Abstract
Chemotherapy is still one of the main cancer therapy treatments, but the curative effect of chemotherapy is relatively low, as such the development of a new cancer treatment is highly desirable. The gradual maturation of nanotechnology provides an innovative perspective not only for cancer therapy but also for many other applications. There are a diverse variety of nanoparticles available, and choosing the appropriate carriers according to the demand is the key issue. The performance of nanoparticles is affected by many parameters, mainly size, shape, surface charge, and toxicity. Using nanoparticles as the carriers to realize passive targeting and active targeting can improve the efficacy of chemotherapy drugs significantly, reduce the mortality rate of cancer patients, and improve the quality of life of patients. In recent years, there has been extensive research on nanocarriers. In this review, the effects of several major parameters of nanoparticles on their physical and chemical properties are reviewed, and then the recent progress in the application of several commonly used nanoparticles is presented.
Introduction
Due to the insight into the cancer etiology and pathology and the dramatic advances in tumor diagnosis and treatment, cancer mortality has declined in recent years. However, cancer remains one of the most deadly diseases, affecting the quality of life of patients and their families seriously.Citation1 Currently, the main treatments used in tumor therapy are: surgery, chemotherapy, radiotherapy, immunotherapy, biological therapy, and Chinese medicine treatment.Citation2–Citation4 Particularly, chemotherapy is not only one of the main therapy of cancer treatment, but also can be used as an adjuvant therapy combined with other therapies such as surgery and radiotherapy to enhance the curative effect.Citation5–Citation8 But the clinical stage of tumor cannot be reflected accurately via current tumor diagnosis and pre-diagnosis, making correct and timely treatment to the patient very difficult.Citation9 At the meantime, drawbacks of chemotherapy have been revealed gradually: many chemotherapy drugs cannot distinguish the tumor cells and normal cells accurately, so they would attack the body’s normal tissue cells along with tumor cells, thus causing intolerant side effects such as rash, alopecia, severe liver and kidney function decline, cardiotoxicity, secondary infection, bone marrow suppression (which affect the effect of chemotherapy), and the life quality of the patients seriously.Citation10–Citation14 In addition, there is a consensus that multiple drug resistance (MDR) is an important reason to reduce the effect of chemotherapy.Citation15–Citation20 There has been much research on the mechanism of MDR in the recent years,Citation21,Citation22 and scientists are trying to explore the mechanisms to overcome MDR such that chemotherapy drugs could exhibit a higher efficacy.
In recent years, we have profoundly studied the mechanism of tumor invasion and metastasis, as well as the phenotype of tumor cells. Some targeted drugs have also been developed as a specific treatment for cancer chemotherapy.Citation23 However, it is not enough to improve the specificity of chemotherapy drugs alone. On the one hand, because of the presence of several biological barriers, such as the mono-nuclear phagocyte system,Citation24 blood–brain barrier (BBB),Citation25,Citation26 kidney filtration,Citation27,Citation28 and so on, chemotherapy drugs have difficulty in reaching the tumor site and may not reach the effective concentration in some tissues. On the other hand, the role of chemotherapy drugs will also be affected by human metabolism. Meanwhile, when it comes to preventing tumor recurence and MDR, these chemotherapy drugs cannot achieve satisfactory results. To solve these problems, we need new specific anticancer drugs and precise drug delivery systems. It is necessary to seek a highly targeted drug delivery pathway for drugs.Citation29
Nowadays, with an increased understanding of nanomaterials and the application of nanotechnology, the combination of nanotechnology and cancer chemotherapy has attracted wide attention and exploration, which also provides a new promising opportunity for cancer-targeted therapy.Citation30,Citation31 Several nanodrugs have also been used in clinical practice (). Higuchi et al conducted a multicenter study on the efficacy and tolerability of albumin nanoparticles in combination with paclitaxel and carboplatin in the treatment of recurrent and advanced non-small-cell lung cancer in a sample size of 25 patients,Citation32 which indicated nanomedicine may be a promising treatment. At present, nanoparticles that are commonly used include liposomes, polymer nanoparticles, dendrimers, carbon nanotubes, silica nanoparticles, metal nanoparticles, magnetic nanoparticles, and so on. In this paper, the effects of several major parameters of nanoparticles on their physical and chemical properties are reviewed, and we evaluate the newest advances in using nanoparticles as delivery systems for anticancer drugs ().
Table 1 Nanoparticle-based products for cancer approved by FDA and/or EMA
Table 2 The newest advances and targeted sites in using nanoparticles as delivery systems for anticancer drugs
Main parameters of nanoparticles
Size
Size is one of the most important parameters for the selection of nanocarriers. The size of nanoparticles can influence the cellular uptake, and due to the tendency to form clusters in the solution, the size of nanoparticles can also increase.Citation33,Citation34 A recent study on the uptake of oxide nanoparticles by human pneumonocytes in vitro showed that the aggregation effect of nanoparticles is based on their size.Citation35,Citation36 The observation and analysis of three types of magnetic nanoparticles (MNPs) by Ge et al indicated that MNPs with different sizes and surface characters can cause different cell responses, especially in aggregation of MNPs, and the larger particles would produce higher cellular uptakes.Citation37 A similar study on the relationship between the size of ceria nanoparticles and cellular uptake showed a linear relationship within a certain range. The cellular uptake of larger particles was significantly higher than that of smaller particles in the same concentration.Citation35 Bartczak et al reported that the uptake of spherical gold nanoparticles with diameters <50 nm was higher than that of 14 or 74 nm particles.Citation38 The results of Pfeiffer et al suggested that the size of gold and silver nanoparticles is affected by pH of the surrounding environment; when the pH is <7, the aggregation of particles is more obvious, which makes the size and stability of particles to increase significantly.Citation39 In addition, the size of nanoparticles can also affect its removal in vivo. Yu et al’s study on inorganic nanoparticles showed that the smaller the particles, the higher the renal clearance.Citation40 Particle size can also influence the pathway of cellular uptake. It is reported that spherical particles with size ≤200 nm enter the cell through clathrin-mediated cell uptake, which is the major mechanism of these large nanoparticles.Citation41,Citation42
Shape
The shape of the particles is also an important parameter that affects their performance.Citation43,Citation44 Studies have shown that pathways by which particles enter the cells, cycling time, targeting effect, ability to overcome biological barrier, and other properties depend largely on particle shape and size, because these characteristics are likely to influence the particles in the blood transport, especially in small vessels and tumor vessels, and how cells perceive and respond.Citation45 Bartczak et al studied gold nanoparticles of four different shapes: spherical particles, rod-shaped particles, hollow particles, and silica-gold core-shell particles. The results showed that the cellular uptake of particles of different shapes was different; the uptake of spherical particles was the highest and that of hollow particles was the lowest.Citation38 In hydrodynamics, the shape plays an important role in the transport of particles in the fluid and is well recognized.Citation46–Citation48 In particular, the shape and shape correlation factors, such as aspect ratio or geometric structure, can affect the transport properties of particles and the interaction between the cells and the particles.Citation49 Chan reported that compared with rod-shaped gold nanoparticles, spherical gold nanoparticles of similar size had a higher tendency to be taken up by Hela cells, and the cellular uptake of spherical particles of size 14 or 75 nm was 2.75–5 times that of rod-shaped particles of size 75×14 nm.Citation50,Citation51
Surface charge
Surface charges are closely related to various biological performances of the nanoparticles,Citation52,Citation53 such as solubility, biodistribution, stability, cellular uptake, cytotoxicity, and the like. The charge response between particles and cells is an important basis for these biological performances.Citation54 The experimental results of Tang et al showed that when the nanoparticles are dispersed in the culture medium, only positively charged particles can be ingested by the cells; if the particles are connected to the protein, the electrostatic difference between the positively and negatively charged particles can be almost completely eliminated.Citation55 Although protein-coated particles may help identify antigens or receptors, they are not very important in determining particle-to-cell attraction. The adsorption between particle and cell membrane and membrane transport by the surface charge,Citation55–Citation57 mainly because the cell membrane is negatively charged, sometimes with a small amount of positive charge of the patch, therefore, the positively charged particles are more easily than negatively charged or neutral particles by cell membrane adsorption. Graf et al, based on the study of silica nanoparticles, found that high positive charge particles can induce effective cellular internalization, while negatively charged particles and the poly(ethylene glycol) (PEG)-functionalized particles show reduced cellular uptake.Citation58
Toxicity and cytotoxic effects
Inevitably, the nanoparticles are potentially toxic to the human body, therefore nanoparticles must undergo rigorous screening and testing before they can be applied to clinical practice.Citation59–Citation61 Therefore, toxicity is an important consideration for the choice of nanocarriers. More research needs to be carried out in this respect. It is well known that the toxicity of nanoparticles is related to size, shape, concentration.Citation62 Ge et al’s study showed that at lower concentrations (<40 μg/mL), the cytotoxic effect of MNPs was smaller than that at high concentrations (>80 μg/mL).Citation63 The toxicity may be related to the reactions between nanoparticles and biological environment in the human body. In addition, the structure of nanoparticles itself is also an important reason for determining its toxicity, especially the core material. The leakage of toxic substances caused by the decomposition of nanoparticles may be the simplest mechanism for their toxicity.Citation64 The decomposition can be mitigated using an inorganic core or a shell, such as a silicon shell, which is structurally stable on its surface or embedded in a cross-shaped polymer.
These various parameters of nanoparticles are important considerations that determine their properties, which are demonstrated by strong theoretical and experimental results, and are important for the apparent design of nanomedicine. For these above factors, rational use of these parameters and to be modified to change the physicochemical properties of nanoparticles, such as permeability, drug loading, targeting specificity, overcome MDR, low toxicity, and finally realize the optimization control of application of nanomedicine treatment to cancer, that is, to achieve the individual tumor precise treatment requirements.
Main types of nanoparticles
Liposomes
Liposomes are spherical structures consisting of a hydrophilic core and a hydrophobic shell, which enables them to carry both hydrophilic and lipophilic drugs.Citation65,Citation66 The use of liposomes as nanocarriers for chemotherapy has many advantages, such as high drug encapsulation efficiency and drug loading capacity, good stability, specific targeting and lymphatic orientation, sustained release effects, good biocompatibility, low immunogenicity, and less side effects.Citation67,Citation68 It is demonstrated that liposomes can change the transdermal behavior of high-molecule-weight drugs in transdermal preparations.Citation69 The second generation of liposomes (modified by PEG) can effectively avoid the phagocytosis of mononuclear macrophage system in vivo, thereby prolonging the cycle time by using hydrophilic carbohydrates and polymer modifications.Citation70 If the target molecules are further connected to the surface of the liposomes, active targeted transport can be realized, so that the chemotherapeutic drugs are effectively accumulated in the tumor tissue. In addition, liposomes as nanocarriers of chemotherapy drugs have also been used in breast cancer, ovarian cancer, and Kaposi’s sarcoma treatment, and achieved good results.Citation71,Citation72 Recently, the liposomes have been used to target CD45 and/or CD90 of T-cells in vitro and in vivo to realize adoptive immunotherapy.Citation73 Liposomes have recently been used to deliver the drug into the inner ear to overcome the blood-cochlear obstacle and round window membrane, and provide a promising efficacy for inner ear disease.Citation74 Wang et al modified doxorubicin-liposome (DOX-liposome) with polymethacrylate derivatives (DOX-ERLP). The in vitro study on MCF7/Adr cells and liver cancer H22 cells showed DOX-ERLP can cause cancer cell death efficiently,Citation75 and the in vivo study on H22-bearing mice presented an obvious cancer cell apoptosis and necrosis compared with control groups.Citation75 Recently, Truzzi et al focused on the antitumor effects through lymphatic circulation, using solid lipid nanoparticles (SLNs) to encapsulate iron oxide nanoparticles and heparin, to simulate the intestinal lymphatic absorption of oral administration in CaCo-2 cells;Citation76 this approach demonstrates that SLNs can be used as an important route of delivery for oral administration. Zhang et al designed lipid-coated zinc phosphate hybrid nanoparticles coupled with vaccine based on the specific immune response in vivo, in order to transfer peptide and adjuvant to achieve tumor immunotherapy.Citation77 Based on the study of metastatic melanoma model, it was confirmed that the nano-vaccine could enhance the presentation of tumor antigen and induce cytotoxic T lymphocytes (CTL) response, and enhance the monitoring of immune system to tumor growth, metastasis. This nanoparticle-based vaccine can also be applied for the treatment of other tumors and thus has a bright future in the tumor immune treatment.Citation77
Polymeric micelles
Polymeric micelles are a type of micelles composed of block copolymers, which consist of hydrophilic and hydrophobic monomer units.Citation78–Citation80 The core-shell structure of the polymer micelles can be varied by changing the composition of the monomers in the polymer chain.Citation81,Citation82 The core of polymeric micelles is composed of densely packed polymer matrix, which can be filled with hydrophobic drugs.Citation83 This structure enables the polymer micelles to be utilized as suitable and effective nanocarriers. Liu et al designed poly(lactic-co-glycolic acid) nanoparticles modified with transferrin to use it as a carrier for DOX and then targeted the nanomedicine to leukemia K562 cells showing high expression of transferrin receptor; the result showed that the effect of DOX on killing tumor cells was significantly increased.Citation84 Li et al has demonstrated good stability, efficient cellular uptake, and cytotoxicity of DOX-loaded copolymers on Hela cells and COS7 cells by in vitro tests.Citation85 Shaarani et al designed an in vitro drug release study and showed Pluronic polymer (F127) can be used as a good carrier for thymoquinone and some other similar drugs;Citation86 the results suggested that polymeric micelles had the potential to increase the bioavailability of drugs to specific cells. Gao et al designed a micellar system called 7-pep HD micelles, and the in vitro study on MCF/Adr cells and in vivo study on MDR tumors-loaded female nude mice indicated that these biodegradable micelles can effectively target the MDR tumor whose surface overexpresses transferrin and overcomes MDR.Citation87 Gastric cancer tends to overexpress MiR-21; to combat this condition, Wu et al developed a novel nano-delivery system called anti-miRNA oligonucleotide-21-human epidermal growth factor receptor-poly(ethylene glycol)-poly(ε-caprolactone) nanoparticles, and then used it to encapsulate trastuzumab to target gastric cancer cells in vitro and in vivo.Citation88 As a result, gastric cancer cells were more sensitive to drugs, and this demonstrated the enormous potential of antibody-dependent targeted transport. In addition, Nam et al constructed a novel nanocarrier based on the mussel-inspired mineralization using calcium phosphate-assembled polymer nanocarrier to load DOX. A series of analyses and in vitro tests in SCC7 cells proved that the nanocarrier has good stability, high cellular uptake, and low toxicity, and may be used in the delivery of many hydrophobic antineoplastic drugs in the future.Citation89
Dendrimers
Dendrimers are a class of molecules that are polymerized from a large number of branched monomers.Citation90 There are many functional groups on its surface that can be used as chemical reaction sites.Citation91 In general, the dendrimers bind to the drugs mainly by administering the drug into the the dendrimers or connecting the drugs to the functional groups on its surface.Citation92 The use of dendrimers in the drug delivery can effectively prevent the enzymatic hydrolysis of the drug in the body, thus it can extend the drug action time and increase the efficacy. At present, drug-loaded nanoparticles conjugated with paclitaxel have been used in the treatment of tumors. In addition, the nanocarrier prepared by dendrimers shows enhanced permeability and retention effect (), so it can achieve passive targeted administration. Currently, drug-loaded nanoparticles conjugated with paclitaxel have been used in the treatment of tumor.Citation93,Citation94 Nguyen et al studied letrozole-loaded poly(amidoamine) (PAMAM) dendrimer G3.5 coated Hep by an in vitro release test, which showed a potential drug release ability of pH- and redox-responsive PAMAM dendrimers;Citation95 this study indicated that dendrimers can be used as effective nanocarriers. Zarebkohan et al developed a PAMAM-PEG-serine-arginine-leucine (SRL) nanocarrier to target C6 glioma. In in vitro tests, GFP, green fluorescence protein (GFP)-loaded dendrimer showed specific target ability, which indicated PAMAM-PEG-SRL nanocarrier had potential to be used for gene delivery to overcome BBB barrier and brain diseases.Citation96 In addition, it is reported that dendrimers have been applied for psoriasis skin treatment in an in vitro study.Citation97 Soibermon et al used G4-PAMAM dendrimer to deliver dexamethasone for corneal inflammation. An in vivo study in a rat mild alkali burn model showed dendrimer to be a potential drug delivery platform for corticosteroids to address sustained delivery and enhanced bioavailability for eye diseases.Citation98
Figure 1 Enhanced permeability and retention effect. We can see from the picture that big NPs will be removed by some protein once they enter the blood, whereas NPs >100 nm can be trapped inside the solid tumors effectively, so they remain in the blood circulation for a long period. Therefore, the size of nanomedicine is very important.
Abbreviation: NPs, nanoparticles.
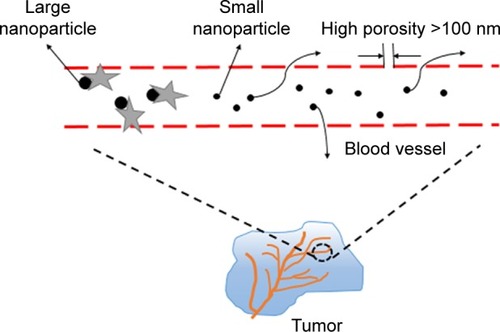
Carbon nanotubes
Carbon nanotubes (CNTs) are made of single or multilayer graphene sheets.Citation99 They are characterized by a large surface area, stable nature, and unique optical, electronic, and other excellent properties.Citation72 CNTs are not only a new carrier for drug transport, but also an important tool for tumor imaging and physical ablation. According to the number of wall layers, CNTs can be divided into single-walled CNTs (SWCNTs) and multiwalled CNTs (MWCNTs).Citation100 Simple CNTs structure cannot be applied to drug delivery directly, only through the peptide, protein, nucleic acid or drug molecules functionalized with low toxicity and nonimmunogenicity, can be used as drug carrier. Most of the current studies on the use of CNTs as drug delivery systems are focused on chemotherapy drugs, such as DOX, paclitaxel, and so on. However, it is necessary to further explore the interaction between CNTs and organism, the interaction mechanism between cells, the biocompatibility, and safety. Dong et al explored the potential of MWCNTs-transactivator of transcription-chitosan (TC) as carriers of DOX against BEL-7402 cells in vitro, which demonstrated that this drug delivery system had good treatment efficacy on cancer and revealed its application potential for cancer therapy.Citation101 It is well known that CNTs play an important role in gene therapy. Huzil et al compared metallic SWCNTs with semiconducting CNTs on siRNA delivery; the results in murine PAM212 keratinocytes showed that metallic SWCNTs can be transferred into the nucleus, while the transport of semiconducting CNTs was limited since they could only only enter the cytoplasm.Citation102 This result suggests that metallic SWCNTs can provide a specific target to the nucleus and has a potential to apply in gene delivery. In addition, Tan et al explored silibinin-loaded CNTs with surface coating surfactant and polymer in mouse fibroblast cells, proved its biocompatibility was improved significantly, and the results also showed the sustained release effect of this drug delivery system.Citation103 Qin et al reported the adverse effects of SWCNTs in an in vitro study, and showed that long-term and repeated intravenous administration of carboxylated SWCNTs can cause persistent accumulation and induce fibrogenesis in rat lungs.Citation104 The results suggest that the issue of long-term toxicity associated with nanoparticles needs to be addressed. The physicochemical properties of nanomaterials play an important role in improving the cell delivery of nanoparticles, and the surface functionalization could change these properties to satisfy the various demands. For instance, Iannazzo et al reported that MWCNTs modified by hydrophilic moieties at free carboxylic groups induce a better water dispersibility, which is relevant for the interaction between biological tissue and nanomaterials.Citation105 Both Bianco et al and Pistone et al highlighted that the biocompatibility, biodegradability, and release ability of CNTs can be modified by the surface functionalization (eg, hydrophilic PEG chain) and the introduction of structural defects.Citation106,Citation107
Mesoporous silica nanoparticles
According to the different preparation processes, mesoporous silica nanoparticles (MSNs) can have a variety of shapes and sizes.Citation108 Gao et al used different sizes of MSNs to carry DOX, and then used them against drug-resistant breast cancer cells (MCF-7/Adr); the results suggested that the antitumor activity of MSNs showed a dependence on the pore size.Citation109 The larger pore size of MSNs can make the cancer cells to absorb DOX faster, so that the rapid accumulation of intracellular drugs plays a strong role in the reversal of MDR. It is clear that MSNs as a carrier for targeted therapy have great potential for overcoming MDR. Su et al reported that red blood cell membrane-coated MSNs loaded with DOX and chlorin e6, which form a new type of nanoparticle, can produce a longer cycle time, good imaging effect, sustained drug release, as well as an obvious anticancer effect in 4T1 breast cancer mouse model.Citation110 In most cases, MSNs are used to encapsulate small hydrophobic drugs, but the recent use of transport gene sequences has become a new research focus.Citation111 Prabhakar et al proved that MSNs modified by polyether imide can deliver siRNA and induce a special interaction between cells and nanoparticles; the results demonstrated high cellular uptake and entry into the endosomes in MDA-MB-231 cells,Citation112 which indicated MSN-based nanocarriers provide a potential therapy for effective and specific gene delivery. Biswas designed a MSN carrier to deliver valsartan (VAL). The MSNs were modified by aminopropyl groups and pH-sensitive polymer Eudragit L 100-55. The in vitro tests showed that this combination of nanoparticles and VAL has a good solubility and higher bioavailability compared to the individual drugs, and the in vivo tests on rats showed this nanodrug can lead to more sustained antihypertensive effects.Citation113 Cheng et al designed Da-tocopheryl polyethylene glycol 1000 succinate-functionalized polydopamine-modified MSNs loaded with DOX, and through the mice model tests proved that they had a strong inhibitory effect on MDR non-small-cell lung cancer cells, which provided a creative idea for the application of nanodrug delivery system to overcome the MDR.Citation114
Gold nanoparticles
Metal nanoparticles are also commonly used in drug delivery systems, of which the more commonly used are gold nanoparticles (GNPs). It is well known that GNPs have good biocompatibility, high binding affinity, high selective target property, and low toxicity compared with other inorganic nanoparticles,Citation115 so GNPs have been widely used and have become a hotspot in the research of new nanocarriers. Cui et al designed PEG-modified GNPs to deliver DOX, and the results showed that the nanomedicine had higher solubility, stability, and dispersibility.Citation116 In addition, GNPs have a unique two-step drug release, respectively, in the lysosome and in the cytoplasm, thus a controlled and sustained release of the drug can be achieved which in turn can improve the antitumor effect of the drugs.Citation116 Hamzawy et al designed to use GNPs and liposome-embedded GNPs (LGNPs) to deliver temozolomide (TMZ). TMZ-loaded GNPs (TGNPs) and TMZ-loaded LGNPs (LTGNPs) were used against urethane-induced lung cancer in BALB/c mice through intratracheal inhalation. The results of this in vivo study showed that both TGNPs and LTGNPs have an improved curative effect and low toxicity, and the synergistic effect produced by LTGNPs can improve the properties of the particles.Citation117 Alalaiwe et al conducted a test in order to evaluate the quantitative oral bioavailability of PEG-coated GNPs in rats by measuring the concentration of gold in the blood, liver, spleen, and kidneys of rats at different time intervals after oral administration of a certain dose of GNPs;Citation118 however, this classical approach to analyze bioavailability needs more studies. In recent years, hyperthermia and photodynamic therapy have received considerable attention, and numerous studies have shown their great potential for cancer treatment. Freitas et al attached two tetracarboxylated zinc phthalocyanines to the surface of gold nanorods to form a new nano-delivery system, and then were made to act on melanotic B16F10 and amelanotic B16G4F melanoma cells. The evaluation showed that more than 90% of the melanoma cells are eliminated. The synergistic effect of hyperthermia and photodynamic therapy in this novel nanodrug delivery system has the potential to effectively treat tumors.Citation119 A novel β-cyclodextrin-{poly(lactide)-poly(2-(dimethylamino) ethylmethacrylate)-poly[oligo(2-ethyl-2-oxazoline) methacrylate]}21 [β-CD-(PLA-PDMAEMA-PEtOxMA)21]-based unimolecular micelle GNPs were designed by Lin et al. They studied the computer topography imaging and drug delivery functions of this nanocarrier in HepG2 tumor cells and NOD/SCID mice model. At the same time, the dissipative particle dynamics (DPD) simulations found that hydrophilic shell with long side chains and high degree of polymerization can increase the stability and the ability of controlled release; thus, the nanocarrier had multiple functions and increased the efficacy of anticancer drugs and played a guiding role for the optimization design of the nanocarrier.Citation120 Recent studies on targeted drug delivery systems based on nanoparticle nanocarriers have utilized enhanced plasmonic nanogrids, enabling Raman spectroscopic evaluation of anticancer drugs and targeting loading and release of ligands. Kurzatkowska et al and Madison et al, respectively, designed and tested a novel spherical GNP-based surface-enhanced Raman scattering (SERS) nanogrid sensor, and their results proved that this new drug delivery system can be applied to the immobilization of anticancer drug and small molecule linkers embedded in the nanogrid sensor.Citation121,Citation122 Similar SERS nanobiosensors were also applied to investigate DNA damage by chemotherapeutic drug DOX.Citation123
A wide variety of nanoparticles have been used extensively, and nanopharmaceuticals have the unlimited potential to be used in cancer diagnosis and treatment. In recent years, the exploration of new nanomedicine and drug carrier materials has been focused, and we are increasingly aware of the importance of cancer treatment and the need for precision treatment. In the context of the widespread need for costly treatment of neoplasms, many families are burdened with huge debts. However, research on new nanomedicine is still on the way forward, because on a large scale, nanotechnology has provided more opportunities to develop more effective personalized treatment platform, which is of extraordinary significance.
Conclusion
Recently, the application of nanoparticles in the treatment of cancer has been extensively increased, which provides a promising approach to overcome the tumor in future. Through a series of theoretical and in vivo/vitro experiments, we can see that the characteristics of nanoparticles can be specially designed according to our needs, which requires a deeper understanding of tumor and nanotechnology. However, the current nanomedicine products are still in clinical trials, and the curative effect is not very precise compared with conventional chemotherapy. Nanomedicine needs further exploration, but this does not prevent nanotechnology from being the mainstay of cancer therapy in the future. Based on the mechanism of action, cell uptake, drug concentration, stability, efficacy, toxicity, and other characteristics, what we should to explore is preparing nanomedicine and regulating its performance, so that we can realize the goal of nanomedicine automation detection, intelligent monitoring, and mass production of the ideal target in the end. First of all, we need to conduct more rigorous research on animal models to verify the feasibility of nanomedicine. For nanodrug safety, especially long-term toxicity, we need to further obtain more data, reduce the nonspecific uptake of nanoparticles (in liver cancer, pancreatic cancer, and other high-risk, microenvironment-related malignant tumors), and should actively study the mechanism of nanotechnology in regulating tumor microenvironment and improving the malignant phenotype and efficacy. Blood system tumors should actively carry out nano-biological treatment and nano-enzyme diagnosis and treatment based on new peptides, antibodies, and aptamers, so as to overcome the MDR of the tumor. In addition, the advantages of nanodrugs compared with traditional chemotherapy should be further proved; besides, the design of personalized nanomedicine should be more reasonable and precise, and its cost needs to be more suitable for the level of public consumption, so that more patients can enjoy the developments in science and technology (). We believe that in the near future, after full research, the wide use of nanodrugs for clinical purposes will become a reality.
Acknowledgments
This work was supported by the Key Medical Projects of Jiangsu Province (BL2014078) and the Key Department of Jiangsu Province (2016–2020).
Disclosure
The authors report no conflicts of interest in this work.
References
- LiuSEpigenetics advancing personalized nanomedicine in cancer therapyAdv Drug Deliv Rev201264131532154322921595
- QiaoSLMaYWangYA general approach of stimuli-induced-aggregation for monitoring tumor therapyACS Nano20171177301731128628744
- ChangAYDaoTGejmanRSA therapeutic T cell receptor mimic antibody targets tumor-associated PRAME peptide/HLA-I antigensJ Clin Invest201712772705271828628042
- StahlMWalzMKRiera-KnorrenschildJPreoperative chemotherapy versus chemoradiotherapy in locally advanced adenocarcinomas of the oesophagogastric junction (POET): Long-term results of a controlled randomised trialEur J Cancer20178118319028628843
- LiangPCChenYCChiangCFDoxorubicin-modified magnetic nanoparticles as a drug delivery system for magnetic resonance imaging-monitoring magnet-enhancing tumor chemotherapyInt J Nanomedicine2016112021203727274233
- LoRVRSchusterMEvaluating hepatitis B virus reactivation during solid tumor chemotherapy: evidence to guide pretreatment hepatitis B screening and prophylaxisAnn Intern Med20161641646526595449
- HendijaniFJavanmardSHDual protective and cytotoxic benefits of mesenchymal stem cell therapy in combination with chemotherapy/radiotherapy for cancer patientsCrit Rev Eukaryot Gene Expr201525320320726558944
- ShahidSReview of hematological indices of cancer patients receiving combined chemotherapy & radiotherapy or receiving radiotherapy aloneCrit Rev Oncol Hematol201610514515527423975
- HahnWCWeinbergRAModelling the molecular circuitry of cancerNat Rev Cancer20022533134112044009
- BoermanLMMaassSvan der MeerPLong-term outcome of cardiac function in a population-based cohort of breast cancer survivors: a cross-sectional studyEur J Cancer201781566528601706
- ZhangYSunMHuangGMaintenance of antiangiogenic and anti-tumor effects by orally active low-dose capecitabine for long-term cancer therapyProc Natl Acad Sci U S A201711426E5226E523528607065
- FanYPLiaoJZLuYQMiR-375 and doxorubicin co-delivered by liposomes for combination therapy of hepatocellular carcinomaMol Ther Nucleic Acids2017718118928624193
- ZhengZXieDSuHTreatment outcome comparisons between exons 19 and 21 EGFR mutations for non-small-cell lung cancer patients with malignant pleural effusion after first-line and second-line tyrosine kinase inhibitorsTumour Biol2017396 1393383453
- FengCRuiMShenHTumor-specific delivery of doxorubicin through conjugation of pH-responsive peptide for overcoming drug resistance in cancerInt J Pharm20175281–232233328606508
- JanuchowskiRSterzynskaKZaorskaKAnalysis of MDR genes expression and cross-resistance in eight drug resistant ovarian cancer cell linesJ Ovarian Res2016916527756418
- HunyadiACsabiJMartinsAMolnarJBalazsATothGBackstabbing P-gp: side-Chain cleaved ecdysteroid 2,3-dioxolanes hyper-sensitize MDR cancer cells to doxorubicin without efflux inhibitionMolecules2017222 pii: E199
- CinciLLuceriCBigagliEDevelopment and characterization of an in vitro model of colorectal adenocarcinoma with MDR phenotypeCancer Med2016561279129127016279
- TsyganovMMFreidinMBIbragimovaMKGenetic variability in the regulation of the expression cluster of MDR genes in patients with breast cancerCancer Chemother Pharmacol201780225126028601971
- TorreggianiERoncuzziLPerutFZiniNBaldiniNMultimodal transfer of MDR by exosomes in human osteosarcomaInt J Oncol201649118919627176642
- YeSZhangJShenJNVP-TAE684 reverses multidrug resistance (MDR) in human osteosarcoma by inhibiting P-glycoprotein (PGP1) functionBr J Pharmacol2016173361362626603906
- KimMSHaneyMJZhaoYDevelopment of exosome-encapsulated paclitaxel to overcome MDR in cancer cellsNanomedicine-UK2016123655664
- LiXPanLShiJNuclear-targeting MSNs-based drug delivery system: global gene expression analysis on the MDR-overcoming mechanismsAdv Healthc Mater20154172641264826450832
- MudshingeSRDeoreABPatilSBhalgatCMNanoparticles: emerging carriers for drug deliverySaudi Pharm J201119312914123960751
- LiuZLiXXiuBA novel and simple preparative method for uniform-sized PLGA microspheres: preliminary application in antitubercular drug deliveryColloids Surf B Biointerfaces201614567968727289309
- TosiUMarnellCSChangRAdvances in molecular imaging of locally delivered targeted therapeutics for central nervous system tumorsInt J Mol Sci2017182 pii: E351
- Martin-RapunRDe MatteisLAmbrosoneAGarcia-EmbidSGutierrezLde la FuenteJMTargeted nanoparticles for the treatment of Alzheimer’s diseaseCurr Pharm Des201723131927195228025949
- BidwellGRMahdiFShaoQA kidney-selective biopolymer for targeted drug deliveryAm J Physiol Renal Physiol20173121F54F6427784692
- GustafsonHHHolt-CasperDGraingerDWGhandehariHNanoparticle uptake: the phagocyte problemNano Today201510448751026640510
- Available from: https://www.ncbi.nlm.nih.gov/
- DaSPRamosMABonifacioBVNanotechnological strategies for vaginal administration of drugs – a reviewJ Biomed Nanotechnol20141092218224325992455
- YouCWuHWangMA strategy for photothermal conversion of polymeric nanoparticles by polyanilin for smart control of targeted drug deliveryNanotechnology2017281616510228257002
- HiguchiMTakagiHOwadaYEfficacy and tolerability of nanoparticle albumin-bound paclitaxel in combination with carboplatin as a late-phase chemotherapy for recurrent and advanced non-small-cell lung cancer: a multi-center study of the Fukushima lung cancer association group of surgeonsOncol Lett20171364315432128599432
- BenneNvan DuijnJKuiperJJiskootWSlutterBOrchestrating immune responses: how size, shape and rigidity affect the immunogenicity of particulate vaccinesJ Control Release201623412413427221070
- SilvaALSoemaPCSlutterBOssendorpFJiskootWPLGA particulate delivery systems for subunit vaccines: linking particle properties to immunogenicityHum Vaccin Immunother20161241056106926752261
- LimbachLKLiYGrassRNOxide nanoparticle uptake in human lung fibroblasts: effects of particle size, agglomeration, and diffusion at low concentrationsEnviron Sci Technol200539239370937616382966
- KyrychenkoAPaskoDAKaluginONPoly(vinyl alcohol) as a water protecting agent for silver nanoparticles: the role of polymer size and structurePhys Chem Chem Phys201719138742875628217797
- GeYZhangYXiaJEffect of surface charge and agglomerate degree of magnetic iron oxide nanoparticles on KB cellular uptake in vitroColloids Surf B Biointerfaces200973229430119564099
- BartczakDMuskensOLNittiSSanchez-ElsnerTMillarTMKanarasAGInteractions of human endothelial cells with gold nanoparticles of different morphologiesSmall20128112213022102541
- PfeifferCRehbockCHuhnDInteraction of colloidal nanoparticles with their local environment: the (ionic) nanoenvironment around nanoparticles is different from bulk and determines the physicochemical properties of the nanoparticlesJ R Soc Interface20141196 20130931
- YuMZhengJClearance pathways and tumor targeting of imaging nanoparticlesACS Nano2015976655667426149184
- HerdHDaumNJonesATHuwerHGhandehariHLehrCMNanoparticle geometry and surface orientation influence mode of cellular uptakeACS Nano2013731961197323402533
- BoualleguiYBenYRTurkiFMezniAOueslatiREffect of exposure time, particle size and uptake pathways in immune cell lysosomal cytotoxicity of mussels exposed to silver nanoparticlesDrug Chem Toxicol201716
- CulverKSShinYJRotzMWMeadeTJHersamMCOdomTWShape-dependent relaxivity of nanoparticle-based T1 magnetic resonance imaging contrast agentsJ Phys Chem C Nanomater Interfaces201612038221032210928008338
- RampersaudSFangJWeiZThe effect of cage shape on nanoparticle-based drug carriers: anticancer drug release and efficacy via receptor blockade using dextran-coated iron oxide nanocagesNano Lett201616127357736327960523
- MoghimiSMHunterACMurrayJCLong-circulating and target-specific nanoparticles: theory to practicePharmacol Rev200153228331811356986
- ShindePVCampbellCJYunYHSlackSMGoetzDJParticle diameter influences adhesion under flowBiophys J20018041733174311259287
- LamprechtASchaferULehrCMSize-dependent bioadhesion of micro- and nanoparticulate carriers to the inflamed colonic mucosaPharm Res200118678879311474782
- ChatterjeeTChatterjeeBKSahaTHoqueKMChakrabartiPStructure and function of Vibrio cholerae accessory cholera enterotoxin in presence of gold nanoparticles: dependence on morphologyBiochim Biophys Acta201718615 Pt A97798628215703
- NishiyamaNNanomedicine: nanocarriers shape up for long lifeNat Nanotechnol20072420320418654260
- JiangWKimBYRutkaJTChanWCNanoparticle-mediated cellular response is size-dependentNat Nanotechnol20083314515018654486
- ChithraniBDChanWCElucidating the mechanism of cellular uptake and removal of protein-coated gold nanoparticles of different sizes and shapesNano Lett2007761542155017465586
- PengYLuBWangNLiLChenSImpacts of interfacial charge transfer on nanoparticle electrocatalytic activity towards oxygen reductionPhys Chem Chem Phys201719149336934828165087
- MouYXingYRenHThe effect of superparamagnetic iron oxide nanoparticle surface charge on antigen cross-presentationNanoscale Res Lett20171215228102523
- PittellaFZhangMLeeYEnhanced endosomal escape of siRNA-incorporating hybrid nanoparticles from calcium phosphate and PEG-block charge-conversional polymer for efficient gene knockdown with negligible cytotoxicityBiomaterials201132113106311421272932
- TangJLiLHowardCBMahlerSMHuangLXuZPPreparation of optimized lipid-coated calcium phosphate nanoparticles for enhanced in vitro gene delivery to breast cancer cellsJ Mater Chem B Mater Biol Med20153336805681227213045
- HolgateSTExposure, uptake, distribution and toxicity of nanomaterials in humansJ Biomed Nanotechnol20106111920499827
- LeonenkoZFinotEAmreinMAdhesive interaction measured between AFM probe and lung epithelial type II cellsUltramicroscopy200710710–1194895317561346
- GrafCGaoQSchutzISurface functionalization of silica nanoparticles supports colloidal stability in physiological media and facilitates internalization in cellsLangmuir201228207598761322524440
- ColbyAHOberliesNHPearceCJHerreraVLColsonYLGrinstaffMWNanoparticle drug-delivery systems for peritoneal cancers: a case study of the design, characterization and development of the expansile nanoparticleWiley Interdiscip Rev Nanomed Nanobiotechnol201793 Epub 2017 Feb 9
- TomankovaKHorakovaJHarvanovaMCytotoxicity, cell uptake and microscopic analysis of titanium dioxide and silver nanoparticles in vitroFood Chem Toxicol20158210611525846500
- TomankovaKHorakovaJHarvanovaMReprint of cytotoxicity, cell uptake and microscopic analysis of titanium dioxide and silver nanoparticles in vitroFood Chem Toxicol201585203026518667
- NamdariMEatemadiASoleimaninejadMHammedATA brief review on the application of nanoparticle enclosed herbal medicine for the treatment of infective endocarditisBiomed Pharmacother20178732133128064105
- GeYZhangYXiaJEffect of surface charge and agglomerate degree of magnetic iron oxide nanoparticles on KB cellular uptake in vitroColloids Surf B Biointerfaces200973229430119564099
- PelazBCharronGPfeifferCInterfacing engineered nanoparticles with biological systems: anticipating adverse nano-bio interactionsSmall201399–101573158423112130
- TrojerMALiYWallinMHolmbergKNydenMCharged microcapsules for controlled release of hydrophobic actives Part II: surface modification by LbL adsorption and lipid bilayer formation on properly anchored dispersant layersJ Colloid Interface Sci201340981723928487
- LiuDChenLJiangSFormulation and characterization of hydrophilic drug diclofenac sodium-loaded solid lipid nanoparticles based on phospholipid complexes technologyJ Liposome Res2014241172624236407
- ZhangYXuanSOwoseniOAmphiphilic polypeptoids serve as the connective glue to transform liposomes into multilamellar structures with closely spaced bilayersLangmuir201733112780278928248521
- GaoJWangZLiuHWangLHuangGLiposome encapsulated of temozolomide for the treatment of glioma tumor: preparation, characterization and evaluationDrug Discov Ther20159320521226193943
- MohammedMIMakkyAMTeaimaMHAbdellatifMMHamzawyMAKhalilMATransdermal delivery of vancomycin hydrochloride using combination of nano-ethosomes and iontophoresis: in vitro and in vivo studyDrug Deliv20162351558156425726990
- LiYNGFRecent progress in doxorubicin nano-drug delivery systems for reserving multidrug resisitance2014113177181
- YangFJinCJiangYLiposome based delivery systems in pancreatic cancer treatment: from bench to bedsideCancer Treat Rev201137863364221330062
- XinliDHZSApplications of nanocarriers with tumor molecular targeted in chemotherapyChemistry2012757621627
- ZhengYTangLMabardiLKumariSIrvineDJEnhancing adoptive cell therapy of cancer through targeted delivery of small-molecule immunomodulators to internalizing or noninternalizing receptorsACS Nano20171133089310028231431
- MohammadianFEatemadiADaraeeHInner ear drug delivery using liposomesCell Mol Biol (Noisy-le-grand)20176312833
- WangWShaoAZhangNFangJRuanJJRuanBHCationic polymethacrylate-modified liposomes significantly enhanced doxorubicin delivery and antitumor activitySci Rep201774303628225062
- TruzziEBongioCSacchettiFSelf-assembled lipid nanoparticles for oral delivery of heparin-coated iron oxide nanoparticles for theranostic purposesMolecules2017226 pii: E963
- ZhuangXWuTZhaoYLipid-enveloped zinc phosphate hybrid nanoparticles for codelivery of H-2K(b) and H-2D(b)-restricted antigenic peptides and monophosphoryl lipid A to induce antitumor immunity against melanomaJ Control Release2016228263726921522
- ParveenSuphiyaMRMMNanoparticles: a boon to drug delivery, therapeutics, diagnostics and imagingNanomedicine-UK20128147166
- LukowiakMCThotaBNHaagRDendritic core-shell systems as soft drug delivery nanocarriersBiotechnol Adv2015336 Pt 31327134125868804
- RasolonjatovoBGomezJPMemeWPoly(2-methyl-2-oxazoline)-b-poly(tetrahydrofuran)-b-poly(2-methyl-2-oxazoline) amphiphilic triblock copolymers: synthesis, physicochemical characterizations, and hydrosolubilizing propertiesBiomacromolecules201516374875625517924
- GargSMVakiliMRLavasanifarAPolymeric micelles based on poly(ethylene oxide) and alpha-carbon substituted poly(varepsilon-caprolactone): an in vitro study on the effect of core forming block on polymeric micellar stability, biocompatibility, and immunogenicityColloids Surf B Biointerfaces201513216117026037706
- ZhangYRenTGouJStrategies for improving the payload of small molecular drugs in polymeric micellesJ Control Release201726135236628163211
- GaucherGMarchessaultRHLerouxJCPolyester-based micelles and nanoparticles for the parenteral delivery of taxanesJ Control Release2010143121219925835
- LiuPZhangHWuXTf-PEG-PLL-PLGA nanoparticles enhanced chemosensitivity for hypoxia-responsive tumor cellsOnco Targets Ther201695049505927574446
- LiYMChangXPChengYJChenSHeFZhuoRXMercaptan acids modified amphiphilic copolymers for efficient loading and release of doxorubicinColloids Surf B Biointerfaces201715322022828258030
- ShaaraniSHamidSSMohdKNThe influence of Pluronic F68 and F127 nanocarrier on physicochemical properties, in vitro release, and antiproliferative activity of Thymoquinone drugPharmacognosy Res201791122028250648
- GaoWYeGDuanXYangXYangVCTransferrin receptor-targeted pH-sensitive micellar system for diminution of drug resistance and targetable delivery in multidrug-resistant breast cancerInt J Nanomedicine2017121047106428223798
- WuFLZhangJLiWEnhanced antiproliferative activity of antibody-functionalized polymeric nanoparticles for targeted delivery of anti-miR-21 to HER2 positive gastric cancerOncotarget2017840671896720228978026
- NamHYMinKHKimDEChoiJRLeeHJLeeSCMussel-inspired poly(L-DOPA)-templated mineralization for calcium phosphate-assembled intracellular nanocarriersColloids Surf B Biointerfaces201715721522228599182
- Available from: http://eprints.unife.it./
- FurerVLVandyukovAEMajoralJPCaminadeAMKovalenkoVISpectroscopic and molecular structure investigation of the phosphorus-containing G′2 dendrimer with terminal aldehyde groups using DFT methodSpectrochim Acta A Mol Biomol Spectrosc201513722022625222317
- LiuMFrechetJMDesigning dendrimers for drug deliveryPharm Sci Technolo Today199921039340110498919
- MishraBPatelBBTiwariSColloidal nanocarriers: a review on formulation technology, types and applications toward targeted drug deliveryNanomedicine-UK201061924
- YangWChengYXuTWangXWenLPTargeting cancer cells with biotin-dendrimer conjugatesEur J Med Chem200944286286818550227
- NguyenTLNguyenTHNguyenCKNguyenDHRedox and pH responsive poly (amidoamine) dendrimer-heparin conjugates via disulfide linkages for letrozole deliveryBiomed Res Int20172017858921228246606
- ZarebkohanANajafiFMoghimiHRHemmatiMDeevbandMRKazemiBSRL-coated PAMAM dendrimer nano-carrier for targeted gene delivery to the glioma cells and competitive inhibition by lactoferrinIran J Pharm Res201615462964028243262
- GungorSRezigueMNanocarriers mediated topical drug delivery for psoriasis treatmentCurr Drug Metab201718545446828228078
- SoibermanUKambhampatiSPWuTSubconjunctival injectable dendrimer-dexamethasone gel for the treatment of corneal inflammationBiomaterials2017125385328226245
- SanginarioAMiccoliBDemarchiDCarbon nanotubes as an effective opportunity for cancer diagnosis and treatmentBiosensors (Basel)201771 pii: E9
- Du ZhuoLiGongkeZSApplication of carbon nanotubes in sample pretreatmentChemistry201103201208
- DongXSunZWangXZhuDLiuLLengXSimultaneous monitoring of the drug release and antitumor effect of a novel drug delivery system-MWCNTs/DOX/TCDrug Deliv201724114315128156171
- HuzilJTSaliajEIvanovaMVSelective nuclear localization of siRNA by metallic versus semiconducting single wall carbon nanotubes in keratinocytesFuture Sci OA201513O17
- TanJMKarthivashanGGaniSAFakuraziSHusseinMZIn vitro drug release characteristic and cytotoxic activity of silibinin-loaded single walled carbon nanotubes functionalized with biocompatible polymersChem Cent J2016108128028386
- QinYLiSZhaoGLong-term intravenous administration of carboxylated single-walled carbon nanotubes induces persistent accumulation in the lungs and pulmonary fibrosis via the nuclear factor-kappa B pathwayInt J Nanomedicine20171226327728115845
- IannazzoDPistoneAGalvagnoSSynthesis and anti-HIV activity of carboxylated and drug-conjugated multi-walled carbon nanotubesCarbon201582548561
- BiancoAKostarelosKPratoMMaking carbon nanotubes biocompatible and biodegradableChem Commun (Camb)20114737101821018821776531
- PistoneAIannazzoDAnsariSTunable doxorubicin release from polymer-gated multiwalled carbon nanotubesInt J Pharm20165151–2303627720871
- QinYLiMQiuQPiJTChenHYThe application of nanoparticles drug system in cancer multidrug resistanceProgress in Modern Biomedicine20142855995600
- GaoYChenYJiXControlled intracellular release of doxorubicin in multidrug-resistant cancer cells by tuning the shell-pore sizes of mesoporous silica nanoparticlesACS Nano20115129788979822070571
- SuJSunHMengQZhangPYinQLiYEnhanced blood suspensibility and laser-activated tumor-specific drug release of theranostic mesoporous silica nanoparticles by functionalizing with erythrocyte membranesTheranostics20177352353728255347
- MamaevaVSahlgrenCLindenMMesoporous silica nanoparticles in medicine – recent advancesAdv Drug Deliv Rev201365568970222921598
- PrabhakarNZhangJDesaiDStimuli-responsive hybrid nanocarriers developed by controllable integration of hyperbranched PEI with mesoporous silica nanoparticles for sustained intracellular siRNA deliveryInt J Nanomedicine2016116591660827994460
- BiswasNModified mesoporous silica nanoparticles for enhancing oral bioavailability and antihypertensive activity of poorly water soluble valsartanEur J Pharm Sci20179915216027993684
- ChengWLiangCXuLTPGS-functionalized polydopamine-modified mesoporous silica as drug nanocarriers for enhanced lung cancer chemotherapy against multidrug resistanceSmall20171329
- VandoorenJOpdenakkerGLoadmanPMEdwardsDRProteases in cancer drug deliveryAdv Drug Deliv Rev20169714415526756735
- CuiTLiangJJChenHThe performance of doxorubicin-conjugated gold nanoparticles: Regulation of drug locationACS Appl Mater Interfaces20179108569858028218512
- HamzawyMAAbo-YoussefAMSalemHFMohammedSAAntitumor activity of intratracheal inhalation of temozolomide (TMZ) loaded into gold nanoparticles and/or liposomes against urethane-induced lung cancer in BALB/c miceDrug Deliv201724159960728240047
- AlalaiweARobertsGCarpinonePMunsonJRobertsSInfluence of PEG coating on the oral bioavailability of gold nanoparticles in ratsDrug Deliv201724159159828222611
- FreitasLFHamblinMRAnzengruberFZinc phthalocyanines attached to gold nanorods for simultaneous hyperthermic and photodynamic therapies against melanoma in vitroJ Photochem Photobiol B201717318118628595072
- LinWYaoNQianLpH-responsive unimolecular micelle-gold nanoparticles-drug nanohybrid system for cancer theranosticsActa Biomater20175845546528583900
- KurzatkowskaKSantiagoTHepelMPlasmonic nanocarrier grid-enhanced Raman sensor for studies of anticancer drug deliveryBiosens Bioelectron20179178078728142123
- MadisonSMariaHControlled release of targeted anti-leukemia drugs azacitidine and decitabine monitored using surface-enhanced Raman scattering (SERS) spectroscopyMediterranean J Chemistry201764125132
- IlkhaniHHughesTLiJZhongCJHepelMNanostructured SERS-electrochemical biosensors for testing of anticancer drug interactions with DNABiosens Bioelectron20168025726426851584