Abstract
Angiogenesis plays an important role in tumor development and metastasis, and many cancer cells upregulate VEGF expression to promote angiogenesis. Silencing VEGF expression by RNA interference is expected to be a promising strategy to suppress the tumor growth. However, low transfection efficiency and instability are the main barriers for small interfering RNA (siRNA) delivery. In this study, we developed polycation liposome-encapsulated calcium phosphate nanoparticles (PLCP) for siRNA delivery in vivo. VEGF expression silencing effect in MCF-7 cells was investigated by real-time quantitative polymerase chain reaction and Western blot assay. VEGF siRNA mediated by PLCP can reduce 60%–80% VEGF expression in vitro, which was significantly higher than that mediated by Lipofectamine 2000. Furthermore, significant tumor growth and angiogenesis inhibition were observed in MCF-7 xenografts mice when treated with PLCP/VEGF siRNA or combined with doxorubicin. In conclusion, the combination of silencing VEGF expression and chemotherapeutics would be a potential treatment for cancer therapy.
Introduction
Angiogenesis, one of the major hallmarks of cancer, plays an important role in vasculature establishment for growth and hematogenous metastasis.Citation1 In angiogenesis, there is a change from normal recruitment of endothelial progenitor cells to pathologic sprouting of new vessels.Citation2 Specified cytokines and growth factors secreted by tumor cells and stromal cells stimulate proliferation of vascular endothelial cell.Citation3 Among these factors, VEGF, upregulated by many cancer cells, is known as closely associated with increased aggressiveness and metastasis.Citation4 Clinical studies have indicated that VEGF can be targeted to reduce angiogenesis, and VEGF inhibition has been shown to promote survival and slow tumor growth in ovarian, cervical, colorectal, renal, lung, and breast cancers.Citation5
RNA interference, a highly conserved gene silencing mechanism, is one of the most widely used procedures for gene targeting in cutting edge technology and has potential for use in clinical therapy.Citation6 Many diseases are caused by the inappropriate expression of disease-related genes, including cancer, obesity, heart disease, and diabetes.Citation7,Citation8 Small interfering RNA (siRNA) treatments represent advantages over conventional ones because of their powerful and highly specific gene silencing ability.Citation9 Synthetic siRNA mediates target gene silencing by promoting mRNA degradation in the cytoplasm. However, low transfection efficiency and poor stability in physiological conditions are the main obstacles for siRNA delivery in clinical application.
The development of safe and effective siRNA delivery system would enhance the clinical application of gene silencing siRNA in cancer therapy. Several approaches have been used to overcome the obstacle of siRNA delivery, including complexation, entrapment, and conjugation, most of which were based on a lipidic or polymeric scaffold. Cationic lipid-based vectors have several advantages including stability and favorable cellular internalization, while toxicity and protection of siRNA can be major challenges.Citation10,Citation11 Various polymeric nanoparticles have also been explored for siRNA delivery, such as polyethylenimine (PEI),Citation12 chitosan,Citation13 and dendrimers.Citation14 Polymer-based complexes have been shown to protect siRNA from degradation, to mediate cellular uptake, and to efficiently promote lysosomal protection and escape into the cytoplasm. However, their strongly positive charge promotes the colloidal instability in vivo and leads to difficult dissociation of siRNA from complexes. Calcium phosphate (CaP) has been developed for the delivery of DNA since 1970s and was used for siRNA delivery in recent years. CaP shows negligible cytotoxicity for siRNA delivery due to its inherent biocompatibility and biodegradability. In addition, CaP can be dissolved in the acidic endosome to help siRNA release into the cytosol- and silence-specific genes.Citation15
In our previous study, we have developed a siRNA delivery system, polycation liposome-encapsulated calcium phosphate nanoparticles (PLCP), using CaP/siRNA nanoparticles combined with polycation liposomes (PCLs).Citation16 PCL was developed to improve the ability of endosomal escape by combining the protonation of PEI and the membrane destabilization of 1,2-dioleoyl-sn-glycero-3-phosphoethanolamine (DOPE), which led to high transfection efficiency. When encapsulating CaP, PCL could control the growth of CaP precipitates, which would lead to large aggregation and reduce stability. Anti-green fluorescent protein (GFP) siRNA transferred by PLCP to MCF-7 cells that stably express GFP represented high gene silencing efficiency with low cytotoxicity. In this study, we further investigated the VEGF siRNA delivery efficiency of PLCP both in vitro and in MCF-7 xenograft mice. Tumor inhibition ratio and angiogenesis status were assessed. In addition, synergetic tumor inhibition effect combined with doxorubicin (DOX) was also studied.
Materials and methods
Materials
Pluronic F68 and DOPE were purchased from Sigma-Aldrich (St Louis, MO, USA). PEI-cholesterol (PEI-Chol) was synthesized as previously reported.Citation17 Dulbecco’s Modified Eagle’s Medium (DMEM), trypsin, fetal bovine serum (FBS), and Lipofectamine 2000 were purchased from Thermo Fisher Scientific (Waltham, CA, USA). DOX hydrochloride was kindly provided by Hisun Pharmaceutical Co. Ltd. (Taizhou, Zhejiang, China). Random siRNA and VEGF siRNA were synthesized, modified, and purified by 100 Biotech Company (Hangzhou, Zhejiang, China). All other chemical reagents were of analytical grade from Sinopharm Chemical Reagent Company (Shanghai, China).
Preparation of PLCP
CaP/siRNA nanoparticles were prepared as previously reported.Citation16 Briefly, CaCl2 (75 mM), Pluronic F68 (1%), and siRNA solution (0.1 mg/mL) were mixed and incubated for 10 min. Na2HPO4 (6 mM), Pluronic F68 (1%), and sodium citrate (24 mM) were mixed and added to an equal volume of abovementioned solution dropwise under stirring for 30 min. The mass ratios of CaCl2 and siRNA were studied by gel retardation assays. CaP/siRNA nanoparticles (15 μL) with different mass ratios (ranging from 1 to 25) were loaded onto a 0.5% agarose gel. Free siRNA was used as a control. Electrophoresis was performed at 90 V for 60 min. The gel was visualized by ethidium bromide staining.
PCLs were prepared by film dispersion methodCitation18 with a lipid mixture of PEI-Chol and DOPE (molar ratio 1:1), and then hydrated with CaP nanoparticle solution followed by sonication and filtration through 0.22 μm filter. PLCP were constructed of CaP/siRNA nanoparticles as inner aqueous phase and PCL as outer lipid layer. The N/P ratio (1 mol PEI-Chol per mole siRNA phosphate) of PCL and CaP/siRNA was 20. Particle size was measured by laser diffraction spectrometry (Zetasizer 3000HS, Malvern Instruments, Malvern, UK). Morphologies of PLCP were observed by transmission electron microscopy (TEM, JEM-1200EX, JEOL Ltd., Tokyo, Japan).
Cell culture and gene silencing assay in vitro
Human breast adenocarcinoma cell lines (MCF-7) were obtained from the Institute of Biochemistry and Cell Biology, Shanghai Institutes for Biological Sciences (Shanghai, China). MCF-7 cells were cultured in DMEM containing 10% FBS at 37°C in 5% CO2. Cells were regularly passaged and reseeded 24 h before gene silencing experiments. In order to evaluate the gene silencing ability, three different VEGF siRNA sequences were synthesized (), which were mediated by PLCP and Lipofectamine 2000. For gene silencing experiments, 1.5×104 MCF-7 cells were seeded with 2 mL of DMEM containing 10% FBS on 12-well plates. The next day, VEGF siRNA (1 μg siRNA/well) was transfected using Lipofectamine 2000 (5 μL) and PLCP (N/P =20) into cells for 5 h, and then the medium was replaced with fresh medium. Cells were harvested, and the RNA was extracted using Trizol reagent (Thermo Fisher Scientific), reverse-transcribed to cDNA, and amplified by polymerase chain reaction (PCR). The following PCR conditions were used: initial denaturation at 95°C for 3 min, then 95°C for 12 s, 62°C for 40 s for 40 cycles. The related VEGF mRNA expression was determined after 24 h by real-time quantitative PCR (RT-qPCR), using the 2−ΔΔCT quantitation method with hGAPDH (human glyceraldehyde-3-phosphate dehydrogenase) as a housekeeping gene. The primer sequences of human VEGFA (hVEGFA) and hGAPDH for amplifying are shown in .
Table 1 Sequence of siRNA
Table 2 Primer sequences of hVEGFA and hGAPDH
The total protein was isolated 48 h posttransfection, and the expression level of VEGF was determined by Western blot assay. Cell extracts were prepared in lysis buffer, then transferred to sodium dodecyl sulfate–polyacrylamide gel (10%) electrophoresis (SDS-PAGE), and the resolved proteins were transferred electrophoretically to polyvinylidene fluoride membranes (EMD Millipore, Billerica, MA, USA). The hGAPDH was used as loading control. Protein levels were quantified using Gel-Pro Analyzer 4.0 software and normalized to the levels of hGAPDH.
Tumor and angiogenesis inhibition in vivo
The animal experiment was in accordance with Zhejiang University guidelines for the welfare and ethics of the experimental animals, and the protocol was reviewed and approved by the Institution Animal Ethics Committee (The Second Affiliated Hospital of Zhejiang University, School of Medicine). BALB/C-nu female mice (4–6 weeks) were obtained from Laboratory Animals Central of Academy of Military Medical Sciences and were maintained under a 12 h light/12 h dark photoperiod for in vivo tumor inhibition experiments. Tumor xenografts were generated by injecting 1×106 MCF-7 cells in 0.2 mL DMEM subcutaneously into right forelimb armpit. When the tumors reached a size of ~100 mm3 (Day 10 after inoculation), the mice were randomized into different treatment groups (n=5). Saline, PLCP, and PLCP with either the scrambled siRNA or the specific VEGF siRNA were intratumorally injected twice a week (0.5 nM siRNA, 100 μL). DOX was peritoneally injected once a week with a dosage of 2 mg/kg. For the combination therapy (PLCP/VEGF siRNA and DOX), the dosage of DOX was reduced to 1.2 mg/kg.
The body weight changes and physiological state of mice were recorded during the whole therapeutic period. Tumor volume was measured every 3 days after first administration and calculated by the formula: V = πab2/6, where a is the maximum and b is the minimum diameter of the tumor. At the end of the animal study (Day 28 after inoculation) or when tumor volume exceeded 1,000 mm3, mice were sacrificed and the tumors were excised and weighed. The tumor inhibition rate was calculated by the following formula: tumor inhibition ratio = (mean tumor weight(Control) − mean tumor weight(Treatment))/mean tumor weight(Control) ×100%. The tumor tissue samples were fixed, sectioned, and stained with anti-CD34 to detect vascular endothelial cells. The areas of increased vascularity were chosen at 100× magnification, and the microvessel density (MVD) was calculated at 400× magnification (five views) according to the procedure reported by Weidner et al.Citation19 In addition, VEGF and hypoxia-inducible factor-1α (HIF-1α) protein expression of tumor tissues were also assessed by immunohistochemistry.
The standard operation procedure was performed. The tumor tissues were fixed in 4% formaldehyde and embedded in paraffin. The paraffin section (4 μm) was hydrated with xylene, 100% ethanol, 90% ethanol, 80% ethanol, 70% ethanol, and distilled water. Then, the sections were incubated with primary antibodies against CD34 (1:50), VEGF (1:200), or HIF-1α (1:500) overnight at 4°C individually. After incubation with secondary antibodies at room temperature for 15 min, the expression was visualized by 3,3′-diaminobenzidine tetrahydrochloride staining. Finally, the sections were counterstained with hematoxylin and dehydrated and observed with a microscope.
Statistical analysis
The data were presented as mean ± SD, and the statistical analysis was performed using Student’s t-test for two groups or one-way analysis of variance for multiple groups. A P-value of 0.05 or less was considered significant.
Results
Characteristics of PLCP
The mean particle sizes of CaP nanoparticles, PCL, and PLCP were 30.6±2.5 nm polydispersity index (PDI 0.206), 234±22.6 nm (PDI 0.252), and 207±17.5 nm (PDI 0.236), respectively. The size distribution is shown in Figure S1. Morphological characterization of PLCP was carried out using TEM. As shown in , CaP nanoparticles and PCLs were spherical in shape with smooth surface and uniform particle size correlating with the results of laser diffraction spectrometry. PLCP showed a core-membrane structure with CaP nanoparticles in inner aqueous phase. Due to flexibility of lipid bilayer, PLCP could encapsulate multiple CaP nanoparticles.
Figure 1 Images of transmission electron microscopy and gel retardation assays.
Notes: (A) Transmission electron microscopy analysis of PCL (a), CaP nanoparticles (b), and PLCP (c). (B) Gel retardation assays of CaP nanoparticles with various mass ratios of CaCl2 and siRNA.
Abbreviations: CaP, calcium phosphate; PCL, polycation liposome; PLCP, polycation liposome-encapsulated calcium phosphate nanoparticles; siRNA, small interfering RNA.
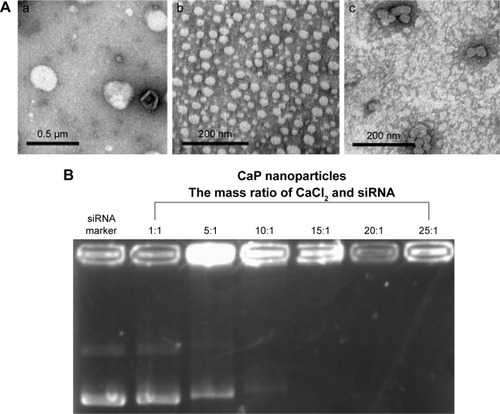
CaP nanoparticles can bind siRNA by electrostatic interactions. With the increasing mass ratio of CaCl2 and siRNA, the amount of unbound siRNA decreased as shown in gel retardation assays results (). The unbound siRNA strap disappeared when the mass ratio was 15, which suggests that CaP nanoparticles can bind siRNA efficiently at that mass ratio. In this study, we used CaP nanoparticles with the mass ratio of 25.
VEGF inhibition in vitro
The efficacy of PLCP-mediated siRNA delivery was investigated in MCF-7 cells. We constructed three different VEGF siRNA sequences targeting VEGF mRNA. PLCP, representing significantly higher VEGF mRNA silencing efficiency than Lipofectamine 2000 after 24 h transfection (). The lowest VEGF mRNA expression reached 19% when VEGF siRNA was mediated by PLCP, while 53% VEGF mRNA expression was observed when it was mediated by Lipofectamine 2000. VEGF siRNA 2 showed the highest gene silencing efficacy, and little biological efficacy was found when cells were treated with random siRNA and blank carriers. The results indicated that gene silencing efficiency might be correlated with sequence specificity. Thus, VEGF siRNA 2 was chosen for the following Western blot determination. The amplification curves for RT-qPCR are shown in Figure S2.
Figure 2 Gene silencing efficiency of VEGF-siRNA in MCF-7 cells.
Notes: (A) Relative expression levels of VEGF mRNA in MCF-7 cells determined by RT-qPCR after 24 h transfection. *P<0.05 (PLCP/VEGF-siRNA 1 vs Lipofectamine 2000/VEGF-siRNA 3) and **P<0.01 (PLCP/VEGF-siRNA 2 vs Lipofectamine 2000/VEGF-siRNA 3). (B) VEGF protein expression relative to hGAPDH in MCF-7 cells determined by Western blot after 48 h transfection. (C) Semiquantitative gray scale results of Western blot. *P<0.05 and **P<0.01. 1 μg siRNA/well.
Abbreviations: hGAPDH, human glyceraldehyde-3-phosphate dehydrogenase; PLCP, polycation liposome-encapsulated calcium phosphate nanoparticles; RT-qPCR, real-time quantitative polymerase chain reaction; siRNA, small interfering RNA; Scr, scramble.
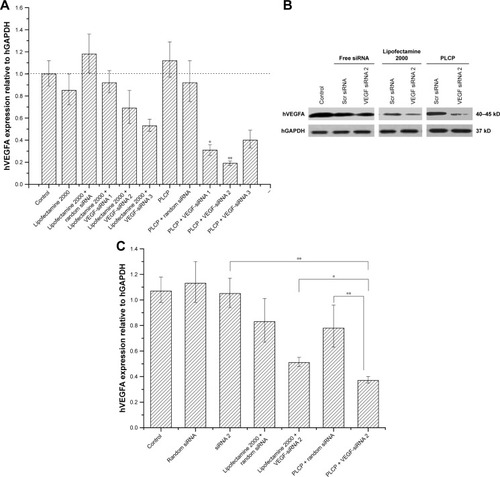
After a 48 h transfection, VEGF protein level was determined using Western blot assay () and converted to semiquantitative gray scale. As shown in , VEGF protein levels (63% and 49%) were obviously inhibited when VEGF siRNA 2 was mediated by PLCP and Lipofectamine 2000, respectively. These results were similar to those of RT-qPCR. The comparison between negative control group and blank carrier groups revealed the absence of non-specific effects.
Tumor growth inhibition in vivo
To evaluate the tumor inhibition efficacy of VEGF siRNA mediated by PLCP and combined treatment with DOX on MCF-7 xenograft in mice, tumor volumes were measured every 3 days after first administration, and tumor tissues were weighed after excising. Considering animal ethics, mice were sacrificed when tumor volume exceeded 1,000 mm3. shows that tumor volume grew fast in saline, PLCP, and PLCP/scramble (Scr) siRNA groups. The final tumor volume reached 1,145 mm3 (saline), 1,067 mm3 (PLCP), and 1,204 mm3 (PLCP/Scr siRNA), respectively, which were significantly larger than treatment groups. In contrast, the tumor volume was obviously inhibited after VEGF siRNA and DOX administration. After 28 days of inoculation, the tumor volumes of PLCP/VEGF siRNA group, DOX group, and PLCP/VEGF siRNA + DOX group were 356 mm3, 139 mm3, and 75 mm3, respectively. The tumor inhibition ratio was calculated by tumor weight (). Higher inhibition rates were observed in PLCP/VEGF siRNA (~72%) and DOX (~84%) groups compared with control and blank carrier groups. We also found that the combination therapy of VEGF siRNA and DOX may possess a more powerful ability on tumor inhibition (~91%). To evaluate the safety of PLCP, the body weight changes and physiological state of mice were recorded during the whole therapeutic period. No significant change in body weight was found ().
Figure 3 Tumor growth inhibition in vivo.
Notes: (A) Tumor growth curves of mice (n=5). (B) Comparison of tumor weight among mice that received different treatments. **P<0.01. (C) The changes in body weight.
Abbreviations: DOX, doxorubicin; PLCP, polycation liposome-encapsulated calcium phosphate nanoparticles; siRNA, small interfering RNA; Scr, scramble.
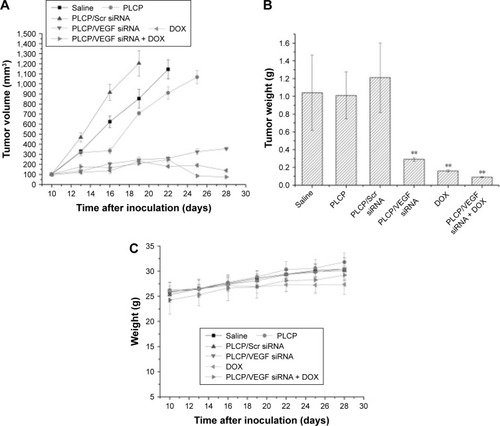
Immunohistochemistry study
Angiogenesis inhibition efficacy was evaluated by immunohistochemistry study. CD34 is the marker of vascular endothelial cells and CD34-positive cells displayed brown on the cytoplasm. Fewer immunoreactive microvessels were observed in the tumor tissue sections of VEGF siRNA-treated groups (). The MVD in VEGF siRNA group was about 58 microvessels/field of view, which was dramatically lower compared to saline (153 microvessels/field of view) and DOX (91 microvessels/field of view) groups (). Furthermore, we determined VEGF and HIF-1α protein expression in tumor tissues. VEGF protein expression was observed at low level when those mice were treated with VEGF siRNA (); however, it was expressed at higher level in control groups (more positive cells which presented in dark brown). Similar immunohistochemistry results of HIF-1α protein were observed ().
Figure 4 Determination of MVD in tumor xenograft among mice that received different treatments.
Notes: (A) Immunohistochemistry images. (B) Quantitation of MVD. *P<0.05 and **P<0.01. Saline (a), PLCP (b), PLCP/Scr siRNA (c), PLCP/VEGF siRNA (d), DOX (e), and PLCP/VEGF siRNA + DOX (f).
Abbreviations: DOX, doxorubicin; MVD, microvessel density; PLCP, polycation liposome-encapsulated calcium phosphate nanoparticles; Scr, scramble; siRNA, small interfering RNA.
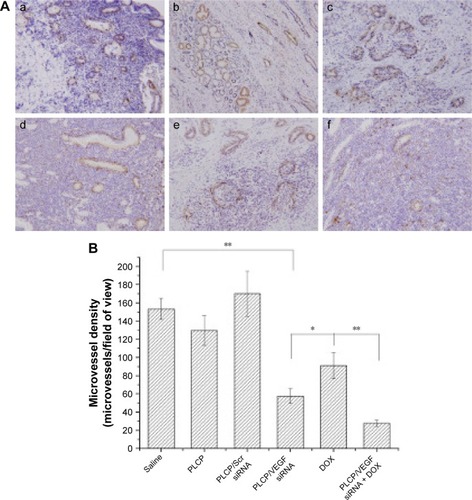
Figure 5 Expression of VEGF and HIF-1α by immunohistochemistry.
Notes: Expression of VEGF (A) and HIF-1α (B) in tumor xenograft among mice that received different treatments. Saline (a), PLCP (b), PLCP/Scr siRNA (c), PLCP/VEGF siRNA (d), DOX (e), and PLCP/VEGF siRNA + DOX (f).
Abbreviations: DOX, doxorubicin; HIF-1α, hypoxia-inducible factor-1α; PLCP, polycation liposome-encapsulated calcium phosphate nanoparticles; Scr, scramble; siRNA, small interfering RNA.
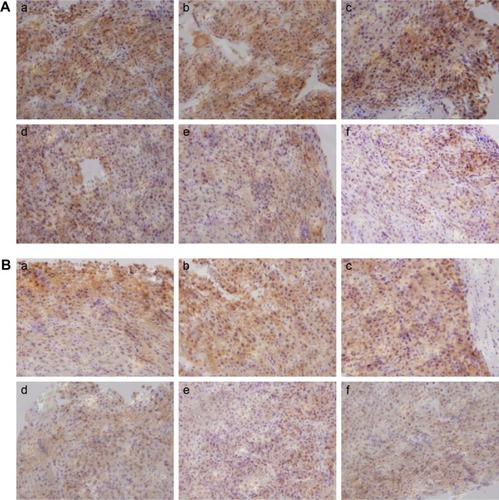
Discussion
Angiogenesis is known to be necessary for tumor progression. There was an autonomous system of vessels developing under the strict control of stimulating and inhibiting factors in tumor tissues.Citation20 VEGF showed a predominant role in tumor angiogenesis. Silencing VEGF expression by RNA interference is expected to be a promising strategy to suppress the tumor growth. siRNA is able to silence its target gene when it is successfully delivered into cytoplasm.Citation21 Delivery systems that enhance siRNA localization to the cytoplasm can facilitate gene silencing by siRNA therapeutics. Ideal siRNA delivery systems should be developed to be efficiently internalized by target cells, to allow efficient endosomal escape, and to selectively release siRNA into the cytoplasm. Widely used for the delivery of siRNA are cationic lipids or cationic polymers. Recently, various strategies have been utilized to design more complex structure with components of surface, intermediate layer, and core.Citation22 These components drive electrostatic interaction with siRNA and increase siRNA loading in vectors.Citation23 We have developed a core-membrane siRNA delivery system called PLCP composed of CaP nanoparticles core coated with PCL.
CaP nanoparticles were considered highly biocompatible and potentially useful in gene delivery;Citation15 however, the uncontrollable growth of CaP precipitates would affect their transfection.Citation24 In this study, we did further optimization on the mass ratio of CaCl2 and siRNA to obtain more stable and smaller particle size of CaP nanoparticles. The results of gel retardation assay indicated that with the increase in mass ratio, the stronger electrostatic interaction between CaCl2 and siRNA was formed (). The addition of sodium citrate could also effectively stabilize CaP precipitates by specific adsorption of citrate anions onto CaP nanocrystals, which increases the negative surface charge of CaP nanoparticles and consequently increases repulsive interparticle forces.Citation25 TEM results showed that multiple CaP nanoparticles are encapsulated into PCL (). We presumed that this special core-membrane structure would further stabilize the precipitation by hydrophobicity of outer lipid bilayer which was consistent with that which Li et al reported.Citation26
The outer lipid bilayer of PLCP could provide effective cellular internalization and endosomal escape by the synergistic effect of PEI and DOPE.Citation18 In addition, CaP nanoparticle core dissolves in acidic endosomes which increase the osmotic pressure and cause endosomal membrane rupture to release siRNA into cytoplasm.Citation27 Compared with commercial reagent, more than two times silencing activity in MCF-7 cells was found when treated with PLCP/VEGF siRNA, as shown in . Gene silencing activity on target mRNA level would be highly sequence specific. It is important to use siRNAs containing functional sequences to reduce the potential for unintended interactions between RNA species.Citation28 We designed three different VEGF siRNA sequences, and the RT-qPCR results indicated that VEGF siRNA 2 is the optimal sequence for the subsequent study expecting to reduce undesirable adverse effects. Gene silencing results would reflect on correspondence protein expression finally. There existed a time lag between protein expression and mRNA expression in cells. From the Western blot results (), the weakest VEGF protein strap was observed after 48 h transfection by PLCP. Although the difference in VEGF protein expression level got close between Lipofectamine 2000/VEGF siRNA and PLCP/VEGF siRNA, the latter showed superiority in protein inhibition. Free siRNA including VEGF siRNA and random siRNA showed little biological activity due to their instability and fast degradation.Citation29
In order to understand the potency of PLCP in tumor inhibition application, VEGF siRNA was delivered into the tumor xenografts. The results demonstrated that PLCP led to a significantly higher tumor growth inhibition than negative control saline (), while the use of Scr siRNA showing little tumor inhibition also confirmed the sequence specificity in line with in vitro study. As anticipated, decreased expression of VEGF in tumor tissues was well correlated with the reduced MVD and increased tumor inhibition potency ( and ). These results suggested that silencing VEGF expression would inhibit angiogenesis efficiently in the early stage of tumor formation and result in tumor growth inhibition. VEGF is a key protein downstream of HIF-1α under hypoxic conditions. In general, the expression of VEGF is controlled by HIF-1α in cancer cells. HIF-1α can activate the expression of the VEGF gene by binding to the hypoxia response element in the VEGF promoter. Then VEGF promotes vascular remodeling under hypoxia conditions. Interestingly, our study found that HIF-1α level seems to be affected by VEGF as well as in tumor tissues (). These results were consistent with those reported by Salva et al.Citation30 They found that HIF-1α inhibition rate can reach 43% when VEGF gene was knocked down. HIF-1α expression is induced via activation of PI3K/AKT signaling, which is required for VEGF expression.
Several studies and clinical application experiences have focused on the combination therapy of angiogenesis inhibitor and chemotherapeutics, such as bevacizumab combined with paclitaxel and carboplatin for non-small-cell lung cancer (NSCLC) treatment,Citation31 bevacizumab codelivery with DOX for breast cancer treatment,Citation32 and co-delivery of VEGF siRNA and DOX by nanocomplexes to human hepatocarcinoma QGY-7703 cells.Citation33 However, the combination therapy was still compromised by several obstacles: the overexpression of HIF-1α resulting from tumor vascular inhibition would induce the enhanced metastasis and drug resistance and systemic toxicity and myelosuppression caused by chemotherapeutics.Citation34 Here, we combined VEGF-siRNA and DOX for MCF-7 xenograft treatment. Compared to PLCP/VEGF siRNA or DOX used alone, the combination therapy can significantly inhibit tumor growth (~91%, ) even using 60% dosage of DOX. Meanwhile, reduced HIF-1α expression in tumor tissues was also observed, which suggests that metastasis and drug resistance would be decreased.
Conclusion
In summary, a stable core-membrane siRNA delivery system was developed in order to achieve enhanced tumor angiogenesis inhibition effect. VEGF siRNA mediated by PLCP represented superior capacity of gene silencing, resulting in significant angiogenesis and tumor growth inhibition. These results suggested that delivery of VEGF siRNA via PLCP to inhibit angiogenesis would be a promising approach for breast cancer treatment, especially combined with DOX.
Acknowledgments
This study was supported by the National Natural Science Foundation of China (NSFC No 81001409 and No 81402872) and Zhejiang Provincial Natural Science Foundation of China (No LY17H300001 and No LY17H160002).
Disclosure
The authors report no conflicts of interest in this work.
Supplementary materials
Figure S1 Size distribution measured by laser diffraction spectrometry.
Note: Size distribution of CaP nanoparticles (A), PCL (B), and PLCP (C).
Abbreviations: CaP, calcium phosphate; PCL, polycation liposomes; PLCP, polycation liposome-encapsulated calcium phosphate nanoparticles.
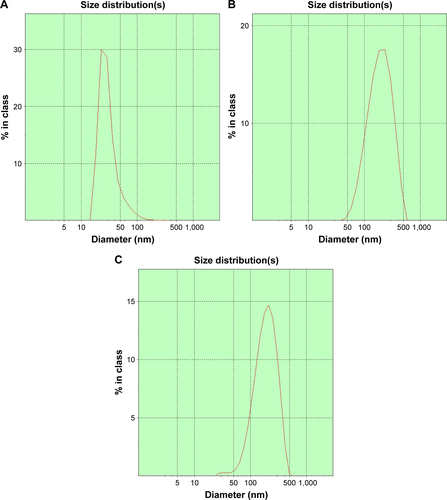
Figure S2 Amplification curves for RT-qPCR.
Notes: (A) Control. (B) Lipofectamine 2000. (C) Lipofectamine 2000 + random siRNA. (D) Lipofectamine 2000 + VEGF-siRNA 1. (E) Lipofectamine 2000 + VEGF-siRNA 2. (F) Lipofectamine 2000 + VEGF-siRNA 3. (G) PLCP. (H) PLCP + random siRNA. (I) PLCP + VEGF-siRNA 1. (J) PLCP + VEGF-siRNA 2. (K) PLCP + VEGF-siRNA 3.
Abbreviations: GAPDH, glyceraldehyde-3-phosphate dehydrogenase; PLCP, polycation liposome-encapsulated calcium phosphate nanoparticles; RT-qPCR, real-time quantitative polymerase chain reaction; siRNA, small interfering RNA.
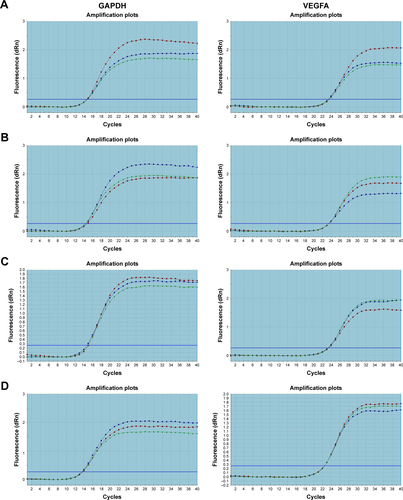
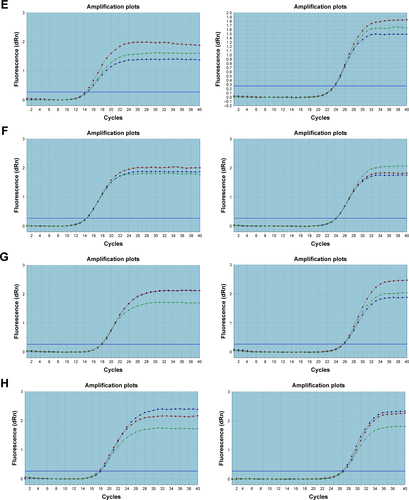
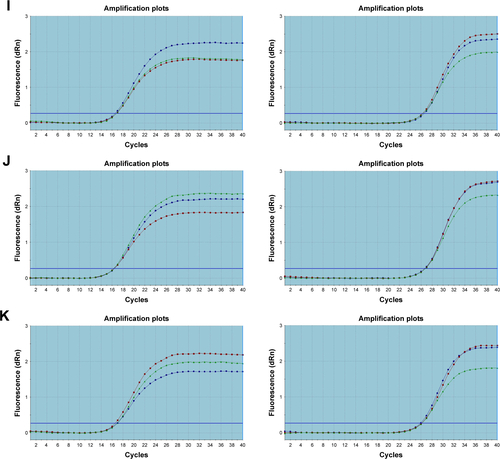
References
- WeltiJLogesSDimmelerSCarmelietPRecent molecular discoveries in angiogenesis and antiangiogenic therapies in cancerJ Clin Invest201312383190320023908119
- RibattiDDjonovVIntussusceptive microvascular growth in tumorsCancer Lett2012316212613122197620
- CarmelietPJainRKAngiogenesis in cancer and other diseasesNature2000407680124925711001068
- RamanathanROlexALDozmorovMBearHDFernandezLJTakabeKAngiopoietin pathway gene expression associated with poor breast cancer survivalBreast Cancer Res Treat2017162119119828062977
- JainRKDudaDGClarkJWLoefflerJSLessons from phase III clinical trials on anti-VEGF therapy for cancerNat Clin Pract Oncol200631244016407877
- LaiSRAndrewsLGTollefsbolTORNA interference using a plasmid construct expressing short-hairpin RNAMethods Mol Biol2007405313718369815
- BarataPSoodAKHongDSRNA-targeted therapeutics in cancer clinical trials: current status and future directionsCancer Treat Rev201650354727612280
- DorsettYTuschlTsiRNAs: applications in functional genomics and potential as therapeuticsNat Rev Drug Discov20043431832915060527
- OfekPTiramGSatchi-FainaroRAngiogenesis regulation by nanocarriers bearing RNA interferenceAdv Drug Deliv Rev Epub201722
- LuJJLangerRChenJA novel mechanism is involved in cationic lipid-mediated functional siRNA deliveryMol Pharm20096376377119292453
- KnudsenKBNorthevedHKumarPEIn vivo toxicity of cationic micelles and liposomesNanomedicine201511246747725168934
- LeeKBaeKHLeeYLeeSHAhnCHParkTGPluronic/polyethylenimine shell crosslinked nanocapsules with embedded magnetite nanocrystals for magnetically triggered delivery of siRNAMacromol Biosci201010323924519924685
- MaoSSunWKisselTChitosan-based formulations for delivery of DNA and siRNAAdv Drug Deliv Rev2010621122719796660
- ZhouJWuJHafdiNBehrJPErbacherPPengLPAMAM dendrimers for efficient siRNA delivery and potent gene silencingChem Commun20062223622364
- MaitraACalcium phosphate nanoparticles: second-generation nonviral vectors in gene therapyExpert Rev Mol Diagn20055689390516255631
- ZhangJSunXShaoRLiangWGaoJChenJPolycation liposomes combined with calcium phosphate nanoparticles as a non-viral carrier for siRNA deliveryJ Drug Deliv Sci Technol201530pt A16
- ChenJLWangHGaoJQChenHLLiangWQLiposomes modified with polycation used for gene delivery: preparation, characterization and transfection in vitroInt J Pharm20073431–225526117624698
- ChenJYuZChenHGaoJLiangWTransfection efficiency and intracellular fate of polycation liposomes combined with protamineBiomaterials20113251412141821047681
- WeidnerNSempleJPWelchWRFolkmanJTumor angiogenesis and metastasis – correlation in invasive breast carcinomaN Engl J Med1991324118
- BergersGBenjaminLETumorigenesis and the angiogenic switchNat Rev Cancer20033640141012778130
- HeSZhangDChengFGongFGuoYApplications of RNA interference in cancer therapeutics as a powerful tool for suppressing gene expressionMol Biol Rep20093682153216319117119
- KimHJKimAMiyataKKataokaKRecent progress in development of siRNA delivery vehicles for cancer therapyAdv Drug Deliv Rev2016104617727352638
- RaoNMCationic lipid-mediated nucleic acid delivery: beyond being cationicChem Phys Lipids2010163324525220060819
- KakizawaYFurukawaSKataokaKBlock copolymer-coated calcium phosphate nanoparticles sensing intracellular environment for oligodeoxynucleotide and siRNA deliveryJ Control Release200497234535615196761
- LeeuwenburghSCAnaIDJansenJASodium citrate as an effective dispersant for the synthesis of inorganic-organic composites with a nanodispersed mineral phaseActa Biomater20106383684419751849
- LiJYangYHuangLCalcium phosphate nanoparticles with an asymmetric lipid bilayer coating for siRNA delivery to the tumorJ Control Release2012158110811422056915
- KovtunAHeumannREppleMCalcium phosphate nanoparticles for the transfection of cellsBiomed Mater Eng2009192–324124719581719
- GorskiSAVogelJDoudnaJARNA-based recognition and targeting: sowing the seeds of specificityNat Rev Mol Cell Biol201718421522828196981
- SelvamCMutisyaDPrakashSRangannaKThilagavathiRTherapeutic potential of chemically modified siRNA: recent trendsChem Biol Drug Des Epub201745
- SalvaETuranSOErenFAkbugaJThe enhancement of gene silencing efficiency with chitosan-coated liposome formulations of siRNAs targeting HIF-1alpha and VEGFInt J Pharm2015478114715425445537
- YamasakiMMurakamiINakanoKCarboplatin plus weekly paclitaxel combined with bevacizumab as first-line treatment for non-small cell lung cancerAnticancer Res201737292392828179353
- TangYSoroushFTongZKianiMFWangBTargeted multidrug delivery system to overcome chemoresistance in breast cancerInt J Nanomedicine20171267168128176940
- HanLTangCYinCDual-targeting and pH/redox-responsive multi-layered nanocomplexes for smart co-delivery of doxorubicin and siRNABiomaterials201560425225982552
- FengQYuMZWangJCSynergistic inhibition of breast cancer by co-delivery of VEGF siRNA and paclitaxel via vapreotide-modified core-shell nanoparticlesBiomaterials201435185028503824680191