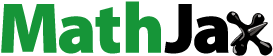
Abstract
Background
The intercalation of an antibiotic drug, cefadroxil (CD), into the inter-gallery of Zn, Al nitrate-layered double hydroxide (LDH) was accomplished using a co-precipitation method. This formed a nanostructured organic–inorganic hybrid material that can be exploited for the preparation of a controlled release formulation.
Materials and methods
The drug–LDH nanohybrid was characterized by using field emission scanning electron microscopy (FE-SEM), energy dispersive X-ray spectroscopy (EDS), Fourier transform infrared spectroscopy (FTIR) thermogravimetric (TG) analysis, X-ray powder diffraction (XRD) and UV–visible (UV–vis) absorption spectroscopy, which confirmed the intercalation process. Release tests of nanohybrid in the presence or absence of NaCl or poly-acrylamide (PAM) were performed in vitro in gastric (pH 1.2), lysosomal (pH 4.0), intestinal (pH 6.8) and blood (pH 7.4) simulated fluid using UV–vis spectroscopy.
Results
At pH 1.2, LDH was dissolved and intercalated antibiotic released from ZnAl-CD in a molecular form, which led to a significant increase in the antibiotic’s solubility. Results showed that the release of drug from nanohybrid at pH 4.0, 6.8 and 7.4 was a sustained process.
Conclusion
This material might reduce side effects by the release of the drug in a controlled manner. However, it was found that the presence of Cl or PAM species in the release media has a negative impact on the release behavior. The weathering mechanism is responsible for the release of CD from the nanocomposite at pH 1.2, while the mechanism of anion exchange may be responsible for the release behavior at pH 4.0, 6.8 and 7.4. A number of kinetic models were chosen to gain more insights into the mechanisms of drug release. At pH 1.2, the zero-order model most satisfactorily explained the release kinetics of CD, while the release data of CD at pH 4.0, 6.8 and 7.4 were governed by Bhaskar kinetics.
Introduction
In recent years, drug administration techniques have received extensive attention from pharmaceutical researchers; in particular, controlled release drug delivery systems release the bioactive agent at a specific rate and at the right place to perform its task with efficiency.Citation1–Citation3 These systems aim to maintain drug concentration in the blood or in target tissues at the desired levels for a longer period of time, to decrease the administration frequency and also minimize possible side effects.Citation4–Citation6
Recently, new materials, namely, nanohybrids or heterostructured nanocomposites, have been widely used in the drug delivery field. Of the heterostructured nanomaterials, layered nanohybrids can be easily manipulated. These materials are typically prepared via intercalation reaction, which can be defined as the incorporation of an organic guest into a two-dimensional (2-D) layered inorganic matrix, yielding interesting hybrid organic/inorganic nanocomposite materials that can used as carriers of drugs and biological active species ().Citation7,Citation8
Figure 1 Schematic illustration of organic intercalation into two-dimensional nanomaterials to obtain an organic–inorganic hybrid nano-composite material.
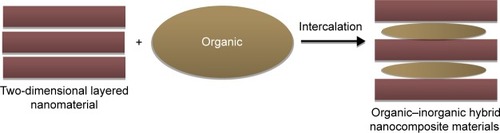
As an important class of inorganic layered nanomaterials, clays have been widely studied as active molecular ion delivery vehicles which can be either cationic with negatively charged aluminosilicate layers or anionic with positively charged hydroxide layers.Citation8,Citation9 Layered double hydroxide (LDH) is a synthetic analog of naturally occurring mineral hydrotalciteCitation10 and has contributed to the development of drug delivery applications due to its advantages such as anion exchange properties,Citation11 low cytotoxicity, simple preparation,Citation12 biocompatibility and biodegradability properties,Citation13 basicity of the matrix,Citation14 and low cost.Citation15 It also leads to the achievement of the following: (i) stabilization of the drugs,Citation16 (ii) improving both their solubility and cellular uptake,Citation15,Citation17 and (iii) controllably releasing them.Citation18
The chemical composition of LDH can be described by the formula [MII1−xMIIIx (OH)2]x+ (An−)x/n⋅mH O, where MII, MIII, and An− represent divalent, trivalent metal ions and an interlayer anion of valence n, respectively. The x value is the molar ratio MIII/(MII + MIII) and m is the amount of water in moles.Citation19 The structure is similar to that of brucite (Mg(OH)2), where a portion of the divalent cations are substituted by trivalent ones, which leads to positively charged sheets.Citation20 These charges are balanced by the interlayer anions.Citation21 Thus, LDH structure is composed of MII and MIII, which can be accommodated in the octahedral sites in the brucite-like layers and the hydroxyl groups located on the vertices of the octahedral.Citation15,Citation19
Due to the effectiveness and importance of biocompatible Zn, Al LDH, we have chosen this material as nanocapsules for cefadroxil (CD). In its ionic form, it is found that zinc plays a vital role in the human body, where it has the ability to prevent DNA damage, as well as being useful in cancer therapy and controlling the activities of many enzymes.Citation22
CD is one of the first-generation cephalosporin β-lactam antibiotics and acts against both Gram-positive and Gram-negative bacteria. Delivery in a nanocapsule may overcome its negative effects and low solubility that lead to poor absorption of drug by living organisms.Citation23
A series of pharmaceutically active compounds including diclofenac, methotrexate, chloramphenicol, salicylate, cefazolin, ketoprofen, lawsone and chlorogenic acid were intercalated into Zn, Al LDHs, and the feasibility of LDH-based drug delivery systems was demonstrated.Citation8,Citation22,Citation24–Citation28 CD has been intercalated into gastroretentive delivery systems (GRDS) in order to analyze its release in acidic pH and to examine the solubility and stability of CD. It was found that the release of CD formulation was sustained for around 14 hours.Citation29 A similar study used hydoxy propyl methyl cellulose as a delivery system for CD to measure the in vitro drug release rate at pH 1.2 and found that the release period was prolonged.Citation30 Conversely, the current study demonstrates that LDH is dissolved rapidly and, consequently, CD is fully released at pH 1.2.
The objectives of this work were to evaluate the ability of LDH to intercalate an antibiotic, and to study the influence of release media pH, and the effect of the presence of exchangeable anions (chloride ions, NaCl) or a macromolecule (poly-acrylamide; PAM) in the release medium, on the release rate and the amount of drug released, as well as the effectiveness of the LDH matrix in increasing solubility of the antibiotic and reducing side effects. In order to investigate these objectives, we have intercalated CD in Zn–Al-LDH, containing nitrate as the interlayer anion, by a co-precipitation method. Then, the prepared CD delivery system was characterized by using different physicochemical techniques. After that, we quantitatively monitored the controlled release process of the drug. Finally, the release profile data were fitted by mathematical models in order to evaluate the CD-release mechanisms and kinetics.
Materials and methods
Materials
The metal salts used in the synthesis of the drug–LDH nanohybrid were zinc nitrate hexahydrate (Zn(NO3)2⋅6H2O, EMD Millipore, Billerica, MA, USA) and aluminum nitrate nonahydrate (Al(NO3)3⋅9H2O, Fluka). CD (C16H17N3O5S, MW 363.392 g/mol)and sodium hydroxide pellets (98%) were obtained from Sigma-Aldrich Co. (St Louis, MO, USA) and BDH Chemical Ltd, respectively. Deionized water (ELGA, specific resistivity 18 MΩ) was used for the preparation of the sample and washing the obtained product. All chemicals in this synthesis were used as received without further purification.
Preparation and characterization
Synthesis of LDH-intercalated drugs
Intercalation of the drug into LDH was carried using the co-precipitation method at constant pH.Citation18,Citation31 A 200 mL solution containing the metal ions (3.751 g of Al(NO3)3⋅9H2O (0.01 mol) and 5.949 g of Zn(NO3)2⋅6H2O (0.02 mol)), was slowly added to the CD solution, which was prepared by dissolving 0.363 g (0.001 mol) of CD in 100 mL of deionized water, Zn2+/Al3+/guest molar ratio 0.2/0.1/0.01. Then the freshly prepared NaOH solution (0.5 M) was added drop by drop to yield pH = 9; when addition was completed the suspension was vigorously stirred at 60°C for 24 h in order to reach equilibrium. Following this, the product was centrifuged at 2,900 rpm for 10 minutes and washed with deionized water three times to remove the unreacted salt ions, impurities and excess drug. The precipitate was dried at room temperature and the sample was named ZnAl-LDH–CD.
Characterization
X-ray diffraction (XRD)
The samples’ crystal structure was recorded by using X-ray diffraction (XRD) with a diffractometer (Thermo Scientific™ ARL™ X’TRA Powder Diffractometer; Thermo Fisher Scientific, Waltham, MA, USA) having λ = 0.154 nm of Cu Kα radiations. The operating current and voltage of the instrument was 50 mA and 40 KV, respectively.
Fourier transform infrared (FTIR)
FTIR spectroscopy was used to obtain direct evidence on the intercalation process as well as the interaction between the drug anions, and LDH and the interlayer water. FTIR spectra of the pristine LDH, and drug–LDH were measured with a Nicolet™ iS™10 FTIR spectrometer (Thermo Fisher Scientific) at 25°C. The spectrum of each sample was collected by cumulating 50 scans, with 32 cm−1 resolution in the mid-infrared (4,000–400 cm−1).
Thermogravimetric (TG) analysis
The TG technique is used to determine the decomposition temperature of the interlayer drug anion, and its content in the ZnAl-CD LDH nanoparticle. Additionally, it can provide information about the thermal stability of drug–LDH. Thermogravimetric (TG) analysis was performed on a Thermal Analysis Q500 (TA Instruments, New Castle, DE, USA) under a nitrogen atmosphere between 25 and 1,000°C at 5°C/minute heating rate.
Ultraviolet–visible (UV–vis) absorption spectroscopy
The potential interactions between host and guest as well as those between guest and guest, stability of the interlayer drug and release from the nanocomposite were studied by UV–vis absorption spectroscopy (GENESYS 10S UV–vis, Thermo Fisher Scientific) using quartz cuvettes.
Field emission scanning electron microscopy (FE-SEM)
The FE-SEM (JSM-7600F, JEOL, Tokyo, Japan) was used to examine the surface morphology of the organic–inorganic nanohybrid.
Scanning electron microscopy/energy-dispersive X-ray spectroscopy (SEM-EDS)
The elemental composition of the drug-LDH sample was examined using EDS (Oxford) operated at 20 kV attached to the SEM (JSM-7600F, JEOL).
Controlled release study of the anions from the nanohybrids into various media
The release experiments were carried out as follows. The release media included buffer A as a simulated gastric fluid (pH 1.2), buffer B as a simulated lysosomal fluid (pH 4.0), buffer C as a simulated intestinal fluid (pH 6.8), and buffer D as a simulated blood fluid (pH 7.4).
A known amount of nanohybrid (10 mg of drug–LDH) was dispersed in release medium solution (100 mL). The mixture was continuously stirred at a slow speed and kept at 37°C. At specified time intervals, a certain volume of solution (2.0 mL) was withdrawn, centrifuged at 1,300 rpm for 100 s and the resulting supernatant was filtered to remove the insoluble particles, then the absorbance of the CD was determined at 230 nm and further the amount of drug was estimated from absorbance using the calibration curve, through which the percentage of drug liberated was calculated.
Release studies also were performed in the presence of NaCl, by dissolving 10 mg of sodium chloride in the flask containing the nanohybrid sample, and analyzing as previously mentioned.
Additionally, the release processes were studied after adding 1.7 mL of PAM ((C3H5NO)n, Sigma-Aldrich Co.) to 100 mL of release medium that containing 8 mg of bionanocomposite sample (drug–LDH).
Mathematical modeling of drug release
The release kinetics of the drug can be determined through mathematical models.
Zero-order kinetics
The zero-order model that describes the dissolution process related to surface reaction controlled kinetics can be given by EquationEquation 1(1) .Citation32,Citation33
First-order kinetics
The first-order model (EquationEquation 2(2) ) describes the absorption of some drugs, as well as their elimination.Citation35 Unlike the zero-order model, the rate of the release depends on the drug content existing in the LDH matrix.Citation36
Bhaskar model
The Bhaskar model is expressed by EquationEquation 3(3) :
Higuchi model
The Higuchi model explains that drug diffusion from semisolid or solid matrixes is a rate-determining step of the release process.Citation33
Parabolic diffusion model
This model is extensively used to elucidate whether the release process occurs through a surface/edge diffusion process. EquationEquation 5(5) describes the parabolic model.
Korsmeyer–Peppas (K-P) model
In 1984, Korsmeyer and Peppas developed an empirical mathematical equation to confirm the drug mechanism which is represented as:Citation42,Citation43
For the K-P model, an n value < 0.45 corresponds to quasi-Fickian diffusion. If the value of n is in the range 0.45–0.89, the release of the drug occurs through two processes, dissolution of LDHs and the diffusion of the drug, and the release process is called non-Fickian transport or anomalous diffusion mechanism. When n > 0.89, drug release follows dissolution of LDHs and the process is called super case transport II.Citation47,Citation48
Results and discussion
Physicochemical characterization of CD-LDH nanohybrid
XRD
The intercalation of CD into LDH was supported by the powder XRD pattern (). All characteristic crystalline peaks of pristine LDH were clearly observed in the XRD patterns. However, the nanohybrid did not show any kind of drug crystalline peaks. Generally, LDH exhibited a (003) reflection equivalent to the basal spacing of 8.8–8.0 Å, which reflects the formation of NO3− or CO3− or Cl− or SO4− intercalated LDH. However, the CD-LDH nanohybrid exhibited a (003) peak at 3.4°, which gives the basal spacing from 8.0 Å to 25.1 Å for CD-LDH, indicating the intercalation of drugs into LDH. Subtracting the LDH layer thickness (8.0 Å) from the observed basal spacing, the gallery height can be estimated to be 17.1 Å, which is equal to the lateral molecular length of the CD drug. It suggested that intercalated drug molecules are arranged in a lateral manner in the interlayer space.
FTIR
presents the FTIR analysis of the parent Zn-Al LDH-NO3 and the hybrid of CD-LDH. The pristine LDH spectrum () shows a broad absorption band at 3,402 cm−1, which can be considered as a common feature for all LDH-like materials, attributable to the −OH stretching vibrations in the Zn/Al-NO3-LDH layers and physically adsorbed and co-intercalated water.Citation49,Citation50 The cause of low-frequency vibration of (OH) group in ZnAl-LDH compared to that of OH-stretching in free water, around 3,600 cm–1, is the hydrogen bonds formed between the interlamellar water and hydroxide groups of the Zn/Al inorganic layers.Citation50,Citation51 Also, the ZnAl–NO3 sample shows a strong peak at 1,338.4 cm–1 (due to ν of NO3−),Citation52 and a weak band at 1,635.4 cm−1 (δH2O).Citation53 Regarding the low wavenumber region of the spectrum (400–800 cm−1), it is expected that bands correspond to metal–oxygen and metal–hydroxyl vibration modes in the LDH sheets.Citation54–Citation56 These bands confirm the Zn–Al LDH structure.Citation57
Figure 3 FTIR spectra of (a) pristine ZnAl-NO3 LDH and (b) nanohybrid CD-LDH.
Abbreviations: FTIR, Fourier transform infrared spectroscopy; LDH, layered double hydroxide; CD, cefadroxil.
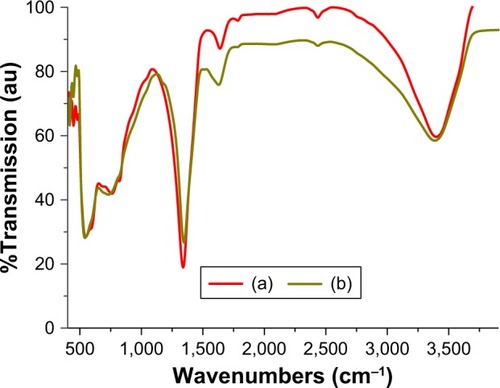
In the spectrum of the ZnAl-CD LDH nanohybrid (), the characteristic peak associated with the parent LDH-like hydroxide group, which also arises from the phenolic hydroxides, appears at 3,390.4 cm−1, which, together with the emergence of bands below 1,000 cm−1, indicates that the layers structure of LDH has not changed during the intercalation process.Citation58–Citation60 In addition, a pair of absorption bands appears at 1,631.6 and 1,350 cm−1 related to carboxylate asymmetric and symmetric stretching, respectively. Compared to −COO stretching vibration (1,650 and 1,400 cm−1) of pure CD,Citation61 the shift of the two characteristic peaks to lower frequencies was due to ionization of CD (carboxylic groups)Citation62–Citation64 and the strong electrostatic interaction of terminal oxygens of organic anions in interlayer with the electropositive cations in layer as well as the formation of bonding hydrogen between oxygen from carboxylate and the hydroxyl groups in layers or with the interlayer water molecules.Citation65–Citation68 Depending on the above results, the CD is intercalated in its anionic form and is stable in the interlayer space of LDH.Citation1,Citation69 Of particular note is that the stretching frequency peak of nitrate groups may be overlapped with −COO− symmetric-stretching peak, which may not be completely removed from the interlayers during the intercalation process.Citation70 This result is in agreement with the results of MeherCitation71 and Ghotbi and Bin Hussein.Citation58
TG analysis
By analyzing the TG curve of material CD-LDH, it is found that there are seven distinguishable stages of weight loss, which is in agreement with the differential thermogravimetric (DTG) analysis result (). In the first and second steps, from 25°C to 160°C, there are about 7.5% and 3.5% losses of the total weight, ascribed to the loss of the physically adsorbed water and water existing within the interlayer galleries, which are attached to the layer of the hydroxide and/or interlayer anion by hydrogen bonds, respectively.Citation72,Citation73 Correspondingly, two peaks at ca. 50°C and 125°C are observed in the DTG analysis.Citation72 The third mass loss (12% weight loss) occurs in the region of 160°C–250°C and corresponds to a peak in the DTG curve at 225°C, possibly due to the loss of hydroxyl group from the Zn–Al layer, the condensation of which leads to formation of water vapor.Citation62,Citation70,Citation74
Figure 4 Thermogravimetric (TG) analysis curves: (A) TG and (B) differential thermogravimetric (DTG) of nanohybrid CD-LDH.
Abbreviations: LDH, layered double hydroxide; CD, cefadroxil.
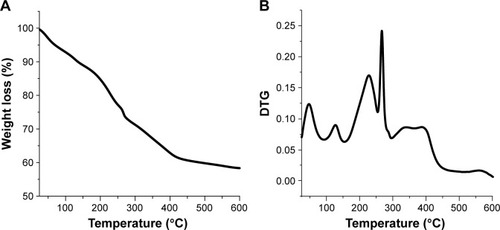
The DTG diagram shows a sharp peak at 260°C and two weak peaks at 340°C and 390°C associated with combustion of the intercalated CD. According to the TG curve, weight loss should take place in three consecutive steps (250°C–440°C), which leads eventually to the producing CO2, H2O and NOx.Citation70,Citation75
The organic CD ions in CD-LDH have a higher decomposition temperature than pure CD; the DTG curve of pure CD shows three weight losses at 191°C, 240°C and 350°C.Citation76 This indicates the improved thermal stability of CD after its intercalation into LDH gallery,Citation77,Citation78 due to the host–guest interactions, which involves electrostatic attraction within the LDH layered structure and hydrogen bonding.Citation70,Citation72 These results are similar to the results of Lonkar et alCitation79 and Wang et alCitation80 who studied the intercalation of antioxidant and glutathione into LDH, respectively.
The final stage at 560°C is due to the formation of ZnO and ZnAl2O4, where a temperature treatment above 440°C causes a collapse of the layered structure of the sample and leads to full calcification of the solid as well as complete burn of drug component, thus giving rise to oxides of the cations.Citation62,Citation81,Citation82
UV–vis spectroscopy
The UV–vis absorption spectrum of pristine CD () shows two characteristic absorption bands at 230 and 263 nm, which are ascribed to the phenolic structure (π-π* transition) and electronic transitions of the enone form (n-π* transition), respectively.Citation83–Citation85
Figure 5 UV–visible absorption of (a) pure cefadroxil and (b) nanohybrid CD-LDH.
Abbreviations: LDH, layered double hydroxide; CD, cefadroxil.
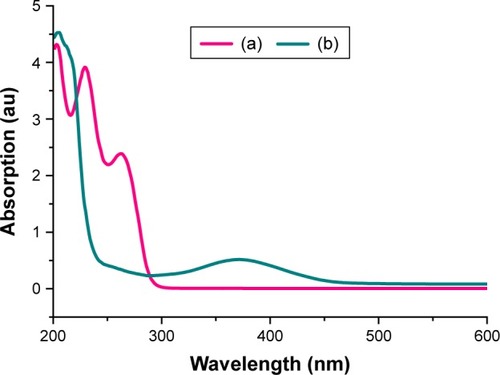
After intercalation of CD in the ZnAl-LDH host (), it is obvious that the absorption band at 230 nm cannot be identified. This may be attributed to the effect of scattering of LDH particles. Meanwhile, the band at 263 nm shows an apparent red shift with Δλ ≈ 111 nm compared to CD.Citation78,Citation86 These results proved that the drug anions are well intercalated between the layers of the LDH.Citation12 The cause of the occurrence of this shift in the spectrum is the interaction between the host and the guest as indicated by FTIR and TG. It is worth mentioning that electrostatic interaction with the host layer led to the stability of drug anions.Citation78,Citation86 Furthermore, the red shift may occur due to guest–guest interaction through π-π* conjugation of the phenol of interlayer CD ions.Citation87,Citation88 Considering the drug–LDH spectrum, the band became broader compared to that in the CD spectrum and this may be due to π-electron delocalization.Citation89
FE-SEM
The surface morphology of the ZnAl-LDH–CD nanohybrid is shown in . The micrographs were obtained with FE-SEM at 430× (), 800× (), 5,000× (), 9,500× (), 10,000× () and 14,000× () magnifications.
Figure 6 FE-SEM micrographs of nanohybrid CD-LDH. (A) 430× magnification, (B) 800× magnification, (C) 5,000× magnification, (D) 9,500× magnification, (E) 10,000× magnification and (F) 14,000× magnification.
Abbreviations: FE-SEM, field emission scanning electron microscopy; LDH, layered double hydroxide; CD, cefadroxil; CEAMR, Center of Excellence for Advanced Materials Research.
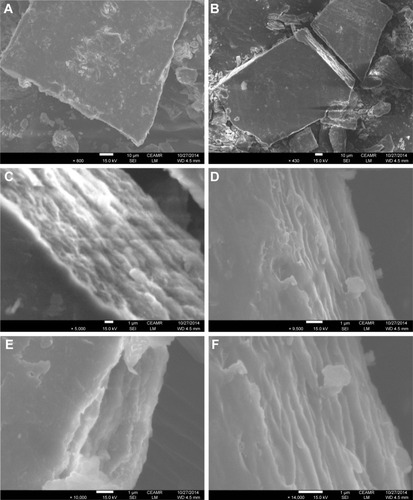
According to the literature,Citation90 FE-SEM micrographs of the synthesized NO3–LDH show uniform and regular particles with a hexagonal plate-like shape. In contrast, the photographs of the nanohybrid reveal non-uniform irregular agglomerates of compact.Citation91,Citation92 Additionally, the FE-SEM analysis of nanohybrid suggests that the CD-LDH particles have a microblock-like structure.Citation93
SEM-EDS
As illustrated in , the EDS pattern of ZnAl-CD confirms the organic and inorganic composition of the CD-LDH nanohybrid, where it shows common energy lines corresponding to C, O, Zn and Al.Citation94 The elemental composition as weight percentages and atomic percentages based on the EDS is shown in .
Table 1 EDSAnalysis of CD-LDH
In vitro drug release testing
The main part of this work is based on the compared release processes of the drug from the organic–inorganic nanohybrid, Zn-Al CD, in the different solution media, in the presence or absence of chloride anions (sodium chloride salt) or macromolecule (PAM). Since the final quantification of the antibiotic is based on a standard curve, relating the absorbance of the solution with the drug concentration, it must build the calibration curve from measurements at 230 nm in all pH medium in the expected concentration range (). It is clear that the dependence of the absorbance on the concentration is linear in the investigated range with regression coefficients larger than 0.9 ().Citation4,Citation26,Citation95 According to the literature, there are two main release mechanisms responsible for the rate of release and the amount released: anion-exchange (coupled with diffusion) of the drug molecules through the LDH material in alkaline or neutral media; and weathering/degradation of the LDH inorganic layers by protonation in strong acidic media.Citation96–Citation98 In mild acidic media, any of the two mechanisms would determine the drug release rate.Citation33
Table 2 Linearity data of CD by UV spectroscopy
CD-Zn/Al-LDH
The drug release profiles, ie, the percentage of CD released into the buffer solution vs time, have been plotted in (strong acidic), 9B (mild acidic), 9C (neutral) and 9D (slightly basic).
Figure 9 Release profiles of CD from the nanohybrid CD-LDH at pH 1.2 (A), at pH 4.0 (B), at pH 6.8 (C) and at pH 7.4 (D).
Abbreviations: PAM, polyacrylamide; CD, cefadroxil; LDH, layered double hydroxide.
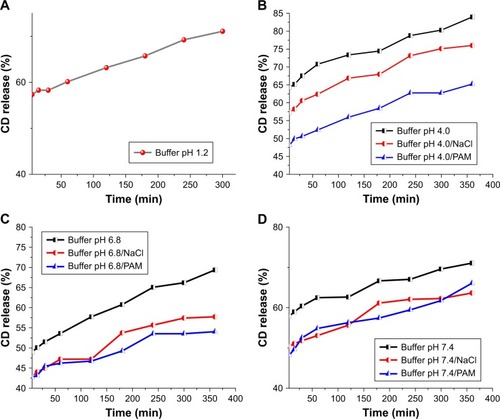
In simulated gastric fluid (), under strong acidic conditions, the LDH is dissolved rapidly and completely and the intercalated CD is fully released, in its molecular form, but not fully dissolved: 71% of CD is dissolved after the end of the experiment from drug-intercalated hydrotalcite.Citation33,Citation99,Citation100
Bin Hussein et alCitation92 pointed out that the layered structure of Zn–Al LDH quickly collapses, releasing α-naphthalene acetate (NAA) in strong acidic conditions. The release behavior for the ZnAl–CD nanohybrid does not demonstrate the possibility of the occurrence of ionic exchange between the interlayer CD and the chloride anions of the buffer solution, where the chloride ion affinity to the LDH layers is too low to cause drug replacement. As a result, there is only one mechanism that controls the release of the drug in this media: solid weathering.Citation33,Citation96 The intercalation process, as well as the rapid release of CD in the gastric medium, leads to an increase in the solubility of CD and its dissolution rate at the stomach.Citation33,Citation101 Ambrogi et alCitation102 and Del Arco et alCitation103 have reported that the presence of inorganic LDH matrix improves the solubility of hardly soluble non-steroidal anti-inflammatory drugs (NSAIDs).
In lysosomal, intestinal and blood simulated fluid, a similar behavior is observed for CD-intercalated LDH in all release media at early stages; about 64%, 49% and 58% of CD is released immediately at pH 4.0, 6.8 and 7.4, respectively.
Subsequently, however, the release of CD-LDH is slow and sustained: after 360 min there is a release amount of 84% at pH 4.0 (); ca. 69% at pH 6.8 () and ca. 71% at pH 7.4 ().Citation33,Citation63,Citation104,Citation105 These findings are consistent with previous research, as reported by Yasin and IsmailCitation27 and Zhang et alCitation106 for samples containing lawsone and methotrexate intercalated in LDHs, where they studied the release of these drugs at different pH values.
The first stage of the release process can be attributed to the release of organic drug that was weakly adsorbed on the surface of the nanocomposite and that in the external part of the lamellar structure (LDH edges). The slow and sustained release of CD in the later stage may depend on the slow anion exchange between interlayered CD and the anions in the fluid.Citation33,Citation63,Citation69 Unlike CD at the interlayer, the CD at the outside layers will be exposed directly to the medium and will be dissolved immediately.Citation99
It is worth mentioning that partial destruction of the lamellar matrix did not happen in simulated lysosomal fluid. This means that the release process is subject to ion exchange mechanism only due to the high crystallinity of the LDH matrix and the great affinity of the intercalated anions for the layers.Citation96 With regard to stability of LDH at and above pH 7, this matrix is able to maintain its lamellar structure because it is more stable at those pH values and, consequently, LDH acts like a drug release modulator and the release process was governed by ion exchange mechanism.Citation107,Citation108
From the release profiles in lysosomal, intestinal and blood simulated fluid, it is clear that the percentage of CD liberated does not reach 100%; this may be because of the characteristics of the ion-exchange reaction. This means that the interlayer anions cannot be exchanged completely.Citation59,Citation109,Citation110 Nejati and RezvaniCitation72 and Xia et alCitation111 have studied the release of olsalazine at pH 7.4 and antihypertensive drugs at pH 4.25; these authors have highlighted that the release of drugs from nanohybrids (drug–LDH) is not complete, for the same reason as above.
The two-step release process can play an active role in therapeutic treatments, where the rapid release in the first stage allows the establishment of a therapeutic dose in a short period of time, while the slow stage allows the maintenance of this dose for long periods, ie, reduces the number of doses that are applied to patients, through the sustained release of drug from the ZnAl-LDH nanohybrid.Citation32,Citation112,Citation113
CD-Zn/Al-LDH/NaCl
As well as the dependence of the CD release rate from the nanohybrid on pH, it is strongly influenced by medium anionic species and their affinity toward the interlayer of the inorganic interlamellar.
– show that the release percentages of CD from CD-LDH nanohybrid in the presence of chloride ions are less than those obtained in the absence of exchangeable anion. The release profiles indicated that about 57%, 43% and 50% CD was released immediately at pH 4.0, 6.8 and 7.4, respectively. About 76% of CD is found to be released after 360 min, in lysosomal medium, while a lower release of CD is found in intestinal medium with release of 58% after 6 h. In blood simulated medium, about 64% of the initial CD amount is released during 360 min.
According to the literature,Citation114,Citation115 the effect of various medium anionic species on the release of herbicides from the interlayer of ZnAl-LDH (ZAN) follows the following order: i) for single system release media, carbonate anion > phosphate anion > chloride anion; ii) for binary system release media, carbonate and phosphate anions > chloride and phosphate anions > chloride and carbonate anions; iii) for all systems release media, carbonate anion > phosphate anion > carbonate and phosphate anions > chloride and carbonate and phosphate anions > chloride and phosphate anions > chloride and carbonate anions > chloride anion.
The reason for the increase of the CD release percentage in single system release media (phosphate ion) compared to the binary anions system of chloride–phosphate is ascribed to the decomposition of potassium phosphate, which leads to the formation of HPO42− and H2PO4−, which strongly compete with other anions in the ion exchange process. Phosphate anions are able to exchange intercalated drugs easily due to their high affinity toward the LDH, thus the release rate of the drug is dominated by phosphate ions.Citation107,Citation114 By contrast, when chloride ions are present in the fluid, the rate of the release will be slower, and the amount of drug released will be lower due to the low ion exchange affinity of chloride anion toward the inorganic interlamellar ZnAl-LDH.Citation116,Citation117 This result is in good agreement with previous reports of Berber et alCitation118 and Nakayama et al.Citation119 This suggests that it is the affinity of the sacrificial anion for LDH which governs the ion exchange process.Citation64
CD-Zn/Al-LDH/PAM
The release studies of CD in the presence of PAM (–) show similar release behaviors to those in the presence of chloride anions. It is found that the amount of drug liberated is decreased in the presence of PAM.
The immediate release of CD is 48%, 43% and 48% at pH 4.0, 6.8 and 7.4, respectively. At pH 4.0, CD release from CD-LDH reaches a maximum of about 65% within 360 min. At pH 6.8, the release percentage of CD from the nanohybrid reaches 54% after 360 min. About 66% amount of CD is released from the LDH at pH 7.4 after 360 min.
It is known that PAM is not ionized (it is an uncharged polymer), but when it is added to the buffer solutions, it will hydrolyze partially, ie, a partially hydrolyzed polyacrylamide (HPAM) will be formed. Hydrolysis of PAM in the presence of H+ as catalyst at pH 4.0 yields a copolymer composed of acrylamide and acrylic acid (), while its hydrolysis in the presence of OH− as catalyst at pH 7.4 yields a copolymer composed of acrylamide and sodium acrylate ().Citation120,Citation121 In the case of an intestinal medium, the existence of hydroxide ions in this medium would provide considerable assistance to the hydrolysis.Citation122 Since the acrylic acid or sodium acrylate units contain carboxyl groups, they shift such co-polymers to moderately anionic copolymers.
Figure 10 General structure for partially hydrolyzed polyacrylamide resulting from the presence of H+ (A) and OH− (B) catalysts in the release media.Citation123,Citation125
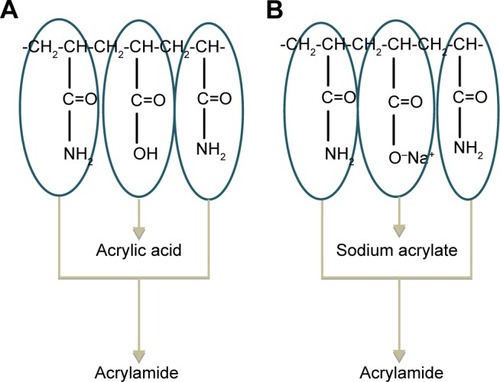
When HPAM COOH or −COONa groups dissociate into −COO−, the polymer exchanges the CD anions existing on the LDH external sheets and then the carboxylate groups (−COO−) of the polymer can interact with the positive surface charge, which leads to inhibition of release of the drug from the nanohybrid. On the other hand, the formation of the negative layer surrounding the LDH particle inhibits the diffusion of CD anions that have the same charge of the diffusion layer.Citation122,Citation124
Release kinetics of drug from CD-LDH nanohybrid
The release can be described as the rate of mass transport from a solid face into the buffer medium at standard conditions.Citation126 For drug delivery systems, three steps can be distinguished for drug release from the matrix: the initial step is liquid penetration to the matrix, followed by interaction between the nanohybrid and the buffer solution at the solid–liquid interface, and then diffusion of the drug into the exterior liquid ().Citation127
Figure 11 The fundamental steps in the release mechanism of the different drugs. (1) The molecules found in the buffer solution move toward the solid–liquid interface; (2) reaction happening at the interface; (3) the dissolved drug molecules are moving in the direction of the bulk solution.Citation126
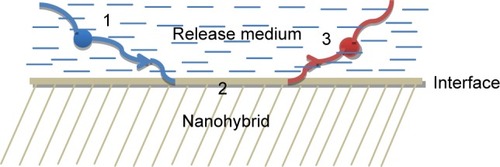
In the present work, we used the dissolution-diffusion kinetic models (zero order, first order, Bhaskar, Higuchi, parabolic diffusion and Korsmeyer–Peppas models), in order to describe the overall release process from the drug delivery system, to facilitate quantitative analysis of the data obtained in release study.Citation128
Through the values of linear correlation coefficients (r2), which are shown in , it is clear that the release mechanisms of the CD from the nanohybrid are the same in all release profiles (II = buffer pH 4.0, III = buffer pH 4.0/NaCl, IV = buffer pH4.0/PAM, V = buffer pH 6.8, VI = buffer pH 6.8/NaCl, VII = buffer pH 6.8/PAM, VIII = buffer pH 7.4, IX = buffer pH 7.4/NaCl and X = buffer pH 7.4/PAM), with the exception of profile I = buffer pH 1.2.
Table 3 Correlation coefficients (r2) and values of the diffusion exponent (n) obtained from CD release data fitting analyses based on several kinetic equations
In general, if we look at the values of coefficients related (r2) obtained from release data fitting analyses based on first-order model ( and ); Bhaskar model ( and ); Higuchi model ( and ); parabolic model ( and ); and Korsmeyer–Peppas ( and ), it can clearly be seen that CD release at pH 1.2 does not depend on (1) the drug concentration in the matrix, (2) the ion-exchange mechanism, (3) the diffusion through the particle, and (4) an external surface/edge diffusion process.Citation33
Figure 12 Fitting the nanohybrid CD-LDH release data at pH 1.2 to different kinetic equations. (A) zero-order model (description of the dissolution process); (B) first-order model (description of the drug absorption); (C) Bhaskar model (description of drug diffusion from insoluble inorganic matrixes); (D) Higuchi model (description of drug diffusion from semi-solid); (E) parabolic model (description of drug diffusion from an external surface); and (F) Korsmeyer–Peppas model (confirmation of the drug release mechanism). It is clear that the zero-order model can explain the release behavior kinetic of CD-LDH nanohybrid at pH 1.2 by providing higher linear correlation coefficient, which means that the release process is based on the dissolution of the drug.
Abbreviations: CD, cefadroxil; LDH, layered double hydroxide; Mt, the drug quantity released at release time; M0, the initial quantity of drug in the nanohybrid; M∞, the drug quantity released in infinite time; t, release time expressed in minutes.
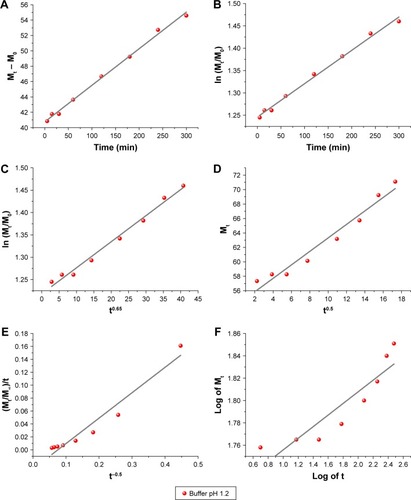
At pH 4.0, 6.8 and 7.4, since the release of the drug occurs by ion exchange with anions existing in the release medium, the following mechanism may be proposed for release of CD from CD-LDH:
Reaction in the external parts of the particle: ion exchange reaction happens between the drug anions (large ions) existing in the external parts of the LDH and the anions (small species) of the release media, which leads to the formation of an external phase with smaller interlayer distance (boundary phase) between the external zone in which phosphates have already replaced the drug anions (small spacing) and the internal zone containing intercalated drug (large spacing). As a result, the ion exchange process will become slow.Citation118,Citation129
Diffusion through the LDH particle: drug anions diffuse from the LDH interlayer region ().
Diffusion of the drug anions from the bulk solution surrounding the LDH particle ().Citation15,Citation107
Figure 13 Representation of drug release in buffer solution.Citation112
Abbreviation: LDH, layered double hydroxide.
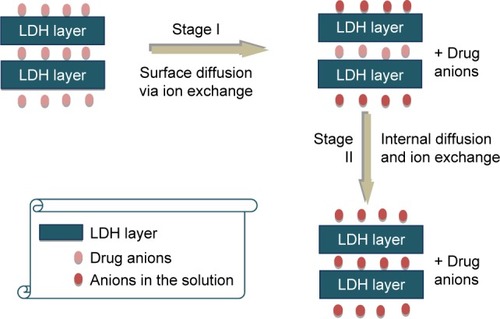
Figure 14 Schematic representation of the diffusion of the drug from the bulk solution surrounding the particle.Citation113
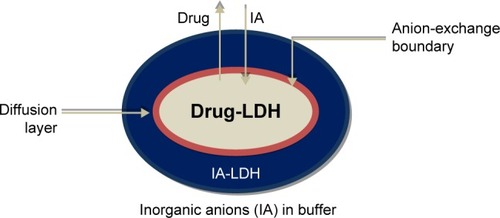
The slowest of these processes will determine the rate of exchange of drug anions with the solution medium.Citation107
As an ion-exchange mechanism is involved when using LDH, Bhaskar et alCitation38 created an equation to determine whether the diffusion through the particle is the rate limiting step of the release process. This equation suggests that simply testing for linearity between ln(Mt/M0) and t0.65 is a possible test of the particle diffusion control.Citation38 When the Bhaskar equation was applied to the measured drug release data, the relationship shown above is perfectly linear (, and ), which means that the rate limiting step of the release process is the diffusion out of particles.Citation65,Citation124
Figure 15 Fitting the nanohybrid CD-LDH release data at pH 4.0 to different kinetic equations. (A) Bhaskar model (description of drug diffusion from insoluble inorganic matrixes); from the Bhaskar model, it is clear that the ion-exchange mechanism is the most responsible for drug liberation; (B) Higuchi model (description of drug diffusion from semi-solid); the Higuchi model confirmed the importance of the diffusion through the particle in controlling the drug release rate; (C) Korsmeyer–Peppas model (confirmation of the drug release mechanism); from the Korsmeyer–Peppas equation, it turned out that the process is called quasi Fickian diffusion; (D) parabolic model (description of drug diffusion from an external surface); R2 values obtained by parabolic diffusion equation confirmed that CD release did not depend on an external surface/edge diffusion process; (E) first-order model (description of the drug absorption); R2 values obtained by first order kinetics confirmed that CD release did not depend on the drug concentration in the matrix; and (F) zero-order model (description of the dissolution process); R2 values obtained by zero order kinetics confirmed that CD release did not depend on the dissolution process.
Abbreviations: CD, cefadroxil; LDH, layered double hydroxide; PAM, polyacrylamide; Mt, the drug quantity released at release time; M0, the initial quantity of drug in the nanohybrid; M∞, the drug quantity released in infinite time; t, release time expressed in minutes.
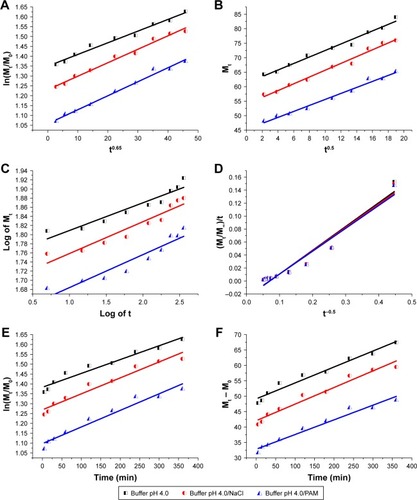
Figure 16 Fitting the nanohybrid CD-LDH release data at pH 6.8 to different kinetic equations. (A) Bhaskar model (description of drug diffusion from insoluble inorganic matrixes); from the Bhaskar model, it is clear that the ion-exchange mechanism is the most responsible for drug liberation; (B) Higuchi model (description of drug diffusion from semi-solid); the Higuchi model confirmed the importance of the diffusion through the particle in controlling the drug release rate; (C) Korsmeyer–Peppas model (confirmation of the drug release mechanism); from the Korsmeyer–Peppas equation, it turned out that the process is called quasi Fickian diffusion; (D) parabolic model (description of drug diffusion from an external surface); R2 values obtained by parabolic diffusion equation confirmed that CD release did not depend on an external surface/edge diffusion process; (E) first-order model (description of the drug absorption); R2 values obtained by first order kinetics confirmed that CD release did not depend on the drug concentration in the matrix; and (F) zero-order model (description of the dissolution process); R2 values obtained by zero order kinetics confirmed that CD release did not depend on the dissolution process.
Abbreviations: CD, cefadroxil; LDH, layered double hydroxide; PAM, polyacrylamide; Mt, the drug quantity released at release time; M0, the initial quantity of drug in the nanohybrid; M∞, the drug quantity released in infinite time; t, release time expressed in minutes.
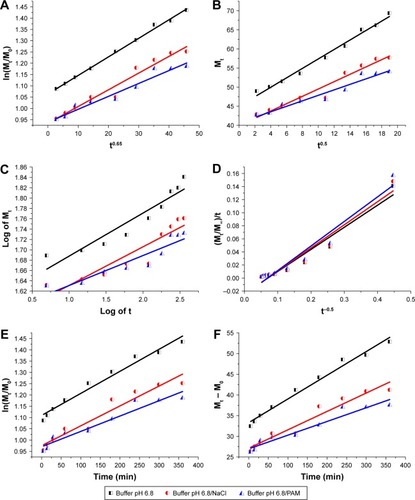
Figure 17 Fitting the nanohybrid CD-LDH release data at pH 7.4 to different kinetic equations. (A) Bhaskar model (description of drug diffusion from insoluble inorganic matrixes); from the Bhaskar model, it is clear that the ion-exchange mechanism is the most responsible for drug liberation; (B) Higuchi model (description of drug diffusion from semi-solid); the Higuchi model confirmed the importance of the diffusion through the particle in controlling the drug release rate; (C) Korsmeyer–Peppas model (confirmation of the drug release mechanism); from the Korsmeyer–Peppas equation, it turned out that the process is called quasi Fickian diffusion; (D) parabolic model (description of drug diffusion from an external surface); R2 values obtained by parabolic diffusion equation confirmed that CD release did not depend on an external surface/edge diffusion process; (E) first-order model (description of the drug absorption); R2 values obtained by first order kinetics confirmed that CD release did not depend on the drug concentration in the matrix; and (F) zero-order model (description of the dissolution process); R2 values obtained by zero order kinetics confirmed that CD release did not depend on the dissolution process.
Abbreviations: CD, cefadroxil; LDH, layered double hydroxide; PAM, polyacrylamide; Mt, the drug quantity released at release time; M0, the initial quantity of drug in the nanohybrid; M∞, the drug quantity released in infinite time; t, release time expressed in minutes.
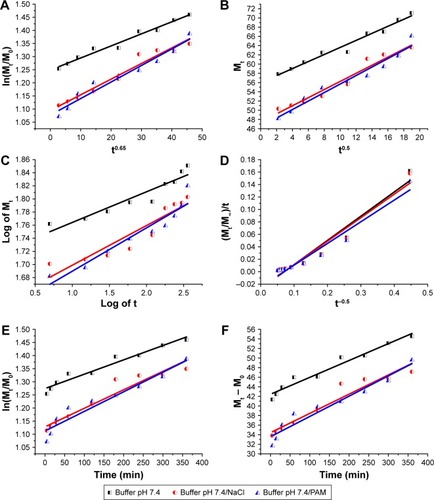
Since the Bhaskar model provides excellent r2 values equal to or more than 0.95 for all media (from II to X; ), it is possible to elucidate the release processes of CD-LDH nanohybrid on the basis of this model. In other words, through this equation, it is clear that the mechanism of the ion exchange is considered fundamental to determine the overall release rateCitation38,Citation130 and the diffusion of the drug from LDH is subject to strict control by the matrix.Citation8
Another kinetic analysis using an equation suitable for diffusion from the matrix is the Higuchi model. This model fits the release data very well (, and ), confirming the significance of the process of the diffusion in controlling the rate of the release of the drug.Citation124
The Korsmeyer–Peppas kinetics mathematical model equation was applied to confirm the diffusion mechanism.Citation131 The correlation coefficients (r2) obtained from the fittings were almost acceptable (, and ). Overall, the calculated values of diffusional exponent n are lower than 0.45 (), which means that the drug release rate is controlled by diffusion from the interlayer of the particle via ion exchange; this process is called quasi-Fickian diffusion mechanism.Citation43,Citation44
By comparing correlation coefficients values obtained by the Bhaskar kinetics model with those obtained by parabolic diffusion equation (, and ), it is evident that diffusion of the CD anions from the surface of LDH particles into the medium solution via anion exchange, which takes a short time, cannot be a rate determining step of the release process.Citation63,Citation106
The experimental data show a poor fit with the first order kinetics compared to the Bhaskar kinetic model (, and ). This means that the release process cannot be based on the amount of the drug in LDH and drug diffusion from LDH is not passive.Citation8,Citation106
The zero-order model fits the experimental data worse than the Bhaskar model (, and ), indicating that the release of the drug is not only considered a single dissolution process.Citation132
Conclusion
CD was successfully intercalated into a Zn-Al nitrate LDH by a co-precipitation technique. The intercalation process was confirmed by FTIR for the nanohybrid, which indicated the presence of functional groups for both the drug and the Zn-Al LDH, confirming the success of the intercalation process. Additionally, TG-DTG analysis proved that thermal stability of CD after intercalation was enhanced compared with its free counterpart, due to interactions between host and guest. Characterization by using UV–vis spectroscopy proved that the interlayer antibiotic anions were stabilized by host–guest and guest–guest interactions. FE-SEM analysis indicated that the CD-LDH nanohybrid has a microblock-like structure. EDS results revealed the nanohybrid contained both organic and inorganic constituents. Release tests of the CD from its interlamellae of the organic–inorganic nanohybrid in a strong acid fluid (pH 1.2) indicated that the LDH was dissolved and intercalated antibiotic released from the sample in a molecular form, while at pH 4.0, 6.8 and 7.4 in the presence or absence of Cl ions or PAM there was immediate release of CD anions that occurred at the beginning of release tests, followed by a slower and sustained release process. So, the ZnAl-CD nanohybrid can be considered as a sustained drug release system allowing increase of the therapeutic efficacy of the antibiotic by maintaining a dose over a long period of time, thus, this system reduces side effects. In general, the release rate of the anion was found to be dependent on the pH of the release medium. The comparison of the release profiles revealed that the percentage of drug released in the absence of the NaCl or PAM in the release media was more than that observed with their presence. The release mechanism had been interpreted on the basis of solid weathering at pH 1.2, while anion-exchange (coupled with diffusion) determined drug release at pH 4.0, 6.8 and 7.4. The release of CD into the gastric simulated fluid can be better expressed by the zero-order model, which means that the release rate of the CD depended on its dissolution. In lysosomal, intestinal, and blood simulated fluid, the Bhaskar model is the best to describe the release behavior kinetic of CD from the CD-LDH nanohybrid. This means that intraparticle diffusion could play a major role in the control of the drug release rate.
Acknowledgments
This project was funded by the Center of Excellence for Advanced Materials Research (CEAMR), King Abdulaziz University, Jeddah, under grant no. (CEAMR-SG-1-438).
Disclosure
The authors report no conflicts of interest in this work.
References
- TammaroLCostantinoUNocchettiMVittoriaVIncorporation of active nano-hybrids into poly (ε-caprolactone) for local controlled release: antifibrinolytic drugAppl Clay Sci200943350356
- ParveenSMisraRSahooSKNanoparticles: a boon to drug delivery, therapeutics, diagnostics and imagingNanomedicine2012814716621703993
- MishraBPatelBBTiwariSColloidal nanocarriers: a review on formulation technology, types and applications toward targeted drug deliveryNanomedicine2010692419447208
- TrikeriotisMGhanotakisDFIntercalation of hydrophilic and hydrophobic antibiotics in layered double hydroxidesInt J Pharm200733217618417070662
- DashSMurthyPNNathLChowdhuryPKinetic modeling on drug release from controlled drug delivery systemsActa Pol Pharm20106721722320524422
- UkmarTMaverUPlaninšekOKaučičVGaberščekMGodecAUnderstanding controlled drug release from mesoporous silicates: theory and experimentJ Control Release201115540941721763374
- ParkDHHwangSJOhJMYangJHChoyJHPolymer–inorganic supramolecular nanohybrids for red, white, green, and blue applicationsProg Polym Sci20133814421486
- PerioliLPosatiTNocchettiMBellezzaFCostantinoUCipicianiAIntercalation and release of antiinflammatory drug diclofenac into nanosized ZnAl hydrotalcite-like compoundAppl Clay Sci201153374378
- SepehrikiaSArefiDDetermination of Pb and Ni in natural water samples after solid phase extraction with layered double hydroxide (LDH) nano-particlesAm J Nanosci Nanotech201318386
- ChiangMFChenECWuTMPreparation, mechanical properties and thermal stability of poly (l-lactide)/γ-polyglutamate-modified layered double hydroxide nanocompositesPolym Degrad Stab2012979951001
- OhJMParkDHChoiSJChoyJHLDH nanocontainers as bio-reservoirs and drug delivery carriersRecent Pat Nanotechnol2012620021722747720
- PanDZhangHZhangTDuanXA novel organic–inorganic microhybrids containing anticancer agent doxifluridine and layered double hydroxides: structure and controlled release propertiesChem Eng Sci20106537623771
- RibeiroLNAlcantaraACDarderMArandaPAraujo-MoreiraFMRuiz-HitzkyEPectin-coated chitosan–LDH bionanocomposite beads as potential systems for colon-targeted drug deliveryInt J Pharm20144631924374607
- RivesVDel ArcoMMartínCIntercalation of drugs in layered double hydroxides and their controlled release: a reviewAppl Clay Sci201488239269
- WeiMPuMGuoJIntercalation of l-Dopa into layered double hydroxides: enhancement of both chemical and stereochemical stabilities of a drug through host-guest interactionsChem Mater20082051695180
- Zümreoglu-KaranBAyANLayered double hydroxides – multifunctional nanomaterialsChem Pap201266110
- OhJMBiswickTTChoyJHLayered nanomaterials for green materialsJ Mater Chem20091925532563
- KimMHParkDHYangJHChoyYBChoyJHDrug-inorganic-polymer nanohybrid for transdermal deliveryInt J Pharm201344412012723357253
- ShafieiSBirganiZTDarvishAAzimiMSSolati-HashjinMLayered double hydroxides for diagnostic applicationsInternational Congress of Evaluation of Medical Diagnosis Modern Technologies2008116 Available from: http://www.academia.edu/19536705/Layered_Double_Hydroxides_for_Diagnostic_ApplicationsAccessed April 10, 2018
- BasuDDasAStöckelhuberKWWagenknechtUHeinrichGAdvances in layered double hydroxide (LDH)-based elastomer compositesProg Polym Sci201439594626
- ChakrabortiMJacksonJKPlackettDBrunetteDMBurtHMDrug intercalation in layered double hydroxide clay: application in the development of a nanocomposite film for guided tissue regenerationInt J Pharm201141630531321708236
- ChakrabortyMDasguptaSSoundrapandianCMethotrexate intercalated ZnAl-layered double hydroxideJ Solid State Chem201118424392445
- BlazheyevskiMYLabuzovaYYKinetic spectrophotometric determination of cefadroxil in pure substance and pharmaceutical dosage formJ Chem Pharm Res20135115121
- FrunzaMLisaGPopaMIMironNDNistorDIThermogravimetric analysis of layered double hydroxides with chloramphenicol and salicylate in the interlayer spaceJ Thermal Anal Calor200893373379
- RyuSJJungHOhJMLeeJKChoyJHLayered double hydroxide as novel antibacterial drug delivery systemJ Phys Chem Solid201071685688
- San RománMSHolgadoMJSalinasBRivesVDrug release from layered double hydroxides and from their polylactic acid (PLA) nano-compositesAppl Clay Sci20137117
- YasinYIsmailNMControlled release of lawsone-intercalated Zn-Al-layered double hydroxideJ Med Sci201313453458
- BarahuieFHusseinMZArulselvanPFakuraziSZainalZDrug delivery system for an anticancer agent, chlorogenate-Zn/Al-layered double hydroxide nanohybrid synthesised using direct co-precipitation and ion exchange methodsJ Solid State Chem20142173141
- ChaudhariSVVaviaPRGastroretentive sustained release floating and swellable cefadroxil formulationJ Bioequiv Bioavail20168294298
- JatavRKBodanaMFormulation and evaluation of floating tablet of cefadroxilCurr Res Pharm Sci2015595105
- CavaniFTrifiroFVaccariAHydrotalcite-type anionic clays: preparation, properties and applicationsCatal Today199111173301
- GuZThomasACXuZPCampbellJHLuGQIn vitro sustained release of LMWH from MgAl-layered double hydroxide nanohybridsChem Mater20082037153722
- RojasRPalenaMCJimenez-KairuzAFManzoRHGiacomelliCEModeling drug release from a layered double hydroxide–ibuprofen complexAppl Clay Sci2012621520
- XuGSunadaHInfluence of formulation change on drug release kinetics from hydroxypropylmethylcellulose matrix tabletsChem Pharm Bull (Tokyo)1995434834877774032
- CostaPSousa LoboJMModeling and comparison of dissolution profilesEur J Pharm Sci20011312313311297896
- KalamMAHumayunMParvezNRelease kinetics of modified pharmaceutical dosage forms: a reviewCont J Pharm Sci200713035
- ShoaibMHTazeenJMerchantHAYousufRIEvaluation of drug release kinetics from ibuprofen matrix tablets using HPMCPak J Pharm Sci20061911912416751122
- BhaskarRMurthyRSRMiglaniBDViswanathanKNovel method to evaluate diffusion controlled release of drug from resinateInt J Pharm1986285966
- HiguchiTMechanism of sustained-action medication. Theoretical analysis of rate of release of solid drugs dispersed in solid matricesJ Pharm Sci1963521145114914088963
- KosmidisKRinakiEArgyrakisPMacherasPAnalysis of Case II drug transport with radial and axial release from cylindersInt J Pharm200325418318812623194
- SerraLDoménechJPeppasNADrug transport mechanisms and release kinetics from molecularly designed poly (acrylic acid-g-ethylene glycol) hydrogelsBiomaterials2006275440545116828864
- KorsmeyerRWPeppasNASolute and penetrant diffusion in swellable polymers. III. Drug release from glassy poly (HEMA-co-NVP) copolymersJ Control Release198418998
- SahooSChakrabortiCKBeheraPKDevelopment and evaluation of gastroretentive controlled release polymeric suspensions containing ciprofloxacin and carbopol polymersJ Chem Pharm Res2012422682284
- KorsmeyerRWGurnyRDoelkerEBuriPPeppasNAMechanisms of solute release from porous hydrophilic polymersInt J Pharm1983152535
- PeppasNAAnalysis of Fickian and non-Fickian drug release from polymersPharm Acta Helv1985601101114011621
- RaniGUKonreddyAKMishraSSenGSynthesis and applications of polyacrylamide grafted agar as a matrix for controlled drug release of 5-ASAInt J Biol Macromol20146537538224508089
- YadavGBansalMThakurNKhareSKharePMultilayer tablets and their drug release kinetic models for oral controlled drug delivery systemME J Sci Res201316782795
- SiepmannJPeppasNAModeling of drug release from delivery systems based on hydroxypropyl methylcellulose (HPMC)Adv Drug Deliv Rev20014813915711369079
- WangZLiuFLuCMg–Al–carbonate layered double hydroxides as a novel catalyst of luminol chemiluminescenceChem Commun20114754795481
- NakamotoKInfrared and Raman Spectra of Inorganic and Coordination CompoundsNew YorkJohn Wiley & Sons1997
- Bin HusseinMZLongCWSynthesis of organo-mineral nanohybrid material: indole-2-carboxylate in the lamella of Zn–Al-layered double hydroxideMater Chem Phys200485427431
- KuraAUAl AliSHHHusseinMZFakuraziSArulselvanPDevelopment of a controlled-release anti-parkinsonian nanodelivery system using levodopa as the active agentInt J Nanomedicine20138110323524513
- LambertJBShurvellHFLightnerDACooksRGIntroduction to Organic SpectroscopyNew YorkMacmillan Publishing Company1987
- ChakrabortyMDasguptaSBosePLayered double hydroxide: inorganic organic conjugate nanocarrier for methotrexateJ Phys Chem Solid201172779783
- WeiMGuoJShiZPreparation and characterization of l-cystine and l-cysteine intercalated layered double hydroxidesJ Mater Sci20074226842689
- XuZPGuZChengXRasoulFWhittakerAKLuGQMControlled release of ketorolac through nanocomposite films of hydrogel and LDH nanoparticlesJ Nanopart Res20111312531264
- DeLeonVHNguyenTDNarMD’SouzaNAGoldenTDPolymer nanocomposites for improved drug delivery efficiencyMater Chem Phys2012132409415
- GhotbiMYBin HusseinMZGallate–Zn–Al-layered double hydroxide as an intercalated compound with new controlled release formulation of anticarcinogenic agentJ Phys Chem Solid20107115651570
- RivesVLayered Double Hydroxides: Present and FutureNew YorkNova Publishers2001
- KloproggeJTFrostRLFourier transform infrared and raman spectroscopic study of the local structure of Mg-, Ni-, and Co-hydrotalcitesJ Solid State Chem1999146506515
- RahimNNaqviSBBibiRIffatWShakeelSMuhammadINDisintegrants combination: development and optimization of a cefadroxil fast disintegrating tabletPak J Pharm Sci2014271467147525176230
- San RománMSHolgadoMJSalinasBRivesVCharacterisation of diclofenac, ketoprofen or chloramphenicol succinate encapsulated in layered double hydroxides with the hydrotalcite-type structureAppl Clay Sci201255158163
- YangJHHanYSParkMParkTHwangSJChoyJHNew inorganic-based drug delivery system of indole-3-acetic acid-layered metal hydroxide nanohybrids with controlled release rateChem Mater20071926792685
- BashiAMHaddawiSMAl-YasariAHKinetics study and controlled release of synthesized phenoxy herbicides-based nanocomposites from 2,4-dichloro- and 2,4,5-trichlorophenoxy acetates with Zn/Al layered double hydroxide interlamellaeIrq Nat J Chem201038279292
- ZhangHZouKGuoSDuanXNanostructural drug-inorganic clay composites: structure, thermal property and in vitro release of captopril-intercalated Mg–Al-layered double hydroxidesJ Solid State Chem200617917921801
- SantosaSJKunartiESSynthesis and utilization of Mg/Al hydrotalcite for removing dissolved humic acidAppl Surf Sci200825476127617
- KamedaTFubasamiYUchiyamaNYoshiokaTElimination behavior of nitrogen oxides from a NO3−-intercalated Mg–Al layered double hydroxide during thermal decompositionThermochim Acta2010499106110
- Morzyk-OciepaBMichalskaDPietraszkoAStructures and vibrational spectra of indole carboxylic acids. Part I. Indole-2-carboxylic acidJ Mol Struct20046887986
- ParkMCKimHParkDHYangJHChoyJHKetoprofen-LDH nanohybrid for transdermal drug delivery systemBull Kor Chem Soc20123318271828
- Al AliSHHAl-QubaisiMHusseinMZIsmailMZainalZHakimMNControlled release and angiotensin-converting enzyme inhibition properties of an antihypertensive drug based on a perindopril erbumine-layered double hydroxide nanocompositeInt J Nanomedicine201272129214122619549
- MeherRRDouble Hydroxide Nano-carrier for Controlled Delivery of Drug Molecules [PhD thesis]RourkelaNational Institute of Technology2012
- NejatiKRezvaniZSynthesis and characterisation of nanohybrids of olsalazine-intercalated Mg-Al layered double hydroxideJ Exp Nanosci20127412425
- ArizagaGGCMangrichASda Costa GardolinskiJEFWypychFChemical modification of zinc hydroxide nitrate and Zn–Al-layered double hydroxide with dicarboxylic acidsJ Colloid Interface Sci200832016817618243238
- ZouKZhangHDuanXStudies on the formation of 5-aminosalicylate intercalated Zn–Al layered double hydroxides as a function of Zn/Al molar ratios and synthesis routesChem Eng Sci20076220222031
- Del ArcoMFernándezAMartínCRivesVIntercalation of mefenamic and meclofenamic acid anions in hydrotalcite-like matrixesAppl Clay Sci200736133140
- FuliasAVlaseTVlaseGThermoanalytical study of cefadroxil and its mixtures with different excipientsRev Chim2010411
- AyANZümreoglu-KaranBTemelARivesVBioinorganic magnetic core-shell nanocomposites carrying antiarthritic agents: intercalation of ibuprofen and glucuronic acid into Mg−Al-layered double hydroxides supported on magnesium ferriteInorg Chem2009488871887719691269
- ChoyJHJungJSOhJMLayered double hydroxide as an efficient drug reservoir for folate derivativesBiomaterials2004253059306414967539
- LonkarSPKutluBLeuteritzAHeinrichGNanohybrids of phenolic antioxidant intercalated into MgAl-layered double hydroxide clayAppl Clay Sci201371814
- WangYWuPLiYZhuNDangZStructural and spectroscopic study of tripeptide/layered double hydroxide hybridsJ Colloid Interface Sci201339456457223266024
- HibinoTTsunashimaAFormation of spinel from a hydrotalcite-like compound at low temperature: reaction between edges of crystallitesClay Clay Mineral199745842853
- KanezakiEThermal behavior of the hydrotalcite-like layered structure of Mg and Al-layered double hydroxides with interlayer carbonate by means of in situ powder HTXRD and DTA/TGSolid State Ionics1998106279284
- RubinoFMSeparation methods for methotrexate, its structural analogues and metabolitesJ Chromatogr B Biomed Sci Appl200176421725411817030
- DonaldLLampmanGMKrizGSIntroduction to Spectroscopy: A Guide for Students of Organic ChemistryFort WorthHarcourt Brace College Publishers1996
- BarretoWJPonzoniSSassiPA raman and UV–vis study of catecholamines oxidized with Mn (III)Spectrochim Acta A Mol Biomol Spectrosc1998556572
- MengJZhangHEvansDGDuanXStudy on the supramolecular structure of sorbic acid intercalated Zn-Al layered double hydroxides and its thermal decompositionChinese Science Bulletin20055025752581
- HeQYinSSatoTSynthesis and photochemical properties of zinc–aluminum layered double hydroxide/organic UV ray absorbing molecule/silica nanocompositesJ Phys Chem Solid200465395402
- AisawaSTakahashiSOgasawaraWUmetsuYNaritaEDirect intercalation of amino acids into layered double hydroxides by coprecipitationJ Solid State Chem20011625262
- FranklinKRLeeENunnCCPreparation and characterisation of layered double hydroxides containing monovalent and divalent ions derived from 5-benzoyl-4-hydroxy-2-methoxybenzenesulfonic acidJ Mater Chem19955565569
- MinagawaKBerberMRHafezIHMoriTTanakaMTarget delivery and controlled release of the chemopreventive drug sulindac by using an advanced layered double hydroxide nanomatrix formulation systemJ Mater Sci Mater Med20122397398122350776
- HusseinMZJubriZBZainalZYahyaAHPamoate intercalated Zn-Al layered double hydroxide for the formation of layered organic-inorganic intercalateMater Sci Poland2004225767
- Bin HusseinMZZainalZYahayaAHFooDWVControlled release of a plant growth regulator, α-naphthaleneacetate from the lamella of Zn–Al-layered double hydroxide nanocompositeJ Control Release20028241742712175754
- BelskayaOBGulyaevaTILeont’evaNNFormation of platinum sites on layered double hydroxide type basic supports: II. Effect of the nature of the interlayer anion of the layered aluminum-magnesium hydroxides on platinum binding and Pt/MgAlOx formationKinetics Catal201152876885
- CarjaGLehutuGDartuLMertensMCoolPLayered double hydroxides reconstructed in calcium glutamate aqueous solution as a complex delivery systemAppl Clay Sci2012653742
- TammaroLCostantinoUBologneseANanohybrids for controlled antibiotic release in topical applicationsInt J Antimicrob Agents20072941742317303393
- TrontoJdos ReisMJSilvérioFBalboVRMarchettiJMValimJBIn vitro release of citrate anions intercalated in magnesium aluminium layered double hydroxidesJ Phys Chem Solid200465475480
- AmbrogiVFardellaGGrandoliniGPerioliLIntercalation compounds of hydrotalcite-like anionic clays with antiinflammatory agents – I. Intercalation and in vitro release of ibuprofenInt J Pharm2001220233211376964
- ParelloMLRojasRGiacomelliCEDissolution kinetics and mechanism of Mg–Al layered double hydroxides: a simple approach to describe drug release in acid mediaJ Colloid Interface Sci201035113413920709325
- HaJUXanthosMDrug release characteristics from nanoclay hybrids and their dispersions in organic polymersInt J Pharm201141432133121601624
- AmbrogiVPerioliLCiarnelliVNocchettiMRossiCEffect of gliclazide immobilization into layered double hydroxide on drug releaseEur J Pharm Biopharm20097328529119539757
- BerberMRMinagawaKKatohMMoriTTanakaMNano-composites of 2-arylpropionic acid drugs based on Mg–Al layered double hydroxide for dissolution enhancementEur J Pharm Sci20083535436018789388
- AmbrogiVFardellaGGrandoliniGNocchettiMPerioliLEffect of hydrotalcite-like compounds on the aqueous solubility of some poorly water-soluble drugsJ Pharm Sci2003921407141812820145
- Del ArcoMFernandezAMartinCSayaleroMLRivesVSolubility and release of fenamates intercalated in layered double hydroxidesClay Minerals200843255265
- WangZWangEGaoLXuLSynthesis and properties of Mg2Al layered double hydroxides containing 5-fluorouracilJ Solid State Chem2005178736741
- HuangXBrazelCSOn the importance and mechanisms of burst release in matrix-controlled drug delivery systemsJ Control Release20017312113611516493
- ZhangXQZengMGLiSPLiXDMethotrexate intercalated layered double hydroxides with different particle sizes: structural study and controlled release propertiesColloids Surf B Biointerfaces20141179810624632036
- AmbrogiVFardellaGGrandoliniGPerioliLTiraltiMCIntercalation compounds of hydrotalcite-like anionic clays with anti-inflammatory agents, II: uptake of diclofenac for a controlled release formulationAAPS PharmSciTech200237782
- PerioliLAmbrogiVDi NautaLNocchettiMRossiCEffects of hydrotalcite-like nanostructured compounds on biopharmaceutical properties and release of BCS class II drugs: the case of flurbiprofenAppl Clay Sci201151407413
- FoggAMDunnJSShyuSGCaryDRO’HareDSelective ion-exchange intercalation of isomeric dicarboxylate anions into the layered double hydroxide [LiAl2 (OH)6] Cl.H2OChem Mater199810351355
- FoggAMDunnJSO’HareDFormation of second-stage intermediates in anion-exchange intercalation reactions of the layered double hydroxide [LiAl2(OH)6] Cl. H2O as observed by time-resolved, in situ x-ray diffractionChem Mater199810356360
- XiaSNiZXuQHuBHuJLayered double hydroxides as supports for intercalation and sustained release of antihypertensive drugsJ Solid State Chem200818126102619
- KongXJinLWeiMDuanXAntioxidant drugs intercalated into layered double hydroxide: structure and in vitro releaseAppl Clay Sci201049324329
- GunawanPXuRDirect control of drug release behavior from layered double hydroxides through particle interactionsJ Pharm Sci2008974367437818271032
- HusseinMZJaafarAMYahayaAHZainalZThe effect of single, binary and ternary anions of chloride, carbonate and phosphate on the release of 2, 4-dichlorophenoxyacetate intercalated into the Zn–Al-layered double hydroxide nanohybridNanoscale Res Lett200941351135720628454
- Bin HusseinMZYahayaAHZainalZKianLHNanocomposite-based controlled release formulation of an herbicide, 2, 4-dichlorophenoxyacetate incapsulated in zinc–aluminium-layered double hydroxideSci Technol Adv Mater20056956962
- MiyataSAnion-exchange properties of hydrotalcite-like compoundsClays Clay Miner198331305311
- ParkerLMMilestoneNBNewmanRHThe use of hydrotalcite as an anion absorbentInd Eng Chem Res19953411961202
- BerberMRHafezIHMinagawaKMoriTTanakaMNanocomposite formulation system of lipid-regulating drugs based on layered double hydroxide: synthesis, characterization and drug release propertiesPharm Res2010272394240120512405
- NakayamaHTakeshitaKTsuhakoMPreparation of 1-hydroxyethylidene-1, 1-diphosphonic acid-intercalated layered double hydroxide and its physicochemical propertiesJ Pharm Sci2003922419242614603487
- KurenkovVFHartanHGLobanovFIAlkaline hydrolysis of poly-acrylamideRuss J Appl Chem200174543554
- ArinaitweEPawlikMA method for measuring the degree of anionicity of polyacrylamide-based flocculantsInt J Mineral Process2009915054
- CostantinoUAmbrogiVNocchettiMPerioliLHydrotalcite-like compounds: versatile layered hosts of molecular anions with biological activityMicroporous Mesoporous Mater2008107149160
- LiBHeJEvansDGDuanXEnteric-coated layered double hydroxides as a controlled release drug delivery systemInt J Pharm2004287899515541916
- AmbrogiVPerioliLRicciMEudragit® and hydrotalcite-like anionic clay composite system for diclofenac colonic deliveryMicroporous Mesoporous Mater2008115405415
- ZeynaliMERabiiABaharvandHSynthesis of partially hydrolyzed polyacrylamide and investigation of solution properties (viscosity behaviour)Iran Polym J200413479484
- SinghviGSinghMReview: in-vitro drug release characterization modelsInt J Pharm Stud Res201127784
- KovandaFMaryškováZKovářPIntercalation of paracetamol into the hydrotalcite-like hostJ Solid State Chem201118433293335
- LokhandwalaHDeshpandeADeshpandeSKinetic modeling and dissolution profiles comparison: an overviewInt J Pharm Biol Sci20134728737
- AtwoodJLLehnJMAlbertiGBeinTSolid-State Supramolecular Chemistry: Two-and Three-Dimensional Inorganic Networks: Volume 7 (Comprehensive Supramolecular Chemistry)OxfordPergamon Press1996
- NgawhirunpatTGoegebakanEDuangjitSAkkaramongkolpornPKumpugdee-VollrathMControlled release of chlorpheniramine from resonates through surface coating with Eudragit®RS 100Int J Pharm Pharm Sci20102107112
- KabirAKLHalderSShumaMLRoufASSFormulation development and in vitro evaluation of drug release kinetics from sustained release aceclofenac matrix tablets using hydroxypropyl methyl celluloseDhaka Univ J Pharm Sci2012113743
- ZhangHPanDDuanXSynthesis, characterization, and magnetically controlled release behavior of novel core-shell structural magnetic ibuprofen-intercalated LDH nanohybridsJ Phys Chem C20091131214012148