Abstract
To develop an alternative treatment for lung cancer, a combination of two potent chemotherapeutic agents – liposomal apigenin and tyroservatide – was developed. The therapeutic potential of this combination was investigated using A549 cells. Apigenin and tocopherol derivative-containing D-alpha-tocopheryl polyethylene glycol 1000 succinate (TPGS) liposomes might improve the delivery of apigenin to tumor cells, both in vitro and in vivo. Importantly, compared to either agent alone, the combination of apigenin TPGS liposomes and tyroservatide exhibited superior cytotoxicity, induced stronger G2 arrest, and suppressed A549 cancer cell invasion at a lower dose. The proapoptotic synergistic effects were also observed in A549 cells using terminal deoxynucleotidyl transferase-mediated dUTP nick-end labeling, flow cytometry, and Western blot analysis. More importantly, in vivo results showed that the combination of apigenin TPGS liposomes and tyroservatide exhibited tumor-growth inhibitory effects in A549 cell-bearing mice. In conclusion, our study showed that this combination therapy could serve as a promising synergistic therapeutic approach to improve outcomes in patients with lung cancer.
Introduction
Cancer is considered a public health problem caused by complex underlying mechanisms. Lung cancer is known as the leading cause of cancer-related deaths worldwide.Citation1 Chemotherapeutic agents are considered central in the clinical management of lung cancer.Citation2,Citation3 Multidrug resistance and non-selective toxicity have been recognized as major problems associated with chemotherapeutic antitumor agents.Citation4–Citation6
Apigenin – a flavonoid extracted from vegetables and fruits, such as celery, parsley, and oranges – exhibits promising antitumor potential against lung cancer. It has many biochemical activities, including targeting of the phosphoinositide 3-kinase (PI3K)/Akt signaling pathway, upregulation of tumor necrosis factor (TNF)-related apoptosis-inducing ligand (TRAIL) death receptors DR4/DR5 expression in a p53-dependent manner, inhibition of glutamine metabolism, and induction of the epidermal growth factor receptor (EGFR) pathway.Citation7–Citation10 However, the clinical use of apigenin is restricted, owing to its poor biopharmaceutical properties, including water insolubility and poor absorption.Citation11 Although apigenin is predicted to be safe for lung cancer treatment, similar to the majority of antitumor drugs, resistance could develop during the course of treatment, which could result in therapeutic failure. These drawbacks could be overcome by using a liposomal form of apigenin and combining it with other chemotherapeutic drugs.
Liposomes – spherical vesicles composed of a phospholipid bilayer membrane and an aqueous core – are widely accepted as passive targeted drug-delivery systems with enhanced membrane permeability and improved retention.Citation12,Citation13 Several commercial liposome-based products are used clinically.Citation14–Citation18 D-alpha-tocopheryl polyethylene glycol 1000 succinate (TPGS) is formed from a water-soluble polyethylene glycol 1000-esterified derivative of vitamin E succinate, and has been used as a component of nanocarriers because it acts as a solubilizer, inhibits P-glycoprotein-mediated multidrug resistance, and enhances the bioavailability of anticancer drugs.Citation19–Citation23 Therefore, apigenin TPGS-coated liposomes were designed to increase the solubility, absorption, and retention, as well as to enhance the antitumor effects of apigenin.
Cancer treatment relying on a single antitumor drug does not usually result in satisfactory outcomes. Recent anticancer therapy using drug combinations has been found to be successful in clinical practice; thus, it is considered a promising strategy for enhancing the therapeutic efficacy.Citation24,Citation25 Moreover, synergistic anticancer drug combinations may counteract drug resistance, reduce the dose required, and enhance the antitumor effects. Therefore, cocktail therapy has been increasingly used as a primary cancer treatment regimen to avoid the poor response and resistance that is often encountered with monotherapy.Citation8,Citation26,Citation27 Tyroservatide (YSV) is a bioactive tripeptide (tyrosyl-seryl-valine) obtained from the spleen of pigs. It has been found to affect integrin-focal adhesion kinase signal transduction and inhibit histone deacetylase activity. YSV has been shown to exhibit antitumor effects in lung cancer.Citation28–Citation31 Therefore, a combination of YSV and apigenin liposomes may reduce chemoresistance.
In the present study, we aimed to compare the effectiveness of apigenin-loaded TPGS-coated liposomes with that of free apigenin. In addition, we investigated the potential synergistic effects of the combination of apigenin liposomes and YSV, and compared the antitumor efficacy of this combination with that of free apigenin and YSV.
Materials and methods
Materials, cell lines, and animals
YSV was synthesized by Shanghai Ziyu Biotech Co, Ltd. (Shanghai, People’s Republic of China). Injection-grade yolk lecithin PC-98T was purchased from Shanghai Advanced Vehicle Technology Ltd. Co. (Shanghai, People’s Republic of China). TPGS was obtained from Sigma-Aldrich (St Louis, MO, USA). Apigenin, coumarin 6 (C-6), 4′,6-diamidino-2-phenylindole (DAPI), and 1′-dioctadecyl-3,3,3′, 3′-tetramethylindotricarbocyanine iodide (DiR) were purchased from Aladdin Scientific Inc. (Shanghai, People’s Republic of China). Antibodies against caspase 3, caspase 8, caspase 9, poly (ADP-ribose) polymerase (PARP), Bax, and Bcl-2 were purchased from Nanjing KeyGen Biotech. Co. Ltd. (Nanjing, People’s Republic of China). Fetal bovine serum (FBS) and Roswell Park Memorial Institute (RPMI) 1640 medium were obtained from Gibco-BRL (Gaithersburg, MD, USA). All other reagents were of chromatography grade. A549 cells were supplied by Nanjing KeyGen Biotech. Inc. (Nanjing, People’s Republic of China) and grown in RPMI 1640 media containing FBS at 37°C in 5% CO2 atmosphere.
Male nude mice were purchased from the SLEK Lab Animal Center of Shanghai (Shanghai, People’s Republic of China), and received care according to the Guidelines on the Use of Laboratory Animals. All animal experiments were performed in accordance with the principles of care and use of laboratory animals, and were approved by the experimental animal administrative committee of Suqian Branch Jiangsu Province Hospital. Ethical and legal approval was obtained prior to the study. The nude mice were subcutaneously inoculated with A549 cells (5×106 cells/mouse); tumor size was measured by a Vernier caliper, and the tumor volume (V) was calculated as follows: V = a × b2/2, where a represents the largest diameter, and b is the smallest one.
Preparation of apigenin-loaded TPGS liposomes
Apigenin-loaded TPGS liposomes were prepared as previously described, but with a slight modification.Citation32 Apigenin, yolk lecithin, and TPGS were dissolved in absolute alcohol, and the solution was evaporated under vacuum. The resultant thin film was hydrated with pure water for 12 h. After complete lipid hydration and formation of liposomes, the coarse dispersion was sonicated using a probe sonicator to obtain the apigenin-loaded TPGS liposomes; C-6 liposomes and DiR liposomes were also prepared using the same method.
Characterization of apigenin-loaded TPGS liposomes
The average size and zeta potential were measured by dynamic light scattering (Mastersizer 2000, Malvern, UK) at a sample temperature of 25°C after equilibration for 5 min. All liposome dispersions were diluted with distilled water (1:10) prior to the measurements.
The morphology of liposomes was investigated by transmission electron microscopy (TEM, JEM 100CX; JEOL, Tokyo, Japan). Apigenin-loaded TPGS liposomes were dropped on copper netting and stained with phosphomolybdic acid for morphological observation.
Cellular uptake and intracellular localization analysis
A549 cells were seeded and cultured at 37°C for 24 h. C-6, which can be encapsulated into various nanocarriers, was used as a model fluorescent molecule to evaluate the cellular uptake efficiency of the liposomes.
After treatment with C-6 or C-6 TPGS liposomes for 4 h, cells were washed with cold phosphate-buffered saline (PBS) thrice and immobilized with absolute ethyl alcohol for 10 min. Then, the cell nuclei were counterstained with DAPI and observed under a fluorescence microscope (IX71; Olympus Corp., Tokyo, Japan) or confocal laser scanning microscope (CLSM; Olympus Corp.).
In vivo bioluminescence imaging
When the tumor volume of A549-bearing male nude mice reached ~100 mm3, DiR or DiR TPGS liposomes were injected into the tail vein. The distribution of fluorescence was observed after injection to examine the targeting effect of TPGS liposomes. After imaging for 12 h, the nude mice were sacrificed, the major organs and tumors were harvested, and fluorescence images were captured.
MTT assay
A549 cells were seeded in a 96-well microtiter plate and cultured overnight. Then, the cells were treated with serial concentrations of apigenin, YSV, and apigenin plus YSV for 24 h. Cell viability was evaluated as described previously.Citation33
Cell-cycle analysis
Cells were seeded into six-well plates and incubated. After treatment with apigenin, YSV, or a combination of both for 24 h, cells were harvested and stained with propidium iodide in the dark for analysis by flow cytometry.
Wound-healing assays
The cell monolayer formed in a 24-well plate was scratched using a 200 μL pipette tip, washed three times with PBS, and then incubated in culture media that contained apigenin, YSV, or a combination of both for 24 h. The invasion potential was investigated using microscopy.
Cell apoptosis assay
DNA fragmentation was observed by terminal deoxynucleotidyl transferase-mediated dUTP nick-end labeling (TUNEL) assay 24 h after treatment. A549 cells were treated with proteinase K for 30 min at 37°C to remove the remaining nucleases. The cytoblasts were stained with the TUNEL reaction solution in a dark room. Apoptotic cytoblasts and normal nuclei were labeled brown and purple, respectively. Both were photographed for morphological examination.
For quantitative analysis of the apoptotic ratio after treatment with apigenin, YSV, and a combination of both for 24 h, A549 cells were trypsinized and resuspended in PBS. Then, the cells were analyzed by flow cytometry using an Annexin V-FITC apoptosis detection kit.
For analysis of apoptotic protein expression after treatment with apigenin, YSV, and a combination of both for 24 h, total protein was isolated after cell treatment with a lysis buffer. Skimmed milk was used to block nonspecific membrane protein binding. Samples were incubated with the primary antibodies overnight at 4°C, and then incubated with the secondary antibodies. The proteins were quantified using Western blot analysis.
Antitumor efficacy in vivo
The antitumor efficacy of apigenin, YSV, and a combination of both was investigated in a xenograft model using nude mice. Mice were randomly divided into five groups (n=5) when the tumor volumes were ~50 mm3. The mice were intravenously treated with apigenin (2.7 μg/kg), YSV (270 μg/kg), or a combination, via the tail vein, every 3 days. Tumor volumes and body weight were recorded every 3 days. Mice were sacrificed 15 days after the initial injection. Tumors were excised for histopathological analysis using hematoxylin and eosin (H&E) staining.
Data analysis
Data are expressed as the means ± standard deviation. Synergism was evaluated using the CompuSyn software with Chou–Talalay plotting. P-values <0.05 were considered statistically significant.
Results and discussion
Characterization of apigenin-loaded TPGS liposomes
The average size, zeta potential, and morphology of apigenin-loaded TPGS liposomes are shown in . The size of apigenin-loaded TPGS liposomes was 118.6±8.1 nm, with a uniform distribution. The apigenin-loaded TPGS liposomes had a negative zeta potential of −6.8±0.28 mV owing to the presence of surface TPGS. As shown in , apigenin-loaded TPGS liposomes existed as homogeneous spheres with a mean diameter of ~100 nm. The liposome size observed by TEM was consistent with that measured by dynamic light scattering.
Cellular uptake and intracellular distribution of TPGS liposomes
After incubation for 4 h at 37°C, green fluorescence was observed in C-6 and C-6 TPGS liposome-treated cells, with the latter showing more intense fluorescence (), which indicated an increased amount of C-6 uptake with C-6 TPGS liposomes. To further elucidate the permeability-enhancing effects of TPGS liposomes, intracellular localization was examined, as shown in . Most of the C-6 was internalized and distributed through the cytoplasm, showing that TPGS liposomes were successfully taken up by A549 cells.
In vivo fluorescence imaging studies
Free DiR and DiR-labeled TPGS liposomes were injected into the tail vein of nude mice to evaluate the targeting effect of TPGS liposomes. As shown in , the DiR TPGS liposomes started to accumulate in tumor tissues 4 h after injection. The fluorescent signal in the tumor sites reached the maximum intensity at 8 h. Subsequently, the fluorescence intensity gradually decreased; however, it remained until 12 h. The ex vivo data showed that the tumor, liver, and spleen retained fluorescence for 12 h. It could be inferred that TPGS-coated liposomes delivered the antitumor drug directly into the tumor, and was retained for a longer period than that delivered through passive diffusion.Citation34 Thus, TPGS-coated liposomal delivery of anticancer drugs would enhance their antitumor efficacy.
Evaluation of the combination effect of apigenin and YSV in A549 cells
Before investigation of the combined effects of apigenin and YSV therapy, we evaluated the cytotoxicity of apigenin and YSV monotherapy in the A549 cell line using an MTT assay. Then, the cytotoxicity of different molar combinations of apigenin and YSV was evaluated. Our results showed that, at a concentration of 2.7 μg/mL, apigenin exhibited weak antitumor effects in A549 cells. When A549 cells were treated with apigenin plus YSV (apigenin, 2.7 μg/mL; YSV, 270 μg/mL), >50% tumor inhibition was observed. However, in the monotherapy treatment groups, <20% inhibition was observed at the same concentrations (). Thus, it could be inferred that the combination of apigenin and YSV exhibited synergistic antitumor effects. To assess whether apigenin and YSV combination at a ratio of 1:100 exhibited synergistic effects against lung cancer, we performed a Chou–Talalay analysis.Citation35,Citation36 Our results showed that the combination index (CI) of apigenin and YSV was less than 1 at an apigenin:YSV ratio of 1:100. The CI for apigenin (0.34, 0.68, 1.35, 2.7, and 5.4 μg/mL) combination treatment was found to be <1 (CI =0.68, 0.65, 0.54, 0.53, and 0.52, respectively), suggesting that this combination interacted synergistically at a ratio of 1:100 (). Therefore, apigenin at 2.7 μg/mL and YSV at 270 μg/mL (ratio =1:100) were selected as optimal doses for combination therapy in the subsequent experiments.
Synergistic effects on cell cycle
The cell-cycle distribution was assayed using flow cytometric analysis. There was an increase in the G2/M phase fraction in the A549 cells treated with either apigenin or YSV alone, compared to that in the control cells (). Moreover, combination therapy significantly increased the fraction of cells in G2/M phase, compared to those treated with either apigenin or YSV alone. When apigenin TPGS liposomes were used, the G2/M population increased dramatically from 28.4% to 38.6%, compared to that of the combination of free apigenin and YSV, which could be attributable to the intracellular accumulation of apigenin delivered from the TPGS liposomes (P<0.05).
Effect on cell invasion
A wound-healing assay was used to evaluate the invasive potential in the presence of the test agents. The results revealed that both apigenin and YSV individually exerted an inhibitory effect on cancer cell invasion. However, the anti-invasive effects of apigenin and YSV combination were greater than that of either apigenin or YSV alone (P<0.05). The cell–cell distance was maximal after treatment with apigenin TPGS liposomes plus YSV, compared to the other groups ().
Proapoptotic effects of the combination treatment in A549 cells
Next, we investigated whether apigenin TPGS liposomes and YSV combination exerted synergistic proapoptotic effects. Qualitative and quantitative apoptosis analyses were performed using inverted microscopy, flow cytometry, and Western blot analysis. When cells were exposed to a combination of apigenin TPGS liposomes and YSV, apoptotic morphology – mainly, chromatin edge fog, membrane aggregation, and nuclear dimpling – was observed (). In the control and apigenin only-treated groups, TUNEL-positive apoptotic cells were distributed sporadically, whereas in the other groups, the number of TUNEL-positive apoptotic cells greatly increased (P<0.05). Interestingly, the number of apoptotic cells was larger in the apigenin TPGS liposomes and YSV combination-treated group, compared to the apigenin and YSV combination group (P<0.05), suggesting that the liposome-enhanced uptake of apigenin increased apoptosis of A549 cells.
Figure 5 TUNEL (A), Annexin V-FITC apoptosis detection (B), and Western blot analysis (C) of apigenin-loaded TPGS liposomes and YSV in vitro in A549 cells (n=3).
Abbreviations: FITC, fluorescein isothiocyanate; TPGS, D-alpha-tocopheryl polyethylene glycol 1000 succinate.
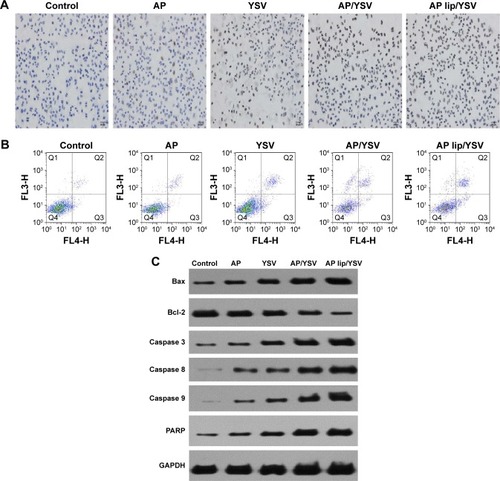
In addition, flow cytometry was performed to distinguish between apoptosis and necrosis. As shown in , apigenin, YSV, and the apigenin and YSV combination induced 5.1%, 10.3%, and 19.6% apoptosis, which indicated that the combination therapy resulted in a 3.84- and 1.91-fold increase in apoptosis, compared to apigenin and YSV alone (P<0.05), respectively. These data showed that apigenin and YSV exhibited synergic proapoptotic effects. Moreover, the combination of apigenin TPGS liposomes and YSV induced 28.9% A549 cell apoptosis, with a 1.47-fold increase compared to the combination of free apigenin and YSV (P<0.05). Therefore, the enhanced uptake of apigenin delivered by TPGS liposomes combined with YSV increased cell apoptosis, which might further result in greater antitumor efficacy.
Expression levels of the key regulator proapoptotic proteins were also measured by Western blot analysis. Combined apigenin and YSV elicited higher expression levels of the key regulator proapoptotic proteins, compared to the individual agents, demonstrating the stronger effects of the combination therapy. In addition, results showed that Bcl-2 levels decreased, whereas Bax, caspase 3, caspase 8, caspase 9, and PARP levels significantly increased. Moreover, Bax/Bcl-2 ratio significantly increased after apigenin TPGS liposomes and YSV combination treatment (). Taken together, the apigenin TPGS liposomes and YSV combination exhibited higher efficacy, compared to free apigenin and the YSV combination.
In vivo antitumor activity
To confirm whether this combination could have an impact on the efficacy of lung cancer treatment, the efficiency of the synergistic antitumor combinations was tested in vivo using A549 tumor-bearing nude mice. As shown in , regarding the individual agents, apigenin had a slightly better antitumor activity at low concentrations; in addition, tumor volumes in the YSV group were significantly smaller than those in the saline-treated group (P<0.05). The combination of apigenin and YSV showed stronger antitumor effects than either monotherapy, confirming the synergistic effects of the combination. Apigenin TPGS liposomes and YSV combination exhibited the strongest antitumor effects (P<0.05), which suggested that TPGS liposomal delivery system was superior. There was no change in body weight of the mice, which indicated that the formulations were safe.
Figure 6 Curves of tumor volumes (A), morphology (B), and typical H&E-stained images (C) (‘N’ represents necrosis area, ‘T’ represents tumor mass) on day 15 after injection of the indicated formulations into the tail vein (n=5).
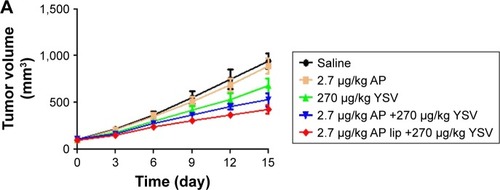
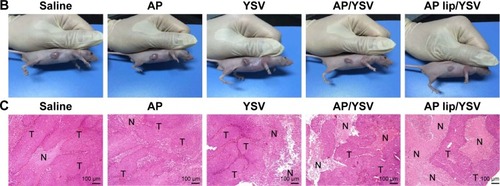
To further confirm the synergistic antitumor effects, H&E staining of tumor slices was performed to assess tumor cell death.Citation37 Both the saline- and apigenin-treated groups showed a large number of living cancer cells that appeared blue. The combination of apigenin and YSV induced significant cancer cell necrosis, which appeared pink, compared to treatment with each individual agent. The combination treatment of apigenin TPGS liposomes and YSV showed the strongest necrotic effects, indicating improved synergism provided by the TPGS liposomal delivery system ().
Conclusion
The present study aimed to study the potential applications of apigenin TPGS liposomes and YSV combination as anticancer therapy. We showed that cocktail therapy using a combination of apigenin TPGS liposomes and YSV, which exhibited significant and synergistic proapoptotic effects, could provide an effective chemotherapeutic regimen for lung cancer treatment. MTT assay showed that low concentrations of apigenin exerted low in vitro cytotoxicity in A549 cancer cells. Imaging of cellular uptake in vitro and in vivo suggested that TPGS liposomes enhanced tumor targeting and apigenin uptake by tumor cells. Cocktail therapy using apigenin TPGS liposomes and YSV exhibited significantly stronger proapoptotic effects in vitro and in vivo, compared to that of apigenin and YSV either alone or in combination. Therefore, the combination of apigenin TPGS liposomes and YSV could be useful in lung cancer treatment in the future.
Acknowledgments
This work was supported by the National Natural Science Foundation of China (Grant no 81403119), 333 project of Jiangsu Province (BRA2016258), and the Science and Technology Support Project of Suqian.
Disclosure
The authors report no conflicts of interest in this work.
References
- SiegelRLMillerKDJemalACancer statistics, 2017CA Cancer J Clin201767173028055103
- TanguturADKumarDKrishnaKVKantevariSMicrotubule targeting agents as cancer chemotherapeutics: an overview of molecular hybrids as stabilising and destabilising agentsCurr Top Med Chem Epub201714
- WijdevenRHPangBAssarafYGNeefjesJOld drugs, novel ways out: drug resistance toward cytotoxic chemotherapeuticsDrug Resist Updat201628658127620955
- WallsGMLyonARHarbinsonMTHannaGGCardiotoxicity following cancer treatmentUlster Med J20178613928298705
- PapadasAAsimakopoulosFMechanisms of resistance in multiple myelomaHandb Exp Pharmacol Epub2017318
- ColmegnaBMorosiLD’IncalciMMolecular and pharmacological mechanisms of drug resistance: an evolving paradigmHandb Exp Pharmacol Epub2017323
- ZhouZTangMLiuYZhangZLuRLuJApigenin inhibits cell proliferation, migration, and invasion by targeting Akt in the A549 human lung cancer cell lineAnticancer Drugs201728444645628125432
- ChenMWangXZhaDApigenin potentiates TRAIL therapy of non-small cell lung cancer via upregulating DR4/DR5 expression in a p53-dependent mannerSci Rep201663546827752089
- LeeYMLeeGOhTIInhibition of glutamine utilization sensitizes lung cancer cells to apigenin-induced apoptosis resulting from metabolic and oxidative stressInt J Oncol201648139940826573871
- ChengCYHuCCYangHJLeeMCKaoESInhibitory effects of scutellarein on proliferation of human lung cancer A549 cells through ERK and NFκB mediated by the EGFR pathwayChin J Physiol201457418218725246059
- ZhangJLiuDHuangYGaoYQianSBiopharmaceutics classification and intestinal absorption study of apigeninInt J Pharm20124361–231131722796171
- CastoldiAHerrCNiederstraßerJCalcifediol-loaded liposomes for local treatment of pulmonary bacterial infectionsEur J Pharm Biopharm Epub20161122
- JøraholmenMWBasnetPAcharyaGŠkalko-BasnetNPEGylated liposomes for topical vaginal therapy improve delivery of interferon alphaEur J Pharm Biopharm201711313213928087379
- FouladiFSteffenKJMallikSEnzyme-responsive liposomes for the delivery of anticancer drugsBioconjug Chem201728485786828201868
- LeeYThompsonDHStimuli-responsive liposomes for drug deliveryWiley Interdiscip Rev Nanomed Nanobiotechnol Epub2017215
- PanahiYFarshbafMMohammadhosseiniMRecent advances on liposomal nanoparticles: synthesis, characterization and biomedical applicationsArtif Cells Nanomed Biotechnol201745478878928278586
- ChangHIYehMKClinical development of liposome-based drugs: formulation, characterization, and therapeutic efficacyInt J Nanomedicine20127496022275822
- BozzutoGMolinariALiposomes as nanomedical devicesInt J Nanomedicine20151097599925678787
- DuhemNDanhierFPréatVVitamin E-based nanomedicines for anti-cancer drug deliveryJ Control Release2014182334424631865
- GuoYLuoJTanSOtienoBOZhangZThe applications of Vitamin E TPGS in drug deliveryEur J Pharm Sci201349217518623485439
- ZhangZTanSFengSSVitamin E TPGS as a molecular biomaterial for drug deliveryBiomaterials201233194889490622498300
- ShenRKimJJYaoMElbayoumiTADevelopment and evaluation of vitamin E d-α-tocopheryl polyethylene glycol 1000 succinate-mixed polymeric phospholipid micelles of berberine as an anticancer nanopharmaceuticalInt J Nanomedicine2016111687170027217747
- HaoJTongTJinKFolic acid-functionalized drug delivery platform of resveratrol based on Pluronic 127/D-α-tocopheryl polyethylene glycol 1000 succinate mixed micellesInt J Nanomedicine2017122279229228392687
- ZhangXYZhangPYCombinations in multimodality treatments and clinical outcomes during cancerOncol Lett20161264301430428101195
- Bhaw-LuximonAJhurryDArtemisinin and its derivatives in cancer therapy: status of progress, mechanism of action, and future perspectivesCancer Chemother Pharmacol201779345146628210763
- CosenzaMCivalleroMPozziSMarcheselliLBariASacchiSThe combination of bortezomib with enzastaurin or lenalidomide enhances cytotoxicity in follicular and mantle cell lymphoma cell linesHematol Oncol2015334166175
- ArasBYerlikayaABortezomib and etoposide combinations exert synergistic effects on the human prostate cancer cell line PC-3Oncol Lett20161153179318427123085
- HuangYTZhaoLFuZTherapeutic effects of tyroservatide on metastasis of lung cancer and its mechanism affecting integrin-focal adhesion kinase signal transductionDrug Des Devel Ther201610649663
- XuQLuRZhuZFEffects of tyroservatide on histone acetylation in lung carcinoma cellsInt J Cancer2011128246047220309941
- FuZLuRJiaJInhibition of five xenografted human cancers and two murine cancers by the tripeptide tyroservatideAnticancer Drugs200718446747017351399
- ZhengMLuRCheXTyroservatide therapy for tumor growth, invasion and metastasis of Lewis lung carcinoma and human lung carcinoma A549Oncology200670641842617245103
- JinXLiMYinLZhouJZhangZLvHTyroservatide-TPGS-paclitaxel liposomes: tyroservatide as a targeting ligand for improving breast cancer treatmentNanomedicine20171331105111527845234
- JinXZhangYZhangZCheDLvHJuglone loaded poloxamer 188/phospholipid mixed micelles evaluated in vitro and in vivo in breast cancerInt J Pharm20165151–235936627744033
- TaurinSNehoffHGreishKAnticancer nanomedicine and tumor vascular permeability; Where is the missing link?J Control Release2012164326527522800576
- ChouTCDrug combination studies and their synergy quantification using the Chou-Talalay methodCancer Res201070244044620068163
- ChouTCTheoretical basis, experimental design, and computerized simulation of synergism and antagonism in drug combination studiesPharmacol Rev200658362168116968952
- ChenMZhangYChenZXieSLuoXLiXSynergistic antitumor efficacy of redox and pH dually responsive micelleplexes for co-delivery of camptothecin and genesActa Biomater20174944445527940163