TSHr), a glycoprotein G-protein-coupled receptor, is overexpressed in the plasma membrane of thyrocytes, especially in aggressive thyroid tumors.Citation9–Citation13 TSHr can specifically bind the thyroid-stimulating hormone (TSH). This specific binding behavior of TSHr with TSH can be adapted for generating a nanoparticle (NP) drug-delivery system to target thyroid cancer.Citation14,Citation15 Indeed, liposomes with gemcitabine and lipid/polymer NPs with cisplatin were developed and TSH was introduced onto these NPs for targeting thyroid cancer. Elevated anticancer effect and increased thyroid localization of the NPs were reported.Citation14–Citation16 However, to further reduce the side effects but to increase the therapeutic efficacy of NPs, triggered release of the anticancer drug is much more desirable.Citation17–Citation25
Materials and methods
Doxorubicin, (Dox) cis-aconitic anhydride, and silicon dioxide (SiO2) with amine group (NH2) groups on the surface were purchased from Sigma-Aldrich. Thyroid–stimulating hormone (TSH) (from human pituitary), 5-carboxyfluorescein (5′-FAM), succinimidyl ester (SE) were also purchased from Sigma-Aldrich Co. (St Louis, MO, USA). Succinimidyl carboxyl methyl ester (mPEG-NHS) and orthopyridyl disulfide PEG Succinimidyl ester (PDP-PEG-NHS) were purchased from Creative PEGWorks (Chapel Hill, NC, USA). All other chemicals were of reagent grade.
Preparation of SiO2/Dox and TSH-SiO2/Dox
PEGylating of the SiO2 NPs (SiO2@PEG)
First, SiO2 (5 mg) with NH2 groups on the surface was dispersed in water in a flask, to which mPEG-NHS (20 mg) was added. The reaction mixture was stirred for 6 h and subjected to dialysis against water in a dialysis bag (molecular cut-off 3,000 Da).
Synthesis of Dox-COOH
Doxorubicin hydrochloride (5 mg) was dissolved in dry dimethyl sulfoxide (DMSO) at room temperature in a dry glass bottle. Hereafter, 5 µL trimethylamine and cis-aconitic anhydride (1.3 mg) were added with stirring. The reaction mixture was stirred overnight and left for conjugation with SiO2 NPs without further purification.
Preparation of Dox-loaded SiO2 NPs (SiO2@Dox)
The DMSO solution containing Dox-COOH prepared in the previous step was used directly for 1-Ethyl-3-(3-dimethylaminopropyl)carbodiimide and n-hydroxysuccinimide (EDC/NHS) activation. EDC (3.2 mg) and NHS (2 mg) was added to the Dox-COOH solution for 2 h. The activated Dox-COOH was further poured into the SiO2@PEG solution purified by dialysis. The reaction mixture was stirred for 6 h and subjected to ultracentrifugation and washing several times with phosphate buffered saline (PBS) to remove the un-reacted Dox-COOH. The unreacted Dox-COOH was collected and quantified by UV-Vis spectrometry.
Preparation of TSH-targeted Dox-loaded SiO2 NPs (TSH-SiO2@Dox)
The preparation of TSH-SiO2@Dox was similar to that of un-targeted SiO2@Dox. A difference is in the preparation of SiO2@PEG. During the preparation process, instead of using only mPEG-NHS, both PDP-PEG-NHS (2 mg) and mPEG-NHS (18 mg) were used. The conjugation of Dox is the same. The unreacted Dox-COOH was collected and quantified by UV-Vis spectrometry. To conjugate TSH to the NPs, they were first treated with 50 mM dithiothreitol (DTT) solution for 30 min. To remove the free DTT, the NPs were ultracentrifuged and washed several times. Thereafter, TSH was added into the solution for ligand conjugation. The NPs thus prepared were collected by ultracentrifugation, and the unconjugated TSH was removed by washing away. The unconjugated TSH could be quantified by enzyme-linked immunosorbent assay (ELISA).
Characterization of NPs by dynamic light scattering (DLS) and transmission electron microscopy (TEM)
The size and zeta potential of the NPs prepared was measured by Zetasizer ZS Nano (Malvern Instruments, Malvern, Worcestershire, UK). The morphology of the NP was observed using a JEOL JEM-200CX instrument (JEOL, Tokyo, Japan).
Acid-triggered release of Dox from the NPs
The release of Dox from the NPs was performed using a dialysis method: 5 mL TSH-SiO2/Dox at a known Dox concentration was put into a dialysis bag (molecular cut-off 3,000 Da). The dialysis bag was thoroughly sealed against any leakage. To monitor the release of Dox, two buffered solutions were used. One was acetate-buffered solution at pH 5.0 and the other was PBS at pH 7.0. The dialysis bag was dialyzed against 50 mL of buffered solution. At a preferable time point, 300 µL of solution was collected for UV-Vis absorbance measurement. The cumulative Dox release was calculated.
Cell lines
FTC-133 human follicular thyroid carcinoma cells were purchased from Sigma Aldrich, and Chinese hamster ovary (CHO) cells were provided by the American Type Culture Collection (Manassas, VA, USA). CHO cells with TSHr (CHO/TSHr+) and CHO cells without TSHr (CHO/TSHr−) cell lines were grown in nutrient mixture F-12 medium with 10% fetal bovine serum and 1% penicillin–streptomycin (P/S). Moreover, FTC-133 thyroid cancer cells were incubated in Dulbecco’s Modified Eagle Medium/nutrient mixture F-12 supplemented with glutamate, D-glucose, pyruvate, and other regular ingredients of normal growth media. The cells were grown in ambient conditions, the media was changed, and cells were sub-cultured from time to time.
Intracellular uptake of SiO2@Dox and TSH-SiO2@Dox by CHO/TSHr+ and CHO/TSHr− cells
CHO/TSHr+ and CHO/TSHr− were seeded in six-well plates at 0.5 million cells per well overnight. SiO2/Dox and TSH-SiO2/Dox at 5 µg Dox/mL were added to the wells, respectively, with PBS as a control. After 3 h, the cells were thoroughly washed by PBS three times and then the cells were trypsinized and collected and fixed by 4% paraformaldehyde. The fluorescence from Dox can be measured by flow cytometry (BD Biosciences, Franklin Lakes, NJ, USA) gated by the PBS-treated group.
Competitive uptake inhibition of TSH-SiO2@Dox by blocking with free TSH
CHO/TSHr+ cells were seeded in six-well plates at 0.5 million cells per well overnight. Prior to NP treatment, free TSH (10 µU/mL) was added to each well to block the surface TSHr. Thereafter, SiO2/Dox and TSH-SiO2/Dox at 5 µg Dox/mL were added to the wells, respectively, with PBS as a control. After 3 h, the cells were thoroughly washed by PBS three times and then trypsinized and collected before fixation by 4% paraformaldehyde. The fluorescence from Dox can be measured by flow cytometry (BD Biosciences, Franklin Lakes, NJ, USA), gated by the PBS-treated group.
TSH-SiO2@Dox by flow cytometry and confocal laser scanning microscopy
To study the co-localization of the NPs and the drug Dox, NPs were first labeled with 5′-FAM by incubating with 5′-FAM-NHS. Un-reacted 5′-FAM was washed away by ultracentrifugation. FTC-133 cells were seeded in six-well plates at a density of 0.5 million per well. Cells were then treated with TSH-SiO2/Dox/5′-FAM, with PBS as a control. After 3 h, the cells were thoroughly washed by PBS three times and then trypsinized and collected prior to being fixed by 4% paraformaldehyde. The fluorescence from Dox (red) and 5′-FAM (green) can be measured by flow cytometry (BD Biosciences).
To study the co-localization of Dox (red) and the NPs by 5′-FAM (green), confocal laser scanning microscopy was used. FTC-133 cells were seeded in six-well plates with a cover slide at a density of 0.2 million per well. Cells were then treated with TSH-SiO2/Dox/5′-FAM. After 3 h, the cells were thoroughly washed by PBS three times and stained by Hoechst 3342, fixed by 4% paraformaldehyde. The co-localization of drug and NPs can be visualized by Dox (red) and 5′-FAM (green) via confocal laser scanning microscopy (FLV-1200).
In vitro cytotoxicity study
FTC-133 cells were seeded in 96-well plates overnight. The cells were then treated with free Dox, SiO2@Dox, and TSH-SiO2@Dox at a preferable drug concentration. After 72 h, 10 µL of 5 mg/mL MTT solution (PBS) was added into each well. After incubation for 4 h, 100 µL acidified sodium dodecyl sulfate solution was added into each well. Overnight, the absorbance at 570 nm of each well was read by a microplate reader. Background absorbance at 650 nm was also read and subtracted.
Colony formation assay
FTC-133 cells were seeded in six-well plates at a density of 2,000 cells per well overnight. Cells were then treated with free Dox, SiO2@Dox, and TSH-SiO2@Dox at a concentration of 0.05 µM. The cells were then left to grow for 2 weeks. Colonies were fixed with glutaraldehyde (6.0% v/v) and stained with crystal violet (0.5% w/v) for 30 min. The cells were then thoroughly washed by water. The colonies were counted using a stereomicroscope.
Apoptosis
FTC-133 cells were seeded in six-well plates at a density of 0.5 million cells per well overnight. Cells were then treated with free Dox, SiO2@Dox, and TSH-SiO2@Dox at a concentration of 10 µM for 24 h. Cells were then washed by PBS three times, trypsinized, and stained by an Annexin-V/fluorescein isothiocyanate apoptosis kit (Abcam, Cambridge, UK). The apoptosis was measured by flow cytometry.
Use of animals
The animal study was approved by the Bai Qiu En Medical Committee on Animal Use of the China–Japan Union Hospital and was carried out in accordance with the China–Japan Union Hospital animal protocol and guidelines (2016SSH0816) for the welfare and ethics of experimental animals. All animals were grouped and housed in cages, provided free access to standard diet and water, and raised under constant conditions.
In vivo anticancer efficacy study
NOD SCID mice were used to develop the thyroid cancer model. FTC-133 cancer cells at a density of 2 million were subcutaneously injected into the right flank of mice. When the tumor grew to ~200 mm3, treatment was started. Mice were randomly grouped into four groups, with six mice in each group (n=6). They were treated with PBS (control), free Dox, SiO2@Dox, and TSH-SiO2@Dox at a dose of 5 mg Dox/kg body weight. Mice were treated at days 0, 6, and 12. Tumor size was measured by callipers, and body weight was monitored every 2 days. The tumor size was calculated by the equation:Citation40–Citation42
where V is the volume of tumor, L and W are the longest and shortest diameter of the tumor, respectively. At the end of the study, tumors in each group were collected and weighed. For the cardiotoxicity study by creatinine kinase (CK) measurement, healthy 4- to 6-week-old Balb/c mice were purchased. Mice were randomly grouped into four groups with three mice in each group (n=3). They were treated with PBS (control), free Dox, SiO2@Dox, and TSH-SiO2@Dox at a dose of 5 mg Dox/kg body weight. Mice were treated at days 0 and 6, killed after 2 weeks, and blood samples were collected.
Statistical analyses
Data are expressed as mean ± standard deviation (SD). Statistical analysis was performed using the Student’s t-test. Significance is defined as by #P>0.05, *P<0.05, **P<0.01, and ***P<0.001.
Results and discussion
Here, we describe a silicon dioxide NP for acid-triggered release of Dox for thyroid cancer therapy. Following a well-reported strategy, Dox was firstly conjugated to cis-aconitic anhydride and this Dox prodrug (Dox-COOH)Citation26 was further conjugated to a PEGylated silicon dioxide NP (SiO2). For thyroid targeting, TSH was linked to NPs via disulfide bond formation (). The TSH-SiO2@Dox thus prepared then showed excellent targeting to the thyroid cancer, with enhanced cytotoxicity and antitumor effect in vivo.
Initially, TSH-SiO2@Dox NPs were characterized by TEM and DLS. Representative images and curves are shown in . TSH-SiO2@Dox-NPs were spherical in morphology, with a mean diameter of 35 nm on TEM. DLS showed that TSH-SiO2@Dox-NPs measured 68 nm in diameter, corresponding to the TEM result. The size discrepancy between TEM and DLS may be due to the dried samples for TEM as compared to the more stretched NPs tested in solution for DLS. By monitoring the UV-Vis absorbance of Dox in the washed-away supernatant, we calculated the drug loading of Dox in the final TSH-SiO2@Dox-NPs as 3 w/w% and loading efficiency at 45.4%. Moreover, following a similar method, the TSH loading was ascertained as 0.25 w/w% by ELISA. Acid-responsive drug delivery would be beneficial for cancer therapy due to the acidic tumor microenvironment.Citation27–Citation30 To show the acid-triggered release of Dox from the NPs, TSH-SiO2@Dox-NPs were put into dialysis bags (molecular weight cut-off 3,500 Da) and then incubated in acetate-buffered solution (pH =5.0) and phosphate-buffered solution (PBS; pH =7.4), respectively. These two pH values at 5.0 and 7.4 could simulate the pH values in the blood circulationCitation31 and within tumor cells.Citation32,Citation33 The cumulative release of Dox outside the dialysis bag was then measured by a UV-Vis spectrometer. As shown in , rapid release of Dox at pH 5.0 was achieved. However, at pH 7.4, even at 72 h, ~40% loaded Dox was released. The fast release of Dox at pH 5.0 can be attributed to the acid-responsive linkage between Dox and cis-aconitic acid. The slow release of the drug at pH 7.4 as well as the triggered release of payload at pH 5.0 could be beneficial for avoiding premature release, prolonging the blood circulation half-life (t1/2) and eventually delivering more drug to tumor sites.
Figure 1 Characterization of TSH-SiO2@Dox. Representative TEM image (A) and DLS curve (B) of TSH-SiO2@Dox NPs. Scale bar =100 nm. Due to the presence of cis-aconitic linkage between the SiO2 and Dox, release of Dox can be triggered by the acidic environment in tumor cells, which was simulated by the acid-triggered drug release as shown in (C).
Abbreviations: DLS, dynamic light scattering; Dox, doxorubicin; NP, nanoparticle; PDI, polydispersity index; SiO2, silicon dioxide; TEM, transmission electron microscopy; TSH, thyroid–stimulating hormone.
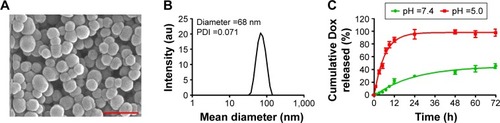
To show the possible targeting effect of TSH-SiO2@Dox NPs, the uptake of NPs on Chinese hamster ovary (CHO) cells, both without (CHO/TSHr−) and with (CHO/TSHr+) TSHr, was monitored by flow cytometry through monitoring the fluorescence from Dox. Results in showed that the cellular uptake of TSH-SiO2@Dox NPs was greater than that of SiO2@Dox NPs and on CHO/TSHr+. However, this was almost the same on the CHO/TSHr− cells, suggesting TSHr-dependent uptake. To further show the specific binding of TSH-SiO2@Dox NPs onto the CHO/TSHr+ cells, free TSH was added to the CHO/TSHr+ cells to competitively bind to the TSHr and the cell uptake was also tested. Results in demonstrated that, in the presence of a competitive inhibitor of free TSH, the uptake of SiO2@Dox and TSH-SiO2@Dox was almost similar, further supporting TSHr-dependent targeting of TSH-SiO2@Dox.
Figure 2 Targeting effect of TSH-SiO2@Dox NPs. Monitoring targeting effect of TSH-SiO2@Dox by flow cytometry as compared to SiO2@Dox uptake by CHO/TSHr+ cells (A) and CHO/TSHr− cells (B). To show the targeting effect, free TSH was added to the cells to competitively bind to the TSHr. In the presence of TSH, SiO2@Dox and TSH-SiO2@Dox showed minimal difference in uptake (C). Data are shown as mean ± SD (n=3). Significance is defined by #P>0.05 and ***P<0.001.
Abbreviations: CHO, Chinese hamster ovary; CHO/TSHr−, CHO cells without TSHr; CHO/TSHr+, CHO cells with TSHr; Dox, doxorubicin; NP, nanoparticle; PBS, phosphate buffered saline; SD, standard deviation; SiO2, silicon dioxide; TSH, thyroid–stimulating hormone; TSHr, TSH receptor.
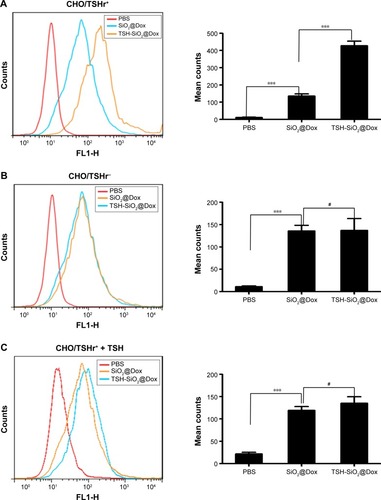
To visualize the uptake of TSH-SiO2@Dox NPs, imaging of the FTC-133 thyroid cancer cells by confocal laser scanning microscopy (CLSM) was performed (). To visualize the drug Dox (red fluorescence) and the NP simultaneously, the NP was labeled by 5′-FAM (green). Hoechst 33342 stained the cell nucleus (blue). The co-localization of the red and green fluorescence in the cell plasma confirmed the simultaneous uptake of NP with Dox. To quantify this, the uptake of the 5′-FAM-labeled TSH-SiO2@Dox NPs by flow cytometry () showed time-dependent internalization of the NPs of both 5′-FAM (green) and Dox (red) from 30 min to 3 h, denoting the simultaneous uptake of NPs with Dox. This simultaneous uptake indicates the stability of NPs, with minimal release of Dox before they entered the cells.
Figure 3 A study of the uptake of TSH-SiO2/Dox/5′-FAM. Confocal laser scanning of cells treated with TSH-SiO2/Dox/5′-FAM after 3 h. The targeting NPs were labeled by 5′-FAM (green). Hoechst stained the cell nucleus. Red fluorescence came from Dox. Scale bars =20 µM (A). To quantitatively monitor the NPs and the drug, cells were treated with TSH-SiO2/Dox/5′-FAM, and then red and green fluorescence were monitored by flow cytometry. PBS-treated group (left), TSH-SiO2@Dox/5′-FAM-treated group at 30 min (middle) and 3 h (right) (B).
Abbreviations: 5′-FAM, 5-carboxyfluorescein; Dox, doxorubicin; NP, nanoparticle; PBS, phosphate buffered saline; SiO2, silicon dioxide; TSH, thyroid–stimulating hormone.
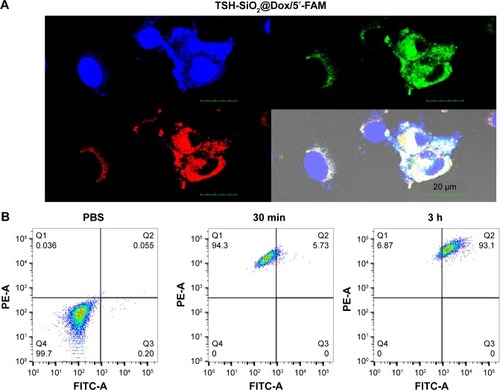
To further study the anticancer drug efficacy in vitro, an MTT assay on FTC-133 thyroid cancer cells was performed on Dox, SiO2/Dox, and TSH-SiO2/Dox (). Compared to the cell viability curve of Dox, the cell viability curve of SiO2/Dox shifted down, indicating the higher potency of SiO2/Dox. Moreover, TSH-SiO2/Dox showed greatest toxicity toward FTC-133 cells. This could be due to the targeting effect of TSH. Further, the IC50 values can be calculated on the basis of the cell viability curve. The IC50 values of Dox, SiO2/Dox, and TSH-SiO2/Dox on FTC-133 cells were 2.32, 0.96, and 0.32 µM, respectively. Targeted NPs TSH-SiO2/Dox increased the potency of Dox ~7.3-fold, whereas this was only ~3-fold for the non-targeted NPs SiO2/Dox, clearly suggesting the benefit of TSH targeting. Subsequently, clonogenic assay assessment of the reproductive viability and colony-forming ability was performed on cells treated with Dox, SiO2/Dox, and TSH-SiO2/Dox, with PBS as a control. As shown in , all drug-treated groups including Dox, SiO2/Dox, and TSH-SiO2/Dox showed less formation of colonies. Moreover, TSH-SiO2/Dox had less colonies than Dox- and SiO2/Dox-treated cells, further indicating the greater potency of TSH-SiO2/Dox via tumor targeting.
Figure 4 In vitro evaluation of the anticancer efficacy of TSH-SiO2@Dox on thyroid cancer cells. Cell viability curve of Dox, SiO2@Dox, and TSH-SiO2/Dox at 72 h (A) and relative cell colonies formation by cells treated with Dox, SiO2@Dox, and TSH-SiO2/Dox, respectively, at an equal Dox concentration at 0.05 µM (B). (C and D) Apoptosis rate of the cells treated with Dox, SiO2@Dox, and TSH-SiO2/Dox. Data shown as mean ± SD (n=3). Significance is defined by *P<0.05, **P<0.01, and ***P<0.001.
Abbreviations: Dox, doxorubicin; NP, nanoparticle; PBS, phosphate buffered saline; SD, standard deviation; SiO2, silicon dioxide; TSH, thyroid–stimulating hormone.
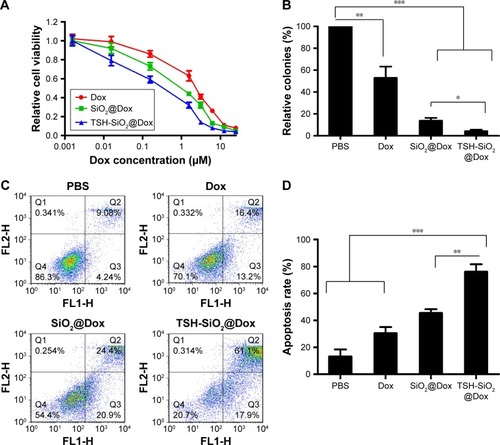
Doxorubicin acts through topoisomerase II inhibition and free radical generation, eventually inducing apoptosis.Citation34 To compare the apoptosis-inducing effect of Dox, SiO2/Dox, and TSH-SiO2/Dox, FTC-133 thyroid cells were treated with these drugs at an equal concentration of Dox. As shown in , Dox induced an apoptosis rate of 29.6%, whereas this was 45.3% and 79.0% for SiO2/Dox and TSH-SiO2/Dox respectively, indicating the advantage of TSH-SiO2/Dox over Dox and SiO2/Dox.
Finally, to show the benefits of TSH-mediated targeting of TSH-SiO2/Dox on the thyroid cancers, an FTC-133 subcutaneous xenograft model was established. Mice were intravenously injected three times (days 0, 6, and 12) with either PBS or the agents Dox, SiO2@Dox, and TSH-SiO2/Dox respectively. The drug Dox dose for mice was 5 mg Dox/kg body weight. Subsequently, the tumor burden and relative body-weight change were monitored every other day. At day 21, the mice were euthanized and the tumors in each group were collected and weighed. As shown in , tumors in PBS-treated mice grew very rapidly. At the end of this study, tumor size was >3,500 mm3. Dox-treated mice showed lesser tumor growth compared to the PBS group; at the endpoint, tumor size was ~1,500 mm3. However, SiO2@Dox showed a slightly better antitumor effect in the FTC-133 cancer model. Last, but not the least, tumor growth in the TSH-SiO2/Dox-treated mice was almost arrested. This result indicated the greater tumor inhibitory ability of TSH-SiO2/Dox. This is possibly due to the targeting effect of TSH on the NPs. Conventional chemotherapeutic agents are known to bring great side effects to animals and human beings. To assess side effects caused by drug treatment, the body weight of the mice in each group was monitored. As shown in , the body weight of the PBS-treated group increased. To quantify antitumor efficacy, at the end of study, all mice were sacrificed and the tumors were collected. The mass in each group is shown in . Mice treated with Dox showed a mean tumor weight of 2.3 g, compared to that of 4.23 g in mice treated with PBS. TSH-SiO2/Dox-treated mice showed a tumor weight of 0.78 g, which was <1.45 g that of the SiO2/Dox-treated group, indicating better antitumor efficacy.
Figure 5 In vivo evaluation of TSH-SiO2/Dox on FTC-133 xenograft model. Tumor shrinkage by TSH-SiO2/Dox as compared to PBS, Dox, and SiO2/Dox-treated mice (n=5, each group). Mice were intravenously injected with either PBS (PBS group) or an equal Dox dose of 5 mg/kg body weight (drug-treatment groups) on days 0, 6, and 12 (A). The body weight change of the mice is shown in B. At the end of this study, mice were sacrificed and tumors were collected and weighed (C). Cardiotoxicity as indicated by the CK is shown in D. Significance is defined by *P<0.05, **P<0.01, and ***P<0.001.
Abbreviations: CK, creatinine kinase; Dox, doxorubicin; NP, nanoparticle; PBS, phosphate buffered saline; SD, standard deviation; SiO2, silicon dioxide; TSH, thyroid–stimulating hormone.
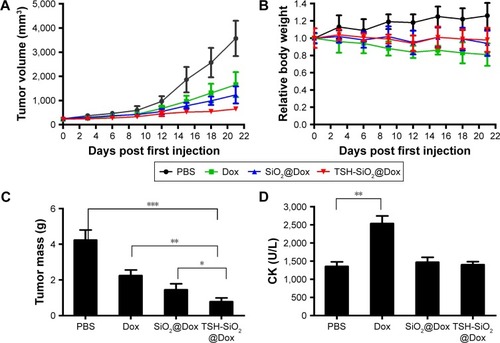
Scheme 1 Synthesis of Dox prodrug and preparation of thyroid-targeting NPs TSH-SiO2@Dox. Dox was firstly conjugated with cis-aconitic anhydride to prepare Dox-COOH (A). SiO2 was PEGylated with mPEG-NHS and PDP-PEG-NHS. PEGylated SiO2 with PDP groups (SiO2@PDP) was further conjugated with Dox and TSH. Targeted NPs with Dox and TSH ligand (TSH-SiO2@Dox) were prepared in this way (B).
Abbreviations: Dox, doxorubicin; DTT, dithiothreitol; mPEG-NHS, Succinimidyl carboxyl methyl ester; NP, nanoparticle; PDP-PEG-NHS, orthopyridyl disulfide PEG Succinimidyl ester; PDP, orthopyridyl disulfide; PEG, polyethylene glycol; SiO2, silicon dioxide; TSH, thyroid–stimulating hormone.
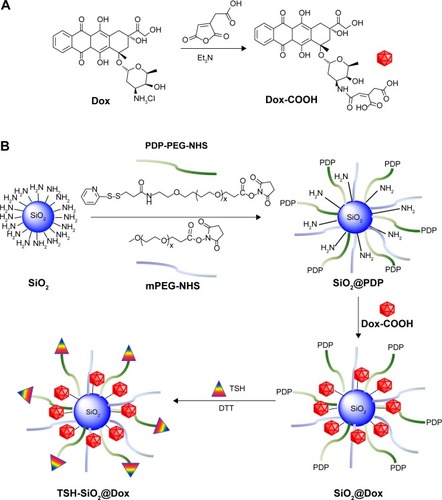
Doxorubicin is known to cause cardiotoxicity.Citation35–Citation38 To unveil whether the NP formulation shifts toxicity, blood CK values were evaluated in the mice treated with PBS, Dox, SiO2@Dox, and TSH-SiO2/Dox, respectively. As shown in , Dox-treated mice showed significantly higher CK values, indicating greater cardiotoxicity. However, both SiO2@Dox- and TSH-SiO2/Dox-treated mice showed lower CK values, which are comparable to those in PBS-treated mice group. This indicated the lower cardiotoxicity induced by these two treatments, suggesting the ability of SiO2 NP to reduce toxicity.
Conclusion
Here we showed the possible targetingCitation39 of thyroid cancer by constructing a TSH-loaded NP delivery system with Dox. By introducing an acid-liable linker between Dox and the NP, acid-triggered release of Dox was achieved. TSH can be attached onto the surface of the NPs by disulfide bond formation. This TSH-SiO2/Dox preferentially accumulates in the TSHr+ cells for targeting thyroid cancers. We then showed cancer targeting by confocal laser scanning microscopy as well as better in vitro anticancer efficacy of TSH-SiO2/Dox over free Dox and non-targeted NPs. This enhanced efficacy was further proved by a greater ability to inhibit the cancer cell colony-formation assay and elevated apoptosis. Further in vivo evaluation of TSH-SiO2/Dox confirmed the possible targeting effect of TSH-SiO2/Dox on thyroid cancers, with better tumor inhibition rate and lower toxicity, which opens the door for the treatment of thyroid cancer in the clinic.
Acknowledgments
The authors gratefully acknowledge the support from the Youth Foundation of the Science and Technology Department of Jilin province (#201201046).
Disclosure
The authors report no conflicts of interest in this work.
References
- LamKYLoCYMetastatic tumors of the thyroid gland: a study of 79 cases in Chinese patientsArch Pathol Lab Med1998122137419448014
- ChenHNicolTLUdelsmanRClinically significant, isolated metastatic disease to the thyroid glandWorld J Surg19992321771809880428
- RokaSKornekGSchüllerJOrtmannEFeichtingerJArmbrusterCCarcinoma showing thymic-like elements – a rare malignancy of the thyroid glandBr J Surg200491214214514760659
- MazzaferriELKloosRTClinical review 128: current approaches to primary therapy for papillary and follicular thyroid cancerJ Clin Endocrinol Metab20018641447146311297567
- Fard-EsfahaniAEmami-ArdekaniAFallahiBAdverse effects of radioactive iodine-131 treatment for differentiated thyroid carcinomaNucl Med Commun201435880881724751702
- WillegaignonJSapienzaMOnoCOutpatient radioiodine therapy for thyroid cancer: a safe nuclear medicine procedureClin Nucl Med201136644044521552020
- ShermanSIThyroid carcinomaLancet2003361935650151112583960
- AinKBAnaplastic thyroid carcinoma: behavior, biology, and therapeutic approachesThyroid1998887157269737368
- NeumannSHuangWTitusSSmall-molecule agonists for the thyrotropin receptor stimulate thyroid function in human thyrocytes and miceProc Natl Acad Sci U S A200910630124711247619592511
- Pujol-BorrellRGiménez-BarconsMMarín-SanchezAColobranRGenetics of Graves’ disease: special focus on the role of TSHR geneHorm Metab Res2015471075376626361261
- DorsamRTGutkindJSG-protein-coupled receptors and cancerNat Rev Cancer200772799417251915
- YamamotoYSakamotoMFujiiGOverexpression of orphan G-protein-coupled receptor, Gpr49, in human hepatocellular carcinomas with beta-catenin mutationsHepatology200337352853312601349
- YuYZhangQBuscagliaJQuantitative real-time detection of carcinoembryonic antigen (CEA) from pancreatic cyst fluid using 3-D surface molecular imprintingAnalyst2016141144424443127193921
- PaolinoDCoscoDGaspariMTargeting the thyroid gland with thyroid-stimulating hormone (TSH)-nanoliposomesBiomaterials201435257101710924836306
- GaoXJLiAQZhangXLiuPWangJRCiaXThyroid-stimulating hormone (TSH)-armed polymer-lipid nanoparticles for the targeted delivery of cisplatin in thyroid cancers: therapeutic efficacy evaluationRsc Adv20155106413106420
- YuYZhangQChangCCDesign of a molecular imprinting biosensor with multi-scale roughness for detection across a broad spectrum of biomoleculesAnalyst2016141195607561727441317
- SunHGuoBChengRMengFLiuHZhongZBiodegradable micelles with sheddable poly(ethylene glycol) shells for triggered intracellular release of doxorubicinBiomaterials200930316358636619666191
- ChenWMengFChengRZhongZpH-Sensitive degradable polymersomes for triggered release of anticancer drugs: a comparative study with micellesJ Control Release20101421404619804803
- WangSShangLLiLMetal-organic-framework-derived mesoporous carbon nanospheres containing porphyrin-like metal centers for conformal phototherapyAdv Mater201628388379838727461987
- FangWJYangJGongJWZhengNPhoto- and pH-triggered release of anticancer drugs from mesoporous silica-coated Pd@Ag nanoparticlesAdv Funct Mater201222842848
- AhmedFPakunluRISrinivasGShrinkage of a rapidly growing tumor by drug-loaded polymersomes: pH-triggered release through copolymer degradationMol Pharm20063334035016749866
- LiuJBuWPanLShiJNIR-triggered anticancer drug delivery by upconverting nanoparticles with integrated azobenzene-modified mesoporous silicaAngew Chem Int Ed Engl201352164375437923495013
- OhKTOhYTOhNMKimKLeeDHLeeESA smart flower-like polymeric micelle for pH-triggered anticancer drug releaseInt J Pharm20093751–216316919481702
- HeCFWangSHYuYJAdvances in biodegradable nanomaterials for photothermal therapy of cancerCancer Biol Med201613329931227807498
- NaskarJPaluiGBanerjeeATetrapeptide-based hydrogels: for encapsulation and slow release of an anticancer drug at physiological pHJ Phys Chem B200911335117871179219708711
- DuCDengDShanLA pH-sensitive doxorubicin prodrug based on folate-conjugated BSA for tumor-targeted drug deliveryBiomaterials201334123087309723374705
- LiuDFBernuzCRFanJA nano-in-nano vector: merging the best of polymeric nanoparticles and drug nanocrystalsAdv Funct Mater20172791604508
- LiuDZhangHCitoSCore/shell nanocomposites produced by superfast sequential microfluidic nanoprecipitationNano Lett201717260661428060521
- LiuDZhangHMäkiläEMicrofluidic assisted one-step fabrication of porous silicon@acetalated dextran nanocomposites for precisely controlled combination chemotherapyBiomaterials20153924925925468375
- LiuDZhangHHerranz-BlancoBMicrofluidic assembly of monodisperse multistage pH-responsive polymer/porous silicon composites for precisely controlled multi-drug deliverySmall201410102029203824616278
- LiYXiaoWXiaoKWell-defined, reversible boronate crosslinked nanocarriers for targeted drug delivery in response to acidic pH values and cis-diolsAngew Chem Int Ed Engl201251122864286922253091
- VaupelPKallinowskiFOkunieffPBlood flow, oxygen and nutrient supply, and metabolic microenvironment of human tumors: a reviewCancer Res19894923644964652684393
- SawantRMHurleyJPSalmasoS“SMART” drug delivery systems: double-targeted pH-responsive pharmaceutical nanocarriersBioconjug Chem200617494394916848401
- DengSYanTJendrnyCDexrazoxane may prevent doxorubicin-induced DNA damage via depleting both topoisomerase II isoformsBMC Cancer20141484225406834
- OlsonRDMushlinPSDoxorubicin cardiotoxicity: analysis of prevailing hypothesesFASEB J1990413307630862210154
- OlsonRDMushlinPSBrennerDEDoxorubicin cardiotoxicity may be caused by its metabolite, doxorubicinolProc Natl Acad Sci U S A19888510358535892897122
- ArolaOJSarasteAPulkkiKKallajokiMParvinenMVoipio-PulkkiLMAcute doxorubicin cardiotoxicity involves cardiomyocyte apoptosisCancer Res20006071789179210766158
- KalyanaramanBJosephJKalivendiSWangSKonorevEKotamrajuSDoxorubicin-induced apoptosis: implications in cardiotoxicityMol Cell Biochem2002234–2351–2119124
- PolegatoBFMinicucciMFAzevedoPSAcute doxorubicin-induced cardiotoxicity is associated with matrix metalloproteinase-2 alterations in ratsCell Physiol Biochem20153551924193325871735
- TomaykoMMReynoldsCPDetermination of subcutaneous tumor size in athymic (nude) miceCancer Chemother Pharmacol19892431481542544306
- O’DonoghueJABardièsMWheldonTERelationships between tumor size and curability for uniformly targeted therapy with beta-emitting radionuclidesJ Nucl Med19953610190219097562062
- HermannPCHuberSLHerrlerTDistinct populations of cancer stem cells determine tumor growth and metastatic activity in human pancreatic cancerCell Stem Cell20071331332318371365