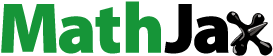
Abstract
Thermoresponsive drug delivery systems are designed for the controlled and targeted release of therapeutic payload. These systems exploit hyperthermic temperatures (>39°C), which may be applied by some external means or due to an encountered symptom in inflammatory diseases such as cancer and arthritis. The objective of this paper was to provide some solid evidence in support of the hypothesis that solid lipid nanoparticles (SLNs) can be used for thermoresponsive targeting by undergoing solid–liquid phase transition at their melting point (MP). Thermoresponsive lipid mixtures were prepared by mixing solid and liquid natural fatty acids, and their MP was measured by differential scanning calorimetry (DSC). SLNs (MP 39°C) containing 5-fluorouracil (5-FU) were synthesized by hot melt encapsulation method, and were found to have spherical shape (transmission electron microscopy studies), desirable size (<200 nm), and enhanced physicochemical stability (Fourier transform infrared spectroscopy analysis). We observed a sustained release pattern (22%–34%) at 37°C (5 hours). On the other hand, >90% drug was released at 39°C after 5 hours, suggesting that the SLNs show thermoresponsive drug release, thus confirming our hypothesis. Drug release from SLNs at 39°C was similar to oleic acid and linoleic acid nanoemulsions used in this study, which further confirmed that thermoresponsive drug release is due to solid–liquid phase transition. Next, a differential pulse voltammetry-based electrochemical chemical detection method was developed for quick and real-time analysis of 5-FU release, which also confirmed thermoresponsive drug release behavior of SLNs. Blank SLNs were found to be biocompatible with human gingival fibroblast cells, although 5-FU-loaded SLNs showed some cytotoxicity after 24 hours. 5-FU-loaded SLNs showed thermoresponsive cytotoxicity to breast cancer cells (MDA-MB-231) as cytotoxicity was higher at 39°C (cell viability 72%–78%) compared to 37°C (cell viability >90%) within 1 hour. In conclusion, this study presents SLNs as a safe, simple, and effective platform for thermoresponsive targeting.
Introduction
Thermoresponsive triggered release of the potent chemotherapeutic drugs has shown promising results in various research reports and clinical trials.Citation1 Thermoresponsive drug delivery systems (TDDS) are sensitive to higher temperature (39°C–45°C) and release payload at target sites, ie, hyperthermic body tissues.Citation2 However, synthesis of the thermoresponsive hydrogels and liposomes generally involves synthesis of block copolymers via complex chemical reactions and use of potentially toxic reagents.Citation3,Citation4 Liposomes are most widely used for thermoresponsive targeting, and one thermoresponsive liposome formulation, ie, ThermoDox®, has gained the US Food and Drug Administration (FDA) approval for the treatment of lung cancer.Citation5 ThermoDox® is administered in combination with the radiofrequency ablation for >45 minutes. Radiofrequency ablation is applied to kill major portion of the tumor and also induces release of doxorubicin to kill remaining tumor mass. However, ThermoDox® has short circulation life and should be administered immediately before radiofrequency ablation.Citation1
Many embodiments of thermoresponsive liposomes show low encapsulation efficiency (EE)Citation6 and unpredictable drug release,Citation3,Citation7 and their blood circulation life is very short.Citation1,Citation8 On the other hand, solid lipid nanoparticles (SLNs) have been reported to show long circulation life (>24 hours) and passively target cancers by enhanced permeability and retention (EPR) effect.Citation9,Citation10 In addition to this, SLN composition and their methods of preparation are considered safe as compared to other novel DDS such as liposomes.Citation11
Previously, SLNs have been used in combination with hydrogels to achieve thermoresponsive drug delivery.Citation12 In this system, hydrogels act as a thermoresponsive component and SLNs are used as a drug reservoir. Recently, we have reported for the first time that SLNs can be used for thermoresponsive drug delivery by tuning their melting point (MP).Citation13 These SLNs are solid at room temperature (37°C) and show minimal drug release. However, at the hyperthermic temperature (>39°C), they melt and undergo solid–liquid phase transition, leading to faster diffusion of drug into the surrounding dissolution medium.Citation13,Citation14 Since then, other research groups have also explored thermoresponsive drug release from SLNs. For example, ud Din et al prepared thermoresponsive SLN-loaded hydrogels for rectal delivery of flurbiprofen. These SLNs remained solid during storage conditions and underwent solid–liquid phase transition (MP 32°C) at physiological temperature.Citation15 In comparison to our reported thermoresponsive SLNs, these nanoparticles would be liquid at normal body temperature and cannot be regarded as TDDS. More recently, Brezaniova et al have reported temoporfin-loaded thermoresponsive SLN for photodynamic therapy of cancer using 1-tetradecanol lipids that melt at 39°C. Although they showed superior photodynamic efficacy against cancer, the critical parameters such as drug release, cytotoxicity or in vivo anticancer activity as a function of temperature (37°C and 39°C) are still not available.Citation6
While designing a smart DDS, periodic drug release is mostly studied by using the dissolution apparatus, which involves removal of samples from the dissolution medium. This protocol requires adjustment of equations to accommodate change in volume or the amount of the drug removed along with samples. Moreover, the samples should be analyzed either by the ultraviolet (UV)–Vis/fluorescence spectrophotometry or by high-performance liquid chromatography (HPLC), which is a laborious and time-consuming procedure. Therefore, an easy, quick and robust method is needed to quantify drug released from drug delivery systems. The differential pulse voltammetry (DPV) method has been used previously for assay of 5-fluorouracil (5-FU) in pharmaceutical dosage forms.Citation16 It involves application of the regular voltage pulses of increasing amplitude, which causes oxidation of the analyte and generation of current. The potential at which oxidation peak appears is characteristic of the analyte, and the height of the peak is directly related to the concentration of the analyte. Thus, DPV can be used for the qualitative and quantitative determination of electrochemically active drugs such as 5-FU.
The need of safe and effective targeted drug delivery systems can be addressed by SLNs. Therefore, this study was designed to comprehensively elucidate SLNs as alternatives to conventional TDDS. Different thermoresponsive lipid mixtures (TLMs) were prepared that would melt at 39°C and fabricate into SLNs. Characterization of solid–liquid phase transition in SLNs and drug release from SLNs were studied as a function of temperature. Furthermore, a DPV method was also developed for real-time determination of 5-FU release from the SLNs at physiological (37°C) and hyperthermic conditions (39°C). Then, in vitro cytotoxicity studies were performed at 37°C and 39°C to confirm thermoresponsive nature of SLNs. To the best of our knowledge, this is the first report to comprehensively characterize thermoresponsive behavior of SLNs and provide a novel method for the real-time monitoring of stimuli-responsive drug release.
Methodology
Materials
Lauric acid (LA), oleic acid (OA), linoleic acid (LIA), Brij 58, Span 80, ethanol and 5-FU were purchased from Sigma-Aldrich Co. (St Louis, MO, USA). Dialysis bags (molecular weight cutoff value 8,000 Da) were purchased from Spectrum Labs (Rancho Dominguez, Canada). Tetrazolium dye (MTS) reagent for cell viability assay was purchased from Promega Corporation (Fitchburg, WI, USA). Human gingival fibroblast (HGF; PCS-201-108) cells and human breast adenocarcinoma cells (MDA-MB-231, HTB-26) were purchased from American Type Culture Collection (ATCC) (Manassas, VA, USA). Dulbecco’s Modified Eagle’s Medium (DMEM) was purchased from GE Healthcare UK Ltd (Little Chalfont, UK). Milli Q deionized water (~18.2 μS) was used throughout the study.
Preparation and characterization of lipid mixtures
LA (12 carbon atoms, MP 44°C) was selected as the solid fatty acid and mixed with either of liquid fatty acids, ie, OA (MP 14°C) or LIA (MP −5°C), in different ratios (3:1–12:1). First, LA was melted to 50°C, and liquid fatty acid was added to it. Lipids were mixed for 5 minutes and cooled down to room temperature to form lipid mixture of LA and OA (LO) and LA and LIA (LL). These lipid mixtures were characterized by differential scanning calorimetry (DSC) to determine MP. Then, a graph was plotted between MP of the lipid mixtures and the proportion of solid fatty acid in the lipid mixture. Straight line equations were derived for the graphs to determine the proportion of solid fatty acids for TLM with MP of 39°C.
Evaluation of solid–liquid phase transition by light transmission studies
It is widely accepted that solid lipids are opaque, whereas oils are transparent to light. Therefore, light transmission studies were performed at 37°C and 39°C.Citation17 Briefly, lipid mixture was taken in a cuvette of UV–Vis spectrophotometer (U2020; IRMECO, Geesthacht, Germany) and reading was measured at 500 nm at 37°C and 39°C.
Preparation of SLNs
SLNs can be prepared by various methods.Citation18 Previously, we have reported that the hot melt encapsulation (HME) method is superior to other methods due to the lack of toxic organic solvents and the ease of process parameters.Citation14 Therefore, HME was selected for the preparation of the SLNs with composition shown in . First, TLM (100 mg) was melted to 45°C and 5-FU was added to it. Stirring was continued for 5 minutes, and aliquot of ethanol was added to facilitate homogenous mixing of 5-FU in the lipid phase. Aqueous phase (5 mL) was prepared by mixing either Brij 58 surfactant (5%) alone or the combination of Brij 58 and Span 80 (3.75% and 1.25%, respectively) in water and heated to 45°C. Then, the lipid phase was added drop wise to the aqueous phase and stirring was continued at 45°C. After 5 minutes, the heater of the hot plate stirrer was switched off and the hot melt emulsion so formed was allowed to cool down to room temperature. The as-prepared SLNs were centrifuged at 14,000 rpm at 4°C and washed with deionized water three times following the same procedure. SLNs were lyophilized in the presence of ethylene glycol as a cryoprotectant at −105°C and reduced pressure for 24 hours.
Table 1 Composition and properties of SLNs
Characterization of SLNs
Thermoresponsive nature of the SLNs was confirmed by the light transmission studies at different temperatures (37°C, 38°C and 39°C) as described earlier. The size and zeta potential of SLNs were measured by using zetasizer (ZS 90; Malvern Instruments, Malvern, UK). The morphology of SLNs was studied by transmission electron microscopy (TEM; JSM 75000; JEOL, Tokyo, Japan).
Yield of the SLNs produced by a method is required from pharmaceutical point of view. In this study, the SLNs produced in a single batch were weighed, and the percentage yield was calculated by using the following formula:Citation19
Stability of 5-FU and its compatibility with excipients was evaluated by attenuated total reflection-Fourier-transform infrared (FTIR) spectroscopy. FTIR spectra were taken for all individual components, 5-FU and drug-loaded SLNs. In case of any chemical interaction, 5-FU will not be released in dissolution studies or its efficacy may be lost in the cytotoxicity studies. Existence of intact 5-FU peaks in the SLNs would indicate its compatibility with other excipients.Citation20 The colloidal stability of SLNs was measured in phosphate-buffered saline (PBS) (1, 0.1 and 0.01 M) and plasma. SLNs were dispersed in each solution and stored at ambient temperature. After 24 hours, SLN dispersions were sonicated for 1 minute and size was evaluated by DLS measurements.Citation21
Drug loading studies
Calibration curve was constructed using standard solutions to find unknown concentration of samples.Citation22 EE is the amount of drug encapsulated in nanoparticles from a given amount of drug used. In other words, it is percent efficiency of nanoparticles to contain drug. First, nanoparticles were separated by ultracentrifugation. After completion, the supernatant was taken and its absorbance was recorded at 258 nm to measure the amount of drug in it using the calibration curve. Then, EE was calculated by the following formula:
Drug release studies by UV–Vis spectrophotometer
The dissolution studies are used to surrogate in vivo drug release behavior. In this study, the dialysis bag method was employed by using United States Pharmacopeia (USP) type II dissolution apparatus with paddle assembly (Pharma Test, Hainburg, Germany).Citation14,Citation23 Cellulose ester dialysis bag (molecular weight cutoff value 10,000 Da) was selected on the basis of molecular weight of drug and size of nanoparticles. The SLNs equivalent to 4 mg 5-FU were taken in a dialysis bag and tied at both ends. The filled dialysis bags were placed in the vessel of the dissolution apparatus containing 250 mL dissolution medium, ie, PBS, of pH 7.4.Citation24 Dissolution studies were carried out by adjusting the paddle speed at 50 rpm, and the temperature was set to 37°C and 39°C for the evaluation of the thermoresponsive drug release. Samples were taken out at predetermined time intervals, and their absorbance was taken by UV–Vis spectrophotometer (U2020). Absorbance was fitted to the straight line equation of the calibration curve to find the amount of the 5-FU released.
Dissolution studies by DPV
The DPV setup for drug release studies consisted of a silver/silver chloride reference electrode, a platinum counter electrode and a graphite working electrode (). First, calibration curve was constructed by adding different amounts of 5-FU (0.05–5 mM) in 25 mL of PBS (0.1 M, pH 7.4) taken in a glass flask. The electrodes were dipped in the PBS-containing flask, and readings were taken using Autolab (PGStat 30; Metrohm, Riverview, FL, USA). Next, the SLNs containing 5-FU (equivalent to 1 mM) were taken in the dialysis bag and placed in the 25 mL of the PBS (pH 7.4). Readings were taken at different time intervals as the electrodes were kept in the dissolution medium throughout the experiment. The amount of 5-FU released was calculated using the calibration curve. In all runs, samples were purged with nitrogen gas to degas and remove oxygen.
Figure 1 Schematic presentation of the DPV method for drug release studies.
Notes: 5-FU released from SLNs will undergo oxidation due to applied pulsed voltage. The oxidized 5-FU is accumulated at the working electrode and produces signal proportional to concentration of 5-FU in the dissolution medium.
Abbreviations: DPV, differential pulse voltammetry; 5-FU, 5-fluorouracil; SLN, solid lipid nanoparticle.
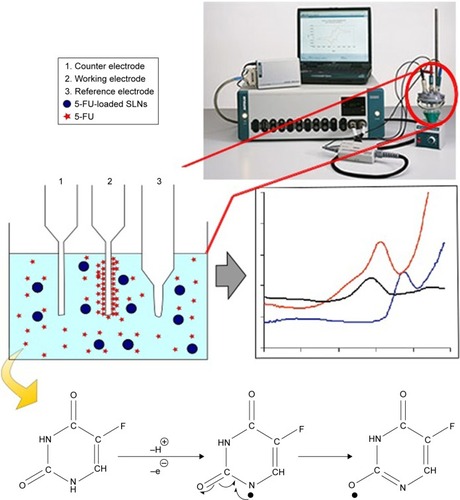
Dissolution kinetics studies
Dissolution profiles obtained by the UV–Vis spectrophotometric method and electrochemical method were analyzed by model-independent and model-dependent approaches as recommended by the FDA for comparison of drug release. Model independent analysis included calculation of difference factor (f1) and similarity factors (f2). f1 calculates percent error, while f2 represents percentage similarity between two curves. Generally, f1 value is <15 and f2 value is >50 to ensure equivalence in two drug release data sets.Citation25
Model dependent analysis was done by fitting dissolution profiles in first-order, zero-order, Korsmeyer–Peppas and Higuchi models.Citation26 The analysis was done by using DDSolver, an extension of MS Excel.
Cytotoxicity studies
HGF cell lines were used as normal cells, whereas MDA-MB-231 cell line was used as a model breast cancer cell line. Cells were defrosted by thawing in warm water and cultured in the DMEM containing 10% fetal bovine serum and 1% penicillin/streptomycin in a 75 cm2 flask. The incubator was set at 37°C and 5% carbon dioxide. Cells were subcultured two times and used for further studies when they reached 80% confluence.
Biocompatibility and cytotoxicity studies were performed against normal tissue cell line (HGF) and breast cancer cell line (MDA-MB-231). Cells were seeded at a density of 15,000 cells/cm2 in a 96-well plate using the DMEM containing 10% fetal bovine serum and 1% penicillin/streptomycin. After 24 hours incubation, samples were added to each cell line with drug concentration ranging from 0.05 to 5 μM for HGF and 0.05 to 1 μM for MDA-MB-231, respectively. For HGF biocompatibility study, samples were incubated with cells for 24 hours, while for SLN study, cytotoxicity of MDA-MB-231 cells was evaluated after 1 hour incubation at both 37°C and 39°C, respectively. All samples were sterilized by UV exposure for 20 minutes. All experiments were performed in triplicate, and results are presented as mean ± SD. Cell viability after exposure to drug-loaded nanoparticles was evaluated by MTS assay.
Statistical analysis
Experiments were performed in replicates, and results are presented as mean ± SD. For the comparison of drug release, different models were applied as discussed earlier. For the comparison of cytotoxicity studies, Student’s t-test and analysis of variance (ANOVA) were used to calculate significance (p), and confidence interval was fixed at 95%.
Results
Preparation and characterization of TLMs
TLMs were prepared in varying ratios of solid and liquid fatty acids. Their MPs were determined by DSC studies, and results were plotted against solid lipid proportion (). We observed a linear decrease in MP of all mixtures when the proportion of solid fatty acids in TLMs decreased with respect to a constant amount of liquid fatty acids. TLMs were identified by straight line equation. The selected TLMs (with MP ~39°C) were found in a molar ratio of 5.26:1 and 2.47:1 for LO and LL, respectively. These TLMs would melt at 39°C, which is the desirable temperature for thermoresponsive drug release.
Figure 2 Preparation of TLMs and their characterization.
Note: (A) Standard curve of lipid mixtures LO and LL for selections of lipid mixture with MP of 39°C, (B) light transmittance from lipid mixtures LO and LL for solid–liquid phase transition, and (C) light transmittance of SLNs prepared from LO (FLOM and FLOD) and LL (FLLD).
Abbreviations: TLM, thermoresponsive lipid mixture; LO, lauric acid and oleic acid; LL, lauric acid and linoleic acid; MP, melting point; SLN, solid lipid nanoparticle; 5-FU, 5-fluorouracil; FLOM, 5-FU-loaded lauric acid and oleic acid nanoparticles prepared with mono-surfactant system; FLOD, 5-FU-loaded lauric acid and oleic acid nanoparticles prepared with double-surfactant system; FLLD, 5-FU-loaded lauric acid and linoleic acid nanoparticles prepared with double-surfactant system; SLN, solid lipid nanoparticle.
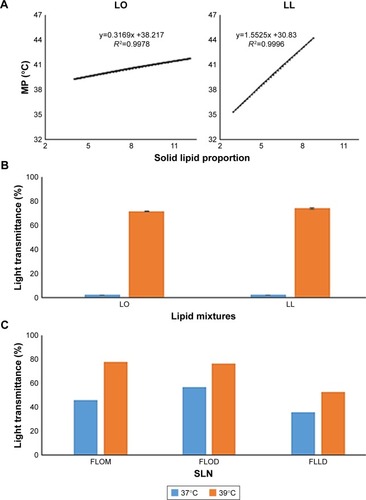
We confirmed thermoresponsive behavior of the TLMs by performing light transmission studies. All TLMs remained solid at 37°C as negligible light transmission was observed (). However, high light transmission (>75%) was observed at 39°C for both LO and LL, respectively, which indicated conversion of TLM into more transparent liquid phase.Citation17
Preparation and characterization of SLNs
The SLNs were prepared by the HME method, which is a one pot method and uses only water as a solvent in addition to the SLN components. Therefore, the SLNs prepared in this study by just physical mixing of fatty acid can be a safe alternative to other TDDS. The process parameters used in this method were similar to those required for conventional semisolid dosage forms and may be feasible for large-scale production. 5-FU-loaded lauric acid and linoleic acid nanoparticles prepared with mono-surfactant system (FLLM) were unstable and formed a gel-like phase immediately. Therefore, it was not characterized further and removed from the study. Size and zeta potential of SLNs are important due to many reasons. First, they should be small enough to cross the biological barriers such as fenestrated blood vessels with fenestrations or pore size of 50–150 nm.Citation27 Second, nanoparticles production should be homogenous for better performance in vitro and in vivo.Citation28 On the other hand, zeta potential is important with respect to colloidal stability of the SLNs. Nanoparticles with a zeta potential between 20 and 40 mV will have sufficient repulsion to remain homogeneously dispersed in the aqueous dispersion.Citation29 The size of 5-FU-loaded lauric acid and oleic acid nanoparticles prepared with mono-surfactant system (FLOM), 5-FU-loaded lauric acid and oleic acid nanoparticles prepared with double-surfactant system (FLOD) and 5-FU loaded lauric acid and linoleic acid nanoparticles prepared with double-surfactant system (FLLD) was <200 nm (polydispersity index [PDI] <0.2), and zeta potential was <−30 mV in all cases, which is desirable in drug delivery applications (). We confirmed thermoresponsive nature of as-prepared SLNs by light transmission studies. Light transmission of SLN dispersions was higher at 39°C as compared to 37°C (). This can be attributed to liquid-phase SLNs at 39°C that are more transparent than solid SLNs.Citation17 No change was observed in the SLN size when stored with plasma or low-concentration PBS. However, they aggregated in 1 M PBS, and the size of FLOM, FLOD and FLLD was increased to 360, 514 and 419 nm, respectively. The higher electrolyte concentration caused reduction in electrostatic repulsion, which resulted in aggregation of SLNs.Citation30
Drug loading studies
The EE of 5-FU with different formulations is given in . No modification was done to improve EE because it might have affected the thermoresponsive behavior of prepared SLNs. The amount of 5-FU encapsulated in SLNs was sufficient for further analysis.
Transmission electron microscopy
Transmission electron micrograph reveals that FLOM (), FLOD (), FLLM () and FLLD () possess spherical shape and smooth surface. Aggregated nanoparticles of FLLD can also be seen, and, therefore, it was excluded from further studies.
Figure 3 TEM images of (A) FLOM, (B) FLOD, (C) FLLM and (D) FLLD.
Abbreviations: TEM, transmission electron microscopy; FLOM, 5-FU-loaded lauric acid and oleic acid nanoparticles prepared with mono-surfactant system; FLOD, 5-FU-loaded lauric acid and oleic acid nanoparticles prepared with double-surfactant system; FLLM, 5-FU-loaded lauric acid and linoleic acid nanoparticles prepared with mono-surfactant system; FLLD, 5-FU-loaded lauric acid and linoleic acid nanoparticles prepared with double-surfactant system; 5-FU, 5-fluorouracil.
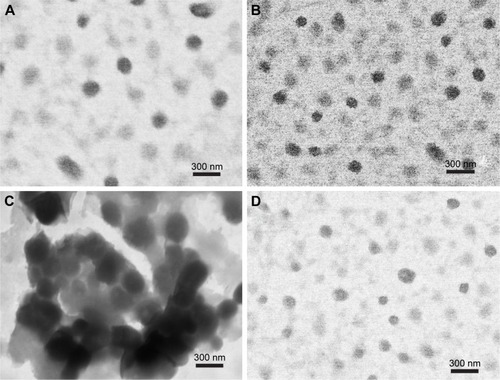
FTIR studies
The primary amine (−NH) stretching of 5-FU is present between 3,300 and 3,400 cm−1, which indicates that 5-FU was present intact in SLNs.Citation20 The low intensity of 5-FU peaks was due to the amount of 5-FU in the SLNs ().
Figure 4 FTIR spectra of FLOM, FLOD and FLLD.
Abbreviations: FTIR, Fourier transform infrared spectroscopy; FLOM, 5-FU-loaded lauric acid and oleic acid nanoparticles prepared with mono-surfactant system; FLOD, 5-FU-loaded lauric acid and oleic acid nanoparticles prepared with double-surfactant system; FLLD, 5-FU-loaded lauric acid and linoleic acid nanoparticles prepared with double-surfactant system; 5-FU, 5-fluorouracil.
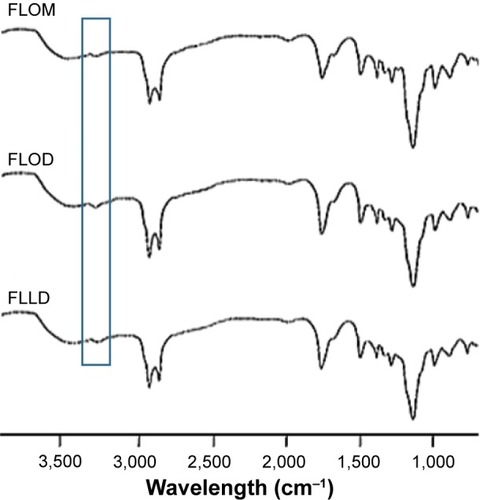
Thermoresponsive drug release studies by UV–Vis spectrophotometry
First, USP type II apparatus was used for dissolution experiment, and the amount of 5-FU released was determined by a UV–Vis spectrophotometer using the calibration curve (). We found that 5-FU release was slow at 37°C (<28%) and exhibited faster drug release (>90%) at 39°C, which is characteristic of TDDS, such as thermoresponsive liposomes ().Citation8 The significantly higher drug release at 39°C (p-value <0.05) confirmed that the SLNs show thermoresponsive drug release at hyperthermic temperature. It can also be seen that the drug release at 39°C is similar to the drug release from model OA emulsions used in the study (), thus suggesting that the thermoresponsive drug release from SLNs is due to their existence in the liquid state at hyperthermic temperature. Although TDDS have been reported with targeting temperature of >41°C by using external hyperthermia, we have prepared SLNs to release payload at 39°C, which is also known as low critical temperature and is more commonly encountered in the clinical setup.Citation2,Citation4 Among the three formulations, FLOM showed relatively faster drug release as 100% drug was released in <5 hours. This may be due to the fact that the double surfactant system formed a compact coating that also retarded the release of 5-FU.
Figure 5 Graphical presentation of the (A) calibration curve of 5-FU prepared by using UV–Vis spectrophotometer and (B) 5-FU release from SLNs at 37°C (solid lines) and 39°C (dashed lines) by using USP dissolution apparatus (data = mean ± SD, n=3).
Abbreviations: 5-FU, 5-fluorouracil; UV-Vis, ultraviolet-visible; SLN, solid lipid nanoparticle; FLOM, 5-FU-loaded lauric acid and oleic acid nanoparticles prepared with mono-surfactant system; FLOD, 5-FU-loaded lauric acid and oleic acid nanoparticles prepared with double-surfactant system; FLLD, 5-FU-loaded lauric acid and linoleic acid nanoparticles prepared with double-surfactant system; FOD, 5-FU-loaded oleic acid microemulsion with double-surfactant system; FOM, 5-FU-loaded oleic acid microemulsion with mono-surfactant system; USP, United States Pharmacopeia.
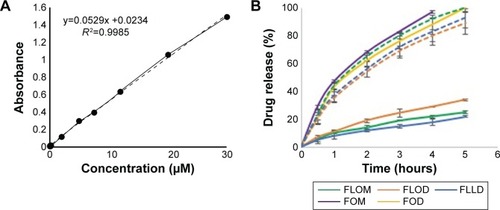
Thermoresponsive drug release studies by DPV method
The DPV method was used for real-time detection of thermoresponsive drug release from SLNs. 5-FU peak was observed at 1.15 V, and the difference in peak intensity was recorded at different drug concentrations (0.05–2.5 mM). The DPV method showed linear relation between 5-FU concentration and current measurement by electrodes (), which implies that the DPV method can be used for the detection of this drug using a calibration curve (). In the drug release studies, the DPV method also confirmed thermoresponsive drug release from the SLNs as the sustained drug release pattern at 37°C was transformed into faster release at 39°C (). DPV is a one pot method and required smaller amount of dissolution media. It also eliminated the need to take samples from the dissolution medium for analysis and subsequent adjustment of the formulas for samples removed. Previously, other novel methods have been suggested to monitor drug release such as Förster resonance energy transfer (FRET). However, FRET is used mainly to measure release of fluorescent drug molecules,Citation31 whereas DPV can be used for both fluorescent and nonfluorescent molecules. The drug release profiles obtained by the DPV method corroborates the results of the UV–Vis detection method. Therefore, the DPV method may be used as a simple and efficient alternative to conventional dissolution studies for real-time analysis of drug release.
Figure 6 DPV method for the (A) DPV response to different concentrations of 5-FU, (B) calibration curve of 5-FU as plot of drug concentration and measured electric current, and (C) 5-FU release from SLNs at 37°C (solid lines) and 39°C (dashed lines) (data = mean ± SD, n=3).
Abbreviations: DPV, differential pulse voltammetry; 5-FU, 5-fluorouracil; SLN, solid lipid nanoparticle; FLOM, 5-FU-loaded lauric acid and oleic acid nanoparticles prepared with mono-surfactant system; FLOD, 5-FU-loaded lauric acid and oleic acid nanoparticles prepared with double-surfactant system; FLLD, 5-FU-loaded lauric acid and linoleic acid nanoparticles prepared with double-surfactant system.
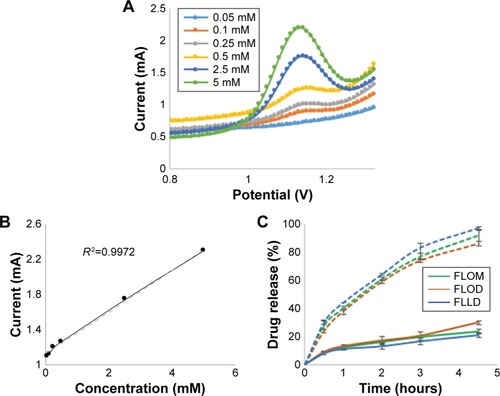
Dissolution kinetic analysis
In drug release kinetics analysis, all SLN formulations fit better to the zero-order model depicting a constant drug release at 37°C (). It can also be seen that the values of zero-order rate constant are higher at 39°C, which confirms faster drug release at the hyperthermic temperature. In the Korsmeyer–Peppas model, the value of release exponent “n” was between 0.5 and 1 at 37°C, whereas it was <0.5 at 39°C. These results suggested that the 5-FU release from the SLNs was anomalous at 37°C and diffusion controlled at 39°C. In SLNs, the aqueous dissolution medium has slow penetration in lipids, which leads to sustained release of drug over prolonged period of time.Citation14 Furthermore, the surfactant coating the SLN surface may be removed and diffuse to the surrounding aqueous phase. The pores caused by the removal of the surfactant molecules may facilitate the penetration of water corresponding to the erosion-controlled release mechanism, although it is a slow process.Citation14 In this situation, drug release mechanism will be anomalous, ie, superimposition of diffusion and erosion drug release. At 39°C, the drug release was faster and the diffusion would prevail as drug release mechanism. In the DPV method, the value of “n” was between 0.5 and 1 at both temperatures, suggesting that anomalous drug release occurs at both temperatures, although “n” values were closer to the diffusion region at 39°C. Similarly, all formulations showed good fit to the Higuchi model at both temperatures, thus suggesting that the diffusion was the predominant mechanism for the release of the 5-FU.
Table 2 Kinetic modeling of drug release profiles by data fitting in different models
Comparison of both dissolution methods is presented in terms of difference and similarity factors (). In all cases, the values of difference factors were <15 and values of similarity factors were >50. This depicts that drug release profiles obtained by the UV-Vis spectrophotometer and DPV are similar. The agreement in the results of both methods proves that the DPV method can be used for real-time monitoring of drug release from nanoparticles.
Table 3 Similarity factors and difference factors of drug release profiles by UV–Vis spectrophotometer and the DPV method
Cytotoxicity studies
The cytotoxicity studies were performed against HGF cells for 24 hours at 37°C, and the results are shown in . The results indicated that blank SLNs showed good compatibility (>90% cell viability) even at a high dose, whereas 5-FU-loaded SLN formulations showed dose-dependent cytotoxicity. Among all the 5-FU-loaded nanoparticles, FLOM generated relatively higher cytotoxicity, but cell viability was still >80% after 24 hours. Nevertheless, the difference in cytotoxicity of FLOM, FLOD and FLLD was insignificant (p>0.05). On the other hand, all formulations showed thermoresponsive cytotoxicity against the MDA-MB-231 cells (). Although low cytotoxicity was observed at 37°C (2%–8%), it was higher at 39°C (22%–28%) after 1 hour exposure. The significantly higher cytotoxicity (p<0.05) at hyperthermic temperature was due to the higher amount of 5-FU released at certain temperature. 5-FU belongs to antimetabolites family of anticancer drugs. It exerts cytotoxic effects by blocking nucleic acid synthesis, ie, by incorporation of its metabolites in RNA and DNA and by inhibiting thymidylate synthase.Citation32 In addition, the cytotoxicity of SLNs was lower than the cytotoxicity induced by the same concentration of 5-FU solution (). The reason for this observation was that the 5-FU was released from SLNs over time and the whole drug was not available to induce cytotoxicity during 1-hour study period. The cytotoxicity studies were conducted for 1 hour at hyperthermic temperature in accordance with the generally used protocol for thermotherapy of cancer.Citation7,Citation33
Figure 7 Biocompatibility and cytotoxicity of the (A) blank and 5-FU-loaded SLNs against HGF cells after 24 hours and (B) 5-FU-loaded SLNs against breast cancer cells (MDA-MB-231) after 1 hour at 37°C and 39°C (data = mean ± SD, n=3).
Note: (B) 37 and 39 represent temperature (°C) of drug release studies.
Abbreviations: 5-FU, 5-fluorouracil; SLN, solid lipid nanoparticle; HGF, human gingival fibroblast; FLOM, 5-FU-loaded lauric acid and oleic acid nanoparticles prepared with mono-surfactant system; FLOD, 5-FU-loaded lauric acid and oleic acid nanoparticles prepared with double-surfactant system; FLLD, 5-FU-loaded lauric acid and linoleic acid nanoparticles prepared with double-surfactant system; LOM, lauric acid and oleic acid nanoparticles prepared with mono-surfactant system; LOD, lauric acid and oleic acid nanoparticles prepared with double-surfactant system; LLD, lauric acid and linoleic acid nanoparticles prepared with double-surfactant system.
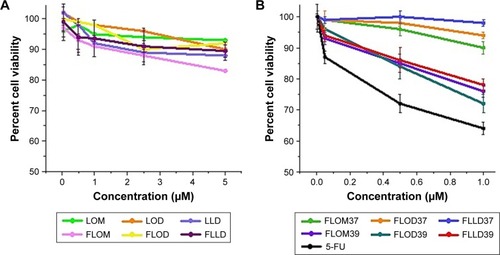
Conclusion
This article reports a quick and reliable method for the preparation of SLNs by physical mixing of solid and liquid fatty acids. The thermoresponsive behavior of lipids and as-prepared SLNs was confirmed by higher light transmission from the liquid phase at 39°C. Drug release studies showed that 5-FU release was higher at 39°C due to the presence of liquid phase than that of 37°C. Further proof of thermoresponsive drug release from SLNs was obtained by devising a DPV method for real-time electrochemical detection of 5-FU, which also confirmed thermoresponsive drug release at 39°C. A model-independent approach confirmed the agreement in results of spectrophotometry and DPV methods. In model-dependent analysis, drug release was found to be diffusion controlled at 39°C as predicted by good fit to Higuchi and Korsmeyer–Peppas model (n<0.5). SLNs were biocompatible to HGF cells and exhibited 5-FU dose-dependent cytotoxicity to MDA-MB-231 breast cancer cells. Two to three times higher cytotoxicity was achieved against cancer cells at 39°C, which also suggested thermoresponsive targeting by using SLNs. Hence, this study provided a thermoresponsive targeting platform by using SLNs that may be simple, economical and a safe alternative to other thermoresponsive DDS.
Acknowledgments
The authors acknowledge the contribution of Naveed Akhter and Anum Munawar during different experiments. The authors would also like to thank the National Institute of Biotechnology and Genetic Engineering, Pakistan; The Islamia University of Bahawalpur, Pakistan, and Northeastern University, MA, USA, for providing research facilities. MR also availed two scholarships from the Higher Education Commission of Pakistan under the Indigenous PhD Scholarship (Pin: 213-53204-2BM2-034) and International Research Support Initiative Program (Pin: IRSIP 32 PSc 15) during this study.
Disclosure
The authors report no conflicts of interest in this work.
References
- DouYHynynenKAllenCTo heat or not to heat: challenges with clinical translation of thermosensitive liposomesJ Control Release2017249637328122204
- MadniASarfrazMRehmanMLiposomal drug delivery: a versatile platform for challenging clinical applicationsJ Pharm Pharm Sci201417340142625224351
- LuTten HagenTLInhomogeneous crystal grain formation in DPPC-DSPC based thermosensitive liposomes determines content release kineticsJ Control Release2016247647228042084
- Al-AhmadyZKostarelosKChemical components for the design of temperature-responsive vesicles as cancer therapeuticsChem Rev201611663883391826934630
- BulbakeUDoppalapudiSKommineniNKhanWLiposomal formulations in clinical use: an updated reviewPharmaceutics201792e1228346375
- BrezaniovaIHrubyMKralovaJTemoporfin-loaded 1-tetrade-canol-based thermoresponsive solid lipid nanoparticles for photodynamic therapyJ Control Release2016241344427622757
- ChristensenKYVizcayaDRichardsonHLavouéJAronsonKSiemiatyckiJRisk of selected cancers due to occupational exposure to chlorinated solvents in a case–control study in MontrealJ Occup Environ Med201355219820823147555
- LokerseWJKneepkensECten HagenTLEggermontAMGrüllHKoningGAIn depth study on thermosensitive liposomes: optimizing formulations for tumor specific therapy and in vitro to in vivo relationsBiomaterials20168213815026761778
- GreishKEnhanced permeability and retention (EPR) effect for anticancer nanomedicine drug targetingCancer Nanotechnol20106242537
- PrianoLEspostiDEspostiRSolid lipid nanoparticles incorporating melatonin as new model for sustained oral and transdermal delivery systemsJ Nanosci Nanotechnol20077103596360118330178
- DoktorovovaSSoutoEBSilvaAMNanotoxicology applied to solid lipid nanoparticles and nanostructured lipid carriers–a systematic review of in vitro dataEur J Pharm Biopharm201487111824530885
- HaoJWangXBiYFabrication of a composite system combining solid lipid nanoparticles and thermosensitive hydrogel for challenging ophthalmic drug deliveryColloids Surf B Biointerfaces201411411112024176890
- RehmanMAsadullah MadniWSKIhsanAKhanMIChaudharyOJSustained release solid lipid nanoparticles of simultaneously loaded hydrophobic drug, diacerein and gold nanoparticles and their potential for thermoresponsive fluxPaper presented at: Macro 20142014Chiang Mai, Thailand
- RehmanMAsadullah MadniAIKhanWSSolid and liquid lipid-based binary solid lipid nanoparticles of diacerein: in vitro evaluation of sustained release, simultaneous loading of gold nanoparticles, and potential thermoresponsive behaviorInt J Nanomedicine201510280525897224
- ud DinFMustaphaOKimDWNovel dual-reverse thermosensitive solid lipid nanoparticle-loaded hydrogel for rectal administration of flurbiprofen with improved bioavailability and reduced initial burst effectEur J Pharm Biopharm201594647225979136
- ShahANosheenEZafarFPhotochemistry and electrochemistry of anticancer uracilsJ Photochem Photobiol B201211726927723123164
- HelgasonTSalminenHKristbergssonKMcClementsDJWeissJFormation of transparent solid lipid nanoparticles by microfluidization: influence of lipid physical state on appearanceJ Colloid Interface Sci201544811412225723787
- MüllerRHMäderKGohlaSSolid lipid nanoparticles (SLN) for controlled drug delivery–a review of the state of the artEur J Pharm Biopharm200050116117710840199
- HarshaSPharmaceutical suspension containing both immediate/sustained-release amoxicillin-loaded gelatin nanoparticles: preparation and in vitro characterizationDrug Des Devel Ther2013710271033
- CoatesJInterpretation of infrared spectra, a practical approachEncycl Anal Chem Epub2000915
- KhanMIMadniAPeltonenLDevelopment and in-vitro characterization of sorbitan monolaurate and poloxamer 184 based niosomes for oral delivery of diacereinEur J Pharm Sci201695889527600819
- FarooqMAhmadMMadniAHanifMKhanMRDevelopment and evaluations of aceclofenac microcapsules for colon-targeted delivery: an in vitro studyAdv Polym Technol2015342e21482
- LuoYKirkerKRPrestwichGDCross-linked hyaluronic acid hydrogel films: new biomaterials for drug deliveryJ Control Release200069116918411018555
- WangYLiXWangLXuYChengXWeiPFormulation and pharmacokinetic evaluation of a paclitaxel nanosuspension for intravenous deliveryInt J Nanomedicine201161497150721796250
- US Department of Health and Human ServicesFood and Drug AdministrationCenter for Drug Evaluation and Research (CDER)Guidance for Industry: Dissolution Testing of Immediate Release Solid Oral Dosage Forms [1997] Available from: https://www.fda.gov/downloads/drugs/guidances/ucm070237.pdfAccessed October 25, 2017
- Barzegar-JalaliMAdibkiaKValizadehHKinetic analysis of drug release from nanoparticlesJ Pharm Pharm Sci200811116717718471378
- SutariyaVBPathakYBiointeractions of NanomaterialsBoca Raton, FLCRC Press2014
- UmairMJavedIRehmanMNanotoxicity of inert materials: the case of gold, silver and ironJ Pharm Pharm Sci201619216118027518167
- ZhaoLZhuBJiaYHouWSuCPreparation of biocompatible carboxymethyl chitosan nanoparticles for delivery of antibiotic drugBiomed Res Int20132013e236469
- FreitasCStability determination of solid lipid nanoparticles (SLN TM) in aqueous dispersion after addition of electrolyteJ Microencapsul199916159719972503
- TangJKongBWuHCarbon nanodots featuring efficient FRET for real-time monitoring of drug delivery and two-photon imagingAdv Mater Deerfield2013254565696574
- LongleyDBHarkinPDJohnstonPG5-fluorouracil: mechanism of action and clinical strategiesNat Rev Cancer2003333033812724731
- SwensonCEHaemmerichDMaulDHKnoxBEhrhartNReedRAIncreased duration of heating boosts local drug deposition during radiofrequency ablation in combination with thermally sensitive liposomes (ThermoDox) in a porcine modelPLoS One20151010e013975226431204