Abstract
Graphene-based materials (GBMs) are widely used in many fields, including biomedicine. To date, much attention had been paid to the potential unexpected toxic effects of GBMs. Here, we review the recent literature regarding the impact of GBMs on programmed cell death (PCD). Apoptosis, autophagy, and programmed necrosis are three major PCDs. Mechanistic studies demonstrated that the mitochondrial pathways and MAPKs (JNK, ERK, and p38)- and TGF-β-related signaling pathways are implicated in GBMs-induced apoptosis. Autophagy, unlike apoptosis and necroptosis which are already clear cell death types, plays a vital pro-survival role in cell homeostasis, so its role in cell death should be carefully considered. However, GBMs always induce unrestrained autophagy accelerating cell death. GBMs trigger autophagy through inducing autophagosome accumulation and lysosome impairment. Mitochondrial dysfunction, ER stress, TLRs signaling pathways, and p38 MAPK and NF-κB pathways participate in GBMs-induced autophagy. Programmed necrosis can be activated by RIP kinases, PARP, and TLR-4 signaling in macrophages after GBMs exposure. Though apoptosis, autophagy, and necroptosis are distinguished by some characteristics, their numerous signaling pathways comprise an interconnected network and correlate with each other, such as the TLRs, p53 signaling pathways, and the Beclin-1 and Bcl-2 interaction. A better understanding of the mechanisms of PCD induced by GBMs may allow for a thorough study of the toxicology of GBMs and a more precise determination of the consequences of human exposure to GBMs. These determinations will also benefit safety assessments of the biomedical and therapeutic applications of GBMs.
Introduction
Graphene materials have various applications and are widely used in the biomedical field in tumor therapy,Citation1–Citation3 diagnostics,Citation4,Citation5 bio-imaging,Citation6–Citation8 and drug delivery.Citation9,Citation10 The widespread use of graphene materials has prompted concerns about the possible human health and environmental safety impacts of these materials. GBMs present in biomedical and non-biomedical products have shown potential toxicity to human beings, animals, and cells.Citation11–Citation13 Several reviews have summarized that GBMs resulted in various degrees of cell death.Citation14,Citation15 For example, GO and rGO treatments resulted in dose-dependent cell death in A549 cells and HUVEC cells, and the functionalization decreased cytotoxicity and cell death.Citation16,Citation17 Cell death is a signal that reflects the toxicity of GBMs,Citation18–Citation20 and this review focuses on the mechanisms of GBMs-induced PCD.
A systematic classification of cell death was recently presented in a set of recommendations by the Nomenclature Committee on Cell Death.Citation21 PCD is proposed to be death of a cell in any pathological format, when mediated by an intracellular program. PCD balances cell death with survival of normal cells when the equilibrium becomes disturbed. It is suggested that PCD is mediated by specific cellular mechanisms and that several signal pathways are activated and involved in these processes.Citation22 Several PCD modalities, including apoptosis, autophagy, and programmed necrosis (also called necroptosis), have been investigated in the studies of the toxicity of nanomaterials.Citation23,Citation24 In particular, apoptosis, autophagy, and necroptosis are usually involved in GBMs toxicology.Citation25–Citation27 Many studies have reported that the different physicochemical properties of GBMs result in various cellular toxicities, and GBMs induce cell death in dose- or time-dependent manner.Citation28 However, the detailed mechanism of PCD induced by GBMs has not been well elucidated. Since many other reviews had illustrated that the different physicochemical properties of GBMs largely impacted on the cell toxicity,Citation29–Citation31 we concentrate on summarizing the pathways of PCD induced by GBMs in this review, and leave aside the effect of administration route, administration dose, and the physicochemical properties of GBMs.
Notably, apoptosis, autophagy, and necroptosis can be distinguished by their unique morphological differences and physiological processes; however, the underlying signaling pathways are intricate and delicate.Citation32,Citation33 Evidence has illustrated that apoptosis and necroptosis are two manners of cell death, whereas autophagy can play either pro-survival or pro-death roles. Under some conditions, apoptosis, autophagy, and necroptosis pathways are somehow cross-linked with each other. Herein, we review some studies on GBMs-induced PCD and summarize part of the signaling pathways, and analyze some differences and correlation between PCDs.
GBMs induce apoptosis in cells
Apoptosis is one of the most important toxic features described by studies of GBMs toxicity. GBMs induce apoptosis both in vivo and in vitro, and the extent of apoptosis differs depending on the varying properties of GBMs, including surface area, layer number, lateral dimension, functional groups, and surface chemistry. Recently, hydrophilic GO showed cellular uptake resulting in apoptosis following cell morphological changes from an elongated to a spherical morphology; conversely, hydrophobic rGO was found to be mostly adsorbed at cell surface without internalization at a dose of 20 mg/L.Citation34–Citation36 Graphene layers (10 μg/mL) induced apoptosis by activating caspase-3 in a time-dependent manner.Citation37 GO nanoribbons (100 μg/mL) and GO polyethylenimine (1.6 μg/mL) triggered severe apoptosis by translocating to the cell nucleus.Citation38,Citation39 However, polyvinyl pyrrolidone-coated GO and nano-GO coated with polyethylene glycol triggered weaker apoptosis compared to GO in a dose-dependent manner.Citation40,Citation41
GBMs-induced apoptosis somehow promoted diseases. The cell cycle arrest and DNA fragmentation caused by GO could induce germline apoptosis, affecting gonad development at the concentration of 10 mg/L.Citation42 GQDs may translocate to the brain and localize to the nucleus of the diencephalon, causing Parkinson’s disease-like symptoms by inducing cell apoptosis and senescence.Citation43 Long-term and chronic exposure to GBMs may result in some diseases or do harm to the health.
However, numerous studies have demonstrated the therapeutic value of GBMs in cancer cells. GBMs, similarly to several anticancer compounds, exert their inhibitory effect on tumors by arresting the cell cycle at a specific checkpoint, activating apoptosis, or a combined effect of both processes.Citation44 In vivo, GO and rGO injections decrease the mass and volume of tumors, and in vitro, GO and rGO (50 μg/mL) treatments induce apoptosis by the upregulation of caspase-3 and membrane leakage.Citation45,Citation46 GQDs (100 μg/mL) induced apoptosis of U251 human glioma cells displaying morphological and biochemical characteristics, such as phosphatidylserine externalization, caspase activation, and DNA fragmentation.Citation47 Similarly, PEGylated silver nanoparticles coated with GQDs exerted a distinct antitumor effect at the level of 100 μg/mL by triggering apoptosis only in cancer cells without impacting the viability of normal cells.Citation48 Consequently, understanding of how to improve the antitumor effects and decrease the toxicity of normal cells may allow good use of GBMs in biomedicine.
Since apoptosis induced by GBMs emerges in many situations, we summarize some information to describe GBMs-induced apoptosis, such as the physiochemical properties and functionalization of GBMs, the exposed cells, the exposed dose and time, and so on. The information is collectively presented in .
Table 1 GBMs induced apoptosis
The signaling pathways involved in GBM-induced apoptosis
Apoptosis, or type I PCD, is a gene-controlled cell death process maintaining cell homeostasis and is characterized by specific morphological changes such as cell shrinkage, nuclear condensation and fragmentation, and the appearance of apoptotic bodies.Citation49,Citation50 Apoptosis is divided into extrinsic (death receptor pathway) and intrinsic (mitochondrial pathway) pathways.Citation51 The extrinsic pathway is mediated by the TNF receptor superfamily, and the binding ligands are Fas ligand, TNF-R, or TRAIL.Citation52 The Fas/Fas-L complex recruits the FADD and pro-caspase-8 to form the DISC. This protein complex serves as a platform for caspase activation, and the autocatalytic activation of caspase-8 at the DISC leads to the proteolysis of pro-caspase-3 into active caspase-3, triggering the apoptotic process.Citation53 The intrinsic pathway is under the control of mitochondrial proenzymes with a variety of functions, including the dissipation of the mitochondrial transmembrane potential and the release of proapoptotic proteins into the cytosol, as well as a second, mitochondria-derived activator of caspases. After being triggered by extracellular stimuli or intracellular signals, internal cytochrome c is released into the cytosol from the permeable mitochondrial membranes and recruits Apaf-1 and pro-caspase-9 to the apoptosome, which activates the downstream caspase-9/3 signaling cascade and consequently results in apoptosis.Citation54,Citation55
Apoptosis is a complex process that can be activated through several signaling pathways.Citation56 Recently, much attention has been paid to clarifying the intricate mechanism of apoptosis in GBMs-induced toxicity. GBMs-induced apoptosis usually has the fundamental features of caspase activation, DNA fragmentation, increased oxidative stress, and calcium efflux.Citation57,Citation58
Mechanistic studies demonstrated that mitochondria are implicated in apoptotic cell death, which act as major control points responsible for regulating apoptosis. It is well accepted that mitochondria participate in the intrinsic pathway of apoptosis and that they release soluble proteins from the intermembrane space to the cytosol to initiate caspase activation.Citation59,Citation60 For example, caspase-3, one of the most important executioner caspases, and PARP are activated by mitochondrial pathways that initiate pristine graphene-mediated apoptosis.Citation61,Citation62 Exposure to graphene increases intracellular ROS generation, including mitochondria-produced superoxide, which subsequently results in the loss of the MMP.Citation62,Citation63 Disruptions of the MMP may lead to broken electron transfer chain, decline in ATP synthesis, and release of proapoptotic molecules from the mitochondria to cytoplasm. Similarly, GO and rGO treatments also cause mitochondrial damage and accelerate apoptosis by generating oxidative stress.Citation64,Citation65 Exposure to few-layer graphene caused elevation of cytoplasmic Ca2+ concentration and mitochondrial membrane depolarization, and eventually, the abnormal opening of the mitochondrial permeability transition pore led to more severe mitochondrial damage and the initiation of apoptosis.Citation57 Notably, TLRs also contribute to the loss of MMP, consequently inducing apoptosis after exposure to rGO.Citation66
In addition to mitochondrial pathways, the MAPKs (JNK, ERK, and p38)- and TGF-β-related signaling pathways are also involved in GBMs-induced apoptosis.Citation62 The MAPK (JNK, ERK, and p38) signaling pathway involves a family of serine/threonine protein kinases that regulates cell proliferation, cell survival, and apoptosis.Citation67,Citation68 JNK activates the Bcl-2 protein family proapoptotic members, Bim and Bax, eliciting MOMP during GBM-induced apoptosis.Citation69,Citation70 Subsequently, mitochondrial proapoptotic factors activate caspase-3, an important effector caspase involved in apoptosis.Citation71 The p38 protein is activated by ROS and can induce apoptosis following the stimulation of TNF-α, the recruitment of caspase-8 and caspase-3, and the activation of the downstream effector protein PARP. In addition, TGF-β participating in cell apoptosis has been shown in several cell lines;Citation72–Citation74 however, the TGF-β-related signaling pathways have not been well studied in GBM-induced apoptosis. The proteins downstream of TGF-β, Smad proteins, activate proapoptotic factors and relocate from the mitochondria to the cytosol to regulate cell apoptosis. These pathways may be a good research direction for further study of GBMs-induced apoptosis. GBMs-induced apoptosis pathways are important parts of GBMs-induced toxicity, and some of them are delineated in .
Figure 1 The signaling pathways involved in GBM-induced apoptosis. The schematic diagram delineates the extrinsic (death receptor pathway) and intrinsic (mitochondrial pathway) pathways of apoptosis. GBMs may induce apoptosis through mitochondrial pathways and the MAPKs- and TGF-β-related signaling pathways. Mitochondria act as major control points involving in regulation of apoptosis. Caspases (caspase-3, caspase-8), PARP, and Bcl-2 protein family proapoptotic members (Bim and Bax) are activated and mitochondrial outer membrane permeabilization and the loss of mitochondrial membrane permeabilization occur, and are involved in the pathways during GBM-induced apoptosis.
Abbreviation: GBMs, graphene-based materials.
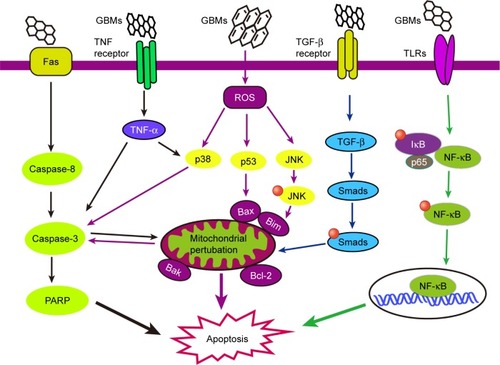
GBMs induce autophagy in cells
In the current toxicology study of GBMs, there is no detailed research on distinguishing between autophagy, autophagic cell death, and cell death caused by autophagy. Rather, we just discuss autophagy triggered or inhibited by GBMs in the following text. GO triggers autophagy in macrophages in a concentration-dependent manner. Furthermore, GO can induce autophagy in various cell lines, including SNU-449, Mahlavu, A549, HEK293, and RAW 264.7 macrophages, at a concentration of 100 μg/mL by stimulating the TLR signaling cascades.Citation75 GO exposure to the human-hamster hybrid (AL) cells significantly elicited genuine autophagy by increasing the level of LC3-II and the autophagic flux even at a low dose (1 μg/mL) or an early time point (4 h).Citation76 GO was phagocytosed by murine colon carcinoma (CT26) cells and simultaneously triggered autophagy by TLR-4/9 signaling cascades at a dose of 50 μg/mL.Citation77 It was also suggested that GO was a potent autophagy inducer in CT26 colon cancer cells, and thus in chemotherapy, GO combined with the chemotherapy drug cisplatin (CDDP) (GO/CDDP) diverted the autophagic flux and improved antitumor effects at a dose of 50 μg/mL.Citation78 GNPs promoted autophagy by increasing the conversion from LC3B-I to LC3B-II and the level of p62 protein at 24 h after exposure in a dose-dependent manner in BEAS-2B cells.Citation79 Similarly, GQDs also significantly dose-dependently increased ROS generation and induced autophagy with an increase in the expression of LC3-II/I and Beclin-1 in macrophages or U251 human glioma cells.Citation26,Citation47 Since autophagy may eliminate GBM particles or provide energy through cleaning the remnant organelle in cells, it may become a primary index to evaluate the toxicity of GBMs in occupational exposure or biomedical application. A summary of GBMs’ physicochemical properties, exposed cells, toxicokinetics, and the autophagy results from the literature reviewed here is listed in .
Table 2 GBMs induced autophagy in cells
The signaling pathways involved in GBMs-induced autophagy
Autophagic cell death is sometimes called type II PCD, and some experts had come up with an opposite opinion that autophagy might not cause cell death, and autophagy is different from autophagic cell death. They said that autophagic cell death is the cell death caused by autophagy rather than the cell death that autophagy participates in. Moreover, they pointed out that if the inhibitor of autophagy cannot prevent cell death, it cannot be called autophagic cell death.Citation80 Until now, the underlying mechanism of autophagy is far from clear. In addition, in the study of toxicity induced by GBMs, there are no detailed debates about whether autophagy can be called type II PCD. Furthermore in most situations, autophagy is taken as one kind of cell death or the main reason of cell death. GBMs always induced unrestrained autophagy, which would accelerate rather than prevent cell death.
Autophagy is characterized by the formation of autophagosomes, with double membrane-bound structures wrapping organelles and cytoplasmic macromolecules that subsequently fuse with lysosomes to form autolysosomes, thereby degrading the contents of the encapsulated vacuole.Citation81,Citation82 mTOR is the checkpoint at the initiation step of autophagy. Autophagy is initiated by negatively regulating the PI3K/Akt pathway and mTOR activity; however, Bcl-2 can bind to Beclin-1 and reverse this outcome. Moreover, the activated AMPK can induce autophagy through a phosphorylation event that stimulates TSC1/TSC2 activity, and the TSC1/TSC2 complex can negatively regulate mTORC1. In contrast, the phosphorylation and inactivation of TSC1/TSC2 allow for mTOR activation via the PI3K/Akt signaling pathway inhibiting autophagy.Citation83–Citation86 Many signaling pathways of autophagy have been verified in diseases, but those pathways need to be further studied in biocompatibility and toxicology of GBMs.
The classic process of autophagy is autophagosome accumulation and LC3-I/LC3-II conversion. Nascent LC3 is modified at its c-terminal domains by Atg4 and becomes LC3-I, which subsequently couples with phosphatidylethanolamine to become LC3-II. LC3-II then translocates to the autophagosome membrane,Citation87–Citation89 and the short hairpin RNA against LC3-II can block the autophagic response.Citation47 GO induced autophagosome accumulation and lysosome impairment disrupting intracellular homeostasis and promoted cell death after GBMs treatment in primary murine peritoneal macrophages and other cell lines.Citation90,Citation91 GO overload on lysosomal vesicles caused lysosomal membrane destabilization and significant lysosome fusion, which consequently resulted in the blockade of autophagosomes and autophagosome accumulation.Citation81 It was elicited that lysosomal membrane permeabilization, lysosomal membrane rupture, and cytoskeleton disruption might be involved in lysosomal membrane destabilization, eventually resulting in autophagosome accumulation.Citation92 GO also leads to a decrease of p62, a protein that directly binds to LC3 and selectively incorporates into autophagosomes.Citation93 Moreover, p62 is preferentially degraded by autolysosomes; this degradation process is inversely correlated with autophagosomal maturation and degradation.Citation94 However, a study reported that GO inhibited the maturation of autolysosomes and prevented the fusion between autophagosomes and lysosomes, thereby reducing the integrity of autophagic flux.Citation95 GBMs led to different consequences of autophagy depending on the various concentrations, exposure times, and particle sizes.
Mitochondrial dysfunction and ER stress play vital roles in GBMs-induced autophagy. GBMs have been shown to induce mitochondrial damage and provoke autophagy in a human bronchial epithelial cell line (BEAS-2B cells).Citation79 ROS and NO generation after GNPs treatment resulted in decreased numbers of mitochondria, ER, and Golgi apparatus, and mitochondrial fission-initiated mitochondrial autophagy.Citation96 GNPs dramatically increased the levels of p62 and Bcl-2 proteins to induce autophagy.Citation79 The adaptor protein p62 can bind to ubiquitinated mitochondrial proteins and LC3 on autophagosomes, recruiting autophagic membranes for mitochondrial clearance.Citation97 Furthermore, Bcl-2 and Bcl-xL are antiapoptotic proteins that bind to Beclin-1, and the dissociation of the Bcl-2–Beclin-1 complex is essential for the initiation of autophagy.Citation98 Moreover, the dissociation of the Bcl-2–Beclin-1 complex allows Beclin-1 to be activated by AMBRA1, a protein that may reside on mitochondria and bind to Bcl-2 until it is released by autophagic stimuli.Citation99 Autophagy selectively removed mitochondria and adjusted the number of mitochondria to supply energy, adapting to the cell survival environment after GBMs exposure.
Different TLRs such as TLR-2, TLR-3, TLR-4, and TLR-7 are proposed as autophagy inductors,Citation100–Citation102 and some TLR signaling pathways participate in GO-induced autophagy. GO-activated autophagy is mediated through TLR-4/9 signaling pathways associated with the downstream signaling proteins MyD88, TRAF6, and NF-κB.Citation75 GO binds to TLRs activating MyD88 or TRIF, and recruiting the downstream proteins TRAF6 and Beclin-1. Beclin-1 can interact with TRAF6 to accelerate its activation and the formation of autophagosomes.Citation101,Citation103 Similarly, GO can be phagocytosed by CT26 colon cancer cells, triggering autophagy by both TLR-4 and TLR-9 signaling cascades. TLRs transduce signals first by MyD88, followed by TRAF6, NF-κB, and IRF7, eventually resulting in various cellular responses including secretion of cytokines TNF-α, IL-1β, and IFNs.Citation77 Except for TLR-4/9, the other TLRs have not been well studied in GBM-induced autophagy. It can be forecasted that the other TLRs may attract a lot of attention in the future.
Other signaling pathways are also involved in GBMs-induced autophagy. The p38 MAPK and NF-κB pathways participate in GQD-induced autophagy in THP-1 macrophages. GQDs significantly increase the phosphorylation of p38 MAPK and p65 and promote NF-κB translocation to the nucleus.Citation26 The overexpression or activation of p38α disrupts the p38–mAtg9 interaction and thereby influences LC3 lipidation and p62 degradation, thus controlling the induction of autophagy.Citation104 Moreover, mTORC1 is a major checkpoint in autophagy, and the activation or inhibition of mTOR pathway has been studied in other diseases or nanomaterials that may be investigated in future studies of GBM-induced PCD. Some pathways of GBM-induced autophagy are demonstrated in .
Figure 2 The signaling pathways involved in GBM-induced autophagy. The classic process of autophagy is autophagosome accumulation and LC3-I/LC3-II conversion which occurs after GBM treatment in murine peritoneal macrophages and other cell lines. Mitochondrial dysfunction and ER stress, TLRs, p38 MAPK, and NF-κB pathways participate in GBMs-induced autophagy. mTORC1 is a major checkpoint in autophagy, and Beclin-1 connects autophagy with apoptosis.
Abbreviations: GBMs, graphene-based materials; MOMP, mitochondrial outer membrane permeabilization.
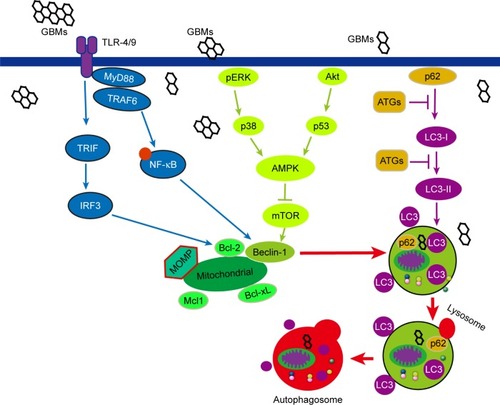
GBMs induce necroptosis and relative pathways involved
There are fewer studies of GBM-induced necroptosis compared to apoptosis and autophagy, and the mechanisms of necroptosis are not well studied. With the discovery of key regulators of necrotic death, such as RIP kinases and PARP, the concept of programmed necrosis has recently gained ground. RIP3 phosphorylation forms a tight RIP1–RIP3 complex, which is often needed to initiate the necrotic programme.Citation105 The results of previous studies have demonstrated that GO/CDDP potentiates CT26 cell death through programmed necrosis when the cells are exposed to a concentration of 50 mg/mL for 24 h. GO/CDDP induces programmed necrosis by increasing the levels of RIP1, RIP3, and HMGB1, a nuclear protein that is released into cytosol and then released from cells after the initiation of necrosis.Citation78
Programmed necrosis, type III PCD, is a crucial type of cell death for the preservation of tissue homoeostasis, the elimination of damaged cells, and immune responses.Citation106,Citation107 Signs of programmed necrosis include cell swelling, organelle dysfunction, and cell lysis.Citation108,Citation109 Although necrosis has always been considered an accidental form of cell death, the concept of programmed necrosis has been universally recognized since the discovery of key mediators of programmed necrosis, such as RIP kinases and PARP.Citation53 The death receptor (TNFR1) and TLRs can induce RIPK1- and RIPK3-dependent necrosis in the presence of FADD, caspase-8, TRADD, and TIR-related adaptor proteins. Furthermore, calcium-activated calpain can invoke JNK resulting in necroptosis.Citation110,Citation111
Programmed necrosis can also be activated by TLR-4 signaling in macrophages. GO (40 μg/mL)-induced programmed necrosis in macrophages occurs through a TLR-4-dependent signaling pathway and is partially TNF-dependent; this latter process also involves RIP-dependent and RIP-independent signaling pathways.Citation27 GO disrupts the integrity of the cytoskeleton of macrophages and promotes ROS generation, which is critical to TLR-4-mediated macrophagic necrosis. Blocking TLR-4 signaling with a selective inhibitor or using TLR-4-deficient BMDMs leads to resistance against GO-triggered necrosis. Moreover, GO increases TNF-α secretion upon activation of the TLR-4 pathway, and TNF-α triggers programmed necrosis via the activation of the kinases RIP1 and RIP3 or other signaling pathways. The study of the mechanism underlying GO-mediated programmed necrosis is just a good beginning, the outcome the relative mechanism is far from clear and complete. Other programmed necrosis pathways involved in the diseases have not been discussed in the study of GBMs. Some of the pathways of GO-triggered programmed necrosis are displayed in .
Figure 3 The signaling pathways involved in GBMs-induced necroptosis. RIP kinases and PARP are key regulators of programmed necrotic death, and the tight RIP1–RIP3 complex can initiate the necrotic program. GBMs can induce programmed necrosis through RIP-dependent and RIP-independent signaling pathways in macrophages via a TLR-4-dependent manner or partially by a TNF-dependent manner.
Abbreviation: GBMs, graphene-based materials.
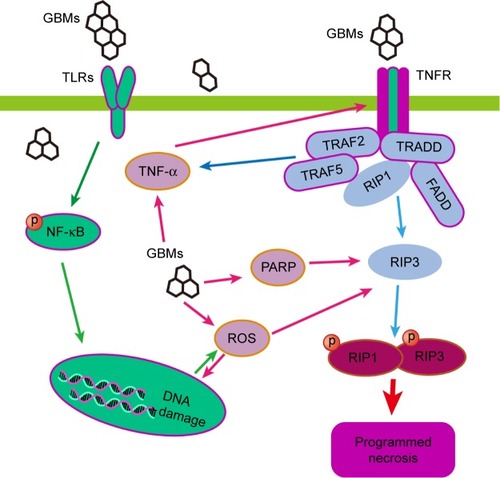
Some differences and relationships of GBMs-induced PCDs
Differences in PCD
From the discussion above, we find that we cannot sum up the law that GBMs induced what kinds of PCDs under certain circumstance. Different modes of PCDs appear in the same cells, which may be related to the cell phenotype, the physicochemical property and exposure time of GBMs, etc.Citation112 Often, several kinds of PCDs coexist in the same cell; however, we have not yet studied which kinds of PCDs come first in research of GBMs, nor do we try to understand how GBMs induce several PCDs and maintain the balance. All of these queries may be answered in the future.
PCDs are distinguished by some characteristics. Apoptosis is morphologically characterized by cell shrinkage, chromatin aggregation, DNA fragmentation, and apoptotic body formation. In apoptosis, the early degradation of cytoskeleton occurs, but organelles degradation is preserved until the later stages. By contrast, autophagy occurs in the absence of chromatin condensation with massive autophagic vacuolization in the cytoplasm.Citation81,Citation113 Thus, the early disruption of organelles occurs, but the cytoskeleton is preserved until the late stage in the process of autophagy. Necroptosis is another morphologically and mechanistically distinct modality of cell death, characterized by mitochondrial lysis and lysosomal membrane rupture. In contrast to apoptosis and autophagy, necroptosis is characterized by a significant inflammatory response.Citation114 Furthermore, unlike apoptosis which occurs in a caspase-dependent manner, necroptosis is caspase-independent. The phosphorylation and formation of RIP1 and RIP3 which only occur in necroptosis are specific indicators of necroptosis.Citation105,Citation115
Several cross-linked pathways in PCD
Although apoptosis, autophagy, and necroptosis have different characteristics, their signaling pathways have certain correlations with each other. For instance, the suppression of apoptosis can trigger autophagy,Citation116 while the absence of the caspase signaling pathway can trigger programmed necrosis; however, a specific inhibitor of programmed necrosis, Nec-1, can revert the death pathway to apoptosis.Citation53 From studies of other diseases, we conclude that the same signaling pathway plays different roles in different PCD processes and that different signaling pathways cross-link with each other to activate the same downstream effector molecules.
Biologists have investigated how apoptosis, autophagy, and necroptosis are interconnected.Citation53 Apoptosis and autophagy are two primary PCD mechanisms that interact with each other and often occur in the same cells.Citation117 Apoptosis and autophagy can share key molecular regulators, such as p53, Akt, JNK, and Beclin-1.Citation118 Apoptosis can suppress autophagy partly via the caspase kinase-mediated cleavage of essential autophagy proteins.Citation119 Autophagy stimulates apoptosis by depleting endogenous inhibitors or attenuates apoptosis by selectively reducing proapoptotic proteins.Citation120 Apoptosis, autophagy, and necroptosis are initiated by a combination of related death receptors and ligands. For example, apoptosis and necrosis appear to be regulated by different forms of caspase-8: a fully processed, heterotetrameric form of caspase-8 induces apoptosis; however, caspase-8 mutants and caspase-8 inhibition activate necroptosis in some cell lines.Citation106,Citation121 Autophagy can trigger or block necroptosis in response to starvation and antigen stimulation in several cell lines.Citation21,Citation122–Citation124
Oxidative stress is involved in the toxic effects of GBMs. The generation of ROS is considered the primary cause of cell death.Citation31,Citation34 The increased ROS generation leads to apoptosis and autophagy with an increase in the expression levels of apoptotic and autophagic effectors, such as caspase-3, caspase-9, Beclin-1, Bax, Bad, and LC3-I/II, after exposure to GBMs.Citation26,Citation27 ROS induces the loss of the mitochondrial membrane that potentially initiates both apoptosis and necrosis.Citation125,Citation126
Numerous signaling pathways comprise an interconnected network of apoptosis, autophagy, and necroptosis, such as the TLRs, p53 signaling pathways, and the Beclin-1 and Bcl-2 interaction. It is known that apoptosis can be induced via TLR-2, TLR-6, and TLR-9 pathways accompanied by the downstream proteins NIK and IκB kinase in GBMs-mediated toxicity.Citation66,Citation127–Citation129 Interestingly, autophagy is elicited through the TLR-4 and TLR-9 pathways accompanied by the downstream adaptor proteins, MyD88, TRIF, and TRAF6, which were activated in RAW 264.7 macrophage cells and CT26 colon cancer cells after exposure to GO.Citation75,Citation77 Moreover, programmed necrosis can also be triggered via the TLR-4 pathway, so TLR-4-deficient BMDMs or a selective TLR-4 inhibitor CLI-095 constrains the effects of GO triggering programmed necrosis.Citation27 p53, a vital regulator of apoptosis, can modulate the MOMP and thereby govern the Bcl-2 family to trigger apoptosis.Citation130 In addition, p53 negatively controls autophagy through the AMPK/mTOR-dependent pathway by inhibiting mTOR via the activation of AMPK or the transactivation of DRAM.Citation131,Citation132 p53 is also involved in the regulation of necrosis.Citation133–Citation136 The functional and structural interactions between Beclin-1 and the antiapoptotic proteins Bcl-2 and Bcl-xL participate to some degree in the cross-linking of apoptosis, autophagy, and necroptosis.Citation98,Citation106,Citation137,Citation138
Collectively, studies have indicated that apoptosis, autophagy, and necroptosis are independent but interconnected by similar pathways and share initiator or effector molecules. A part of the interrelationship of apoptosis, autophagy, and programmed necrosis is shown in .
Figure 4 The interrelationships between programmed cell death. The schematic diagram depicts that apoptosis, autophagy, and necroptosis are independent but interconnected. They are often mediated by similar pathways, such as the TLR signaling pathway, the Beclin-1 and Bcl-2 interaction, and the p53 signaling pathway, and share initiator or effector molecules, comprising a complicated and delicate network.
Abbreviation: GBMs, graphene-based materials.
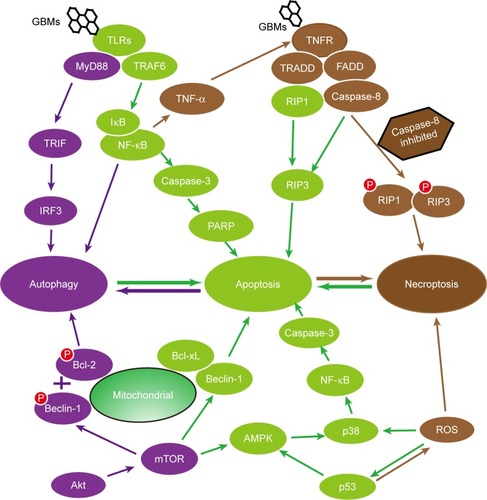
Summary
After summarizing and analyzing hundreds of articles on the toxic study of GBMs, we found that one or two or more kinds of PCDs occur simultaneously or successively in the same cells, and different types of cell deaths emerge simultaneously or successively depending on the physicochemical property, exposure dose, and exposure time of GBMs (these factors are not the focus of this review). The molecular mechanisms of PCD are multifaceted, complex, and interconnected, and the mechanism of PCD induced by GBMs is far from clear and complete. Understanding the mechanisms of PCD and relationships between apoptosis, autophagy, and programmed necrosis may benefit to figure out further the mechanism of GBMs-induced toxicity. The cognition of autophagy and apoptosis was limited by the experimental conditions and experimental methods years ago. The experimental techniques are improving over time, and our cognition of PCD is improving. Some original conclusions may need to be revised, redefined, and changed. Studies of GBMs-induced necroptosis are limited, and hence, more concerns are needed.
The role of autophagy in cell death should be carefully considered. Some researchers believed that autophagy may more likely be a kind of regulation mechanism, related to apoptosis or necrosis pathway components. The instinct of autophagy to remove the damaged proteins and organelles is of great significance for cell survival. Autophagy may make the last desperate efforts for survival in the dying cells.Citation139 Some studies have suggested that autophagy occurs ahead of apoptosis and necroptosis, and autophagy can promote or inhibit apoptosis/necroptosis. The ultimate goal of autophagy restraining apoptosis and necrosis may be to maintain cell survival. Once it cannot maintain survival, autophagy must promote cell death in a reasonable way to help maintain local homeostasis.Citation140,Citation141 From the study on GBMs toxicity, we can identify that the duty of autophagy is to degrade the intracellular proteins and abnormal cellular components, which may ultimately lead to cell death. However, there is no enough evidence to prove that autophagic cell death occurs through autophagosome accumulation, and hence, more work is needed to distinguish the autophagic cell death induced by GBMs.
To clarify the mechanisms of GBM-induced PCD is beyond the scope of this review. However, the insights regarding GBM-induced PCD could be harnessed for more reliable assessments of the health effects of GBMs. The dose and time of occupational exposure of GBMs is huge, and hence, relative studies are not enough. Moreover, since GBMs can induce PCD in cancer cells, the application of GBMs in therapy will benefit to curing tumors/cancers combined with their powerful drug delivery function. However, we need to pay more attention to this study approach.
Abbreviations
BMDMs | = | bone marrow-derived macrophages |
GBMs | = | graphene-based materials |
GNPs | = | graphene nanoplatelets |
GO | = | graphene oxide |
GQDs | = | graphene quantum dots |
MMP | = | mitochondrial membrane potential |
MOMP | = | mitochondrial outer membrane permeabilization |
PCD | = | programmed cell death |
rGO | = | reduced graphene oxide |
Acknowledgments
This review was supported by the National Natural Science Foundation of China (81550011, 51172283, 81400557), Natural Science Foundation of Guangdong Province (2015A030313299), Guangdong Provincial Medical Research Foundation (A2016360), and Science and Technology Planning Project of Guangdong Province (2016A010103017).
Disclosure
The authors report no conflicts of interest in this work.
References
- YangKFengLLiuZStimuli responsive drug delivery systems based on nano-graphene for cancer therapyAdv Drug Deliv Rev2016105Pt B22824127233212
- HuDZhangJGaoGShengZCuiHCaiLIndocyanine green-loaded polydopamine-reduced graphene oxide nanocomposites with amplifying photoacoustic and photothermal effects for cancer theranosticsTheranostics2016671043105227217837
- BattogtokhGKoYTGraphene oxide-incorporated pH-responsive folate-albumin-photosensitizer nanocomplex as image-guided dual therapeuticsJ Control Release2016234102027164545
- TeixeiraSRLloydCYaoSPolyaniline-graphene based α-amylase biosensor with a linear dynamic range in excess of 6 orders of magnitudeBiosens Bioelectron20168539540227196256
- ShiraiAHenaresTGSueyoshiKEndoTHisamotoHFast and single-step immunoassay based on fluorescence quenching within a square glass capillary immobilizing graphene oxide-antibody conjugate and fluorescently labelled antibodyAnalyst2016141113389339427127806
- LinJChenXHuangPGraphene-based nanomaterials for bioimagingAdv Drug Deliv Rev2016105Pt B24225427233213
- WengXNeethirajanSA microfluidic biosensor using graphene oxide and aptamer-functionalized quantum dots for peanut allergen detectionBiosens Bioelectron20168564965627240012
- Ali TahirAUllahHSudhagarPAsri Mat TeridiMDevadossASundaramSThe application of graphene and its derivatives to energy conversion, storage, and environmental and biosensing devicesChem Rec20161631591163427230414
- McCallionCBurthemJRees-UnwinKGolovanovAPluenAGraphene in therapeutics delivery: problems, solutions and future opportunitiesEur J Pharm Biopharm201610423525027113141
- LiuHLiTLiuYQinGWangXChenTGlucose-reduced graphene oxide with excellent biocompatibility and photothermal efficiency as well as drug loadingNanoscale Res Lett201611121127094825
- SanchezVCJachakAHurtRHKaneABBiological interactions of graphene-family nanomaterials: an interdisciplinary reviewChem Res Toxicol2012251153421954945
- GurunathanSKimJHSynthesis, toxicity, biocompatibility, and biomedical applications of graphene and graphene-related materialsInt J Nanomedicine2016111927194527226713
- OrecchioniMMénard-MoyonCDeloguLGBiancoAGraphene and the immune system: challenges and potentialityAdv Drug Deliv Rev2016105Pt B16317527235665
- LalwaniGD’AgatiMKhanAMSitharamanBToxicology of graphene-based nanomaterialsAdv Drug Deliv Rev2016105Pt B10914427154267
- ZhangBWeiPZhouZWeiTInteractions of graphene with mammalian cells: molecular mechanisms and biomedical insightsAdv Drug Deliv Rev2016105Pt B14516227569910
- HorváthLMagrezABurghardMKernKForróLSchwallerBEvaluation of the toxicity of graphene derivatives on cells of the lung luminal surfaceCarbon201364114560
- ChongYMaYShenHThe in vitro and in vivo toxicity of graphene quantum dotsBiomaterials201435195041504824685264
- SinghZApplications and toxicity of graphene family nanomaterials and their compositesNanotechnol Sci Appl20169152827051278
- PatlollaAKRandolphJKumariSATchounwouPBToxicity evaluation of graphene oxide in kidneys of Sprague-Dawley ratsInt J Environ Res Public Health201613438027043588
- LewinskiNColvinVDrezekRCytotoxicity of nanoparticlesSmall200841264918165959
- GalluzziLVitaleIAbramsJMMolecular definitions of cell death subroutines: recommendations of the Nomenclature Committee on Cell Death 2012Cell Death Differ201219110712021760595
- AndónFTFadeelBProgrammed cell death: molecular mechanisms and implications for safety assessment of nanomaterialsAcc Chem Res201346373374222720979
- LiuJFengXWeiLChenLSongBShaoLThe toxicology of ion-shedding zinc oxide nanoparticlesCrit Rev Toxicol201646434838426963861
- SternSTJohnsonDNRole for nanomaterial-autophagy interaction in neurodegenerative diseaseAutophagy2008481097110018927490
- BhattacharyaSMishraSGuptaPLiquid phase collagen modified graphene that induces apoptosisRSC Adv201554444744457
- QinYZhouZWPanSTGraphene quantum dots induce apoptosis, autophagy, and inflammatory response via p38 mitogen-activated protein kinase and nuclear factor-kappaB mediated signaling pathways in activated THP-1 macrophagesToxicology2015327627625446327
- QuGLiuSZhangSGraphene oxide induces toll-like receptor 4 (TLR4)-dependent necrosis in macrophagesACS Nano2013775732574523734789
- OuLSongBLiangHToxicity of graphene-family nanoparticles: a general review of the origins and mechanismsPart Fibre Toxicol20161315727799056
- YangKLiYTanXPengRLiuZBehavior and toxicity of graphene and its functionalized derivatives in biological systemsSmall201399–101492150322987582
- SeabraABPaulaAJde LimaRAlvesOLDuránNNanotoxicity of graphene and graphene oxideChem Res Toxicol201427215916824422439
- JaroszASkodaMDudekISzukiewiczDOxidative stress and mitochondrial activation as the main mechanisms underlying graphene toxicity against human cancer cellsOxid Med Cell Longev20162016585103526649139
- BialikSZalckvarEBerYRubinsteinADKimchiASystems biology analysis of programmed cell deathTrends Biochem Sci2010351055656420537543
- TanMLOoiJPIsmailNMoadAIMuhammadTSProgrammed cell death pathways and current antitumor targetsPharm Res20092671547156019407932
- ChatterjeeNEomHJChoiJA systems toxicology approach to the surface functionality control of graphene-cell interactionsBiomaterials20143541109112724211078
- ZhangWYanLLiMDeciphering the underlying mechanisms of oxidation-state dependent cytotoxicity of graphene oxide on mammalian cellsToxicol Lett20152372617126047786
- HatamieSAkhavanOSadrnezhaadSKAhadianMMShirolkarMMWangHQCurcumin-reduced graphene oxide sheets and their effects on human breast cancer cellsMater Sci Eng C Mater Biol Appl20155548248926117780
- ZhangYAliSFDervishiECytotoxicity effects of graphene and single-wall carbon nanotubes in neural phaeochromocytoma-derived PC12 cellsACS Nano2010463181318620481456
- MbehDAAkhavanOJavanbakhtTMahmoudiMYahiaLHCytotoxicity of protein corona-graphene oxide nanoribbons on human epithelial cellsAppl Surf Sci2014320596601
- DingZZhangZMaHChenYIn vitro hemocompatibility and toxic mechanism of graphene oxide on human peripheral blood T lymphocytes and serum albuminACS Appl Mater Interfaces2014622197971980725371999
- OrecchioniMBedognettiDSgarrellaFMarincolaFMBiancoADeloguLGImpact of carbon nanotubes and graphene on immune cellsJ Transl Med20141213824885781
- JeongJChoHJChoiMWangSLChungBHLeeJSIn vivo toxicity assessment of angiogenesis and the live distribution of nano-graphene oxide and its PEGylated derivatives using the developing zebrafish embryoCarbon201593431440
- ZhaoYWuQWangDAn epigenetic signal encoded protection mechanism is activated by graphene oxide to inhibit its induced reproductive toxicity in Caenorhabditis elegansBiomaterials201679152426686978
- RenCHuXLiXZhouQUltra-trace graphene oxide in a water environment triggers Parkinson’s disease-like symptoms and metabolic disturbance in zebrafish larvaeBiomaterials201693839427085073
- ChanKTMengFYLiQCucurbitacin B induces apoptosis and S phase cell cycle arrest in BEL-7402 human hepatocellular carcinoma cells and is effective via oral administrationCancer Lett2010294111812420153103
- GurunathanSHanJWKimESParkJHKimJHReduction of graphene oxide by resveratrol: a novel and simple biological method for the synthesis of an effective anticancer nanotherapeutic moleculeInt J Nanomedicine2015102951296925931821
- JaworskiSSawoszEKutwinMIn vitro and in vivo effects of graphene oxide and reduced graphene oxide on glioblastomaInt J Nanomedicine2015101585159625759581
- MarkovicZMRisticBZArsikinKMGraphene quantum dots as autophagy-inducing photodynamic agentsBiomaterials201233297084709222795854
- HabibaKEncarnacion-RosadoJGarcia-PabonKImproving cytotoxicity against cancer cells by chemo-photodynamic combined modalities using silver-graphene quantum dots nanocompositesInt J Nanomedicine20161110711926766909
- NishidaKYamaguchiOOtsuKCrosstalk between autophagy and apoptosis in heart diseaseCirc Res2008103434335118703786
- SaptarshiSRDuschlALopataALBiological reactivity of zinc oxide nanoparticles with mammalian test systems: an overviewNanomedicine (Lond)201510132075209226135328
- EumKHLeeMCrosstalk between autophagy and apoptosis in the regulation of paclitaxel-induced cell death in v-Ha-ras-transformed fibroblastsMol Cell Biochem20113481–2616821069434
- MeierPVousdenKHLucifer’s labyrinth – ten years of path finding in cell deathMol Cell200728574675418082600
- OuyangLShiZZhaoSProgrammed cell death pathways in cancer: a review of apoptosis, autophagy and programmed necrosisCell Prolif201245648749823030059
- RepnikUStokaVTurkVTurkBLysosomes and lysosomal cathepsins in cell deathBiochim Biophys Acta201218241223321914490
- GhobrialIMWitzigTEAdjeiAATargeting apoptosis pathways in cancer therapyCA Cancer J Clin200555317819415890640
- ChangHYNishitohHYangXIchijoHBaltimoreDActivation of apoptosis signal-regulating kinase 1 (ASK1) by the adapter protein DaxxScience19982815384186018639743501
- SasidharanASwaroopSChandranPNairSKoyakuttyMCellular and molecular mechanistic insight into the DNA-damaging potential of few-layer graphene in human primary endothelial cellsNanomedicine20161251347135526970024
- ChoiYJKimEHanJKimJHGurunathanSA novel biomolecule-mediated reduction of graphene oxide: a multifunctional anti-cancer agentMolecules201621337526999102
- KroemerGGalluzziLBrennerCMitochondrial membrane permeabilization in cell deathPhysiol Rev20078719916317237344
- VauxDLApoptogenic factors released from mitochondriaBiochim Biophys Acta20111813454655020713095
- GhavamiSHashemiMAndeSRApoptosis and cancer: mutations within caspase genesJ Med Genet200946849751019505876
- LiYLiuYFuYThe triggering of apoptosis in macrophages by pristine graphene through the MAPK and TGF-beta signaling pathwaysBiomaterials201233240241122019121
- MarkovicZMHarhaji-TrajkovicLMTodorovic-MarkovicBMIn vitro comparison of the photothermal anticancer activity of graphene nanoparticles and carbon nanotubesBiomaterials20113241121112921071083
- PintoAMGonçalvesICMagalhãesFDGraphene-based materials biocompatibility: a reviewColloids Surf B Biointerfaces201311118820223810824
- RussierJTreossiEScarsiAEvidencing the mask effect of graphene oxide: a comparative study on primary human and murine phagocytic cellsNanoscale2013522112341124724084792
- ReshmaSCSyamaSMohananPVNano-biointeractions of PEGylated and bare reduced graphene oxide on lung alveolar epithelial cells: a comparative in vitro studyColloids Surf B Biointerfaces201614010411626741270
- TorresMFormanHJRedox signaling and the MAP kinase pathwaysBiofactors2003171–428729612897450
- KyriakisJMAvruchJMammalian MAPK signal transduction pathways activated by stress and inflammation: a 10-year updatePhysiol Rev201292268973722535895
- ZhouSWangYZhuJJSimultaneous detection of tumor cell apoptosis regulators Bcl-2 and Bax through a dual-signal-marked electrochemical immunosensorACS Appl Mater Interfaces20168127674768226946947
- DudekISkodaMJaroszASzukiewiczDThe molecular influence of graphene and graphene oxide on the immune system under in vitro and in vivo conditionsArch Immunol Ther Exp (Warsz)201664319521526502273
- RameshSWildeyGHowePHTransforming growth factor beta (TGFbeta)-induced apoptosis: the rise & fall of BimCell Cycle200981111719106608
- LeightJLWozniakMAChenSLynchMLChenCSMatrix rigidity regulates a switch between TGF-β1-induced apoptosis and epithelial–mesenchymal transitionMol Biol Cell201223578179122238361
- FrancoDLMainezJVegaSSnail1 suppresses TGF-beta-induced apoptosis and is sufficient to trigger EMT in hepatocytesJ Cell Sci2010123Pt 203467347720930141
- ZhuBZhaiJProhibitin regulates TGF-beta induced apoptosis as a downstream effector of Smad-dependent and -independent signalingProstate2010701172619725029
- ChenGYYangHJLuCHSimultaneous induction of autophagy and toll-like receptor signaling pathways by graphene oxideBiomaterials201233276559656922704844
- LiuYWangXWangJGraphene oxide attenuates the cytotoxicity and mutagenicity of PCB 52 via activation of genuine autophagyEnviron Sci Technol20165063154316426876502
- ChenGYChenCLTuanHYGraphene oxide triggers toll-like receptors/autophagy responses in vitro and inhibits tumor growth in vivoAdv Healthc Mater2014391486149524652749
- ChenGYMengCLLinKCGraphene oxide as a chemosensitizer: diverted autophagic flux, enhanced nuclear import, elevated necrosis and improved antitumor effectsBiomaterials201540122225498801
- ParkEJLeeGHHanBSToxic response of graphene nanoplatelets in vivo and in vitroArch Toxicol20158991557156824980260
- ShimizuSYoshidaTTsujiokaMArakawaSAutophagic cell death and cancerInt J Mol Sci20141523145315324566140
- SternSTAdiseshaiahPPCristRMAutophagy and lysosomal dysfunction as emerging mechanisms of nanomaterial toxicityPart Fibre Toxicol201293217222
- LinCTsaiSCTsengMTAKT serine/threonine protein kinase modulates baicalin-triggered autophagy in human bladder cancer T24 cellsInt J Oncol2013423993100023354080
- BrugarolasJLeiKHurleyRLRegulation of mTOR function in response to hypoxia by REDD1 and the TSC1/TSC2 tumor suppressor complexGenes Dev200418232893290415545625
- ArshamAMNeufeldTPThinking globally and acting locally with TORCurr Opin Cell Biol2007186589597
- BudanovAKarinMp53 target genes sestrin1 and sestrin2 connect genotoxic stress and mTOR signalingCell2008134345146018692468
- ChanEYLongattiAMcknightNCToozeSAKinase-inactivated ULK proteins inhibit autophagy via their conserved C-terminal domains using an Atg13-independent mechanismMol Cell Biol200929115717118936157
- MizushimaNYoshimoriTLevineBMethods in mammalian autophagy researchCell2010140331332620144757
- PatelASLinLGeyerAAutophagy in idiopathic pulmonary fibrosisPLoS One201277L56L69
- MaXWuYJinSGold nanoparticles induce autophagosome accumulation through size-dependent nanoparticle uptake and lysosome impairmentACS Nano20115118629863921974862
- IchimuraYKumanomidouTSouYSStructural basis for sorting mechanism of p62 in selective autophagyJ Biol Chem200828333228472285718524774
- WanBWangZXLvQYSingle-walled carbon nanotubes and graphene oxides induce autophagosome accumulation and lysosome impairment in primarily cultured murine peritoneal macrophagesToxicol Lett2013221211812723769962
- Johnson-LylesDNPeifleyKLockettSFullerenol cytotoxicity in kidney cells is associated with cytoskeleton disruption, autophagic vacuole accumulation, and mitochondrial dysfunctionToxicol Appl Pharmacol2010248324925820713077
- SahaniMHItakuraEMizushimaNExpression of the autophagy substrate SQSTM1/p62 is restored during prolonged starvation depending on transcriptional upregulation and autophagy-derived amino acidsAutophagy201410343144124394643
- PankivSClausenTHLamarkTp62/SQSTM1 binds directly to Atg8/LC3 to facilitate degradation of ubiquitinated protein aggregates by autophagyJ Biol Chem200728233241312414517580304
- RubinszteinDCCuervoAMRavikumarBIn search of an “autophagomometer”Autophagy20095558558919411822
- RoyAKolattukudyPEMonocyte chemotactic protein-induced protein (MCPIP) promotes inflammatory angiogenesis via sequential induction of oxidative stress, endoplasmic reticulum stress and autophagyCell Signal201224112123213122820500
- ThomasRLGustafssonABMitochondrial autophagy – an essential quality control mechanism for myocardial homeostasisCirc J201377102449245423985961
- PattingreSTassaAQuXBcl-2 antiapoptotic proteins inhibit Beclin 1-dependent autophagyCell2005122692793916179260
- StrappazzonFVietri-RudanMCampelloSMitochondrial BCL-2 inhibits AMBRA1-induced autophagyEMBO J20113071195120821358617
- AnandPKTaitSWLamkanfiMTLR2 and RIP2 pathways mediate autophagy of Listeria monocytogenes via extracellular signal-regulated kinase (ERK) activationJ Biol Chem201128650429814299122033934
- ShiCSKehrlJHTRAF6 and A20 regulate lysine 63-linked ubiquitination of Beclin-1 to control TLR4-induced autophagySci Signal20103123ra4220501938
- DelgadoMAElmaouedRADavisASKyeiGDereticVToll-like receptors control autophagyEMBO J20082771110112118337753
- XuCLiuJHsuLCLuoYXiangRChuangTHFunctional interaction of heat shock protein 90 and Beclin 1 modulates Toll-like receptor-mediated autophagyFASEB J20112582700271021543763
- WebberJLRegulation of autophagy by p38alpha MAPKAutophagy20106229229320087063
- ChoYSChallaSMoquinDPhosphorylation-driven assembly of the RIP1-RIP3 complex regulates programmed necrosis and virus-induced inflammationCell200913761112112319524513
- HanJZhongCQZhangDWProgrammed necrosis: backup to and competitor with apoptosis in the immune systemNat Immunol201112121143114922089220
- DegterevAHuangZBoyceMChemical inhibitor of nonapoptotic cell death with therapeutic potential for ischemic brain injuryNat Chem Biol20139311211923222885
- WuWLiuPLiJNecroptosis: an emerging form of programmed cell deathCrit Rev Oncol Hematol201282324925821962882
- MccallKGenetic control of necrosis – another type of programmed cell deathCurr Opin Cell Biol201022688288820889324
- GalluzziLKroemerGNecroptosis: a specialized pathway of programmed necrosisCell200813571161116319109884
- GolsteinPKroemerGCell death by necrosis: towards a molecular definitionTrends Biochem Sci2007321374317141506
- PatschanSGoligorskyMSAutophagy: the missing link between non-enzymatically glycated proteins inducing apoptosis and premature senescence of endothelial cells?Autophagy20084452152318367870
- LiJJHartonoDOngCNBayBHYungLYAutophagy and oxidative stress associated with gold nanoparticlesBiomaterials201031235996600320466420
- KroemerGGalluzziLVandenabeelePClassification of cell death: recommendations of the Nomenclature Committee on Cell Death 2009Cell Death Differ200916131118846107
- HeSWangLMiaoLReceptor interacting protein kinase-3 determines cellular necrotic response to TNF-alphaCell200913761100111119524512
- AmelioIMelinoGKnightRACell death pathology: cross-talk with autophagy and its clinical implicationsBiochem Biophys Res Commun2011414227728121963447
- MaiuriMCZalckvarEKimchiAKroemerGSelf-eating and self-killing: crosstalk between autophagy and apoptosisNat Rev Mol Cell Biol20078974175217717517
- FimiaGMPiacentiniMRegulation of autophagy in mammals and its interplay with apoptosisCell Mol Life Sci201067101581158820165902
- MariñoGNiso-SantanoMBaehreckeEHKroemerGSelf-consumption: the interplay of autophagy and apoptosisNat Rev Mol Cell Biol2014152819424401948
- YouleRJNarendraDPMechanisms of mitophagyNat Rev Mol Cell Biol201112191421179058
- PeterMEProgrammed cell death: apoptosis meets necrosisNature2011471733831031221412328
- NikoletopoulouVMarkakiMPalikarasKTavernarakisNCrosstalk between apoptosis, necrosis and autophagyBiochim Biophys Acta20131833123448345923770045
- BonapaceLBornhauserBCSchmitzMInduction of autophagy-dependent necroptosis is required for childhood acute lymphoblastic leukemia cells to overcome glucocorticoid resistanceJ Clin Invest201012041310132320200450
- FarkasTDaugaardMJäätteläMIdentification of small molecule inhibitors of phosphatidylinositol 3-kinase and autophagyJ Biol Chem201128645389043891221930714
- LevineBYuanJAutophagy in cell death: an innocent convict?J Clin Invest2005115102679268816200202
- KimJSHeLLemastersJJMitochondrial permeability transition: a common pathway to necrosis and apoptosisBiochem Biophys Res Commun2003304346347012729580
- KimASChaeCHKimJChoiJYKimSGBăciuţGSilver nanoparticles induce apoptosis through the Toll-like receptor 2 pathwayOral Surg Oral Med Oral Pathol Oral Radiol2012113678979822668707
- ChakrabortyDCMukherjeeGBanerjeePBanerjeeKKBiswasTHemolysin induces Toll-like receptor (TLR) independent apoptosis but multiple TLR associated parallel activation of macrophagesJ Biol Chem201128640345423455121846723
- LimEJParkDWLeeJGToll-like receptor 9-mediated inhibition of apoptosis occurs through suppression of FoxO3a activity and induction of FLIP expressionExp Mol Med2010421071272020739833
- VasevaAMarchenkoNJiKTsirkaSHolzmannSMollUMp53 opens the mitochondrial permeability transition pore to trigger necrosisCell201214971536154822726440
- FengZZhangHLevineAJJinSThe coordinate regulation of the p53 and mTOR pathways in cellsProc Natl Acad Sci U S A2005102238204820915928081
- TasdemirEMaiuriMCGalluzziLRegulation of autophagy by cytoplasmic p53Nat Cell Biol200810667668718454141
- BaumannKCell death: multitasking p53 promotes necrosisNat Rev Mol Cell Biol2012138480481
- GreaySJIrelandDJKissickHTInduction of necrosis and cell cycle arrest in murine cancer cell lines by Melaleuca alternifolia (tea tree) oil and terpinen-4-olCancer Chemother Pharmacol201065587788819680653
- PalanisamyAPChengGSutterAGMitochondrial uncoupling protein 2 induces cell cycle arrest and necrotic cell deathMetab Syndr Relat Disord201412213214224320727
- FrankeSSommerMRüsterCAdvanced glycation end products induce cell cycle arrest and proinflammatory changes in osteoarthritic fibroblast-like synovial cellsArthritis Res Ther2009115R13619735566
- OnoKWangXKimSOArmstrongLCBornsteinPHanJMetaxin deficiency alters mitochondrial membrane permeability and leads to resistance to TNF-induced cell killingProtein Cell20101216117321088703
- BorkanSCThe role of BCL-2 family members in acute kidney injurySemin Nephrol201636323725027339388
- ColellARicciJETaitSGAPDH and autophagy preserve survival after apoptotic cytochrome c release in the absence of caspase activationCell2007129598399717540177
- Djavaheri-MergnyMAmelottiMMathieuJNF-kappaB activation represses tumor necrosis factor-alpha-induced autophagyJ Biol Chem200628141303733038216857678
- SasnauskieneAKadziauskasJVezelyteNJonusieneVKirvelieneVDamage targeted to the mitochondrial interior induces autophagy, cell cycle arrest and, only at high doses, apoptosisAutophagy20095574374419571672